Non-nutritive sweeteners and their impacts on the gut microbiome and host physiology
- Department of Nutrition, University of Nevada, Reno, NV, United States
Non-nutritive sweeteners (NNS) are broadly incorporated into foods, especially those representing a growing share of the beverage market. NNS are viewed as a noncaloric and desirable alternative to sugar-based sweeteners and are thought to contribute to reducing overall caloric intake. While these compounds have been studied extensively and have long been considered inert, new research has presented a different view and raises new questions about the effects of NNS on human physiology. Namely, the influence on glucose responses, the gastrointestinal epithelium, and the gut microbiome. As the gut microbiome is now recognized as a major mediator of human health and perturbations to this community are generally associated with negative health trajectories or overt disease, interactions between NNS and the gut microbiome are of increasing interest to clinicians and researchers. Several NNS compounds are now hypothesized to affect human physiology by modulating the gut microbiome, though the mechanism for this action remains unclear. The purpose of this review is to discuss the history and current knowledge of NNS, their reported utility and effects on host physiology and the gut microbiome, and describes a model for investigating the underlying mechanism behind reported effects of NNS on the gut microbiome.
Introduction
The increased abundance of processed foods among the diets of industrialized nations has led to the overconsumption of non-essential nutrients, such as added or free sugars. Processed food formulations often prioritize consumer perceptions of organoleptic properties of a product, leading many of these goods to contain high amounts of salt and/or sugar. Consequently, overconsumption of added sugar and salt has become a global concern (1–3). To address this concern regarding the consumption of common sweeteners (e.g., sucrose, glucose, natural sugar syrups, and high fructose corn syrup) and their known adverse health effects, food and beverage products now often use non-nutritive Sweeteners (NNS) as replacements for sugar sweeteners.
The use of NNS as sweetening agents and food additives are relatively novel to the human diet and provide a broad range of relative sweetness (4, 5). Overall NNS consumption has almost doubled since the approval of saccharin, the first NNS, however the consensus of safety and efficacy is still debated (6, 7) and NNS remain a controversial topic in food regulatory frameworks worldwide (4, 8–10). Still, their use is widespread as NNS provide a tractable approach to reduce caloric intake, sugar content, and cost (6, 11, 12). The U.S. Food and Drug Administration (FDA), in addition to several international food safety organizations, have assessed numerous NNS as safe for human consumption with no causal relationship between cancer or other health-related issues if consumed within the Acceptable Daily Intake (ADI) (13–15). However, in contrast to the potential benefits of replacing sugar sweeteners with NNS, recent work has implicated the consumption of NNS as being associated with impacts on human physiological responses such as glucose intolerance, as well as cardiovascular disease (16–19).
The gut microbiome has been identified as a major mediator of several physiological processes and communication pathways (e.g., the gut-brain axis) and has been implicated in the modification of xenobiotics (e.g., pharmaceutical drugs) (20–22), and there is now evidence that a similar fate may exist for NNS in the gut (23, 24). Several studies have sought to identify the associations of NNS intake with impacts on human physiology and view the gut microbiome as a mediator of potential effects of NNS on the host (25–27), which raises new questions regarding the safety profile of NNS and whether their interactions with the host and their microbiome are fully understood.
Overall, while the health risks associated with obesity and excess sugar consumption are well known (28–30), there is limited understanding as to whether or how these NNS affect human physiology, whether they may act directly on the host to do so, or if they act indirectly via modulation of the gut microbiome. This review will discuss and summarize the current literature regarding NNS and their chemistry, evidence of physiological impacts on the host, and their potential impact of NNS on the gut microbiome.
The history of NNS, their chemistry, and use
Non-nutritive sweeteners are perceived as a safe and affordable alternative to sugar sweetened beverages (SSBs), particularly in overweight and obese individuals with the goal of limiting caloric intake as well as improving weight management (31–34). Therefore, the prevalence of non-nutritive sweeteners in common diets continues to grow (9, 35). Currently, the US FDA has approved six NNS for use as food additives in the US. These include acesulfame K, advantame, aspartame, sucralose, neotame, and saccharin (Table 1). In addition to two naturally derived zero calorie sweeteners that are Generally Recognized as Safe (GRAS) by the FDA; stevioside and rebaudioside A from the extracts of the stevia plant (Stevia rebaudiana), and monkfruit extract (Siraitia grosernorii) (42, 43). Though these compounds share an effect of perceived sweetness, their chemical composition and the intensity of their perceived sweetness differs significantly.
Despite differences in chemical composition, the consensus of safety and regulatory approvals for NNS has led dietary recommendations and health organizations to encourage their use and the suggested beneficial outcomes – primarily as sugar substitutes with little to no caloric cost (44–46). The Academy of Nutrition and Dietetics (AND) has previously reviewed the techniques and evidence as favorable for use in adults with Type 1 and 2 Diabetes (47), if amounts of consumed NNS do not exceed that of the FDA proposed ADI (48). In addition, the AND supports the use of NNS as a strategy for various diet/health concerns including the limiting of carbohydrate and energy intake as well as blood glucose and/or weight management (49).
Effects of sweeteners on host physiology
Non-nutritive sweeteners carry the advantage over typical sweeteners due to their presumed zero-to-negligible caloric load, as well as producing no direct glycemic effect (48). Despite their extensive usage, the supposed benefits have yet to be established, specifically with reducing body weight. The effects of NNS consumption in relation to body weight management have been largely divided over the main findings and randomized controlled trials in humans are limited (50). Several observational studies have reported weight gain (17, 51, 52), conflicting reports of weight loss (34, 53, 54), or negligible effects on weight (50, 55, 56). A key drawback to many of these studies is determining directionality of the interactions as well as accurate estimates of NNS intake, as these observational studies do not demonstrate causality (44, 57).
A recent meta-analysis of 15 randomized control trials (RCTs) does suggest that there is a modest effect of weight loss in participants who substituted NNS for regular calorie foods (32), which indicates that NNS could be a useful tool to strengthen compliance of weight loss or weight management plans (6). However, the data of the nine reviewed prospective cohort studies suggested a small positive association between NNS intake and body mass index (BMI) whereas evidence from short term observational studies generally have found incomplete energy compensation when NNS was used as a substitution (32). Critically, NNS appear to pose no benefit for weight loss or minimizing weight gain without a restriction of energy intake (6, 58) and are effective only if used as a replacement to caloric sweeteners while also maintaining a caloric deficit (58). Thus, if NNS are used as a substitute to higher calorie alternatives, they do have the potential to aid in weight management (6, 54, 59), though there is no influence of NNS on the hormone incretin in relation to blood glucose, appetite, or weight gain (33). As a result of these varying results the effects of NNS on body weight management, the American Heart Association and American Diabetes Association have both concluded there to be insufficient information to say whether using NNS has the desired impact to reduce body weight (44).
Similar conflicts in the literature have been observed in relation to the influence of NNS on feeding behavior and metabolism (8). Previous work considered these sweeteners predominantly inert in relation to effects on glucose homeostasis because they do not evoke a post-prandial response seen with caloric sweeteners (10, 60). However, NNS are thought to modify energy balance and metabolic functions by means of both central and peripheral mechanisms (61). A strong current theory regarding the physiological interactions is that NNS may impede learned responses regarding glucose control and energy balance (61–65). The cephalic response is considered an innate and learned physiological response to the thought and anticipation of food entering and being digested within the gastrointestinal tract. The major end result is the stimulation of the vagus nerve thereby producing a cascade of actions within the peripheral nervous system (including increased salivation, gastric acid secretion, as well as exocrine and endocrine pancreatic secretions) (66). With continuous exposure to certain foods, the body quickly learns how to respond to an influx of nutrients and for determining satiation. Therefore in the case of sweet compounds entering the oral cavity, like sucrose, a conditioned response is produced looking to predict the caloric uptake as well as compensate with downstream effects to the gut hormones insulin and glucagon-like-peptide-1 (GLP-1) (67). Recent findings report both sucrose and sucralose produce similar stimulations of primary taste pathways (68). Yet, sucrose produces a stronger response and consequently initiates a dopaminergic effect (68) that can be distinguished by the brain, even though the conscious mind could differentiate between the compounds (68). Additionally, saccharin has also been reported to increase insulin levels via the cephalic phase in healthy adult humans (69) and while taste receptors can predict caloric consequences, saccharin has been shown to interfere with this ability in foods that tasted sweet (62) and other NNS have been shown to induce insulin release as well (67, 69).
