- Área de Investigación, Escuela de Nutrición, Universidad de la República, Montevideo, Uruguay
Background: Gut microbiota plays a potential role in human health and different disorders such as autism spectrum disorder (ASD). Therefore, we analyzed gut bacteria composition in children with ASD and typical development (TD), and its relationship with nutritional status and dietary intake.
Methods: A descriptive cross-sectional study was carried out in 3- to 12-year-old children (ASD = 30, TD = 28). Dietary intake (applying food frequency questionnaires) and body mass index-for-age (expressed in z-score) were determined. Children were divided into normal weight and excess weight (risk of overweight + overweight + obesity), and the ASD group was categorized into gluten- and casein-free diet (ASD-diet) or no diet (ASD-no diet). The relative abundance of gut bacteria was analyzed in fecal samples by 16S rRNA sequencing.
Results: Children with excess weight had lower Roseburia than normal weight. Fewer Bifidobacterium longum and higher Clostridium glycolicum were found in the ASD group compared with TD one. Participants with excess weight and ASD had lower Roseburia and Faecalibacterium prausnitzii and higher Eubacterium ventricosum and Flavonifractor plautii than the TD group with the same nutritional status. Positive and negative associations were found between the bacteria genus and species, and the intake of dairy, vegetable drinks, cereals with and without gluten, food source of proteins, fish, food source of fat, and coconut oil, in unadjusted models and after adjustment for age, diet/no diet, ASD/TD.
Conclusion: Significant differences in microbial community composition were found between children with ASD and TD, considering their nutritional status and dietary intake.
Introduction
Autism spectrum disorder (ASD) is a neurodevelopment condition that has had a rapidly increasing prevalence. However, there is no standard treatment due to its complex ethology, involving genetic and environmental factors (1, 2). In the last few decades, it has been recognized that gut microbiota plays a major role in human health and different disorders such as autism (3). Multiple cohort studies indicate that several inflammation-related disorders and neurodevelopmental diseases have been associated with alterations in the gut ecosystem, a condition known as dysbiosis (4, 5). For example, a greater relative abundance of certain bacteria such as Clostridium and Sutterella has been observed in children with ASD as opposed to typical development (TD) ones, but the findings from different investigations are still controversial (6).
Robust literature data show that there is a two-way communication between gut and the brain, in which microbiota, the enteric nervous system, autonomic nervous system, endocrine system, immune system, and central nervous system are involved (4). Bacterial metabolites have been shown to be implicated in the secretion of neurotransmitters that are part of memory, learning, and behavioral processes (7). Diet plays an important role in gut microbiota homeostasis and metabolism, and children with ASD have difficulties in maintaining a balanced diet due to multiple factors such as highly selective food preference and gastrointestinal problems (8). In addition, many families with children with ASD have chosen to follow a gluten- and casein-free diet (GCFD) under the unproven hypothesis that these proteins are metabolized into gluteomorphin and casomorphin and that, via a leaky gut, they bind to opiate receptors in the central nervous system causing autism symptoms (9). There is still no consensus on the use and effectiveness of this type of diet for treating autism, and additional studies are needed to describe the effects of the diet on gut microbiota (9–11).
Dietary intake can modulate gut microbiota throughout life, and this action would depend on the type and amount of foods chosen, which can inflect up to 60% of the microbiome composition since it provides countless substrates for microbial metabolism (12, 13). Furthermore, there are bacteria with specific enzymes that convert certain nutrients into different metabolites that influence brain function (14). For example, from the metabolism of tryptophan, it is possible to obtain indole which has a positive effect on mental health, but another metabolite such as indoxyl sulfate has been linked to the development of ASD.
In relation to food intake and its association with bacterial taxonomy, a study of European children (fed with a Western diet rich in animal protein) and African children (fed with local vegetables and whole grains) showed that children in Burkina Faso have higher levels of Prevotella and lower levels of Bacteroides and Enterobacteriaceae than children from Italy (15). This diet and intestinal microbiota in rural African children have been linked to lower inflammatory conditions and infectious colonic diseases (11, 15). On the contrary, it has been shown that diets with a high intake of red meat, refined carbohydrates and fat, and a lower consumption of fish and vegetables could cause dysbiosis (13).
Moreover, scientific evidence has shown that nutritional status is related to gut microbiota (16). There is a lack of consensus as regards a healthy-type taxonomic microbiome composition, but recent studies show that there is a difference in gut bacteria between obese and lean children and adolescents (17). Bervoets et al. (18) observed that children with obesity had a higher level of Lactobacillus spp. and a lower level of Bifidobacterium vulgatus than the lean ones. A prospective study showed that obesity in children was associated with an increase in Bacteroidaceae and a lower relative abundance of Prevotellaceae compared with children with normal weight (16). The relation between gut bacteria and weight gain is still unclear (12).
It has been shown that early intervention in children's gut microbiota can help prevent health disorders, but it is necessary to elucidate the link between diet and intestinal microbe composition to define a strategy to improve their health (19, 20). The symptoms and comorbidities of ASD could be improved with dietary interventions carried out after a deeper understanding of how foods relate to the intestinal microbiota (14). Therefore, the purpose of this study was to analyze gut bacteria composition in children with ASD and TD, and its relationship with nutritional status and dietary intake.
Materials and methods
Participants and ethics statement
From February to March 2020, we recruited a total of 65 children and adolescents aged 3 to 12 years at the nutritionist's office in Montevideo, Uruguay, through an open call (8); in this gut microbiota study, 30 with ASD and 28 neurotypicals were included (Figure 1). Diagnoses of ASD by a psychiatrist or a pediatric neurology specialist met the criteria of the Diagnostic and Statistical Manual of Mental Disorders (DSM-V) (21). Participants had not been taking medication, antibiotics, or probiotics for at least 1 month prior to enrollment in the study, and no children in the TD group were on a restricted diet. In addition, those diagnosed with attention deficit and hyperactivity disorder, diabetes mellitus, genetic diseases, inborn errors of metabolism, inflammatory bowel disease, celiac disease, and motor disability were excluded from both groups. This research was performed in accordance with the Helsinki Declaration 2000, approved by the Research Ethics Committee of the School of Nutrition, University of the Republic, and registered with the Ministry of Health of Uruguay (No. 282599). The study was explained to the participants' parents by telephone and discussed personally during their first visit to the research clinic, where informed written consent was obtained from every parent.
Sample collection, gut microbiota sequencing, and taxonomic classification
Children's parents were given a fecal microbiota kit (tube with transport media and specimen collection swab) and thorough instructions as to how they should collect the stool samples from their children at home. They collected a single fecal sample that was refrigerated until delivery to the clinic within 48 h. Once received, the samples were transferred to the laboratory with a cold pack and stored in an ultra-freezer at −80°C until analysis.
Extraction of bacterial DNA was performed at Enteria SRL laboratory, which followed the protocol recommended by Quick–DNA Fecal/Soil Microbe Miniprep Kit (Zymo Research—Catalog No. D6010). The quantity and quality of DNA were assessed by measuring absorbance at 260 and 280 nm using the Tecan Infinite M200 Pro (absorbance range 1.8–2 OD280/260). The extracted genomic DNA was sent to Genia laboratory to amplify hypervariable V1-V9 regions of the 16S rDNA gene from bacteria with Ion 16S™ Metagenomics Kit in PCR cycler using the Ion Torrent™ semiconductor sequencing workflow. Amplified fragments were sequenced using the Ion PGM™ Sequencing 400 Kit on the Ion PGM™ platform and analyzed using the Ion 16S™ metagenomics analysis module within Ion Reporter™ software.
Stool samples were studied to determine the relative abundance of gut bacteria. Operational taxonomic units (OTUs) were defined at 97% sequence homology, and the abundances of these bacterial genera or species were normalized. Taxonomic classification was performed using the SILVA 128 reference database up to the species level.
