The relationship between obesity-related H19DMR methylation and H19 and IGF2 gene expression on offspring growth and body composition
- 1Nutrition Department, School of Public Health, University of São Paulo, São Paulo, Brazil
- 2Department of Genetics, Ribeirão Preto Medical School, University of São Paulo, São Paulo, Brazil
Background and objective: Imprinted genes are important for the offspring development. To assess the relationship between obesity-related H19DMR methylation and H19 and IGF2 gene expression and offspring growth and body composition.
Methods: Thirty-nine overweight/obese and 25 normal weight pregnant women were selected from the “Araraquara Cohort Study” according to their pre-pregnancy BMI. Fetal growth and body composition and newborn growth were assessed, respectively, by ultrasound and anthropometry. The methylation of H19DMR in maternal blood, cord blood, maternal decidua and placental villi tissues was evaluated by methylation-sensitive restriction endonuclease qPCR, and H19 and IGF2 expression by relative real-time PCR quantification. Multiple linear regression models explored the associations of DNA methylation and gene expression with maternal, fetal, and newborn parameters.
Results: H19DMR was less methylated in maternal blood of the overweight/obese group. There were associations of H19DMR methylation in cord blood with centiles of fetal biparietal diameter (BPD) and abdominal subcutaneous fat thickness and newborn head circumference (HC); H19DMR methylation in maternal decidua with fetal occipitofrontal diameter (OFD), HC, and length; H19DMR methylation in placental villi with fetal OFD, HC and abdominal subcutaneous fat thickness and with newborn HC. H19 expression in maternal decidua was associated with fetal BPD and femur length centiles and in placental villi with fetal OFD and subcutaneous arm fat. IGF2 expression in maternal decidua was associated with fetal BPD and in placental villi with fetal OFD.
Conclusion: To our knowledge, this is the first study to demonstrate associations of imprinted genes variations at the maternal-fetal interface of the placenta and in cord blood with fetal body composition, supporting the involvement of epigenetic mechanisms in offspring growth and body composition.
1. Introduction
The World Health Organization (WHO) alerts to the high prevalence of overweight and obesity worldwide. Obesity is currently one of the leading public health problems, with projections of ~2.3 billion overweight and 700 million obese adults by 2025 (1). Within this scenario, an increase is observed in the incidence of overweight among women of reproductive age (2). Overweight and obesity affect maternal and child health, contributing to the development of maternal diseases such as gestational diabetes and hypertension and exposing fetuses to an unfavorable metabolic environment, which can lead to the development of chronic diseases later in life (3).
The literature shows that imprinted genes are associated with embryonic and placental development, regulating the metabolism and physiology of the offspring (4). Genomic imprinting is an epigenetic process that silences a parental allele, resulting in monoallelic expression. Changes in the expression pattern of these genes can affect fetal growth and weight at birth (5). The placenta exhibits high expression of imprinted genes, and these genes are important for determining the placental phenotype by regulating environmental responses and nutrient transport (6).
Maternal obesity is associated with altered DNA methylation profiles (7). These alterations mainly occur in differentially methylated regions (DMRs), including imprinted genes such as insulin-like growth factor 2 (IGF2) (8). The IGF2 and H19 genes are two oppositely expressed imprinted genes located adjacent to each other at 11p15.5 that share similar epigenetic transcriptional regulatory mechanisms and play a role in feto-placental development (9).The H19 differentially methylated region (DMR) is paternally methylated and maternally unmethylated, and regulates the imprinted expression of H19 and IGF2 (10).
Animal models involving mice demonstrated that H19 deletion resulted in increased IGF2 expression and fetal overgrowth (11), and that IGF2 deletion resulted in fetal growth restriction (12). In humans, maternal blood IGF2 methylation was associated with birth weight (13) and IGF2 expression in the placenta was associated with fetal growth disorders (14). A cohort study in the Netherlands that evaluated fetal growth through serial measurements with ultrasound and weight of infants at 3 and 6 months of life, also found associations of IGF2 and H19 DNA methylation with fetal and infant growth (15). Despite efforts to a better understanding of the role of IGF2 and H19DMR in the regulation of offspring growth, their relationship with body composition and different tissues, such as placenta, has not yet been fully clarified.
Thus, in this study, we aimed to investigate the impact of maternal overweight/obesity on: (1) fetal-newborn growth and body composition during two gestational periods and postpartum, (2) H19DMR methylation in maternal and umbilical cord blood, maternal decidua and placental villi tissues, (3) the expression of H19 and IGF2 genes in the maternal decidua and placental villi tissues, and (4) the relationship between DNA methylation and gene expression in different maternal and fetal tissues with fetal-newborn growth and body composition.
2. Subjects and methods
2.1. Subjects
This study involved the first 39 overweight/obese and 25 normal weight pregnant women, according to their pre-pregnancy body mass index (BMI), selected in 2017–2018, as part of a large epidemiological prospective study that is still under development and should incorporate 2000 pregnant women—“The Araraquara Cohort Study”. They were followed up during three different periods of pregnancy and at delivery: time 1 (T1), from gestational age ≤ 15 weeks; time 2 (T2), 20–26 weeks; time 3 (T3), 30–36 weeks; and time 4 (T4, at delivery). Pregnant women were eligible for the study after signing the informed consent form approved by the Research Ethics Committee of the School of Public Health, University of São Paulo (protocol number 2.570.576). This study was conducted in compliance with the Helsinki guidelines.
The women were excluded from the study if they had more than 15 weeks of gestation, were under 18 and over 35 years of age, had a pre-pregnancy BMI < 18.5 kg/m2 (malnourished), and had a serious mental illness or infectious diseases. Women who miscarried, gave birth to twins, had a stillborn baby or babies with congenital diseases, or failed to attend one appointment during the follow-up were also excluded.
Demographic and socioeconomic (age, race, marital status, and education), lifestyle (smoking and alcohol consumption), obstetric (parity and morbidity), and nutritional (pre-pregnancy BMI and total weight gain) data were obtained by interview and from the medical records.
2.2. Anthropometric assessment of the pregnant women
Anthropometric assessment (T1, T2, T3, T4) was performed in an ambulatory room. Maternal weight and body composition were assessed by bioimpedance using the Tanita® MC-180 MA equipment (Tanita®, Tokyo, Japan). Pre-pregnancy weight was considered as the weight evaluated up to the 13th week of gestation. Height was measured with a Seca® 206 stadiometer (Seca®, Hamburg, Germany). BMI was calculated using weight and height values [weight (kg)/height2 (m)] and weight gain in pregnancy was compared to the Institute of Medicine recommendations (16). Trained personnel performed all measurements using standardized procedures.
2.3. Fetal growth and body composition
Fetal growth was evaluated by ultrasound at T2 and T3. A trained sonographer performed the assessments with the Siemens ACUSON X300TM ultrasound system, premium edition (Siemens®, Mountain View, CA, USA), equipped with abdominal curvilinear transducers (C5-2, C6-3, V7-3). The following biometric measurements were obtained from the fetus: biparietal diameter (BPD, cm), occipitofrontal diameter (OFD, cm), head circumference (HC, cm), abdominal circumference (AC, cm), femur length (FL, cm), humeral length (HL, cm), and length (cm). The fetal growth measurements were classified according to the centiles of the INTERGROWTH-21st (IG-21) charts (17).
The following fetal body composition parameters were assessed: abdominal subcutaneous fat thickness (SCFT, mm); total thigh tissue = total muscle mass + fat (cm3); thigh muscle mass = internal area of the subcutaneous tissue of the thigh (cm3); subcutaneous thigh fat = total thigh tissue – thigh muscle mass (cm3); total arm tissue = arm muscle mass + fat (cm3); arm muscle mass = internal area of the subcutaneous tissue of the arm (cm3); subcutaneous arm fat = total arm tissue - arm muscle mass (cm3).