The physiological responses to NNS have been further explored in other work. When comparing the effects of sucralose compared to water consumption in obese, insulin sensitive participants who were atypical consumers of NNS, researchers found sucralose increased plasma glucose and insulin levels, as well as an increase in insulin secretion and decrease in clearance compared to the controls who consumed water. However, there were no differences in several other factors of glycemic response including GLP-1, beta cell sensitivity and glucose dependent insulinotropic peptide (GIP) (70). These findings raise the question of whether non-nutritive sweeteners produce significant effects in obese, insulin sensitive populations for whom glucose levels must be tightly regulated. Finally, while NNS have been shown to bind to sweet-taste receptors and induce GLP-1 release (related to glucose homeostasis) in rodent models and other studies (36, 71), this has not been reproduced with human subjects (71).
The widespread use of NNS has been contingent on the negligible caloric cost as well as no influence on post-prandial responses to appetite and energy, which is essential for both diabetic patients and overweight/obese individuals in search of added sugar replacements. To date, the current findings provide mixed results on the effect of weight management, as well as the effects of NNS on insulin, glucose intolerance, and GLP-1 responses. However, studies examining humans for these responses have not stratified participants by gut microbiome composition, which may explain some of the variability of responses among individuals.
Evidence for NNS and gut microbiome interactions
The gastrointestinal tract (GIT) provides for the breakdown of most dietary components and enables efficient uptake of nutrients to meet the nutritional needs of the host (72). In contrast, the gut microbiome is a community of microbes which accesses dietary components during and after digestion and absorption and contributes to the fermentation of dietary components such as fiber (72). In doing so, these microbes facilitate the capture of energy from dietary components which the host is unable to access and facilitates the production of additional nutrients (e.g., vitamins) consequently accessible by the host (72). The influence of this community on host development, nutrition, and health is now being understood (72, 73), as more examples of ancient associations between humans and specific gut microbes are identified (74, 75). These ancient associations are significant relationships between humans and our gut microbes as these microbes were recruited and maintained over millennia to perform key functions in the gut (76).
One of the reasons that vertebrates have recruited these gut microbes is that they collectively represent a significant expansion of the genome in terms of enzymatic and metabolic potential by orders of magnitude (77), facilitating the consumption of diets that would otherwise be toxic (78) or completely indigestible (72). Recent work has also identified strong evidence for the impact of this community on the bioavailability and breakdown of xenobiotic compounds (79), and there has been significant interest in understanding how food additives interact with the gut microbiome (80). While some examples have been identified among food additives such as trehalose, whose introduction into the food supply spawned the emergence of pathogenic and trehalose-consuming Clostridioides difficile (81), there are few comparable studies examining the impact of other food additives on the gut microbiome.
As NNS are among the most common food additives, whose use in food is relatively recent (82), there has been significant interest in understanding the potential of these compounds to alter gut microbiomes (25, 61, 83, 84). Complicating this effort is the relatively limited, but growing, understanding of the specific enzymatic functions found within the human gut (77) and the diversity of NNS chemical structures (Table 1). Given this challenge, some researchers have tested the impacts of selected NNS on individual members of the gut microbiome, but this has been limited primarily to Escherichia coli (85–90).
As the rapid advance of sequencing technologies and analytic software has progressed and costs to generate and analyze the gut microbiome has diminished, there has been an explosion of interest in understanding the gut microbiome and its interactions with the host. There has been some consistency across studies examining gut microbiome responses to the introduction of NNS in rodent models. These studies have spanned the NNS commonly used in foods; saccharin (25, 91), Acesulfame K (92), sucralose (27, 93), rebaudioside A (94, 95), and aspartame (26).
Collectively, studies investigating the impact of NNS on the gut microbiome conclude that while the community may be altered in response to NNS exposure, differences are observed across studies (Table 2), which complicates specific interpretation and direct comparisons while also raising questions as to a potential mechanism of action behind these responses. These findings collectively represent a body of evidence supporting the potential for NNS to alter the gut microbiome, though not all studies have come to this conclusion (104). Notably, these findings have been performed across a variety of murine models providing consistent evidence for the impact of NNS on the gut microbiome, though not all studies show consistent specific changes within the gut microbiome (Table 2). One of the more consistent findings among these studies, however, has been a depletion of Akkermansia muciniphilia when exposed to NNS (saccharin, Acesulfame K, and sucralose) in both adult and infant mice (25, 98). When considering the reported effects of NNS on human health parameters like glucose homeostasis (16, 25), several studies in humans and mouse models report that the depletion of Akkermansia is associated with increased glucose intolerance (116–118) and mechanistic experiments have identified the secretion of a protein, P9, by Akkermansia that induces GLP1 secretion and improves glucose homeostasis in mouse models of obesity and diabetes (119–121). If Akkermansia is indeed depleted by NNS consumption, then given the variable distribution of Akkermansia among humans (122), this may explain some of the variability with respect to impacts of NNS on glucose tolerance. Further, a small human saccharin challenge study reported that the gut microbiome composition differentiated “responders,” who developed impaired glucose tolerance after consuming the maximum ADI of saccharin for 7 days, and “non-responders” who did not develop insulin resistance (25), which suggests that inter-individual variability in gut microbiome composition may mediate the effects of NNS on host glucose responses. Though this was a small study (N = 7), fecal samples from “responders” post-saccharin consumption could recapitulate the glucose intolerance phenotype when transplanted to germ free mice, while fecal samples from the same individuals pre-saccharin consumption did not produce the same effect (25).
Other work has sought to examine the inter-generational impact of NNS on offspring, as NNS can be detected in milk (39). Low dose aspartame (5–7 mg/kg) and stevia (2–3 mg/kg) was associated with alterations to adiposity, insulin sensitivity, glucose tolerance, as well as the mesolimbic reward pathway in pregnant rats (101). While there were minimal differences observed within the fecal microbiota of these animals, relative to the control group, Clostridium leptum is noted to be more abundant within both groups of dams and offspring receiving sweetener supplementation, but C. leptum was not carried over to the offspring of dams receiving water alone (101). There was also an enrichment of the family Porphyromonadaceae within the offspring of rats fed aspartame or stevia. Importantly, the transplant of fecal samples from the offspring of sweetener supplemented dams to germ free mice produced similar physiological effects observed among the NNS-supplemented animals including increased body weight, percent fat mass, and a trend toward reduced glucose tolerance (101). These ex-germ-free mice also displayed an increased abundance of Porphyromonadaceae, comparable to the offspring exposed to aspartame and stevia within the mother's diet. Importantly, this work highlights the impact of these microbiome alterations on host glucose responses and demonstrated that these changes, which resulted from direct exposure to NNS, could impair health in germ-free animals receiving this microbiome composition without the NNS itself (101).
In humans, the effects of artificially sweetened beverage (ASB) consumption by pregnant women has been studied to examine the effects of NNS on infants, particularly the gut microbial composition and the associated function within the initial year of life (123). In a prospective study, infants (N = 100) from 3 to 12 months of age were studied. Half of the study population were infants born to mothers consuming ASBs during pregnancy and half were born to mothers who did not. Infants born to mothers who consumed ASBs were found to have a higher BMI, compared to children of mothers who did not consume ASBs during pregnancy (123). Infant fecal samples were also used to compare the gut microbiome composition of these infants, which identified associations between maternal ASB consumption and beta diversity, as well as a depletion of Bacteroides sp. and enrichment of Provotella copri. The authors reported secondary effects of increased BMI to be associated with higher levels of the urine metabolites spermidine and succinate within exposed infants (123) and elevated succinate circulation has been previously associated with obesity and impaired glucose metabolism (124).