Anthropometric measures and dietary intake
All protocols including anthropometric measures and dietary intake were performed as previously described by Mendive Dubourdieu et al. (8). Participants' height and weight were measured by the same nutritionist researcher. Height was determined by using a portable height rod (208 Seca) with a 810–2,060 mm range and a 1 mm precision, and weight was measured using a portable electronic scale (Seca 813, Hamburg, Germany) with a 100 g accuracy, while subjects were barefoot and wearing light clothing according to techniques standardized by Frisancho (22) and the World Health Organization (WHO) (22, 23). Data were analyzed in Anthro (for children aged 3 to 5 years) and Anthro plus (for children and adolescents aged 5 to 12 years) software (WHO v.1.0.2. 2007), which apply WHO child growth curves (22). Body mass index-for-age (BMI/A), expressed in z-score (z), was the indicator studied to classify children into one of the following categories: normal weight (NW) and excess weight (EW, risk of overweight + overweight + obesity). Cutoff points used for children aged 2–5 years were >3SD, obesity; >2SD, overweight; >1SD, risk of overweight; between <1SD and >-1SD, normal weight; ≤-1SD, risk of wasting; ≤-2SD, emaciation; and ≤-3SD, severe emaciation and in those over 5 years old were ≥2SD, obesity; ≥1SD, overweight; between <1SD and >-2SD, normal weight; ≤-2SD, wasting; and ≤-3SD, severe emaciation. Undernutrition was dismissed because the sample size was small, and therefore, those participants were not considered for anthropometric analysis.
Children with ASD were divided into two groups depending on whether they followed a GCFD (ASD-diet, n = 16) or did not have a restricted diet (ASD-no diet, n = 14). Food intake over the past 3 months was estimated based on data obtained through the SAYCARE study food frequency questionnaire (FFQ) (24), which was adapted to this study to gather information on the consumption of gluten-free and casein-free foods. Children's parents were asked to indicate the consumption frequency and portion size of each food item according to a food photo booklet as a reference. The average daily consumption of each food (g/day or ml/day) was calculated and organized into different groups as follows: (1) “dairy”: milk, yogurt, chocolate milk, dairy desserts, and cheese; (2) “vegetable drinks”: birdseed, chestnut, almond, oat, rice, and coconut drinks; (3) “cereals with gluten”: pasta, bread, cookies, bakery products, breakfast cereals, pizza, and empanadas (dough stuffed with meat, fish, vegetables, etc., baked or fried), “cereals without gluten”: the same foods in the previous group without gluten and rice; (4) “food source of proteins”: meat, minced meat, chicken, pork, eggs, fresh and canned fish, and milanesa steak with and without gluten (a thin slice of beef dipped in beaten eggs and breaded; the fact that 25% of its weight is due to cereal has been taken into account); (5) “food source of fat”: butter, ghee (fat obtained by heating cow milk butter), and oil. Dairy, cereals with gluten, cereals without gluten, food source of fat (for children with typical development and ASD without diet), vegetable drinks, and food source of proteins (for children with ASD and a GCFD) were divided into two subgroups, considering the 50th percentile of the intake (intake ≤p50 and >p50).
Statistical analysis
For statistical analyses, IBM SPSS Statistics 22.0 (IBM Corp, Armonk, NY, USA) was used. We performed the Kolmogorov–Smirnov test to verify variable normal distribution. Data that were not normally distributed were log10 transformed. A p-value of lower than 0.05 was accepted as significant (two-tailed).
To compare genus and species relative abundance according to neurodevelopment (ASD vs. TD) and between autistic groups (ASD-diet vs. ASD-no diet), independent sample t-test was assessed. To study bacteria relative abundance according to nutritional status (NW and EW) in ASD and TD children, the two-way ANCOVA was applied (adjusted for age, birth weight, and GCFD/not restricted diet). Pair comparisons between the different groups were adjusted by the Bonferroni post-hoc test. Comparison between NW and EW in all children was carried out using one-way ANCOVA and correcting for the same potential confounders.
To evaluate associations between gut microbiota and dietary intake, univariate linear regression was used and adjusted for the following potential confounders: age, GCFD/not restricted diet, and TD/ASD (when all the children were analyzed); age (in the TD group), and age and GCFD/not restricted diet (in the ASD). In children with TD and ASD-no diet, differences in bacterial abundances among food intake p50 groups were examined using two-way ANOVA. Finally, the Student t-test and Mann–Whitney test were used to determine whether there were differences in gut bacteria relative abundances between those with higher or lower dairy intake in the ASD-diet group.
Results
Anthropometric characteristics, dietary intake, and age of children with autism spectrum disorder and typical development have been previously published (8). In Table 1, we compared the mean relative abundance of 20 selected genera and 16 species, according to ASD-diet vs. ASD-no diet and ASD vs. TD groups. No significant differences were found in the relative abundances of bacterial genera between neurotypical and ASD children. However, there is a significant difference in the mean relative abundance of Bifidobacterium (p = 0.008), Roseburia (p = 0.002), and Sutterella (p = 0.015) between the ASD-diet and ASD-no diet groups. At the species level, the ASD-diet group showed fewer Bifidobacterium adolescentis (p = 0.046) and Bifidobacterium longum (p = 0.002) but higher Roseburia hominis (p = 0.002) than the ASD-no diet group. Additionally, the ASD group had lower Bifidobacterium longum (p = 0.002) and higher Clostridium glycolicum (p = 0.028) than TD children.
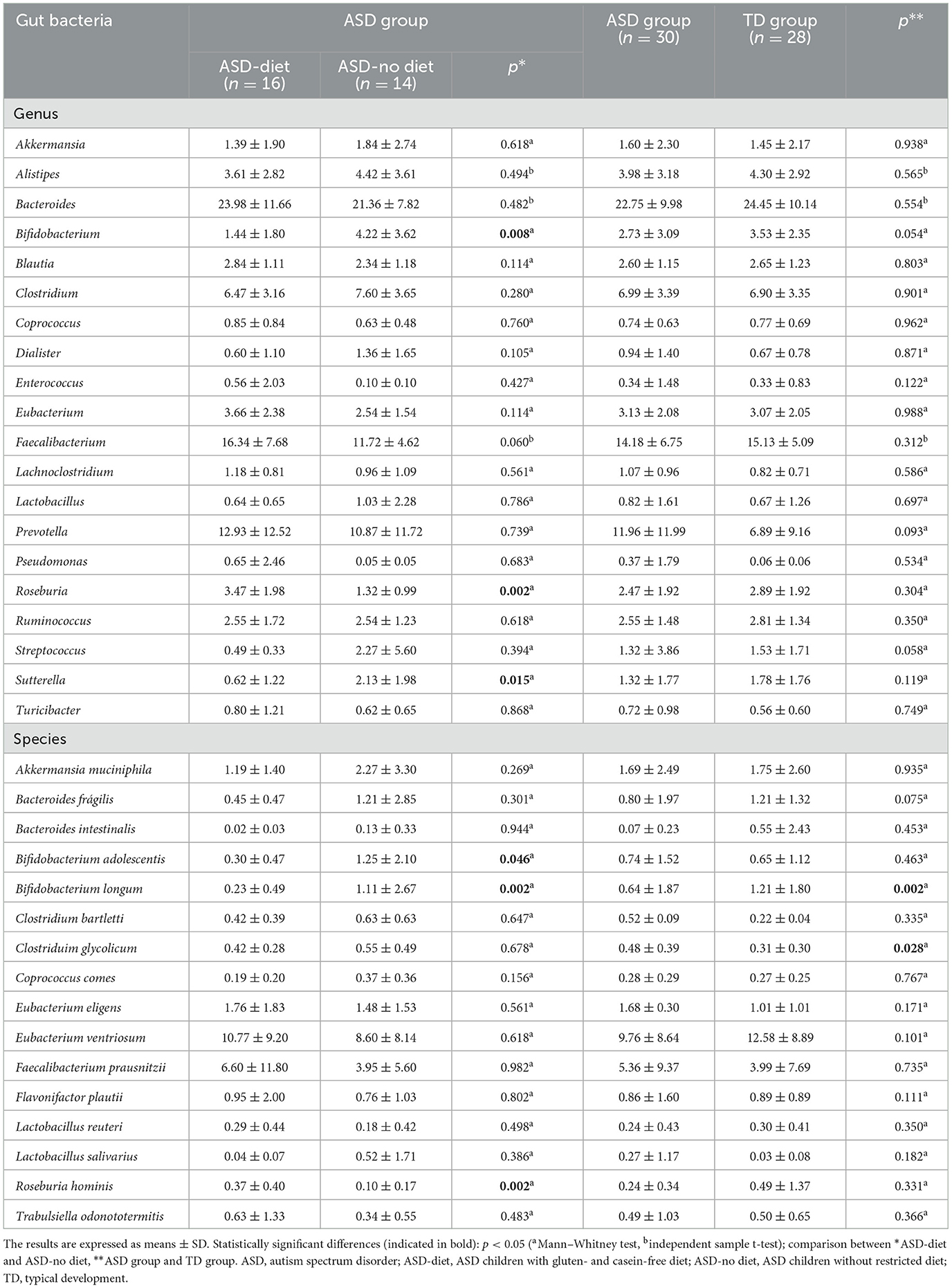
Table 1. Relative abundance of gut bacteria genus and species in children with autism spectrum disorder and typical development.