2.4. Anthropometry of the newborns
After delivery (T4), the newborns were weighed on a Soehnle Multina Plus electronic baby scale (Soehnle®, Gaildorfer, Germany). Length (cm) was measured with a Seca® 416 infantometer (Seca®, Hamburg, Germany) and BMI [birth weight (kg)/length2 (m)] was calculated. Newborn AC (cm) and thoracic circumference (TC, cm) were measured with a Seca® 201 flexible tape (Seca®, Hamburg, Germany). To ensure accuracy and reproducibility of the measurements, the researchers attended a dedicated training course.
2.5. Biological material
A qualified professional collected maternal blood into EDTA VACUETTE® tubes at T3. Cord blood was collected with a sterile slip-tip 20-ml Descarpack® syringe with a 25 × 7 mm needle, transferred without the needle to EDTA VACUETTE® tubes, homogenized manually, and refrigerated. Placenta samples were collected immediately after delivery and the umbilical cord was clamped and removed with scissors for weighing on a Soehnle Multina Plus electronic baby scale (Soehnle®, Gaildorfer, Germany). Maternal decidua and placental villi tissues were extracted from the central cotyledon, and the tissues were separated with scissors, forceps, and magnifying glass on a Petri dish. Approximately 0.1 g of the maternal decidua and placental villi tissues were stored separately in sterile Eppendorf® tubes containing 1 ml of RNAlater™ Stabilization Solution (Life Technologies, Vilnius, LT). Approximately 0.3 g of maternal decidua and placental villi tissues were stored separately in sterile Eppendorf tubes containing 1 ml of phosphate-buffered saline (PBS 1X). The tubes were frozen at −80°C for subsequent RNA and DNA extraction.
2.6. DNA extraction
Total genomic DNA was extracted from maternal blood, cord blood, maternal decidua and placental villi tissues samples using proteinase K (Invitrogen™, Carlsbad, CA, USA) according to the manufacture's protocol, followed by a modified salting-out method (18). The extracted DNA was quantified in a Nanodrop spectrophotometer (Thermo Fisher Scientific Inc., Santa Clara, CA, USA). Samples with an OD260:OD280 ratio higher than 1.8 and an OD260:OD230 ratio between 1.8 and 2.2 were classified as pure. DNA integrity was evaluated by electrophoresis on 2.0% agarose gel with ethidium bromide and diluted to a concentration of ~50 ng/μL.
2.7. Methylation analysis
The methylation-sensitive restriction endonuclease qPCR method was used to determine the level of H19DMR methylation. The H19DMR sequence obtained from the ENSEMBL database (https://www.ensembl.org/index.html) was used for analysis. The region amplified by the primers comprises a 209 bp sequence located at 4,447 bp after the transcription start site, within the imprinting control region (ICR1) located in the H19/IGF2 locus (Supplementary Figure S1). CpGs islands were predicted with MethPrimer2.0 (http://www.urogene.org/methprimer2/) according to the software's parameters. Primers were designed using the Primer3Plus software, Beacon Designer (http://www.premierbiosoft.com/qOligo/Oligo.jsp?PID=1) for analysis of secondary structures, and Primer-BLAST (https://www.ncbi.nlm.nih.gov/tools/primer-blast/) to confirm specificity. The H19DMR primer was designed to flank the restriction site of the methylation-sensitive endonuclease MspI/HpaII (FERMENTAS FastDiges®, Waltham, EUA) at one single cleavage site (Supplementary Table S1).
A set of genomic DNA templates were generated from the samples. Each set consisted of three tubes with equal DNA concentrations submitted to the methylation-sensitive enzyme HpaII, the non-methylation-sensitive enzyme MspI, and a non-enzyme digest (mock). The reaction mixture contained 1 U of enzyme per 200 ng of DNA and 1 × CutStart Buffer, in a total reaction volume of 20 μL. For the non-enzyme control, ultrapure water was added instead of enzyme. All prepared samples were incubated at 37°C for 16 h, followed by heat inactivation at 65°C for 5 min.
The qPCR for methylation was performed in a StepOne Real-Time PCR System (Thermo Fisher Scientific, USA) using 5 μL of Power SYBR® Green PCR Master Mix (Applied Biosystems), 2 pmol of each primer (forward and reverse primers; Supplementary Table S1), 2 μL of DNA template, and ultrapure water, in a total volume of 10 μL. The qPCR conditions were: denaturation at 95°C for 10 s, followed by 40 cycles at 95°C for 5 s and annealing and elongation at 60°C for 30 s. Amplification efficiencies were obtained by standard curve analysis and specificities were evaluated by melting curve analysis using the program from 70 to 95°C at 0.3°C/s. The percentage of methylation was determined using the formula (½)Ctd − Ctnd, where Ctd is the cycle threshold (Ct) of the HpaII-digested DNA and Ctnd is the Ct of the non-enzyme control (19).
2.7.1. Restriction site confirmation
Prior to DNA methylation analysis, polymerase chain reaction-restriction fragment length polymorphism (PCR-RFLP) analysis was carried out to confirm the presence of the restriction site of HpaII/MspI (C∧CGG) in the populations studied. The PCR for each gene was performed in a volume of 25 μL containing 1 μL of 10 × PCR buffer, 1 mM MgCl2, 0.2 mM dNTPs, 1 U of Taq DNA polymerase, 10 pmol of each primer, and 100 ng of genomic DNA. The thermal cycling conditions were 5 min at 94°C, followed by 35 cycles at 94°C for 1 min, 60°C for 40 s, 72°C for 1 min, and a final extension at 72°C for 5 min. The PCR product was digested with 2 U of HpaII in 1 μL of 10 × CutStart buffer and 5 μL of water at 37°C for 16 h, followed by heat inactivation at 65°C for 20 min. The PCR and RFLP products were visualized by 2% agarose gel electrophoresis with ethidium bromide.
2.8. mRNA extraction and cDNA synthesis
For extraction of total mRNA, 100 mg of frozen maternal decidua and placental villi samples were macerated in liquid nitrogen and extracted using the PureLink™ RNA Mini Kit (Thermo Fisher Scientific, Carlsbad, CA, USA) following the manufacturer's protocol. Samples with an OD 260:OD280 ratio higher than 1.8 were classified as pure. RNA integrity was confirmed by 1.5% agarose gel electrophoresis for 90 min. First-strand cDNA was synthesized from 4 μg of total RNA using the SuperScript® IV First-Strand Synthesis System (Thermo Fisher Scientific, Vilnius, LT) and oligo dT primers according to manufacturer's recommendations.
2.9. Gene expression analysis
For gene expression analysis of the H19, tyrosine 3-monooxygenase/tryptophan 5-monooxygenase activation protein zeta (YWHAZ) and glyceraldehyde-3-phosphate dehydrogenase (GAPDH) genes, primers were designed using the Primer3Plus software, Beacon Designer (http://www.premierbiosoft.com/qOligo/Oligo.jsp?PID=1) for the analysis of secondary structures and Primer-BLAST (https://www.ncbi.nlm.nih.gov/tools/primer-blast/) for the confirmation of exon-exon junction specificity. The IGF2 primers were described by (8) Supplementary Table S1.
The quantitative PCR (qPCR) assay was performed with the StepOne Real-Time PCR System (Applied Biosystems, USA) using 10 μL of SYBR Green PCR Master Mix (Applied Biosystems), 1 μL (2 pmol) of each primer, and 2 μL of 1:11 diluted cDNA. The amplification conditions for all primers were 95°C for 10 min, followed by 45 cycles at 95°C for 15 s and 65°C for 1 min. A melting curve stage of 70–95°C was added in all qPCR assays to verify their specificity. The reactions were analyzed in duplicate and negative controls were included to detect contamination. Primer efficiency was obtained by linear regression [efficiency = 10 (−1/slope)] and primers with an efficiency of 90–110% were considered for gene expression analysis. The average Ct was collected and the 2-ΔΔCT was calculated (20) for each sample to obtain the fold-change. The GAPDH and YWHAZ reference genes were used for normalization.