Collectively, the outcomes from these studies investigating the impact of NNS on the gut microbiome suggest that not only is the gut microbiome affected by the consumption of NNS, but that these impacts on the gut microbiome have physiological consequences for the host (25, 88, 98), and that these consequences may be transmitted vertically, from mother to offspring. As NNS can be detected in milk (39), these findings raise questions as to whether these impacts on the gut microbiome of offspring are related to prenatal impacts on the maternal gut microbiome or maternal provision of NNS via milk (39, 125–128), as well as what other confounding lifestyle factors may shape the gut microbiomes of both mothers and their offspring (129).
Potential mechanisms for interactions between gut microbes and NNS
While regulatory review of each sweetener includes extensive toxicology and safety data (14, 130–132), new research related to the gut microbiome has raised questions as to how these NNS have effects on host physiology. When considering the possible mechanism underlying these results, there are several potential routes by which these findings may be rationalized and supported in future work.
First, there may be interactions between NNS and either known taste receptors or unappreciated receptors with affinity for these compounds found in the gut and linked to the capacity for glucose absorption and homeostasis (133). This possibility would suggest that it is not necessary for NNS to be absorbed to shape host physiological responses and by their regular inclusion in foods that we perceive to be sweet, this alone is sufficient to trigger a physiological response as if these compounds were sugar sweeteners themselves, as cephalic phase insulin release appears to have a contextual component (134). While this is certainly a possibility, the absence of consistent evidence supporting insulin release in response to NNS complicates this possibility (57).
Alternatively, these compounds may act directly on the gut epithelium to shape gut epithelial processes, like mucin production and gut barrier function (76, 135–137), which typically regulate the gut microbiome and shape its composition and metabolism (135, 138). These compounds may also have acute effects on keystone species within the gut microbiome itself, and major impacts on mucin production or its structure have been associated with the depletion of taxa reliant on mucin glycans, such as Akkermansia (120). Further, there is some evidence that several distinct NNS can damage bacterial cell membranes and alter cellular permeability with an “antibiotic-like” effect (102). Conversely, the breakdown products of these compounds, either by the host (8, 65, 136, 139) or the gut microbiome (8, 25, 60, 108), may affect the gut microbiome or the gut epithelium and shift microbial populations as has been reported (Table 2; Figure 1).
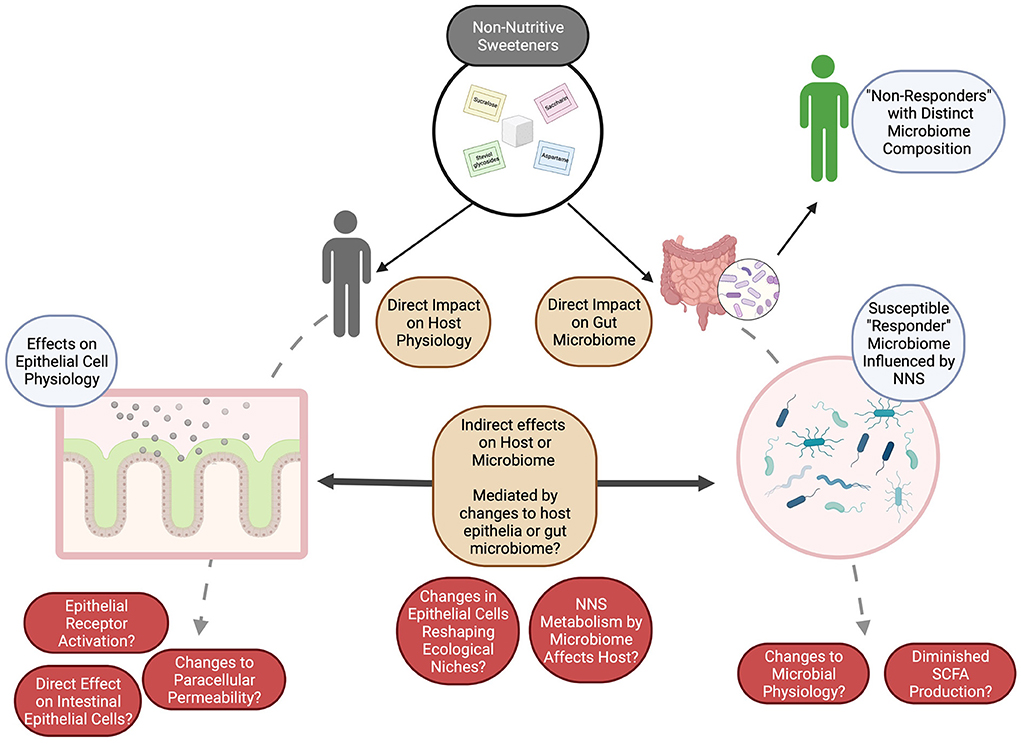
Figure 1. The potential for NNS to shape both the gut microbiome and host responses to these compounds may be either direct or indirect. Many open questions remain (in red) as to how these impacts or interactions may be measured or monitored, but we speculate (brown) that by investigating the impacts of NNS on (1) the host (especially on morphologically distinct epithelial cells), (2) on the gut microbiome, as a whole, and (3) on the interactions between the gut microbiome and the host, the underlying mechanisms can be described and evaluated for their potential to influence human health. Figure created with BioRender. SCFAs, short chain fatty acids.
Together, the reported effects of NNS on human physiology (e.g., impaired glucose tolerance) can be conceptually differentiated to be (1) directly active on the host to influence physiological responses, (2) act on the host epithelium and indirectly influence the gut microbiome composition to influence host responses, or (3) act directly on the gut microbiome to influence its composition. While there is evidence of the first possibility, studies incorporating the microbiome composition of individuals and/or using microbiome transplantation experiments appears to more strongly support an effect of NNS on the gut microbiome, either directly or indirectly, which then influences host health, as generally reported in terms of glucose intolerance, increased body weight, or metabolic modifications (25, 123).
Conclusion
In this review, we assess the microbial and associated metabolic effects of non-nutritive sweeteners and recognize controversies/shortcomings of the existing evidence behind these structurally varied compounds and their use. While there is extensive safety evidence behind NNS, there are growing bodies of work which suggest that NNS in high concentrations may exert possible negative health outcomes within certain susceptible populations/individuals. In particular, susceptible populations may ultimately be identified by their gut microbiome composition, rather than obvious clinical features, given findings identifying responder/non-responder differences among individuals in a small saccharin challenge study (25, 82, 140).
The determination of these effects entails critical evaluation of previously reported confounding factors and a more recognized understanding that each NNS carries individual potential to explain unique metabolic or sensory effects observed (141). Utilizing well-designed and appropriately powered studies in humans, in addition to relevant animal or in vitro models that reflect the human gut microbiome, are critical to comprehend these reported alterations to microbial populations and evaluate their consequences for human health (97, 142). Additionally, the use of gnotobiotic mice have been recognized as one the most informative model when experimentally evaluating responses of the human gut microbiome to dietary challenges (142).
Finally, despite previously unappreciated impacts of NNS, their value must be considered in the context of their role in limiting caloric intake, as alternatives to sugar-sweetened beverages. The value of NNS to efforts limiting the global health burden of obesity and obesity-related disease may outweigh potentially negative effects on human health. While observational studies have linked NNS consumption with an increased risk of cardiovascular disease (17, 143) and acute impacts on glucose responses have been described (16, 25), it is unclear whether short-term consumption is associated with the same outcomes. Further, if acute effects on glucose responses are reversible, and if NNS are consumed in moderation with concomitant caloric reduction, perhaps these food additives can be useful to reduce the significant health risks associated with obesity, which may outweigh the risks of NNS consumption (144). Further research is clearly needed to characterize and assess the potential for NNS to affect human health and the gut microbiome, as well as supporting mechanistic data to identify how these impacts occur. Future studies examining NNS should especially consider the gut microbiome of the study population, whether in animal models of human studies, to more closely determine the relative value of NNS in limiting obesity.
Author contributions
ILR and SAF wrote the manuscript. All authors approved the final manuscript.