In Table 2, the comparison of genus and species relative abundance between nutritional status (normal weight vs. excess weight) and neurodevelopment (ASD vs. TD) groups was studied by two-way ANCOVA. Children in the All group (ASD + TD) with excess weight had a lower relative abundance of Roseburia than normal weight (p = 0.012). In addition, children with excess weight in the ASD group had a lower relative abundance of Roseburia (p = 0.005) and Faecalibacterium prausnitzii (p = 0.038) and a higher relative abundance of Eubacterium ventriosum (p = 0.019) and Flavonifractor plautii (p = 0.043) than those with excess weight in the TD group.
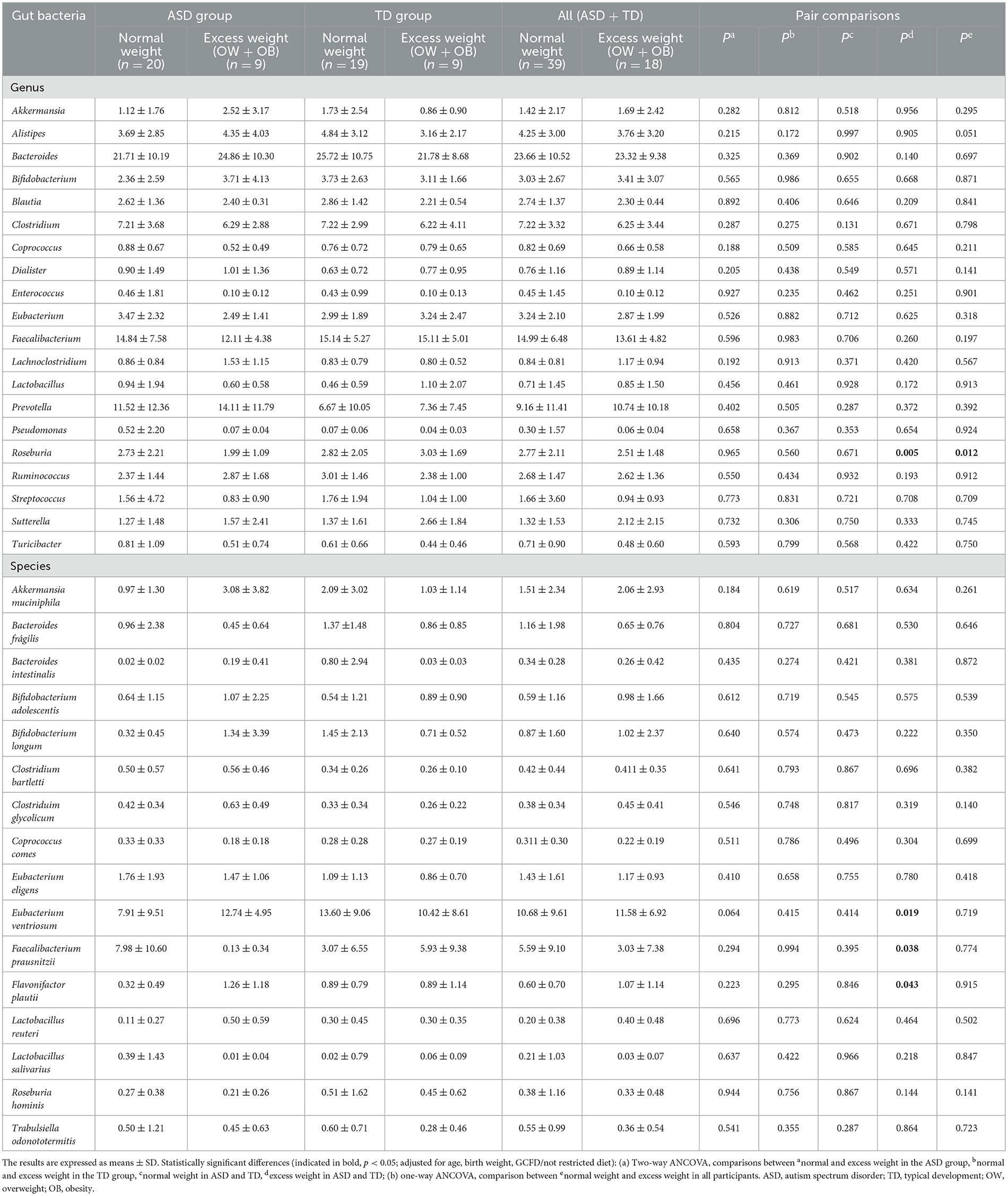
Table 2. Comparison of gut bacterial genus and species between nutritional status groups (normal weight vs. excess weight) and neurodevelopment groups (autism spectrum disorder vs. typical development) by two-way ANCOVA.
The relationship between the relative abundances of bacterial genus and species and children's dietary intake is shown in Figure 2. For the analysis, we applied unadjusted and adjusted (for age, GCFD/no diet and ASD/TD) models. Vegetable drink intake had an association with Enterococcus (n = 10; unadjusted model: r = 0.640, p = 0.046), Pseudomonas (n = 9; unadjusted: r = 0.671, p = 0.048), and Sutterella (unadjusted: n = 12, r = −0.692, p = 0.013), which disappeared after adjustment for covariates; and a relationship with Lactobacillus was independent of age, GCFD/no diet, and ASD/TD (n = 13; unadjusted: r = −0.683, p = 0.010; adjusted: r = −0.906, p = 0.009). Dairy intake (n = 43) had an association with Bacteroides (unadjusted: r = 0.353, p = 0.020; adjusted: r = 0.435, p = 0.048) and Bifidobacterium longum (unadjusted: r = 0.503, p = 0.001; adjusted: r = 0.552, p = 0.009) that remained when the covariates were applied; it also had a relationship with Bifidobacterium (unadjusted: n = 43, r = 0.320, p = 0.036) and Prevotella (unadjusted: n = 43, r = −0.380, p = 0.012) which did not hold after adjustment. Cereals with gluten did not show a significant correlation with bacteria genus or species. However, cereals without gluten (n = 58) had a relationship with Alistipes (unadjusted: r = −0.336, p = 0.015; adjusted: r = −0.373, p = 0.024), Bifidobacterium longum (unadjusted: r = −0.418, p = 0.003; adjusted: r = −0.537, p = 0.006), and Clostridium glycolicum (n = 58; unadjusted: r = 0.364, p = 0.009; adjusted: r = 0.374, p = 0.021), independent of confounding factors. They also had an association with Bifidobacterium (n = 56; unadjusted: r = −0.290, p = 0.041), which disappeared after adjustment for covariates. In addition, the association between cereals with and without gluten intake and Eubacterium eligens (n = 58; unadjusted: r = −0.290, p = 0.027) and coconut oil intake with Ruminococcus (n = 58; unadjusted: r = −0.532, p = 0.011) disappeared after adjustment. The relationship between coconut oil intake and Bacteroides intestinalis (n = 58 unadjusted: r = −0.891, p = 0.001; adjusted: r = −0.776, p = 0.021) and food source of fat (n = 58) with Clostridium glycolicum (unadjusted: r = 0.442, p = 0.001; adjusted: r = −0.430, p = 0.001), Eubacterium ventriosum (unadjusted: r = 0.356, p = 0.008; adjusted: r = 0.518, p = 0.004), and Flavonifractor plautii (unadjusted: r = 0.325, p = 0.026; adjusted: r = 0.337, p = 0.026) was independent of confounding factors. Fish intake was associated with Bacteroides intestinalis (n = 28; unadjusted: r = 0.582, p = 0.023) only in the model without adjustment. Food source of proteins had a relationship with Faecalibacterium (n = 58; unadjusted: r = −0.358, p = 0.006; adjusted: r = −0.349, p = 0.007), Lactobacillus (n = 49; unadjusted: r = −0.365, p = 0.010; adjusted: r = −0.398, p = 0.005), and Lactobacillus reuteri (n = 58; unadjusted: r = −0.363, p = 0.044; adjusted: r = −0.413, p = 0.027) in unadjusted and adjusted models, but the association with Streptococcus disappeared after considering potential confounders (n = 56; unadjusted: r = −0.287, p = 0.032).