2.10. Statistical analysis
Descriptive statistics (mean and standard deviation (SD), frequency, and percentage) were used to summarize the data. The Shapiro-Wilk test was applied to analyze the normality of the data. The t-test for independent samples was used for comparison between groups and the effect size (Glass' delta) was calculated. Categorical variables were compared between the two groups of pregnant women by the chi-square test. Spearman's correlation test was used to investigate the correlation of mean DNA methylation and gene expression levels in each tissue with maternal overweight/obesity, fetal biometry and body composition, and newborn anthropometry. Univariate and multiple linear regression models were used to explore the associations of DNA methylation and gene expression levels in each tissue with markers of fetal biometry and body composition and newborn anthropometry. The outcome measures were BPD, OFD, FL, HL, length, HC, AC, SCFT, total thigh tissue, thigh muscle mass, subcutaneous thigh fat, total arm tissue, arm muscle mass, and subcutaneous arm fat of the fetus at T2 and T3, as well as weight, length, HC, PT, and AC of the newborns at T4. The confounding variables included maternal age, pre-pregnancy BMI, gestational weight gain, gestational age, and newborn sex. Statistical significance was established at p < 0.05 and analysis was performed using SPSS 18.0 (SPSS, Chicago, IL, USA).
3. Results
Table 1 shows the demographic, socioeconomic, and obstetric characteristics of overweight/obese and normal weight pregnant women and their respective newborns. No significant difference was found in age, race, education, parity, or morbidity during pregnancy between groups. Newborns in both groups were similar for gestational age but differed significantly for sex (p = 0.021).
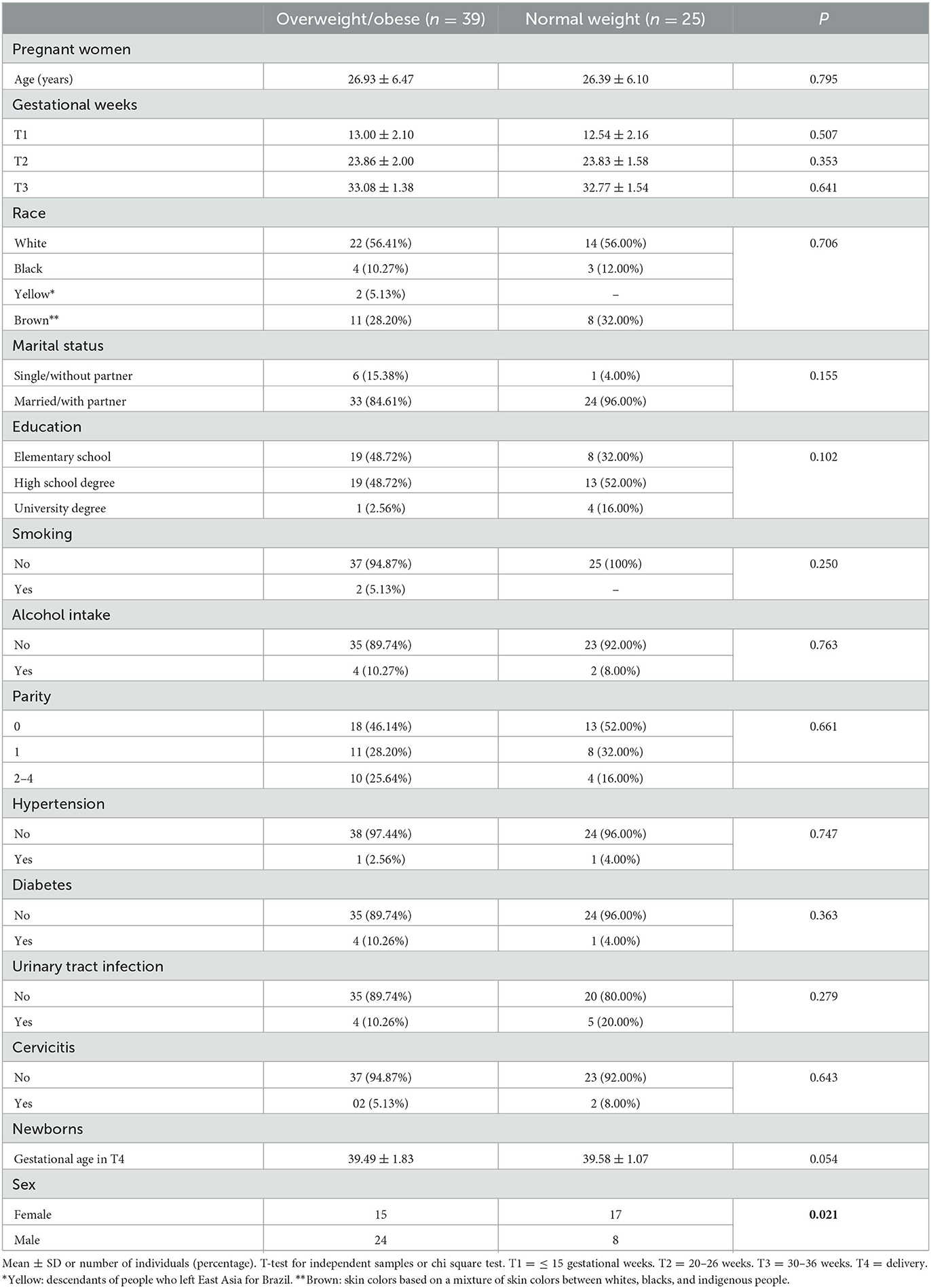
Table 1. Demographic, socioeconomic, and obstetric characteristics of overweight/obese, and normal weight pregnant women and their newborns.
Table 2 shows that pre-pregnancy weight (p = 0.019) and pre-pregnancy BMI (p = 0.018), as well as the amount of pre-pregnancy fat mass (p = 0.007), were higher in the overweight/obese group compared to the normal weight group. The pregnancy BMI at T2, T3, and T4 remained higher in the overweight/obese group but the total gestational weight gain did not differ significantly between groups. Fetuses of overweight/obese pregnant women showed higher centiles of HC at T2 (p = 0.045), BPD (p = 0.049) at T3, and OFD at T3 (p = 0.049) compared to the normal weight group. Fetal body composition parameters such as SCFT (p = 0.021), total arm tissue (p = 0.033), arm muscle mass (p = 0.046), and arm subcutaneous fat (p = 0.029) at T2 were higher in the overweight/obese group compared to the normal weight group. Regarding newborn parameters, HC (p = 0.046) was significantly higher in the overweight/obese group compared to the normal weight group. According to the results of the Glass' delta, all maternal, fetal and newborn parameters that showed statistically significant results in the t-test, had effect sizes considered very large and/or medium.