Funding
The authors are supported by the University of Nevada, Reno College of Agriculture, Biotechnology, and Natural Resources (CABNR) and the Nevada Experiment Station; the University of Nevada, Reno Department of Nutrition; and the Office of the Vice President for Research and Innovation at the University of Nevada, Reno.
Conflict of interest
The authors declare that the research was conducted in the absence of any commercial or financial relationships that could be construed as a potential conflict of interest.
Publisher's note
All claims expressed in this article are solely those of the authors and do not necessarily represent those of their affiliated organizations, or those of the publisher, the editors and the reviewers. Any product that may be evaluated in this article, or claim that may be made by its manufacturer, is not guaranteed or endorsed by the publisher.
References
1. Liu J, Steele EM Li Y, Karageorgou D, Micha R, Monteiro CA. Mozaffarian D. Consumption of ultraprocessed foods and diet quality among US children and adults. Am J Prev Med. (2022) 62:252–64. doi: 10.1016/j.amepre.2021.08.014
2. Jackson SL, King SMC, Zhao L, Cogswell ME. Prevalence of excess sodium intake in the United States — NHANES, 2009–2012. Morb Mortal Wkly Rep. (2016) 64:1393–7. doi: 10.15585/mmwr.mm6452a1
3. Singh GM, Micha R, Khatibzadeh S, Lim S, Ezzati M, Mozaffarian D. Estimated global, regional, and national disease burdens related to sugar-sweetened beverage consumption in 2010. Circulation. (2015) 132:639–66. doi: 10.1161/CIRCULATIONAHA.114.010636
4. Chattopadhyay S, Raychaudhuri U, Chakraborty R. Artificial sweeteners – a review. J Food Sci Technol. (2014) 51:611–21. doi: 10.1007/s13197-011-0571-1
5. Ruiz-Ojeda FJ, Plaza-Díaz J, Sáez-Lara MJ, Gil A. Effects of sweeteners on the gut microbiota: a review of experimental studies and clinical trials. Adv Nutr. (2019) 10:S31–48. doi: 10.1093/advances/nmy037
6. Mattes RD, Popkin BM. Nonnutritive sweetener consumption in humans: effects on appetite and food intake and their putative mechanisms. Am J Clin Nutr. (2009) 89:1–14. doi: 10.3945/ajcn.2008.26792
7. Sylvetsky AC, Jin Y, Clark EJ, Welsh JA, Rother KI, Talegawkar SA. Consumption of low-calorie sweeteners among children and adults in the United States. J Acad Nutr Diet. (2017) 117:441–8.e2. doi: 10.1016/j.jand.2016.11.004
8. Burke MV, Small DM. Physiological mechanisms by which non-nutritive sweeteners may impact body weight and metabolism. Physiol Behav. (2015) 152:381–8. doi: 10.1016/j.physbeh.2015.05.036
9. Sylvetsky AC, Welsh JA, Brown RJ, Vos MB. Low-calorie sweetener consumption is increasing in the United States123. Am J Clin Nutr. (2012) 96:640–6. doi: 10.3945/ajcn.112.034751
10. Magnuson BA, Carakostas MC, Moore NH, Poulos SP, Renwick AG. Biological fate of low-calorie sweeteners. Nutr Rev. (2016) 74:670–89. doi: 10.1093/nutrit/nuw032
11. Sylvetsky AC, Figueroa J, Zimmerman T, Swithers SE, Welsh JA. Consumption of low-calorie sweetened beverages is associated with higher total energy and sugar intake among children, NHANES 2011–2016. Pediatr Obes. (2019) 14:e12535. doi: 10.1111/ijpo.12535
12. K.D. Aparnathi KD. Chemistry and use of artificial intense sweeteners. Int J Curr Microbiol Appl Sci. (2017) 6:1283–96. doi: 10.20546/ijcmas.2017.606.151
13. FDA Center for Food Safety Applied. High-Intensity Sweeteners. FDA (2017). Available online at: https://www.fda.gov/food/food-additives-petitions/high-intensity-sweeteners (accessed February 15, 2021).
14. Renwick AG, Walker R. An analysis of the risk of exceeding the acceptable or tolerable daily intake. Regul Toxicol Pharmacol. (1993) 18:463–80. doi: 10.1006/rtph.1993.1071
15. EFSA. Sweeteners. (2013). Available online at: https://www.efsa.europa.eu/en/topics/topic/sweeteners (accessed July 5, 2022).
16. Kuk JL, Brown RE. Aspartame intake is associated with greater glucose intolerance in individuals with obesity. Appl Physiol Nutr Metab. (2016). 41:795–8. doi: 10.1139/apnm-2015-0675
17. Azad MB, Abou-Setta AM, Chauhan BF, Rabbani R, Lys J, Copstein L, et al. Nonnutritive sweeteners and cardiometabolic health: a systematic review and meta-analysis of randomized controlled trials and prospective cohort studies. Can Med Assoc J. (2017) 189:E929–39. doi: 10.1503/cmaj.161390
18. Mullee A, Romaguera D, Pearson-Stuttard J, Viallon V, Stepien M, Freisling H, et al. Association between soft drink consumption and mortality in 10 European countries. JAMA Intern Med. (2019) 179:1479–90. doi: 10.1001/jamainternmed.2019.2478
19. Mossavar-Rahmani Y, Kamensky V, Manson JE, Silver B, Rapp SR, Haring B, et al. Artificially sweetened beverages and stroke, coronary heart disease and all-cause mortality in the women's health initiative. Stroke. (2019) 50:555–62. doi: 10.1161/STROKEAHA.118.023100
20. Croom E. Chapter Three - metabolism of xenobiotics of human environments. In: Hodgson E, editor. Progress in Molecular Biology and Translational Science. Toxicology and Human Environments. Cambridge, MA: Academic Press (2012), p. 31–88. doi: 10.1016/B978-0-12-415813-9.00003-9
21. Calatayud Arroyo M, García Barrera T, Callejón Leblic B, Arias Borrego A, Collado MC. A review of the impact of xenobiotics from dietary sources on infant health: early life exposures and the role of the microbiota. Environ Pollut. (2021) 269:115994. doi: 10.1016/j.envpol.2020.115994
22. Maurice CF, Haiser HJ, Turnbaugh PJ. Xenobiotics shape the physiology and gene expression of the active human gut microbiome. Cell. (2013) 152:39–50. doi: 10.1016/j.cell.2012.10.052
23. Bornemann V, Werness SC, Buslinger L, Schiffman SS. Intestinal metabolism and bioaccumulation of sucralose in adipose tissue in the rat. J Toxicol Environ Health A. (2018) 81:913–23. doi: 10.1080/15287394.2018.1502560
24. Suez J, Korem T, Zilberman-Schapira G, Segal E, Elinav E. Non-caloric artificial sweeteners and the microbiome: findings and challenges. Gut Microbes. (2015) 6:149–55. doi: 10.1080/19490976.2015.1017700
25. Suez J, Korem T, Zeevi D, Zilberman-Schapira G, Thaiss CA, Maza O, et al. Artificial sweeteners induce glucose intolerance by altering the gut microbiota. Nature. (2014) 514:181–6. doi: 10.1038/nature13793
26. Palmnäs MSA, Cowan TE, Bomhof MR, Su J, Reimer RA, Vogel HJ, et al. Low-dose aspartame consumption differentially affects gut microbiota-host metabolic interactions in the diet-induced obese rat. PLoS ONE. (2014) 9:e109841. doi: 10.1371/journal.pone.0109841
27. Bian X, Chi L, Gao B, Tu P, Ru H, Lu K. Gut Microbiome response to sucralose and its potential role in inducing liver inflammation in mice. Front Physiol. (2017) 8:487. doi: 10.3389/fphys.2017.00487
28. Fidler Mis N, Braegger C, Bronsky J, Campoy C, Domellöf M, Embleton ND, et al. Sugar in infants, children and adolescents: a position paper of the European society for paediatric gastroenterology, hepatology and nutrition committee on nutrition. J Pediatr Gastroenterol Nutr. (2017) 65:681–96. doi: 10.1097/MPG.0000000000001733
29. Faruque S, Tong J, Lacmanovic V, Agbonghae C, Minaya DM, Czaja K. The dose makes the poison: sugar and obesity in the United States – a review. Pol J Food Nutr Sci. (2019) 69:219–33. doi: 10.31883/pjfns/110735
30. Alam YH, Kim R, Jang C. Metabolism and health impacts of dietary sugars. J Lipid Atheroscler. (2022) 11:20–38. doi: 10.12997/jla.2022.11.1.20
31. Raben A, Vasilaras TH, Møller AC, Astrup A. Sucrose compared with artificial sweeteners: different effects on ad libitum food intake and body weight after 10 wk of supplementation in overweight subjects. Am J Clin Nutr. (2002) 76:721–9. doi: 10.1093/ajcn/76.4.721
32. Miller PE, Perez V. Low-calorie sweeteners and body weight and composition: a meta-analysis of randomized controlled trials and prospective cohort studies123. Am J Clin Nutr. (2014) 100:765–77. doi: 10.3945/ajcn.113.082826
33. Fernstorm J,. Non-Nutritive Sweeteners Obesity | Annual Review of Food Science Technology. (2015). Available online at: https://www-annualreviews-org.unr.idm.oclc.org/doi/10.1146/annurev-food-022814-015635 (accessed February 15, 2021).