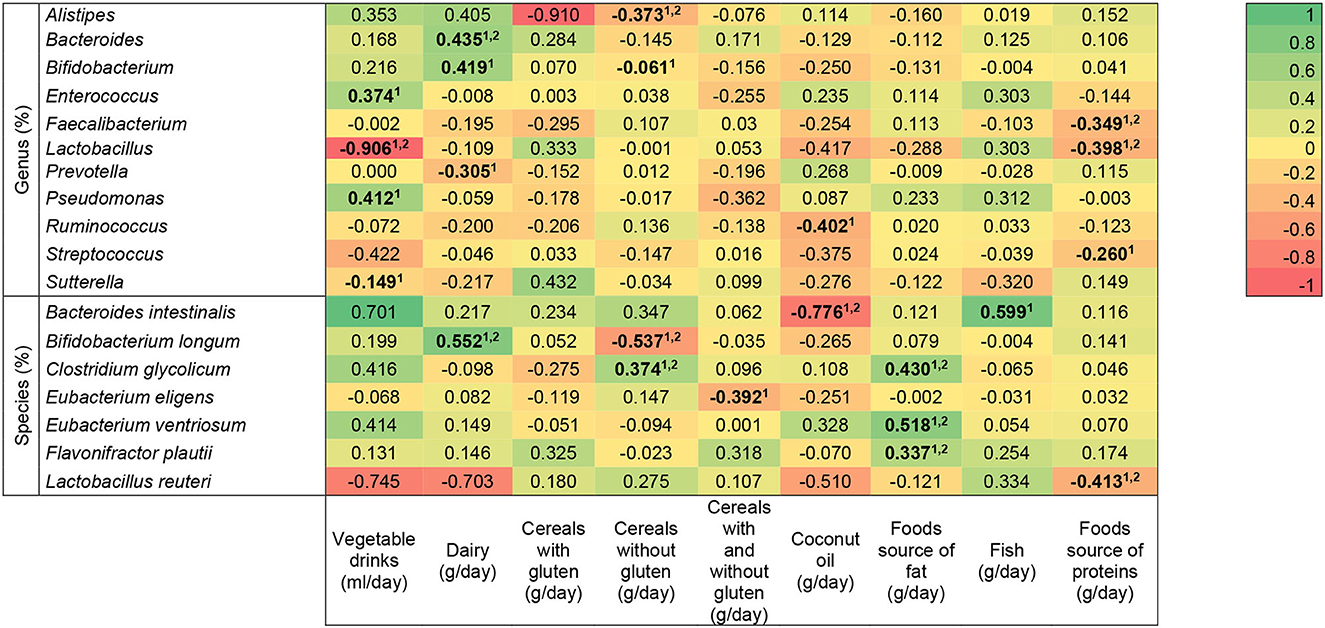
Figure 2. Heat map of the association between bacterial genera and species and dietary intake in children with autism spectrum disorder and neurotypical. Statistically significant differences (indicated in bold): p < 0.05 (linear regression model 1unadjusted, and 2adjusted for age, GCFD/not restricted diet, autism spectrum disorder/typical development). The R-values presented correspond to the adjusted models.
The association between bacteria genus and species and dietary intake in children with typical development is presented in Figure 3, where unadjusted and age-adjusted models were used. Dairy intake (n = 43) had an association with Alistipes (unadjusted: r = 0.427, p = 0.026; adjusted: r = 0.509, p = 0.022), Bacteroides (unadjusted: r = 0.498, p = 0.008; adjusted: r = 0.584, p = 0.007), Bifidobacterium ventrosum (unadjusted: r = 0.388, p = 0.046; adjusted: r = 0.545, p = 0.013), Bifidobacterium longum (unadjusted: r = 0.544, p = 0.004; adjusted: r = 0.621, p = 0.007), and Eubacterium ventrosum (unadjusted: r = 0.541, p = 0.004; adjusted: r = 0.426, p = 0.021), independent of the age, but the association with Prevotella (unadjusted: r =−0.547, p = 0.003) disappeared after adjustment, and the relationship with Sutterella (adjusted: r =−0.459, p = 0.045) only appeared when the covariate was considered. Cereals with gluten had a relationship with Lactobacillus (unadjusted: n = 49, r = 0.525, p = 0.007) that disappeared after adjusting, and with Lactobacillus reuteri, it was remained independent (unadjusted: n = 58, r = 0.579, p = 0.015, and adjusted: r = 0.532, p = 0.047). Cereals without gluten intake had an association with Alistipes, independent of age (unadjusted: n = 58, r =−0.439, p = 0.028; adjusted: r =−0.456, p = 0.028), but the association with Clostridium glycolicum disappeared when considering the confounder (unadjusted: n = 58, r = 0.402, p = 0.047). Food source of fat had an association with Lactobacillus (n = 49, unadjusted: r =−0.563, p = 0.003; adjusted: r =−0.508, p = 0.004), Clostridium glycolicum (n = 58; unadjusted: r = 0.546, p = 0.003; adjusted: r = 0.539, p = 0.004), Eubacterium ventriosum (n = 58; unadjusted: r = 0.407, p = 0.035; adjusted: r = 0.351, p = 0.050), and Flavonifractor plautii (n = 58; unadjusted: r = 0.487, p = 0.016; adjusted: r = 0.456, p = 0.021), which remained after adjusting. Fish had an association with Bacteroides intestinalis (unadjusted: n = 58, r = 0.555, p = 0.039), but it disappeared after considering the age. No significant associations were found between bacteria genera or species and cereals with and without gluten, coconut oil, and food source of proteins.
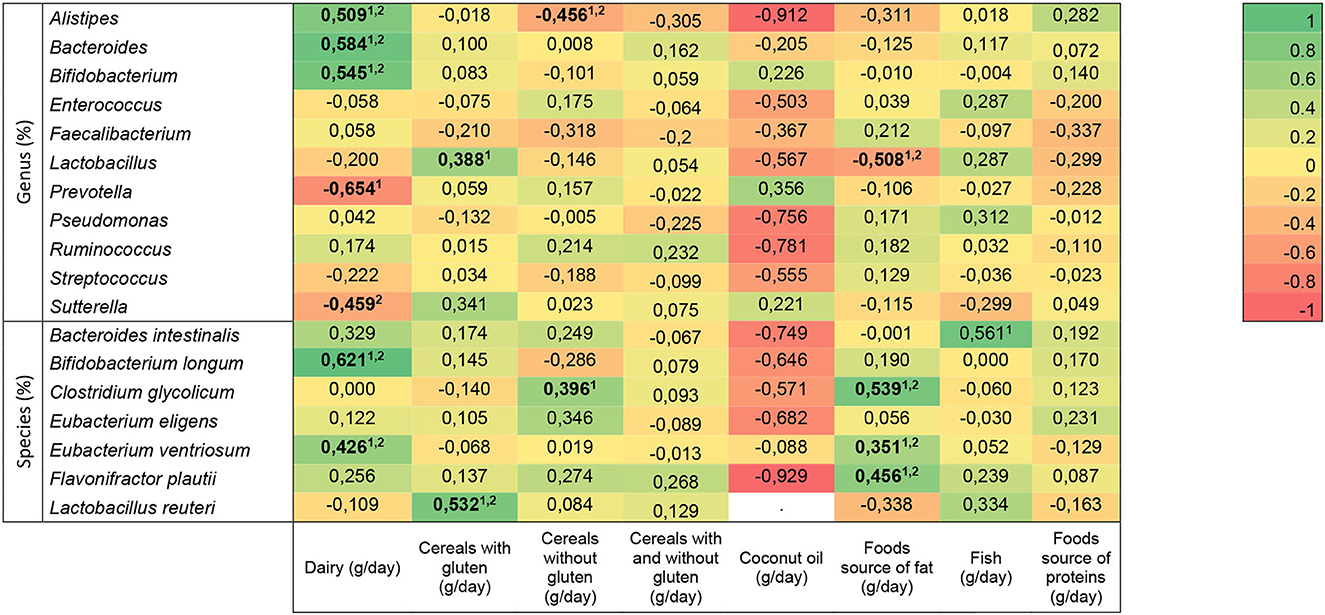
Figure 3. Heat map of the association between bacterial genera and species and dietary intake in neurotypical children. Statistically significant differences (indicated in bold): p < 0.05 (linear regression model 1unadjusted, and 2djusted for age). The R-values presented correspond to the adjusted models.