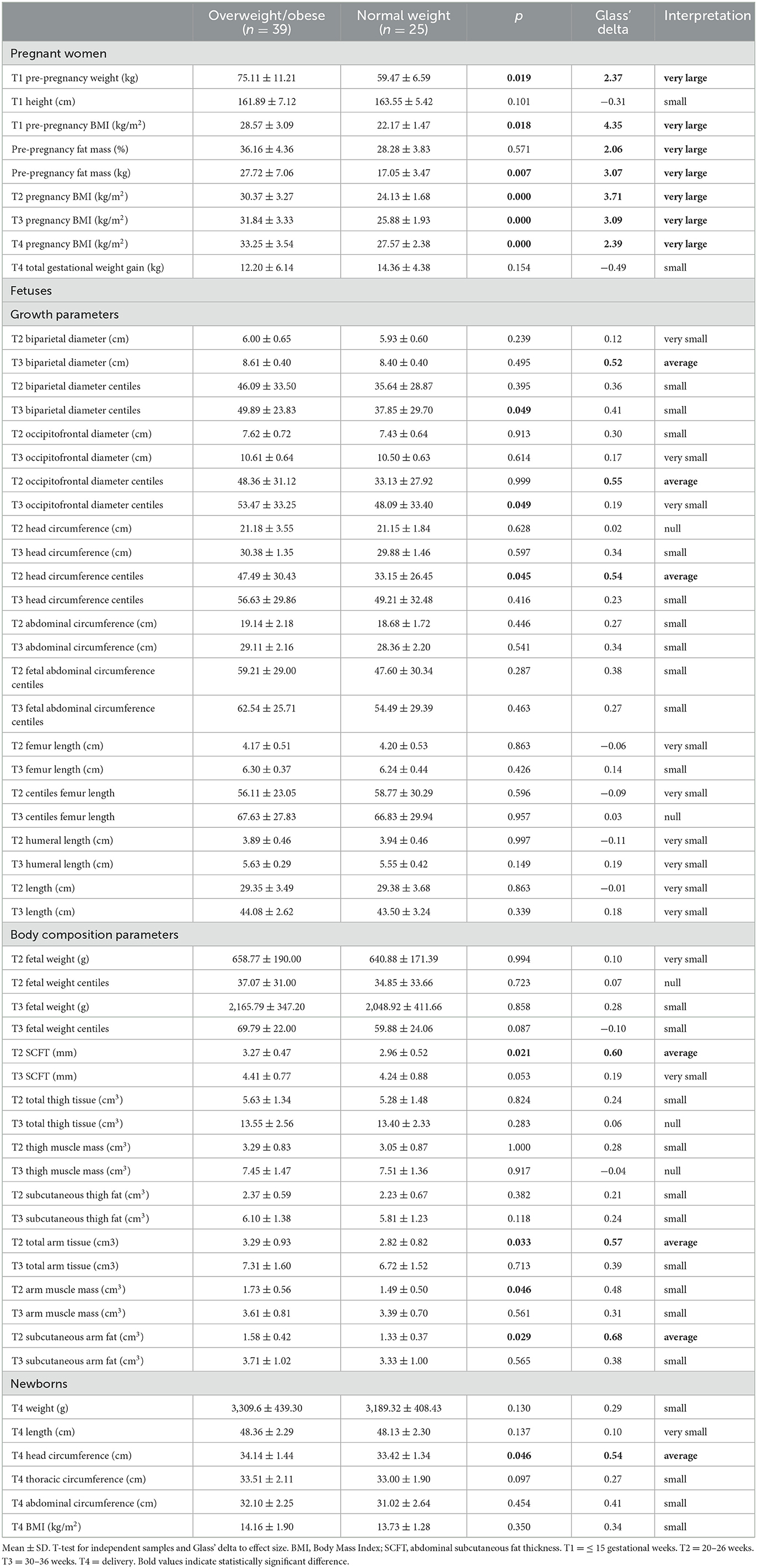
Table 2. Anthropometry and body composition of overweight/obese and normal weight pregnant women and of their fetuses and newborns.
The PCR-RFLP demonstrated that there were no variations in the homologous DNA sequences of the H19DMR, implying the absence of genetic bias. In samples that had variations, the final methylation percentages could be altered due to the gene polymorphism and not to the environmental factor of interest. Figure 1A shows the mean DNA methylation level of H19DMR in maternal blood, cord blood, maternal decidua and placental villi of the overweight/obese and normal weight groups. H19DMR was found to be significantly less methylated (p = 0.038) in maternal blood of the overweight/obese group compared to the normal weight group, but no significant difference between groups was observed for the other tissues (p = 0.553 cord blood; p = 0.344 placental villi; p = 0.608 maternal decidua). Figures 1B, C shows the mean relative gene expression of the H19 and IGF2 genes, respectively, in the maternal decidua and placental villi of the overweight/obese and normal weight groups. There was no difference in gene expression between groups (p = 0.568, H19 placental villi; p = 0.705, IGF2 placental villi; p = 0.705, H19 maternal decidua; p = 0.234, IGF2 maternal decidua). Bonferroni's post-hoc test also revealed no difference in methylation or gene expression in the different tissues between groups. There was a positive correlation between H19DMR methylation in placental villi and in maternal decidua (ρ = 0.788, p = 0.001), between H19 and IGF2 gene expression in maternal decidua (ρ = 0.841, p = 0.001), and between H19 and IGF2 gene expression in placental villi (ρ = 0.912, p = 0.001). Correlations of DNA methylation and gene expression with fetal and newborn parameters, and correlations of DNA methylation itself and with gene expression in different tissues, are shown in Supplementary Tables S2A, B, respectively.
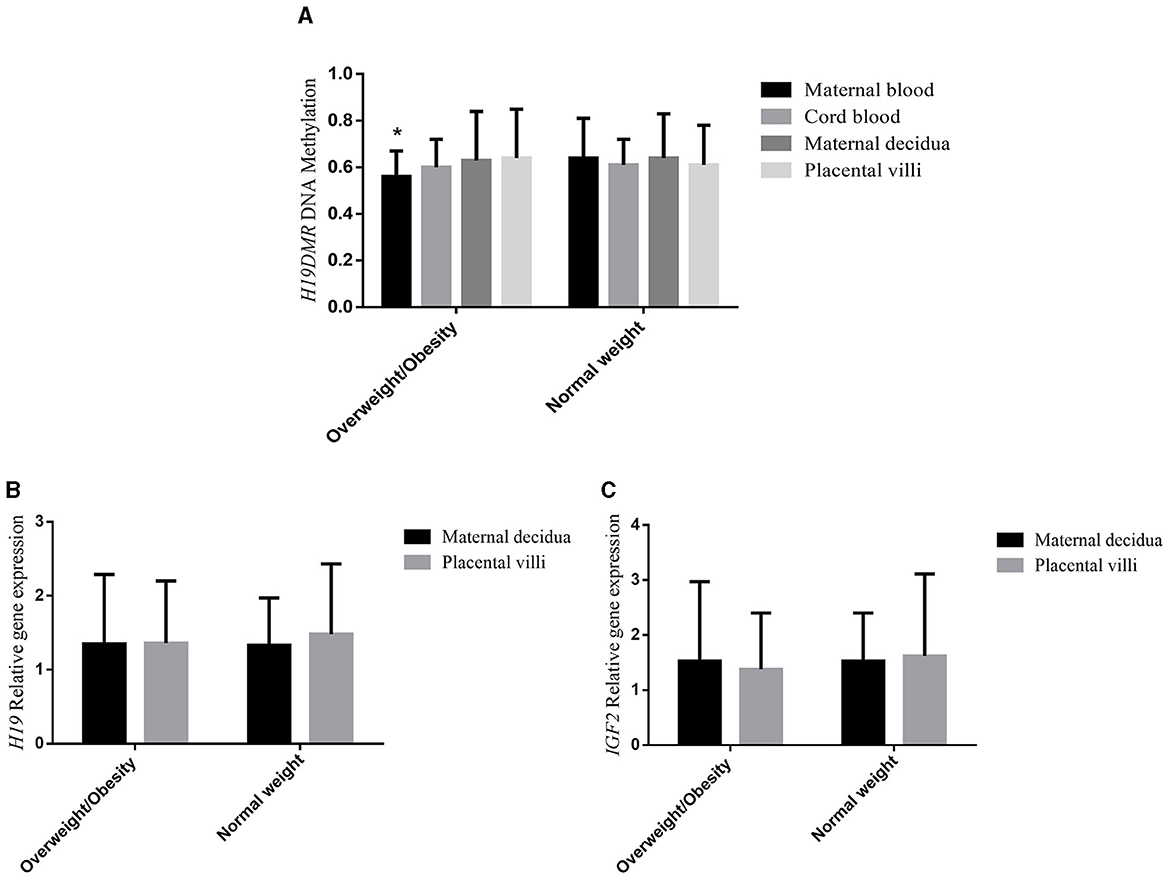
Figure 1. (A) H19DMR methylation, (B) H19 gene expression, and (C) IGF2 gene expression of overweight/obese, and normal weight pregnant women in maternal blood, cord blood, maternal decidua, and placental villi tissues. ANOVA with Bonferroni's post-hoc test. *p < 0.05.
Table 3 shows the associations of mean H19DMR methylation level in different tissues with fetal growth and body composition and newborn anthropometric parameters. There were significant associations of BPD centiles at T2 with methylation in cord blood (p = 0.026); OFD at T3 with methylation in placental villi (p = 0.001) and in maternal decidua (p = 0.019); OFD centiles at T3 with methylation in placental villi (p = 0.001) and in maternal decidua (p = 0.023); HC at T2 with methylation in placental villi (p = 0.004) and in maternal decidua (p = 0.041); HC at T3 with methylation in placental villi (p = 0.009); HC centiles at T3 with methylation in placental villi (p = 0.007); length at T2 with methylation in maternal decidua (p = 0.050); SCFT at T3 with methylation in cord blood (p = 0.004) and in placental villi (p = 0.037), and HC at T4 with methylation in cord blood (p = 0.016) and in placental villi (p = 0.020).