34. Peters JC, Beck J, Cardel M, Wyatt HR, Foster GD, Pan Z, et al. The effects of water and non-nutritive sweetened beverages on weight loss and weight maintenance: a randomized clinical trial. Obes Silver Spring Md. (2016) 24:297–304. doi: 10.1002/oby.21327
35. Daher M, Fahd C, Nour AA, Sacre Y. Trends and amounts of consumption of low-calorie sweeteners: a cross-sectional study. Clin Nutr ESPEN. (2022) 48:427–33. doi: 10.1016/j.clnesp.2022.01.006
36. Brown RJ, Rother KI. Non-Nutritive sweeteners and their role in the gastrointestinal tract. J Clin Endocrinol Metab. (2012) 97:2597–605. doi: 10.1210/jc.2012-1475
37. Otabe A, Fujieda T, Masuyama T, Ubukata K, Lee C. Advantame – an overview of the toxicity data. Food Chem Toxicol. (2011) 49:S2–7. doi: 10.1016/j.fct.2011.06.046
38. Nofre C, Tinti J-M. Neotame: discovery, properties, utility. Food Chem. (2000) 69:245–57. doi: 10.1016/S0308-8146(99)00254-X
39. Sylvetsky AC, Gardner AL, Bauman V, Blau JE, Garraffo HM, Walter PJ, et al. MILK. J Toxicol Environ Health A. (2015) 78:1029–32. doi: 10.1080/15287394.2015.1053646
40. Renwick AG. The metabolism of intense sweeteners. Xenobiotica Fate Foreign Compd Biol Syst. (1986) 16:1057–71. doi: 10.3109/00498258609038983
41. Plaza-Diaz J, Pastor-Villaescusa B, Rueda-Robles A, Abadia-Molina F, Ruiz-Ojeda FJ. Plausible biological interactions of low- and non-calorie sweeteners with the intestinal microbiota: an update of recent studies. Nutrients. (2020) 12:1153. doi: 10.3390/nu12041153
42. Soejima A, Tanabe AS, Takayama I, Kawahara T, Watanabe K, Nakazawa M, et al. Phylogeny and biogeography of the genus Stevia (Asteraceae: Eupatorieae): an example of diversification in the Asteraceae in the new world. J Plant Res. (2017) 130:953–72. doi: 10.1007/s10265-017-0955-z
43. Mooradian AD, Smith M, Tokuda M. The role of artificial and natural sweeteners in reducing the consumption of table sugar: a narrative review. Clin Nutr ESPEN. (2017) 18:1–8. doi: 10.1016/j.clnesp.2017.01.004
44. Gardner C,. Nonnutritive Sweeteners: Current Use Health Perspectives. (2012). Available online at: https://www-ncbi-nlm-nih-gov.unr.idm.oclc.org/pmc/articles/PMC3402256/ (accessed January 30, 2022).
45. Warshaw H, Edelman SV. Practical strategies to help reduce added sugars consumption to support glycemic and weight management goals. Clin Diabetes. (2021) 39:45–56. doi: 10.2337/cd20-0034
46. U.S. Department of Agriculture and U.S. Department of, Health and Human Services. Dietary Guidelines for Americans, 2020-2025, 9th Edition. (2020). Available online at: https://www.dietaryguidelines.gov/ (accessed July 5, 2022).
47. MacLeod J, Franz MJ, Handu D, Gradwell E, Brown C, Evert A, et al. Academy of nutrition and dietetics nutrition practice guideline for type 1 and type 2 diabetes in adults: nutrition intervention evidence reviews and recommendations. J Acad Nutr Diet. (2017) 117:1637–58. doi: 10.1016/j.jand.2017.03.023
48. Evert AB, Boucher JL, Cypress M, Dunbar SA, Franz MJ, Mayer-Davis EJ, et al. Nutrition therapy recommendations for the management of adults with diabetes. Diabetes Care. (2013) 37:S120–43. doi: 10.2337/dc14-S120
49. Fitch C, Keim KS. Position of the academy of nutrition and dietetics: use of nutritive and nonnutritive sweeteners. J Acad Nutr Diet. (2012) 112:739–58. doi: 10.1016/j.jand.2012.03.009
50. Pang MD, Goossens GH, Blaak EE. The impact of artificial sweeteners on body weight control and glucose homeostasis. Front Nutr. (2021) 7:598340. doi: 10.3389/fnut.2020.598340
51. Blum JW, Jacobsen DJ, Donnelly JE. Beverage consumption patterns in elementary school aged children across a two-year period. J Am Coll Nutr. (2005) 24:93–8. doi: 10.1080/07315724.2005.10719449
52. Fowler SP, Williams K, Resendez RG, Hunt KJ, Hazuda HP, Stern MP. Fueling the obesity epidemic? Artificially sweetened beverage use and long-term weight gain. Obesity. (2008) 16:1894–900. doi: 10.1038/oby.2008.284
53. De La Hunty A, Gibson S, Ashwell M. A review of the effectiveness of aspartame in helping with weight control. Nutr Bull. (2006) 31:115–28. doi: 10.1111/j.1467-3010.2006.00564.x
54. Rogers PJ, Appleton KM. The effects of low-calorie sweeteners on energy intake and body weight: a systematic review and meta-analyses of sustained intervention studies. Int J Obes. (2021) 45:464–78. doi: 10.1038/s41366-020-00704-2
55. Higgins KA, Considine RV, Mattes RD. Aspartame consumption for 12 weeks does not affect glycemia, appetite, or body weight of healthy, lean adults in a randomized controlled trial. J Nutr. (2018) 148:650–7. doi: 10.1093/jn/nxy021
56. Azad MB, Archibald A, Tomczyk MM, Head A, Cheung KG, de Souza RJ, et al. Nonnutritive sweetener consumption during pregnancy, adiposity, and adipocyte differentiation in offspring: evidence from humans, mice, and cells. Int J Obes. (2020) 44:2137–48. doi: 10.1038/s41366-020-0575-x
57. Walbolt J, Koh Y. Non-nutritive sweeteners and their associations with obesity and type 2 diabetes. J Obes Metab Syndr. (2020) 29:114–23. doi: 10.7570/jomes19079
58. Wilk K, Korytek W, Pelczyńska M, Moszak M, Bogdański P. The effect of artificial sweeteners use on sweet taste perception and weight loss efficacy: a review. Nutrients. (2022) 14:1261. doi: 10.3390/nu14061261
59. Higgins KA, Mattes RD. A randomized controlled trial contrasting the effects of 4 low-calorie sweeteners and sucrose on body weight in adults with overweight or obesity. Am J Clin Nutr. (2019) 109:1288–301. doi: 10.1093/ajcn/nqy381
60. del Pozo S, Gómez-Martínez S, Díaz LE, Nova E, Urrialde R, Marcos A. Potential effects of sucralose and saccharin on gut microbiota: a review. Nutrients. (2022) 14:1682. doi: 10.3390/nu14081682
61. Liauchonak I, Qorri B, Dawoud F, Riat Y, Szewczuk MR. Non-nutritive sweeteners and their implications on the development of metabolic syndrome. Nutrients. (2019) 11:644. doi: 10.3390/nu11030644
62. Davidson TL, Martin AA, Clark K, Swithers SE. Intake of high-intensity sweeteners alters the ability of sweet taste to signal caloric consequences: implications for the learned control of energy and body weight regulation. Q J Exp Psychol. (2011) 64:1430–41. doi: 10.1080/17470218.2011.552729
63. Green E, Murphy C. Altered processing of sweet taste in the brain of diet soda drinkers. Physiol Behav. (2012) 107:560–7. doi: 10.1016/j.physbeh.2012.05.006