The association between dietary intake and relative abundance of bacteria genus and species in children with ASD is shown in Figure 4. The analysis was unadjusted and adjusted for age and gluten- and casein-free diet/no diet. Vegetable drinks were associated with Lactobacillus in both models (n = 49, unadjusted: r =−0.620, p = 0.042; adjusted: r =−0.919, p = 0.034), but they were associated with Sutterella only without considering confounding factors (n = 49; unadjusted: r =−0.645, p = 0.032). Intake of dairy, cereals with gluten, coconut oil, and food source of fat did not show a significant correlation with gut bacteria. Cereals without gluten had a correlation with Faecalibacterium which was kept after adjustment (n = 58; unadjusted: r = 0.482, p = 0.011; adjusted: r = 0.470, p = 0.040). Cereals with and without gluten had an association with Ruminococcus (n = 58; unadjusted: r =−0.388, p = 0.034), but it disappeared when potential confounders were considered. The association between food source of proteins and Faecalibacterium was observed only when considering the covariates (n = 58; adjusted: r =−0.356, p = 0.049); with Streptococcus, the relationship was found in unadjusted and adjusted models (n = 58; unadjusted: r =−0.437, p = 0.020; adjusted: r =−0.435, p = 0.023).
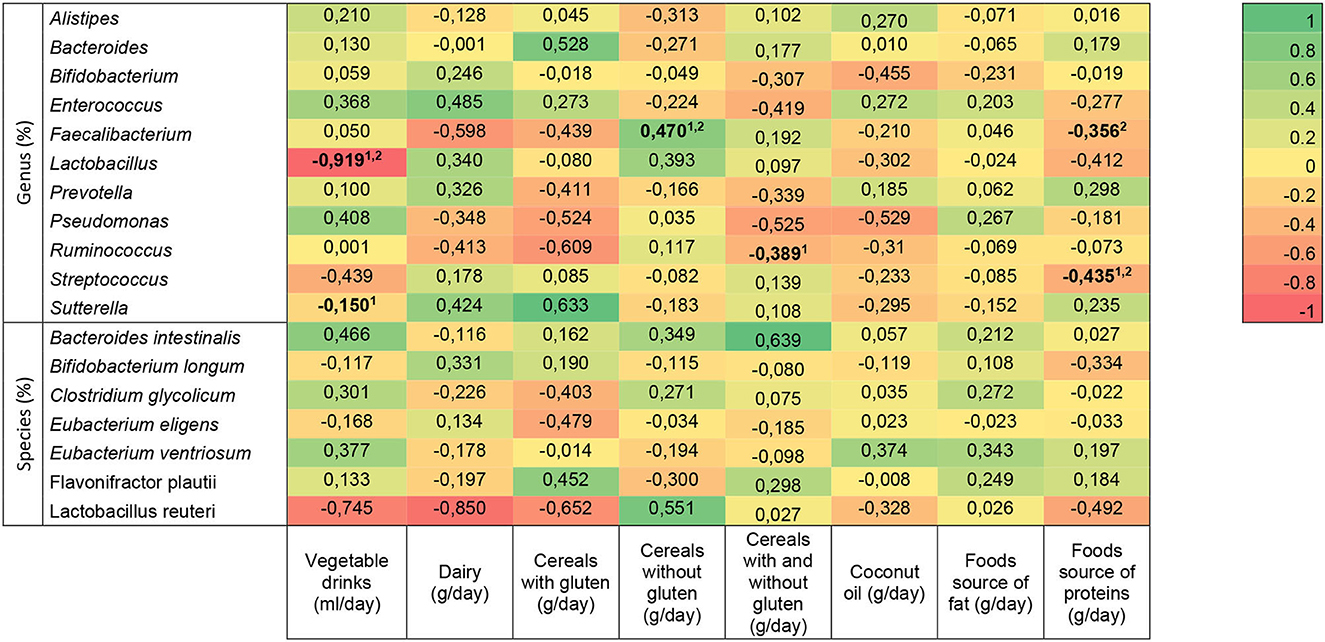
Figure 4. Heat map of the association between bacterial genera and species and dietary intake in autism spectrum disorder children. Statistically significant differences (indicated in bold): p < 0.05 (linear regression model 1unadjusted, and 2adjusted for age, gluten- and casein-free diet/no diet). The R-values presented correspond to the adjusted models.
Table 3 shows the comparison of gut bacterial genus and species between dietary intake groups, categorized by the 50th percentile, in children with TD and ASD without a GCFD by means of two-way ANOVA. Children with ASD-no diet and a dairy intake of >334.3 g/day had a higher relative abundance of Lactobacillus (p = 0.025) and Streptococcus (p = 0.003) than those with an intake of ≤334.3 g/day. In the TD group with a dairy intake of >334.3 g/day, a greater amount of Alistipes (p = 0.013) and Eubacterium ventriosum (p = 0.024) was found as compared with children with a lower dairy intake. When gut bacteria were compared between TD and ASD-no diet children in the dairy intake of >334.3 g/day group, we observed a lower relative abundance of Faecalibacterium (p = 0.005) and a higher one of Lactobacillus (p = 0.012) and Streptococcus (p = 0.009) in ASD-no diet children. In relation to cereals with gluten, Faecalibacterium was higher (p = 0.042) in ASD-no diet children with an intake of >198.35 g/day than TD with the same intake. ASD-no diet children with an intake of cereals without gluten ≤7.12 g/day had higher Flavonifractor plautii (p = 0.013) than those with an intake of >7.12 g/day. In addition, in the group with an intake of cereal without gluten >7.12 g/day, Flavonifractor plautii (p = 0.014) and Eubacterium ventrosum (p = 0.017) were higher in TD children than in ASD-no diet. Regarding food source of fat, when the intake was >25 g/day, the abundance of Faecalibacterium was higher in TD children than in ASD-no diet (p = 0.031).