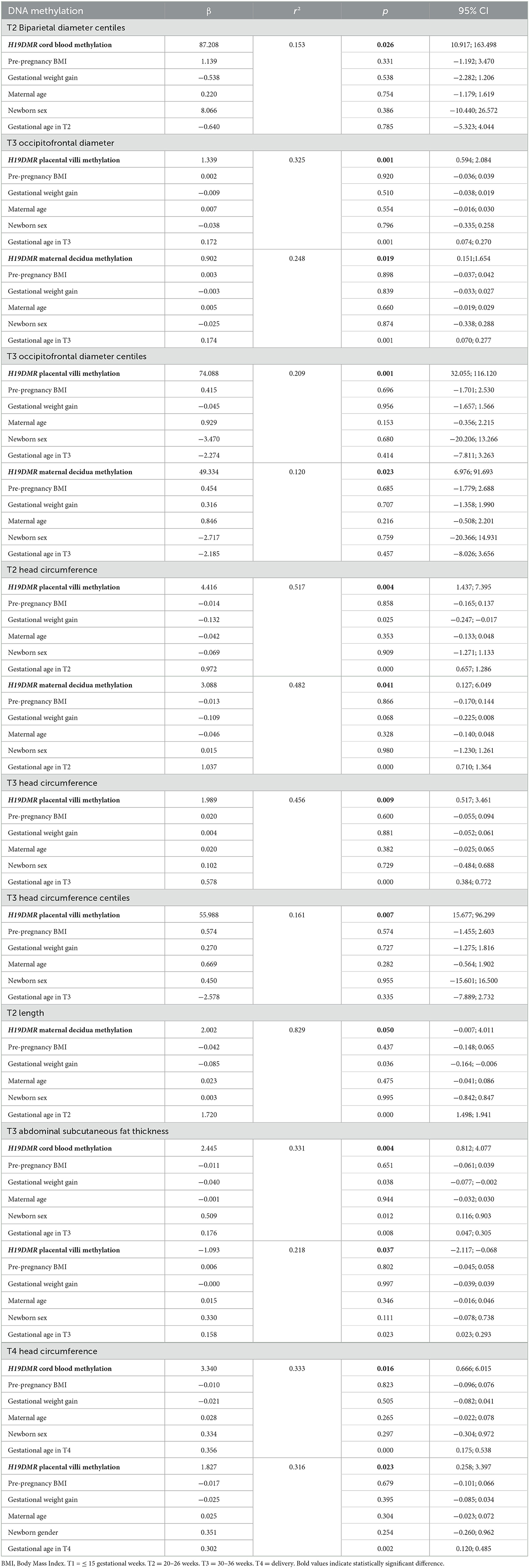
Table 3. Multiple linear regression models showing the associations of mean H19DMR methylation in maternal blood, cord blood, maternal decidua and placental villi tissues with fetal and newborn parameters.
We also found significant associations (Table 4) of the mean H19 and IGF2 gene expression levels in different tissues with fetal growth and body composition and newborn anthropometric parameters: BPD at T3 with H19 expression in maternal decidua (p = 0.018) and with IGF2 expression in maternal decidua (p = 0.011); BPD centiles at T3 with H19 expression in maternal decidua (p = 0.011) and with IGF2 expression in maternal decidua (p = 0.007); OFD at T2 with H19 (p = 0.022) and IGF2 expression in placental villi (p = 0.016); OFD centiles at T2 with H19 (p = 0.006) and IGF2 expression in placental villi (p = 0.005); FL centiles at T2 with H19 expression in maternal decidua (p = 0.041), and subcutaneous arm fat at T3 with H19 expression in placental villi (p = 0.033).
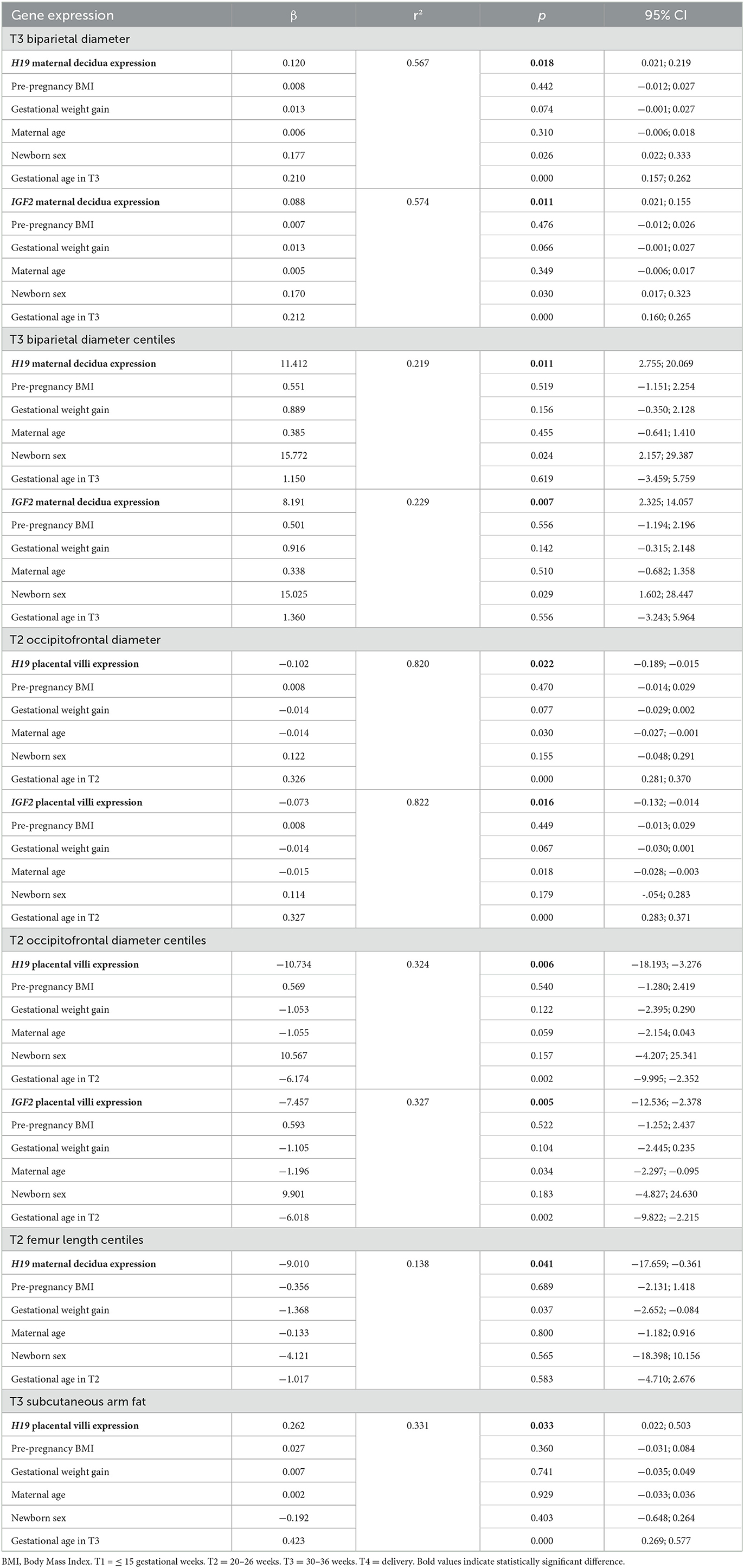
Table 4. Multiple linear regression models showing the associations of mean H19 and IGF2 gene expression in maternal decidua and placental villi tissues with fetal and newborn parameters.
4. Discussion
In this study we seek to understand how maternal overweight/obesity affects DNA methylation in an important ICR and expression of genes possibly regulated by this ICR in different tissues. Furthermore, we wanted to understand how methylation of H19DMR and expression of the H19 and IGF2 genes are associated with the fetal and newborn parameters assessed in this study.