64. Feijó F de M, Ballard CR, Foletto KC, Batista BAM, Neves AM, Ribeiro MFM, et al. Saccharin and aspartame, compared with sucrose, induce greater weight gain in adult Wistar rats, at similar total caloric intake levels. Appetite. (2013) 60:203–7. doi: 10.1016/j.appet.2012.10.009
65. Swithers SE. Artificial sweeteners produce the counterintuitive effect of inducing metabolic derangements. Trends Endocrinol Metab TEM. (2013) 24:431–41. doi: 10.1016/j.tem.2013.05.005
66. Smeets PAM, Erkner A, de Graaf C. Cephalic phase responses and appetite. Nutr Rev. (2010) 68:643–55. doi: 10.1111/j.1753-4887.2010.00334.x
67. Dhillon J, Lee JY, Mattes RD. The cephalic phase insulin response to nutritive and low-calorie sweeteners in solid and beverage form. Physiol Behav. (2017) 181:100–9. doi: 10.1016/j.physbeh.2017.09.009
68. Frank GKW, Oberndorfer TA, Simmons AN, Paulus MP, Fudge JL, Yang TT, et al. Sucrose activates human taste pathways differently from artificial sweetener. Neuroimage. (2008) 39:1559–69. doi: 10.1016/j.neuroimage.2007.10.061
69. Just T, Pau HW, Engel U, Hummel T. Cephalic phase insulin release in healthy humans after taste stimulation? Appetite. (2008) 51:622–7. doi: 10.1016/j.appet.2008.04.271
70. Pepino MY, Tiemann CD, Patterson BW, Wice BM, Klein S. Sucralose affects glycemic and hormonal responses to an oral glucose load. Diabetes Care. (2013) 36:2530–5. doi: 10.2337/dc12-2221
71. Ma J, Chang J, Checklin HL, Young RL, Jones KL, Horowitz M, et al. Effect of the artificial sweetener, sucralose, on small intestinal glucose absorption in healthy human subjects. Br J Nutr. (2010) 104:803–6. doi: 10.1017/S0007114510001327
72. Schneeman BO. Gastrointestinal physiology and functions. Br J Nutr. (2002) 88:S159–63. doi: 10.1079/BJN2002681
73. Lynch SV, Pedersen O. The human intestinal microbiome in health and disease. N Engl J Med. (2016) 375:2369–79. doi: 10.1056/NEJMra1600266
74. Frese SA, Benson AK, Tannock GW, Loach DM, Kim J, Zhang M, et al. The evolution of host specialization in the vertebrate gut symbiont Lactobacillus reuteri. PLoS Genet. (2011) 7:e1001314. doi: 10.1371/journal.pgen.1001314
75. Turroni F, Milani C, Van Sinderen D, Ventura M. Chapter 12 - Bifidobacteria: ecology and coevolution with the host. In: Mattarelli P, Biavati B, Holzapfel WH, Wood BJB, editors. The Bifidobacteria and Related Organisms. Cambridge, MA: Academic Press (2018), p. 213–20 doi: 10.1016/B978-0-12-805060-6.00012-0
76. Van den Abbeele P, Van de Wiele T, Verstraete W, Possemiers S. The host selects mucosal and luminal associations of coevolved gut microorganisms: a novel concept. FEMS Microbiol Rev. (2011) 35:681–704. doi: 10.1111/j.1574-6976.2011.00270.x
77. Qin J, Li R, Raes J, Arumugam M, Burgdorf KS, Manichanh C, et al. A human gut microbial gene catalogue established by metagenomic sequencing. Nature. (2010) 464:59–65. doi: 10.1038/nature08821
78. Kohl KD, Dearing MD. The woodrat gut microbiota as an experimental system for understanding microbial metabolism of dietary toxins. Front Microbiol. (2016) 7:1165. doi: 10.3389/fmicb.2016.01165
79. Clarke G, Sandhu KV, Griffin BT, Dinan TG, Cryan JF, Hyland NP. Gut reactions: breaking down xenobiotic–microbiome interactions. Pharmacol Rev. (2019) 71:198–224. doi: 10.1124/pr.118.015768
80. Cao Y, Liu H, Qin N, Ren X, Zhu B, Xia X. Impact of food additives on the composition and function of gut microbiota: A review. Trends Food Sci Technol. (2020) 99:295–310. doi: 10.1016/j.tifs.2020.03.006
81. Collins J, Robinson C, Danhof H, Knetsch CW, van Leeuwen HC, Lawley TD, et al. Dietary trehalose enhances virulence of epidemic Clostridium difficile. Nature. (2018) 553:291–4. doi: 10.1038/nature25178
82. Whitehouse CR, Boullata J, McCauley LA. The potential toxicity of artificial sweeteners. AAOHN J. (2008) 56:251–61. doi: 10.1177/216507990805600604
83. Pepino MY. Metabolic effects of non-nutritive sweeteners. Physiol Behav. (2015) 152:450–5. doi: 10.1016/j.physbeh.2015.06.024
84. Hughes RL, Davis CD, Lobach A, Holscher HD. An overview of current knowledge of the gut microbiota and low-calorie sweeteners. Nutr Today. (2021) 56:105–13. doi: 10.1097/NT.0000000000000481
85. Shahriar S, Ahsan T, Khan A, Akhteruzzaman S, Shehreen S, Sajib AA. Aspartame, acesulfame K and sucralose- influence on the metabolism of Escherichia coli. Metab Open. (2020) 8:100072. doi: 10.1016/j.metop.2020.100072
86. Shil A, Chichger H. Artificial sweeteners negatively regulate pathogenic characteristics of two model gut bacteria, E. coli and E faecalis. Int J Mol Sci. (2021) 22:5228. doi: 10.3390/ijms22105228
87. Harpaz D, Yeo LP, Cecchini F, Koon THP, Kushmaro A, Tok AIY, et al. Measuring artificial sweeteners toxicity using a bioluminescent bacterial panel. Molecules. (2018) 23:2454. doi: 10.3390/molecules23102454
88. Wang Q-P, Browman D, Herzog H, Neely GG. Non-nutritive sweeteners possess a bacteriostatic effect and alter gut microbiota in mice. PLoS ONE. (2018) 13:e0199080. doi: 10.1371/journal.pone.0199080
89. Markus V, Share O, Shagan M, Halpern B, Bar T, Kramarsky-Winter E, et al. Inhibitory effects of artificial sweeteners on bacterial quorum sensing. Int J Mol Sci. (2021) 22:9863. doi: 10.3390/ijms22189863
90. Denina I, Semjonovs P, Fomina A, Treimane R, Linde R. The influence of stevia glycosides on the growth of Lactobacillus reuteri strains. Lett Appl Microbiol. (2014) 58:278–84. doi: 10.1111/lam.12187
91. Bian X, Tu P, Chi L, Gao B, Ru H, Lu K. Saccharin induced liver inflammation in mice by altering the gut microbiota and its metabolic functions. Food Chem Toxicol Int J Publ Br Ind Biol Res Assoc. (2017) 107:530–9. doi: 10.1016/j.fct.2017.04.045
92. Bian X, Chi L, Gao B, Tu P, Ru H, Lu K. The artificial sweetener acesulfame potassium affects the gut microbiome and body weight gain in CD-1 mice. PLoS ONE. (2017) 12:e0178426. doi: 10.1371/journal.pone.0178426
93. Uebanso T, Ohnishi A, Kitayama R, Yoshimoto A, Nakahashi M, Shimohata T, et al. Effects of low-dose non-caloric sweetener consumption on gut microbiota in mice. Nutrients. (2017) 9:E560. doi: 10.3390/nu9060560
94. de la Garza AL, Romero-Delgado B, Martínez-Tamez AM, Cárdenas-Tueme M, Camacho-Zamora BD, Matta-Yee-Chig D, et al. Maternal sweeteners intake modulates gut microbiota and exacerbates learning and memory processes in adult male offspring. Front Pediatr. (2022) 9:746437. doi: 10.3389/fped.2021.746437