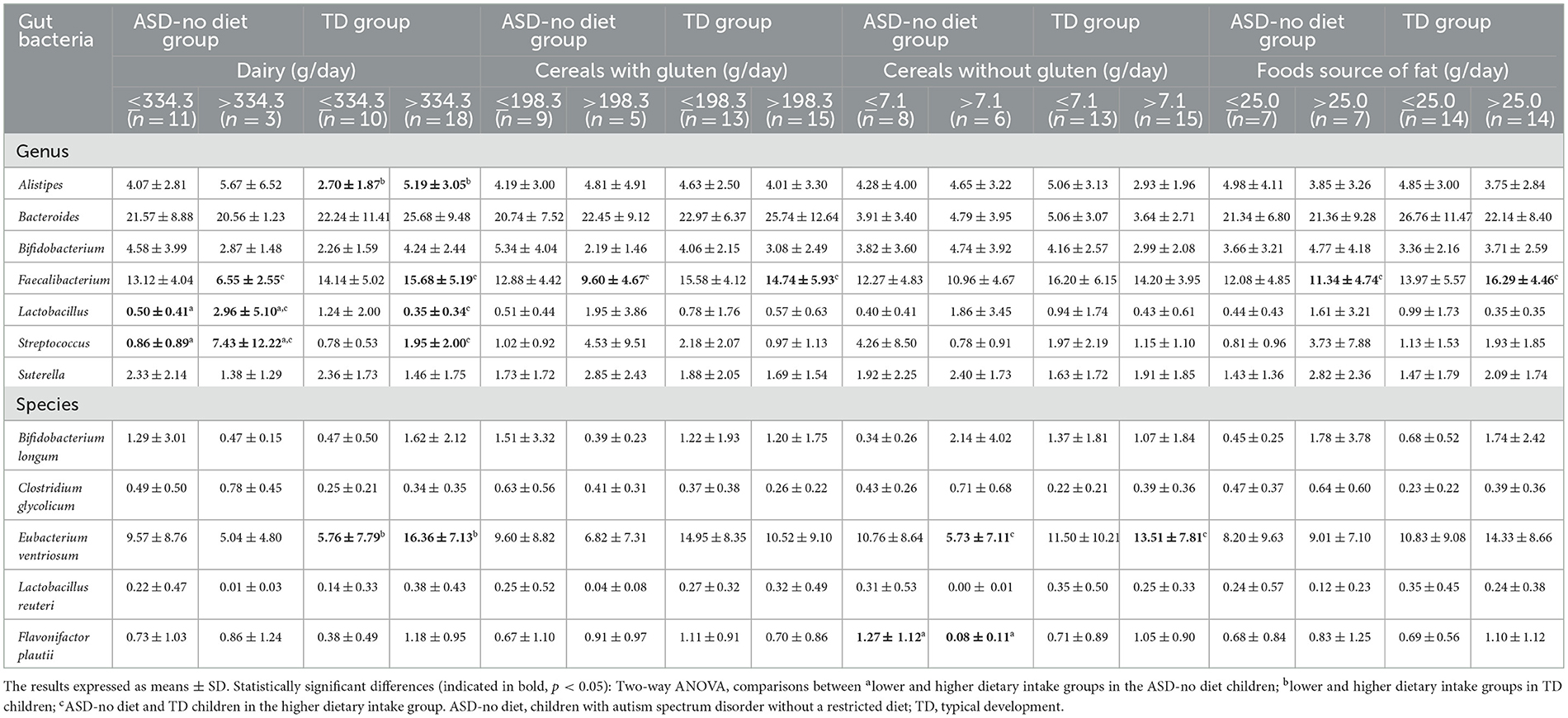
Table 3. Comparison of gut bacterial genus and species between dietary intake groups (categorized by the 50th percentile) in children with typical development and autism spectrum disorder without diet by means of two-way ANOVA.
Table 4 shows the comparison of gut bacterial genus and species between dietary intake groups categorized by the 50th percentile in children with ASD and a GCFD. Alistipes was higher (p = 0.002), and Lactobacillus was lower (p = 0.004) in the >309.5 ml/day vegetable drink intake group than in children with an intake of ≤309.5 ml/day. Higher levels of Faecalibacterium (p = 0.045) were found in individuals who consume >102.46 g/day of cereals without gluten compared with those with lower intake. No significant difference was found when comparing gut bacteria between the higher and the lower intake of food source of proteins.
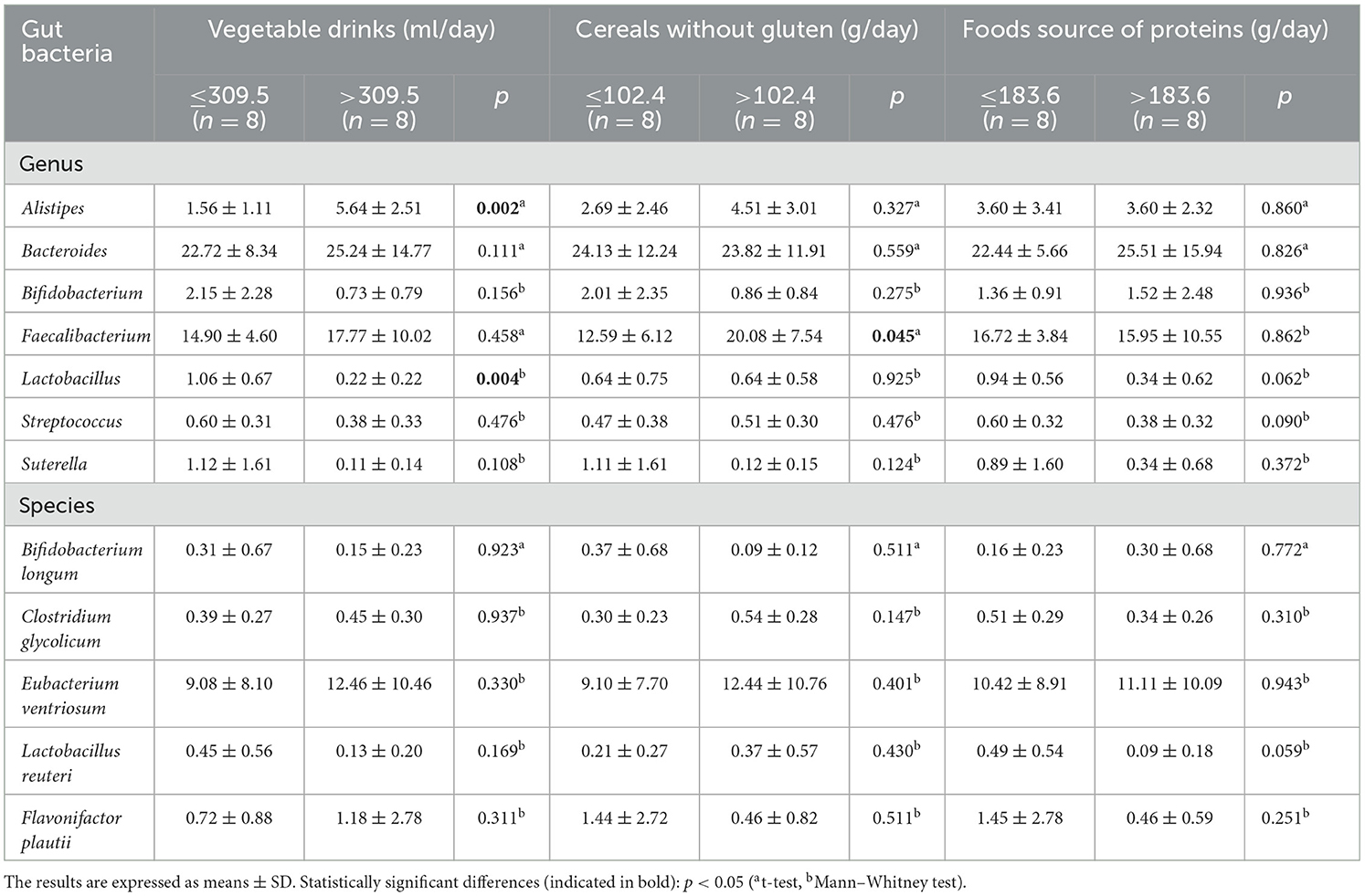
Table 4. Comparison of gut bacterial genus and species between dietary intake groups (categorized by the 50th percentile) in children with autism spectrum disorder and a gluten- and casein-free diet.
Discussion
In this study, differences were observed in the composition of fecal bacteria as per nutritional status and dietary intake in ASD and TD children. Several studies have shown different gut bacteria compositions in children with ASD as compared with TD (25). In contrast, we have not found significant differences at the genus level, but at the species level, we observed lower abundances of Bifidobacterium longum and higher abundances of Clostridium glycolicum in ASD than in the TD group. Wang et al. (26) reported lower levels of Bifidobacterium spp. and Akkermansia muciniphila in children with autism compared with TD ones, but in our study, no significant difference was observed in the abundance of this mucolytic bacterium. Our findings are in line with previous studies that found decreased Bifidobacterium spp. and elevated Clostridium spp. in ASD as compared with controls and suggested that the latter bacterium is a determinant of the risk of autism (12, 27, 28). Research conducted in 1998 hypothesized that ASD could be due to a dysbiosis context with colonization by Clostridium tetani and to its neurotoxic effects in neurons producing gamma-aminobutyric acid (inhibitory neurotransmitter of the central nervous system) (29, 30). Another study shows a positive correlation between Clostridium cluster XVIII and gastrointestinal symptoms such as constipation in autistic and neurotypical subjects (27, 31).