Studies have investigated the role of maternal overweight/obesity in fetal metabolic programming. BMI was found to be associated with changes in DNA methylation in genetic loci in adults, as well as with the level of methylation at CpGs sites (21). However, to our knowledge, there are no prospective studies on the relationship between maternal and fetal genetic information of H19DMR, H19 and IGF2 in different maternal-fetal tissues and fetal body composition. It is known that the higher the BMI, the greater the risk of developing metabolic diseases, especially gestational diabetes and hypertension (22). Despite these risks, we did not find significant numbers of pregnant women who became diabetic or hypertensive, or who had any chronic diseases during pregnancy. In the present study, pregnant women in the overweight/obese group had a higher pre-pregnancy BMI and maintained a high BMI at all time points until the end of pregnancy when compared to pregnant women with a normal pre-pregnancy BMI.
There was no difference in age, race, education, parity or lifestyle between overweight/obese and normal weight pregnant women, considering that both groups were healthy and young. However, one parameter that differed between groups was the sex of the newborn, with a larger number of male newborns in the overweight/obese group and of female newborns in the normal weight group. The literature suggests a relationship between sex and body composition at birth (23) and we therefore chose to evaluate the variables also as centiles since this approach takes into account sex. In addition, we included newborn sex as a confounding variable in the multiple linear regression models. We found higher HC centiles at T2 and higher BPD and OFD centiles at T3 in the overweight/obese group.
The NICHD Fetal Growth Studies-Singletons assessed the association between maternal obesity and longitudinal measurements of fetal growth. Similar to our results the authors observed higher HC in fetuses born to obese women compared to fetuses in the non-obese group, but no difference for fetal BPD. However, this difference occurred between the 33rd and 35th gestational week, while in our study this difference was observed at an earlier time (T2) and at birth (T4) (24).
In addition to biometric parameters for investigating fetal growth, we also used ultrasound to assess fetal body composition. The development of adipose tissue starts to become more important by the 24th week and increases rapidly in the third trimester of gestation (25). Body mass is defined by ultrasound as a two-compartment model that considers fat mass and lean mass as areas of interest. Body tissues are quantified by analyzing the thickness of the subcutaneous tissue; however, a limitation of 2D ultrasound is the inability to characterize visceral fat deposits, which can be of metabolic importance during the fetal period and other periods of life (26).
Regarding fetal body composition, our findings were more specific at T2. At this time point, adipose parameters such as SCFT and fetal subcutaneous arm fat, as well as body composition parameters such as total arm tissue and arm muscle mass, were higher in the group of overweight/obese women. Studies have shown that SCFT can predict fetal macrosomia, SCFT values higher than 6.25 mm were sensitive in predicting large-for-gestational-age babies (27). In our study, SCFT did not remain elevated at T3 and the highest value found was 4.41 mm in the group of overweight/obese pregnant women at T3; thus, our babies were not born with macrosomia. Although ultrasound measurements few days before delivery are important, we do not have such measurements. However, the newborns of this study were not large-for-gestational age, although newborns in the overweight/obese group were on average 120 g heavier than those in the normal weight group.
A retrospective study that investigated SCFT from the second trimester to delivery showed higher values in obese pregnant women (pre-pregnancy BMI 35.4 ± 5.1 kg/m2) compared to non-obese women (pre-pregnancy BMI 29.6 ± 4.4 kg/m2) (28). In our study, overweight and obese women were selected according to their pre-pregnancy BMI and the difference in fat mass between overweight/obese and normal weight pregnant women was significant (15.64 vs. 10.67 kg; p = 0.007). An important fact is that gestational weight gain was similar in the two groups. Therefore, we mainly evaluated the impact of pre-pregnancy BMI and not of weight gain on the fetal and newborn parameters. Within this context, high maternal gestational weight gain can increase nutrient availability to the fetus, with consequent changes in fetal growth parameters and adiposity. However, in this study, although not always significant, all parameters were higher in offspring of overweight/obese pregnant women.
Regarding the methylation of H19DMR in different maternal (maternal peripheral blood and maternal decidua) and fetal (cord blood and placental villi) tissues, we found that this ICR was less methylated in maternal blood of obese/overweight pregnant women compared to the normal weight group. There was no difference in methylation between groups in the other tissues. The hypomethylation of H19 was described in intrauterine growth restriction which, in turn, would affect the biological potential of fetal growth (29). The lower methylation in maternal blood did not negatively regulate fetal or newborn growth in this study. Tabano et al. reported a minimum percentage of H19DMR methylation of 44% in peripheral blood of healthy human adults (30). In our study, lower methylation was observed in the peripheral blood of overweight/obese women (mean level 56%) compared to the normal weight group. However, we emphasize that such values are not directly comparable, since the method used for methylation detection was semiquantitative, while other studies use quantitative methods, such as pyrosequencing. Thus, we therefore believe that this result did not cause a negative impact on newborn growth in this group.
The H19 gene has been suggested to play a role in cancer pathogenesis and is involved in genetic syndromes, as well as in maternal epigenetics and fetal and neonatal growth. Its role in the maternal metabolic and dietary context has also been studied (31). Thus, in addition to weight, maternal metabolic and dietary data would contribute to a better understanding of the modifications of H19DMR in maternal blood. The Newborn Epigenetics Study (NEST) cohort demonstrated higher estimated mean H19DMR methylation levels in cord blood of newborns from obese mothers compared to non-obese mothers (32). Newborns with weight-for-age > 85th centiles had higher H19DMR methylation levels in cord blood than newborns with weight-for-age <85th centiles (7).
In genomic imprinting, only one allele is expressed in the case of certain genes. In humans, a group of genes regulated by genomic imprinting was mapped to the short arm of chromosome 11, in the 11p15.5 region. Two imprinting control regions, one of which is ICR1 that, among other genes, harbors the IGF2 and H19 tumor suppressor genes, were also mapped. ICR1 is methylated on the paternal allele and is regulated by the H19 gene. In the absence of methylation on the maternal allele, enhancers can access the 3′-end of the H19 gene, enabling the binding of a protein or CCCTC-binding factor (CTCF) to ICR1. The CTCF protein acts as an insulator, blocking the access of enhancers to the 3′-end of the IGF2 gene. In the maternal allele, the H19 gene is expressed and the IGF2 gene is silenced. Methylation on the paternal allele blocks the binding of the CTCF protein to ICR1, inhibiting its insulator function and allowing access of enhancers to the 3′-end of the IGF2 gene, with consequent expression of this gene and silencing of H19. The expression of imprinted genes is facilitated by asymmetric epigenetic markers on the maternal or paternal allele, forming clusters that contain DMRs. One of the most studied markers is DNA methylation (10). In addition, H19 is a non-coding RNA, ultimately it would block the transcription of IGF2 tending to reduce fetal growth, but not producing a protein. We emphasize that in this study the region that we call H19DMR is located within ICR1.
We therefore also analyzed the gene expression of H19 and IGF2 in placental villi and maternal decidua; however, we did not find a difference in the expression of these genes between overweight/obese and normal weight women. The same was observed for tissue analysis, with no difference in H19DMR methylation in cord blood, or placental villi and maternal decidua. However, correlation analysis showed strong positive correlations between H19DMR methylation in placental villi and in maternal decidua, between H19 and IGF2 gene expression in maternal decidua, and between H19 and IGF2 gene expression in placental villi. In other words, this study shows a positive correlation between the two tissues studied (maternal decidua and placental villi), with similar patterns of methylation and gene expression.
Despite the theoretical understanding of the genomic imprinting of H19 and IGF2, the presence of contrasting results in the literature indicates that there is not always a direct correlation between methylation and the expression of imprinted genes; thus, other mechanisms may be involved in this pathway. Methylation of the IGF2 and H19 promoters is not a prerequisite for the regulation of the imprinting domain that controls IGF2 and H19 transcription in the human placenta (33). Extraembryonic hypomethylation of H19 had no impact on the expression pattern of IGF2 or H19 (30).