95. Becker SL, Chiang E, Plantinga A, Carey HV, Suen G, Swoap SJ. Effect of stevia on the gut microbiota and glucose tolerance in a murine model of diet-induced obesity. FEMS Microbiol Ecol. (2020) 96:fiaa079. doi: 10.1093/femsec/fiaa079
96. Pfeffer M, Ziesenitz SC, Siebert G. Acesulfame K, cyclamate and saccharin inhibit the anaerobic fermentation of glucose by intestinal bacteria. Z Ernahrungswiss. (1985) 24:231–5. doi: 10.1007/BF02023668
97. Frankenfeld CL, Sikaroodi M, Lamb E, Shoemaker S, Gillevet PM. High-intensity sweetener consumption and gut microbiome content and predicted gene function in a cross-sectional study of adults in the United States. Ann Epidemiol. (2015) 25:736–42.e4. doi: 10.1016/j.annepidem.2015.06.083
98. Olivier-Van Stichelen S, Rother KI, Hanover JA. Maternal exposure to non-nutritive sweeteners impacts progeny's metabolism and microbiome. Front Microbiol. (2019) 10:1360. doi: 10.3389/fmicb.2019.01360
99. Hanawa Y, Higashiyama M, Kurihara C, Tanemoto R, Ito S, Mizoguchi A, et al. Acesulfame potassium induces dysbiosis and intestinal injury with enhanced lymphocyte migration to intestinal mucosa. J Gastroenterol Hepatol. (2021) 36:3140–8. doi: 10.1111/jgh.15654
100. Horwitz DL, McLane M, Kobe P. Response to single dose of aspartame or saccharin by NIDDM patients. Diabetes Care. (1988) 11:230–4. doi: 10.2337/diacare.11.3.230
101. Nettleton JE, Cho NA, Klancic T, Nicolucci AC, Shearer J, Borgland SL, et al. Maternal low-dose aspartame and stevia consumption with an obesogenic diet alters metabolism, gut microbiota and mesolimbic reward system in rat dams and their offspring. Gut. (2020) 69:1807–17. doi: 10.1136/gutjnl-2018-317505
102. Yu Z, Wang Y, Lu J, Bond PL, Guo J. Nonnutritive sweeteners can promote the dissemination of antibiotic resistance through conjugative gene transfer. ISME J. (2021) 15:2117–30. doi: 10.1038/s41396-021-00909-x
103. Sünderhauf A, Pagel R, Künstner A, Wagner AE, Rupp J, Ibrahim SM, et al. Saccharin supplementation inhibits bacterial growth and reduces experimental colitis in mice. Nutrients. (2020) 12:1122. doi: 10.3390/nu12041122
104. Serrano J, Smith KR, Crouch AL, Sharma V, Yi F, Vargova V, et al. High-dose saccharin supplementation does not induce gut microbiota changes or glucose intolerance in healthy humans and mice. Microbiome. (2021) 9:11. doi: 10.1186/s40168-020-00976-w
105. Li J, Zhu S, Lv Z, Dai H, Wang Z, Wei Q, et al. Drinking water with saccharin sodium alters the microbiota-gut-hypothalamus axis in guinea pig. Anim Open Access J MDPI. (2021) 11:1875. doi: 10.3390/ani11071875
106. Naim M, Zechman JM, Brand JG, Kare MR, Sandovsky V. Effects of sodium saccharin on the activity of trypsin, chymotrypsin, and amylase and upon bacteria in small intestinal contents of rats. Proc Soc Exp Biol Med Soc Exp Biol Med N Y N. (1985) 178:392–401. doi: 10.3181/00379727-178-42022
107. Labrecque MT, Malone D, Caldwell KE, Allan AM. Impact of ethanol and saccharin on fecal microbiome in pregnant and non-pregnant mice. J Pregnancy Child Health. (2015) 2:1000193. doi: 10.4172/2376-127X.1000193
108. Abou-Donia MB, El-Masry EM, Abdel-Rahman AA, McLendon RE, Schiffman SS. Splenda alters gut microflora and increases intestinal p-glycoprotein and cytochrome p-450 in male rats. J Toxicol Environ Health A. (2008) 71:1415–29. doi: 10.1080/15287390802328630
109. Wang W, Nettleton JE, Gänzle MG, Reimer RA. A metagenomics investigation of intergenerational effects of non-nutritive sweeteners on gut microbiome. Front Nutr. (2021) 8:795848. doi: 10.3389/fnut.2021.795848
110. Rodriguez-Palacios A, Harding A, Menghini P, Himmelman C, Retuerto M, Nickerson KP, et al. The artificial sweetener Splenda promotes gut proteobacteria, dysbiosis, and myeloperoxidase reactivity in Crohn's disease-like ileitis. Inflamm Bowel Dis. (2018) 24:1005–20. doi: 10.1093/ibd/izy060
111. Sánchez-Tapia M, Miller AW, Granados-Portillo O, Tovar AR, Torres N. The development of metabolic endotoxemia is dependent on the type of sweetener and the presence of saturated fat in the diet. Gut Microbes. (2020) 12:1801301. doi: 10.1080/19490976.2020.1801301
112. Dai X, Guo Z, Chen D, Li L, Song X, Liu T, et al. Maternal sucralose intake alters gut microbiota of offspring and exacerbates hepatic steatosis in adulthood. Gut Microbes. (2020) 11:1043–63. doi: 10.1080/19490976.2020.1738187
113. Nettleton JE, Klancic T, Schick A, Choo AC, Shearer J, Borgland SL, et al. Low-dose stevia (Rebaudioside A) consumption perturbs gut microbiota and the mesolimbic dopamine reward system. Nutrients. (2019) 11:1248. doi: 10.3390/nu11061248
114. Gardana C, Simonetti P, Canzi E, Zanchi R, Pietta P. Metabolism of stevioside and rebaudioside A from Stevia rebaudiana extracts by human microflora. J Agric Food Chem. (2003) 51:6618–22. doi: 10.1021/jf0303619
115. Chi L, Bian X, Gao B, Tu P, Lai Y, Ru H, et al. Effects of the artificial sweetener neotame on the gut microbiome and fecal metabolites in mice. Mol Basel Switz. (2018) 23:E367. doi: 10.3390/molecules23020367
116. Zhang J, Ni Y, Qian L, Fang Q, Zheng T, Zhang M, et al. Decreased abundance of Akkermansia muciniphila leads to the impairment of insulin secretion and glucose homeostasis in lean type 2 diabetes. Adv Sci. (2021) 8:2100536. doi: 10.1002/advs.202100536
117. Dao MC, Everard A, Aron-Wisnewsky J, Sokolovska N, Prifti E, Verger EO, et al. Akkermansia muciniphila and improved metabolic health during a dietary intervention in obesity: relationship with gut microbiome richness and ecology. Gut. (2016) 65:426–36. doi: 10.1136/gutjnl-2014-308778
118. Shin N-R, Lee J-C, Lee H-Y, Kim M-S, Whon TW, Lee M-S, et al. An increase in the Akkermansia spp. population induced by metformin treatment improves glucose homeostasis in diet-induced obese mice. Gut. (2014) 63:727–35. doi: 10.1136/gutjnl-2012-303839
119. Everard A, Belzer C, Geurts L, Ouwerkerk JP, Druart C, Bindels LB, et al. Cross-talk between Akkermansia muciniphila and intestinal epithelium controls diet-induced obesity. Proc Natl Acad Sci. (2013) 110:9066–71. doi: 10.1073/pnas.1219451110
120. Plovier H, Everard A, Druart C, Depommier C, Van Hul M, Geurts L, et al. A purified membrane protein from Akkermansia muciniphila or the pasteurized bacterium improves metabolism in obese and diabetic mice. Nat Med. (2017) 23:107–13. doi: 10.1038/nm.4236