Interestingly, in the ASD-diet group, Bifidobacterium (B.) were significantly lower compared with the ASD-no diet group, and this bacteria had a positive association with dairy intake and a negative association with cereals without gluten in the All (ASD + TD) group. A greater abundance of Bifidobacterium has been described as having beneficial effects on health since it inhibits pathogen growth by releasing bacteriocins (32, 33), and an increase in B. longum can mitigate depression in patients with irritable bowel syndrome through changes in the brain areas involved in mood regulation (34).
In line with our results, a study in adults shows a significant association between gluten-free diet and a reduced relative abundance of Bifidobacterium and Lactobacillus (L.) (35). Importantly, both genera synthesize short-chain fatty acids, that interact with receptors in the gut mucosa and contribute to mucus maintenance, have an antimicrobial effect on pathogens and can reverse leaky gut disorders (36). Therefore, the assumption is that greater abundances of Lactobacillus in the gut are associated with a higher intake of dairy (37). In the present study, we also observed that children with ASD-no diet and a dairy intake of >334.3 g/day had a higher mean of Lactobacillus than those with a lower intake. On the contrary, the ASD-diet group with an intake of >309.5 ml/day of vegetable drinks had a lower abundance of this genus than children with an intake of ≤309.5 ml/day. Considering all participants, the intake of vegetable drinks and food source of proteins had a negative association with Lactobacillus and L. reuteri, respectively. This last bacterial species was found to reverse social deficits in experimental animals with ASD (38).
Apart from that, vegetable drink intake also had a positive association with the facultative anaerobe Enterococcus and a negative association with Sutterella in all children only in the unadjusted model. In relation to this, Mangiola et al. (39) have reported a positive association between Sutterella genus and the development of autism in children. Nevertheless, an increase in Enterococcus has been detected in fecal samples from patients with diarrhea (40). In addition, a pro-inflammatory bacteria named Alistipes (41) was higher in ASD-diet children with an intake of >309.5 ml/day of vegetable drinks than those with a lower intake, but it was also higher in children with TD and a dairy intake of >334.3 g/day than in those with a dairy intake of ≤334.3 g/day, and a positive association between Alistipes and dairy intake was observed in this group. Additionally, in All and TD groups, this bacterium was inversely related to cereals without gluten. Another bacterium with anti-inflammatory properties called Prevotella (42) had a negative association (only in the unadjusted model) with dairy intake considering All and TD children.
In relation to nutritional status, participants in the All group with excess weight had a lower relative abundance of a beneficial butyrate-producing bacterium called Roseburia (R.) (43) than the normal weight ones, and Roseburia and R. hominis were higher in the ASD-diet as compared with the ASD-no diet group. In addition, children with excess weight in the ASD group had a significantly lower relative abundance of Roseburia and Faecalibacterium prausnitzii and a higher relative abundance of Eubacterium ventriosum and Flavonifractor plautii than those with excess weight in the TD group. A study performed in Japan showed that E. ventriosum was significantly associated with obese subjects (44). Moreover, a significantly reduced abundance of this species was described in people with colorectal cancer and could be considered a risk biomarker for the illness (45). On the other hand, Faecalibacterium prausnitzii is responsible for degrading mucin-producing butyrate and peptides that inhibit the NF-kB pathway in intestinal epithelial cells with an anti-inflammatory effect, and it has been associated with a reduced abundance in obesity (46, 47).
It has recently been reported that eating bread made from transgenic low-gliadin wheat produces a significantly higher abundance of Faecalibacterium and Roseburia genera with potentially beneficial changes in the composition of the intestinal microbiota, due to the increase in butyrate, which maintains good gut permeability (48). In our study, considering the ASD group, cereals without gluten also had a positive correlation with Faecalibacterium. Additionally, we observed that the ASD-diet group had a significantly higher level of Faecalibacterium in individuals who consume >102.46 g/day of cereals without gluten as compared with those with lower intake. It is worth mentioning that Jiang et al. (49) observed a negative correlation between Faecalibacterium abundances and the severity of depressive manifestation and overexpression of Alistipes in this psychiatric disorder.
In relation to cereal intake, other studies have shown that a high carbohydrate intake was associated with higher abundances of Bifidobacterium and Lactobacillus in fecal samples (50). In our study, the TD group precisely had a positive relationship between the intake of cereals with gluten and the abundances of Lactobacillus and L. reuteri. Apart from that, by comparison, children with TD and an intake of >7.12 g/day of cereals without gluten had higher abundances of Flavonifractor (F.) plautii than the ASD-no diet group with the same food intake, and in this last group, those with an intake of ≤7.12 g/day had more F. plautii than children with an intake of >7.12 g/day. Mikami et al. (51) have recently reported that an increased abundance of this bacterium has a beneficial effect as a modulator of gut inflammation, mediating IL-17 suppression in animals.
A number of studies have reported that an animal-based diet with high protein and fat intake seems to increase bile-tolerant bacteria called Bacteroides and could boost intestinal bowel disease risk (15, 52). Consistent with the said finding, we found that fish intake was positively associated with Bacteroides intestinalis in All and TD children (without adjustment for potential confounders). Even so, an investigation with mice fed with fish oil for 11 weeks described that, due to the interaction with the gut microbiota, there was less white adipose tissue inflammation and Toll-like receptor activation as compared with the lard diet (53). However, another research shows that in humans, salmon consumption has no effect on gut microbiota of pregnant women (54).
Regarding the intake of food source of fat, a comparison study showed that rats on a diet rich in coconut oil for 2 weeks had a lower abundance of Ruminococcus flavecaciens than those fed with soy oil (55). Along those lines, our study has found a negative association between coconut oil intake and Ruminococcus (unadjusted model) and Bacteroides intestinalis in the All group. In addition, a meta-analysis shows 11 trials with a higher abundance of Ruminococcus (involved in the fermentation of dietary fibers) in ASD children than in TD ones (25), but we have not found a significant difference in those groups.
In short, advances in the study of intestinal microbiota have led to new research, but the results have been disparate due to the complexity of the subject. A number of investigations show that gut microbiota may modulate brain function via metabolic and signaling pathways in charge of social cognition and emotional regulation (56). Our results show how gut microbiota composition is related to food consumption, nutritional status, and neurodevelopment. Based on these results and the possibility of further investigating the interaction between diet, gut microbiota, and autism through intervention studies, it would be possible to establish a clear relationship between specific bacteria profiles, food intake, and neurodevelopment. This could help establish preventive and treatment strategies for autism. Further studies focusing on an associated analysis of these topics in a large sample of children are needed to improve recommendations for this population.
Study limitations
Research limitation is related to the small size of the sample, and the fact that the amount of dietary intake was estimated by the food frequency questionnaire instead of being accurately measured. However, these questionnaires are the most economical and validated method used worldwide, and we consider this study as an important input to the knowledge on this topic and for other types of research, since there are few studies that address the relationship of the intestinal microbiota with dietary intake and nutritional status in children with ASD.
Conclusion
In this study, we observed differences in the composition of gut bacteria in children with autism spectrum disorder and typical development in only two species (Bifidobacterium longum and Clostridium glycolicum), but when we analyzed these two populations taking into account dietary intake and nutritional status, we were able to observe more differences. We found positive and negative associations between the intake of dairy, vegetable drinks, cereals with gluten and without gluten, food source of proteins, fish, food source of fat, and coconut oil, with the gut microbiota, independent of potential confounder variables such as age, being on a gluten- and casein-free diet, and neurodevelopment. Moreover, analyzing and comparing the higher and lower intake of these food groups allowed us to observe in greater depth how intake quantities are associated with higher or lower abundances of gut bacteria. Pending further studies, these results might be considered as a starting point for the nutritional treatment of ASD children.
Data availability statement
The data presented in the study are deposited in the NCBI with links to BioProject accession number PRJNA988151 in the NCBI BioProject database (https://www.ncbi.nlm.nih.gov/bioproject/PRJNA988151).
Ethics statement
The studies involving human participants were reviewed and approved by the Research Ethics Committee of the Universidad de la República's School of Nurition (CEIN). Written informed consent to participate in this study was provided by the participants' legal guardian/next of kin.