In our study, the methylation of H19DMR in cord blood was associated with the centiles of BPD at T2, SCFT at T3, and HC at T4. The methylation of H19DMR in maternal decidua was associated with OFD at T3, OFD centiles at T3, HC at T2, and length at T2. The methylation of H19DMR in placental villi was associated with OFD at T3, OFD centiles at T3, HC at T2 and T3, HC centiles at T3, SCFT at T3, and HC at T4. We also found an association of H19 gene expression in maternal decidua with BPD at T3, BPD centiles at T3, and FL centiles at T2, as well as an association of H19 expression in placental villi with OFD at T2, OFD centiles at T2, and subcutaneous arm fat at T3. IGF2 gene expression in maternal decidua was associated with BPD at T3 and BPD centiles. In addition, there was an association of IGF2 gene expression in placental villi with OFD at T2 and OFD centiles at T2. A study using term placenta and chorionic villus samples found that IGF2 expression in term placentas was not correlated with fetal growth parameters, while IGF2 expression in chorionic villus samples was correlated with crown rump length and birth weight (5). Another study analyzed the effect of maternal factors on the methylation of H19DMR in cord blood and also found changes in DNA methylation to be associated with HC (34).
In the present study, we highlight that: high pre-pregnancy BMI regulated H19DMR methylation in maternal blood and altered some parameters of fetal and newborn growth and fetal adiposity; IGF2 and H19 gene expression did not seem to be the link between obesity and the offspring outcomes investigated; and H19DMR methylation and expression of IGF2 and H19 genes in cord blood and especially in maternal decidua and placental villi regulated several parameters of fetal growth and body composition. To our knowledge, this is the first study to demonstrate associations of imprinted genes variations at the maternal-fetal interface of the placenta and in cord blood with fetal body composition, supporting the involvement of epigenetic mechanisms in offspring growth and body composition.
Strengths of our study include specific evaluation of fetal adiposity by ultrasonography and assessment of maternal blood and cord blood methylation, and maternal decidua and placental villi tissues methylation and gene expression. Limitations of this study were the non-determination of gene expression in maternal and cord blood, the small sample size, and the semi-quantitative method for assessing DNA methylation.
Data availability statement
The raw data supporting the conclusions of this article will be made available by the authors, upon request.
Ethics statement
The studies involving humans were approved by Research Ethics Committee of the School of Public Health, University of São Paulo (protocol number 2.570.576). This study was conducted in compliance with the Helsinki guidelines. The studies were conducted in accordance with the local legislation and institutional requirements. Written informed consent for participation in this study was provided by the participants' legal guardians/next of kin. Written informed consent was obtained from the individual (s), and minor (s)' legal guardian/next of kin, for the publication of any potentially identifiable images or data included in this article.
Author contributions
PR, PA, ER, and JM designed the research. PR coordinated the field work. PA, PN, LT, LB, ML, and LL participated in the collection of clinical data. PA and ND participated in the collection of biological material. PA, JM, and ER performed the experiments, analysis, and interpretation of DNA methylation and expression genic data. PA and PR directed statistical analysis. PA and JM wrote the first draft of the manuscript. PR and ER reviewed the manuscript and provided critical revision. All authors read and approved the final manuscript.
Funding
This study was supported by the São Paulo Research Foundation (FAPESP), grant number 2015/03333-6. PA received Doctorate scholarships from CAPES and FAPESP (grant number 2018/17824-0). ND received Postdoctoral fellowship from FAPESP (grant number 2017/07143-2). FAPESP and CAPES had no role in the design, analysis, or writing of this manuscript.
Acknowledgments
We would like to thank the Health Secretary of Araraquara city Mrs. Eliana Mori Honain for allowing us to carry out our study in the Municipal Maternity Hospital and health centers of the city. We are especially grateful to Dr. Walter Manso Figueiredo, director of the Special Health Care Service of Araraquara (SESA), Lúcia Ortiz, Dr. Ademir Roberto Sala, and Ângela Aparecida Costa for their help with the data collection. We also thank all pregnant women and their babies who participated in this study.
Conflict of interest
The authors declare that the research was conducted in the absence of any commercial or financial relationships that could be construed as a potential conflict of interest.
Publisher's note
All claims expressed in this article are solely those of the authors and do not necessarily represent those of their affiliated organizations, or those of the publisher, the editors and the reviewers. Any product that may be evaluated in this article, or claim that may be made by its manufacturer, is not guaranteed or endorsed by the publisher.
Supplementary material
The Supplementary Material for this article can be found online at: https://www.frontiersin.org/articles/10.3389/fnut.2023.1170411/full#supplementary-material
Supplementary Figure S1. Amplification region of the H19DMR primers.
Supplementary Table S1. Methylation primers and gene expression.
Supplementary Table S2. (A) Correlations of mean H19DMR methylation in maternal blood, cord blood, maternal decidua and placental villi tissues with fetal and newborn parameters. (B) Correlation of DNA methylation itself with gene expression in maternal blood, cord blood, maternal decidua and placental villi tissues.
References
1. World Health Organization. Nutrition: Obesity and Overweight. WHO (2020). Available online at: https://www.who.int/news-room/fact-sheets/detail/obesity-and-overweight (accessed April 25, 2022).
2. Goldstein RF, Abell SK, Ranasinha S, Misso ML, Boyle JA, Harrison CL, et al. Gestational weight gain across continents and ethnicity: systematic review and meta-analysis of maternal and infant outcomes in more than one million women. BMC Med. (2018) 16:1–14. doi: 10.1186/s12916-018-1128-1
3. Girirajan S, Campbell C, Eichler E. Impact of maternal body mass index and gestational weight gain on pregnancy complications: an individual participant data meta-analysis of European, North American and Australian cohorts. Physiol Behav. (2011) 176:139–48.
4. Lambertini L, Marsit CJ, Sharma P, MacCani M, Ma Y, Hu J, et al. Imprinted gene expression in fetal growth and development. Placenta. (2012) 33:480–6. doi: 10.1016/j.placenta.2012.03.001
5. Moore GE, Ishida M, Demetriou C, Al-Olabi L, Leon LJ, Thomas AC, et al. The role and interaction of imprinted genes in human fetal growth. Philos. Trans. R. Soc. B Biol. Sci. (2015) 370:74 doi: 10.1098/rstb.2014.0074
6. Koukoura O, Sifakis S, Spandidos DA. DNA methylation in the human placenta and fetal growth (review). Mol Med Rep. (2012) 5:883–9. doi: 10.3892/mmr.2012.763
7. Perkins E, Murphy SK, Murtha AP, Schildkraut J, Jirtle RL, Demark-Wahnefried W, et al. Insulin-like growth factor 2/H19 methylation at birth and risk of overweight and obesity in children. J Pediatr. (2012) 161:31–9. doi: 10.1016/j.jpeds.2012.01.015
8. Su R, Wang C, Feng H, Lin L, Liu X, Wei Y, Yang H. Alteration in expression and methylation of IGF2/H19 in placenta and umbilical cord blood are associated with macrosomia exposed to intrauterine hyperglycemia. PLoS ONE. (2016) 11:e0148399. doi: 10.1371/journal.pone.0148399
9. Kurukuti S, Tiwari VK, Tavoosidana G, Pugacheva E, Murrell A, Zhao Z, et al. binding at the H19 imprinting control region mediates maternally inherited higher-order chromatin conformation to restrict enhancer access to Igf2. Proc Natl Acad Sci USA. (2006) 103:10684–9. doi: 10.1073/pnas.0600326103
10. Beatty L, Weksberg R, Sadowski PD. Detailed analysis of the methylation patterns of the KvDMR1 imprinting control region of human chromosome 11. Genomics. (2006) 87:46–56. doi: 10.1016/j.ygeno.2005.05.015
11. Leighton PA, Ingram RS, Eggenschwiler J, Efstratiadis A, Tilghman SM. Disruption of imprinting caused by deletion of the H19 gene region in mice. Nature. (1995) 375:34–9. doi: 10.1038/375034a0
12. DeChiara TM, Efstratiadis A, Robertsen EJ. A growth-deficiency phenotype in heterozygous mice carrying an insulin-like growth factor II gene disrupted by targeting. Nature. (1990) 345:78–80. doi: 10.1038/345078a0
13. Montoya-Williams D, Quinlan J, Clukay C, Rodney NC, Kertes DA, Mulligan CJ. Associations between maternal prenatal stress, methylation changes in IGF1 and IGF2, and birth weight. J Dev Orig Health Dis. (2018) 9:215–22. doi: 10.1017/S2040174417000800
14. Nawathe AR, Christian M, Kim SH, Johnson M, Savvidou MD, Terzidou V. Insulin-like growth factor axis in pregnancies affected by fetal growth disorders. Clin Epigenet. (2016) 8:1–13. doi: 10.1186/s13148-016-0178-5
15. Bouwland-Both MI, Van Mil NH, Stolk L, Eilers PHC, Verbiest MMPJ, Heijmans BT, et al. DNA methylation of IGF2DMR and H19 is associated with fetal and infant growth: the generation R study. PLoS ONE. (2013) 8:e0081731. doi: 10.1371/journal.pone.0081731
16. Institute of Medicine (US) and National Research Council (US) Committee to Reexamine IOM Pregnancy Weight Guidelines. In: Rasmussen KM, Yaktine AL, editors. Weight Gain During Pregnancy: Reexamining the Guidelines. Washington, DC: National Academies Press (US) (2009).