121. Yoon HS, Cho CH, Yun MS, Jang SJ, You HJ, Kim J, et al. Akkermansia muciniphila secretes a glucagon-like peptide-1-inducing protein that improves glucose homeostasis and ameliorates metabolic disease in mice. Nat Microbiol. (2021) 6:563–73. doi: 10.1038/s41564-021-00880-5
122. Kraal L, Abubucker S, Kota K, Fischbach MA, Mitreva M. The prevalence of species and strains in the human microbiome: a resource for experimental efforts. PLoS ONE. (2014) 9:e97279. doi: 10.1371/journal.pone.0097279
123. Laforest-Lapointe I, Becker AB, Mandhane PJ, Turvey SE, Moraes TJ, Sears MR, et al. Maternal consumption of artificially sweetened beverages during pregnancy is associated with infant gut microbiota and metabolic modifications and increased infant body mass index. Gut Microbes. (2020) 13:1–15. doi: 10.1080/19490976.2020.1857513
124. Serena C, Ceperuelo-Mallafré V, Keiran N, Queipo-Ortuño MI, Bernal R, Gomez-Huelgas R, et al. Elevated circulating levels of succinate in human obesity are linked to specific gut microbiota. ISME J. (2018) 12:1642–57. doi: 10.1038/s41396-018-0068-2
125. Zhang G-H, Chen M-L, Liu S-S, Zhan Y-H, Quan Y, Qin Y-M, et al. Effects of mother's dietary exposure to acesulfame-K in Pregnancy or lactation on the adult offspring's sweet preference. Chem Senses. (2011) 36:763–70. doi: 10.1093/chemse/bjr050
126. Reid AE, Chauhan BF, Rabbani R, Lys J, Copstein L, Mann A, et al. Early exposure to nonnutritive sweeteners and long-term metabolic health: a systematic review. Pediatrics. (2016) 137:e20153603. doi: 10.1542/peds.2015-3603
127. Cai C, Sivak A, Davenport MH. Effects of prenatal artificial sweeteners consumption on birth outcomes: a systematic review and meta-analysis. Public Health Nutr. (2021) 24:5024–33. doi: 10.1017/S1368980021000173
128. Araújo JR, Martel F, Keating E. Exposure to non-nutritive sweeteners during pregnancy and lactation: Impact in programming of metabolic diseases in the progeny later in life. Reprod Toxicol. (2014) 49:196–201. doi: 10.1016/j.reprotox.2014.09.007
129. Fouhy F, Watkins C, Hill CJ, O'Shea C-A, Nagle B, Dempsey EM, et al. Perinatal factors affect the gut microbiota up to four years after birth. Nat Commun. (2019) 10:1517. doi: 10.1038/s41467-019-09252-4
130. Roberts A. The safety and regulatory process for low calorie sweeteners in the United States. Physiol Behav. (2016) 164:439–44. doi: 10.1016/j.physbeh.2016.02.039
131. Rulis AM, Levitt JA. FDA'S food ingredient approval process: safety assurance based on scientific assessment. Regul Toxicol Pharmacol. (2009) 53:20–31. doi: 10.1016/j.yrtph.2008.10.003
132. FDA Center for Food Safety and Applied. Redbook 2000. US Food Drug Adm. (2018). Available online at: https://www.fda.gov/regulatory-information/search-fda-guidance-documents/guidance-industry-and-other-stakeholders-redbook-2000 (accessed July 5, 2022).
133. Pepino MY, Bourne C. Nonnutritive sweeteners, energy balance and glucose homeostasis. Curr Opin Clin Nutr Metab Care. (2011) 14:391–5. doi: 10.1097/MCO.0b013e3283468e7e
134. Pullicin AJ, Glendinning JI, Lim J. Cephalic phase insulin release: a review of its mechanistic basis and variability in humans. Physiol Behav. (2021) 239:113514. doi: 10.1016/j.physbeh.2021.113514
135. Montagne L, Piel C, Lalles JP. Effect of diet on mucin kinetics and composition: nutrition and health implications. Nutr Rev. (2004) 62:105–14. doi: 10.1111/j.1753-4887.2004.tb00031.x
136. Santos PS, Caria CRP, Gotardo EMF, Ribeiro ML, Pedrazzoli J, Gambero A. Artificial sweetener saccharin disrupts intestinal epithelial cells' barrier function in vitro. Food Funct. (2018) 9:3815–22. doi: 10.1039/C8FO00883C
137. Shil A, Olusanya O, Ghufoor Z, Forson B, Marks J, Chichger H. Artificial sweeteners disrupt tight junctions and barrier function in the intestinal epithelium through activation of the sweet taste receptor, T1R3. Nutrients. (2020) 12:1862. doi: 10.3390/nu12061862
138. Tailford LE, Crost EH, Kavanaugh D, Juge N. Mucin glycan foraging in the human gut microbiome. Front Genet. (2015) 6:81. doi: 10.3389/fgene.2015.00081
139. van Eyk AD. The effect of five artificial sweeteners on Caco-2, HT-29 and HEK-293 cells. Drug Chem Toxicol. (2015) 38:318–27. doi: 10.3109/01480545.2014.966381
140. Palatnik A, Moosreiner A, Stichelen SO-V. Consumption of non-nutritive sweeteners during pregnancy. Am J Obstet Gynecol. (2020) 223:211–8. doi: 10.1016/j.ajog.2020.03.034
141. Morahan HL, Leenaars CHC, Boakes RA, Rooney KB. Metabolic and behavioural effects of prenatal exposure to non-nutritive sweeteners: a systematic review and meta-analysis of rodent models. Physiol Behav. (2020) 213:112696. doi: 10.1016/j.physbeh.2019.112696
142. Lobach AR, Roberts A, Rowland IR. Assessing the in vivo data on low/no-calorie sweeteners and the gut microbiota. Food Chem Toxicol Int J Publ Br Ind Biol Res Assoc. (2019) 124:385–99. doi: 10.1016/j.fct.2018.12.005
143. Yin J, Zhu Y, Malik V, Li X, Peng X, Zhang FF, et al. Intake of sugar-sweetened and low-calorie sweetened beverages and risk of cardiovascular disease: a meta-analysis and systematic review. Adv Nutr. (2020) 12:89–101. doi: 10.1093/advances/nmaa084
Keywords: non-nutritive artificial sweeteners, saccharin, sucralose, aspartame, gut microbiome, dietary additives
Citation: Richardson IL and Frese SA (2022) Non-nutritive sweeteners and their impacts on the gut microbiome and host physiology. Front. Nutr. 9:988144. doi: 10.3389/fnut.2022.988144
Received: 07 July 2022; Accepted: 29 July 2022;
Published: 25 August 2022.
Edited by:
Clara G. De Los Reyes-Gavilan, Spanish National Research Council (CSIC), SpainReviewed by:
Dalia El Khoury, University of Guelph, CanadaAna Maria Calderon De La Barca, Consejo Nacional de Ciencia y Tecnología (CONACYT), Mexico
Copyright © 2022 Richardson and Frese. This is an open-access article distributed under the terms of the Creative Commons Attribution License (CC BY). The use, distribution or reproduction in other forums is permitted, provided the original author(s) and the copyright owner(s) are credited and that the original publication in this journal is cited, in accordance with accepted academic practice. No use, distribution or reproduction is permitted which does not comply with these terms.
*Correspondence: Steven A. Frese, sfrese@unr.edu