17. Papageorghiou AT, Ohuma EO, Altman DG, Todros T, Ismail LC, Lambert A, et al. International standards for fetal growth based on serial ultrasound measurements: The Fetal Growth Longitudinal Study of the INTERGROWTH-21st Project. Lancet. (2014) 384:869–79. doi: 10.1016/S0140-6736(14)61490-2
18. Olerup O, Zetterquist H. HLA-DR typing by PCR amplification with sequence-specific primers (PCR-SSP) in 2 hours: an alternative to serological DR typing in clinical practice including donor-recipient matching in cadaveric transplantation. Tissue Antigens. (1992) 39:225–35. doi: 10.1111/j.1399-0039.1992.tb01940.x
19. Gomes M V, Gomes CC, Pinto W, Ramos ES. Methylation pattern at the KvDMR in a child with Beckwith-Wiedemann syndrome conceived by ICSI. Am J Med Genet Part A. (2007) 143:625–9. doi: 10.1002/ajmg.a.31628
20. Giulietti A, Overbergh L, Valckx D, Decallonne B, Bouillon R, Mathieu C. An overview of real-time quantitative PCR: applications to quantify cytokine gene expression. Methods. (2001) 25:386–401. doi: 10.1006/meth.2001.1261
21. Sharp GC, Lawlor DA, Richmond RC, Fraser A, Simpkin A, Suderman M, et al. Maternal pre-pregnancy BMI and gestational weight gain, offspring DNA methylation and later offspring adiposity: findings from the Avon Longitudinal Study of Parents and Children. Int J Epidemiol. (2015) 44:1–17. doi: 10.1093/ije/dyv042
22. Lewandowska M, Wieckowska B, Sajdak S. Pre-pregnancy obesity, excessive gestational weight gain, and the risk of pregnancy-induced hypertension and gestational diabetes mellitus. J Clin Med. (2020) 9:1–13. doi: 10.3390/jcm9061980
23. O'Tierney-Ginn P, Presley L, Minium J, Hauguel Demouzon S, Catalano PM. Sex-specific effects of maternal anthropometrics on body composition at birth. Am J Obstet Gynecol. (2014) 211:292.e1-292.e9. doi: 10.1016/j.ajog.2013.10.832
24. Zhang C, Hediger ML, Albert PS, Grewal J, Sciscione A, Grobman WA, et al. Association of maternal obesity with longitudinal ultrasonographic measures of fetal growth: findings from the nichd fetal growth studies-singletons. JAMA Pediatr. (2018) 172:24–31. doi: 10.1001/jamapediatrics.2017.3785
25. Demerath EW, Fields DA. Body composition assessment in the infant. Am J Hum Biol. (2014) 26:291–304. doi: 10.1002/ajhb.22500
26. Bernstein I. Fetal body composition. Curr Opin Clin Nutr Metab Care. (2005) 8:613–7. doi: 10.1097/01.mco.0000170757.78737.74
27. Bhat RG, Nathan A, Amar R, Vasudeva A, Adiga P, Bhat PV, et al. Correlation of fetal abdominal subcutaneous tissue thickness by ultrasound to predict birth weight. J Clin Diagnostic Res. (2014) 8:9–11. doi: 10.7860/JCDR/2014/6498.4214
28. Yerlikaya-Schatten G, Feichtinger M, Stopp T, Huhn EA, Chalubinski K, Husslein P, et al. Trajectories of fetal adipose tissue thickness in pregnancies after gastric bypass surgery. Obes Surg. (2020) 30:96–101. doi: 10.1007/s11695-019-04115-9
29. Koukoura O, Sifakis S, Zaravinos A, Apostolidou S, Jones A, Hajiioannou J, et al. Hypomethylation along with increased H19 expression in placentas from pregnancies complicated with fetal growth restriction. Placenta. (2011) 32:51–7. doi: 10.1016/j.placenta.2010.10.017
30. Tabano S, Colapietro P, Cetin I, Grati FR, Zanutto S, Mandò C, et al. Epigenetic modulation of the IGF2/H19 imprinted domain in human embryonic and extra-embryonic compartments and its possible role in fetal growth restriction. Epigenetics. (2010) 5:313–24. doi: 10.4161/epi.5.4.11637
31. Chiu YH, Fadadu RP, Gaskins AJ, Rifas-Shiman SL, Laue HE, Moley KH, et al. Dietary fat intake during early pregnancy is associated with cord blood DNA methylation at IGF2 and H19 genes in newborns. Environ Mol Mutagen. (2021) 62:388–98. doi: 10.1002/em.22452
32. Soubry A, Schildkraut JM, Murtha A, Wang F, Huang Z, Bernal A, et al. Paternal obesity is associated with IGF2 hypomethylation in newborns: results from a Newborn Epigenetics Study (NEST) cohort. BMC Med. (2013) 11:1–10. doi: 10.1186/1741-7015-11-29
33. Guo L, Choufani S, Ferreira J, Smith A, Chitayat D, Shuman C, et al. Altered gene expression and methylation of the human chromosome 11 imprinted region in small for gestational age (SGA) placentae. Dev Biol. (2008) 320:79–91. doi: 10.1016/j.ydbio.2008.04.025
34. Miyaso H, Sakurai K, Takase S, Eguchi A, Watanabe M, Fukuoka H, et al. The methylation levels of the H19 differentially methylated region in human umbilical cords reflect newborn parameters and changes by maternal environmental factors during early pregnancy. Environ Res. (2017) 157:1–8. doi: 10.1016/j.envres.2017.05.006
Keywords: maternal obesity, fetal growth, fetal body composition, imprinted gene, DNA methylation, cord blood, maternal decidua, placental villi
Citation: Argentato PP, Marchesi JAP, Dejani NN, Nakandakare PY, Teles LdFdS, Batista LPR, Leitão MPC, Luzia LA, Ramos ES and Rondó PH (2023) The relationship between obesity-related H19DMR methylation and H19 and IGF2 gene expression on offspring growth and body composition. Front. Nutr. 10:1170411. doi: 10.3389/fnut.2023.1170411
Received: 20 February 2023; Accepted: 04 September 2023;
Published: 21 September 2023.
Edited by:
Hannah Ee Juen Yong, Singapore Institute for Clinical Sciences (A*STAR), SingaporeReviewed by:
Jaclyn Goodrich, University of Michigan, United StatesJun-hua Peng, Guangxi Medicinal Botanical Garden, China
Copyright © 2023 Argentato, Marchesi, Dejani, Nakandakare, Teles, Batista, Leitão, Luzia, Ramos and Rondó. This is an open-access article distributed under the terms of the Creative Commons Attribution License (CC BY). The use, distribution or reproduction in other forums is permitted, provided the original author(s) and the copyright owner(s) are credited and that the original publication in this journal is cited, in accordance with accepted academic practice. No use, distribution or reproduction is permitted which does not comply with these terms.
*Correspondence: Patricia Helen Rondó, phcrondo@usp.br