Author contributions
PM: designed and conducted research, analyzed data, performed statistical analysis, wrote the manuscript, and had primary responsibility for final content. MG: designed research, analyzed data, performed statistical analysis, had primary responsibility for final content, and supervision and review. All authors contributed to the article and approved the submitted version.
Funding
The study was supported by R&D Investment Program (ICEX Spain).
Acknowledgments
The authors would like to thank families for their cooperation, Loreley Castelli for assisting us in uploading the sequences to the data repository, as well as the Agencia Nacional de Investigación e Innovación (ANII), from Uruguay, for the PhD grant to PM.
Conflict of interest
The authors declare that the research was conducted in the absence of any commercial or financial relationships that could be construed as a potential conflict of interest.
Publisher's note
All claims expressed in this article are solely those of the authors and do not necessarily represent those of their affiliated organizations, or those of the publisher, the editors and the reviewers. Any product that may be evaluated in this article, or claim that may be made by its manufacturer, is not guaranteed or endorsed by the publisher.
References
1. Ding X, Xu Y, Zhang X, Zhang L, Duan G, Song C, et al. Gut microbiota changes in patients with autism spectrum disorders. J Psychiatr Res. (2020) 129:149–59. doi: 10.1016/j.jpsychires.2020.06.032
2. Kong X, Liu J, Cetinbas M, Sadreyev R, Koh M, Huang H, et al. New and preliminary evidence on altered oral and gut microbiota in individuals with autism spectrum disorder (ASD): implications for ASD diagnosis and subtyping based on microbial biomarkers. Nutrients. (2019) 11:2128. doi: 10.3390/nu11092128
3. Adak A, Khan MR. An insight into gut microbiota and its functionalities. Cell Mol Life Sci. (2019) 76:473–93. doi: 10.1007/s00018-018-2943-4
4. Rosés C, Cuevas-Sierra A, Quintana S, Riezu-Boj JI, Martínez JA, Milagro FI, et al. Gut microbiota bacterial species associated with mediterranean diet-related food groups in a Northern Spanish population. Nutrients. (2021) 13:636. doi: 10.3390/nu13020636
5. McDonnell L, Gilkes A, Ashworth M, Rowland V, Harries TH, Armstrong D, et al. Association between antibiotics and gut microbiome dysbiosis in children: systematic review and meta-analysis. Gut Microbes. (2021) 13:1–18. doi: 10.1080/19490976.2020.1870402
6. Nogay NH, Nahikian-Nelms M. Can we reduce autism-related gastrointestinal and behavior problems by gut microbiota based dietary modulation? a review. Nutr Neurosci. (2021) 24:327–38. doi: 10.1080/1028415X.2019.1630894
7. Cryan JF, O'Riordan KJ, Sandhu K, Peterson V, Dinan TG. The gut microbiome in neurological disorders. Lancet Neurol. (2020) 19:179–94. doi: 10.1016/S1474-4422(19)30356-4
8. Mendive Dubourdieu P, Guerendiain M. Dietary intake, nutritional status and sensory profile in children with autism spectrum disorder and typical development. Nutrients. (2022) 14:2155. doi: 10.3390/nu14102155
9. Quan L, Xu X, Cui Y, Han H, Hendren RL, Zhao L, et al. A systematic review and meta-analysis of the benefits of a gluten-free diet and/or casein-free diet for children with autism spectrum disorder. Nutr Rev. (2022) 80:1237–46. doi: 10.1093/nutrit/nuab073
10. Keller A, Rimestad ML, Friis Rohde J, Holm Petersen B, Bruun Korfitsen C, Tarp S, et al. The effect of a combined gluten- and casein-free diet on children and adolescents with autism spectrum disorders: a systematic review and meta-analysis. Nutrients. (2021) 13:470. doi: 10.3390/nu13020470
11. Peretti S, Mariano M, Mazzocchetti C, Mazza M, Pino MC, Verrotti Di Pianella A, et al. Diet: the keystone of autism spectrum disorder? Nutr Neurosci. (2019) 22:825–39. doi: 10.1080/1028415X.2018.1464819
12. Rinninella E, Raoul P, Cintoni M, Franceschi F, Miggiano GAD, Gasbarrini A, et al. What is the healthy gut microbiota composition? a changing ecosystem across age, environment, diet, and diseases. Microorganisms. (2019) 7:14. doi: 10.3390/microorganisms7010014
13. Merra G, Noce A, Marrone G, Cintoni M, Tarsitano MG, Capacci A, et al. Influence of mediterranean diet on human gut microbiota. Nutrients. (2020) 13:7. doi: 10.3390/nu13010007
14. Horn J, Mayer DE, Chen S, Mayer EA. Role of diet and its effects on the gut microbiome in the pathophysiology of mental disorders. Transl Psychiatry. (2022) 12:164. doi: 10.1038/s41398-022-01922-0
15. De Filippo C, Di Paola M, Ramazzotti M, Albanese D, Pieraccini G, Banci E, et al. Diet, environments, and gut microbiota. a preliminary investigation in children living in rural and urban Burkina Faso and Italy. Front Microbiol. (2017) 8:1979. doi: 10.3389/fmicb.2017.01979
16. Rampelli S, Guenther K, Turroni S, Wolters M, Veidebaum T, Kourides Y, et al. Pre-obese children's dysbiotic gut microbiome and unhealthy diets may predict the development of obesity. Commun Biol. (2018) 1:222. doi: 10.1038/s42003-018-0221-5
17. Yang YJ, Ni YH. Gut microbiota and pediatric obesity/non-alcoholic fatty liver disease. J Formos Med Assoc. (2019) 118(Suppl 1):S55–61. doi: 10.1016/j.jfma.2018.11.006
18. Bervoets L, Van Hoorenbeeck K, Kortleven I, Van Noten C, Hens N, Vael C, et al. Differences in gut microbiota composition between obese and lean children: a cross-sectional study. Gut Pathog. (2013) 5:10. doi: 10.1186/1757-4749-5-10
19. Lenfestey MW, Neu J. Probiotics in newborns and children. Pediatr Clin North Am. (2017) 64:1271–89. doi: 10.1016/j.pcl.2017.08.006
20. De Filippo C, Di Paola M, Giani T, Tirelli F, Cimaz R. Gut microbiota in children and altered profiles in juvenile idiopathic arthritis. J Autoimmun. (2019) 98:1–12. doi: 10.1016/j.jaut.2019.01.001
21. Wiggins LD, Rice CE, Barger B, Soke GN, Lee L-C, Moody E, et al. DSM-5 criteria for autism spectrum disorder maximizes diagnostic sensitivity and specificity in preschool children. Soc Psychiatry Psychiatr Epidemiol. (2019) 54:693–701. doi: 10.1007/s00127-019-01674-1
22. WHO Multicentre Growth Reference Study Group. WHO child growth standards based on length/height, weight and age. Acta Paediatr Suppl. (2006) 450:76–85. doi: 10.1111/j.1651-2227.2006.tb02378.x
23. Ulijaszek SJ, Kerr DA. Anthropometric measurement error and the assessment of nutritional status. Br J Nutr. (1999) 82:165–77. doi: 10.1017/S0007114599001348
24. Rendo-Urteaga T, Saravia L, Sadalla Collese T, Monsalve-Alvarez JM, González-Zapata LI, Tello F, et al. Reliability and validity of an FFQ for South American children and adolescents from the SAYCARE study. Public Health Nutr. (2020) 23:13–21. doi: 10.1017/S1368980019002064
25. Iglesias-Vázquez L, Van Ginkel Riba G, Arija V, Canals J. Composition of Gut Microbiota in children with autism spectrum disorder: a systematic review and meta-analysis. Nutrients. (2020) 12:792. doi: 10.3390/nu12030792
26. Wang L, Christophersen CT, Sorich MJ, Gerber JP, Angley MT, Conlon MA. Low relative abundances of the mucolytic bacterium Akkermansia muciniphila and Bifidobacterium spp. in feces of children with autism. Appl Environ Microbiol. (2011) 77:6718–21. doi: 10.1128/AEM.05212-11
27. Srikantha P, Mohajeri MH. The possible role of the microbiota-gut-brain-axis in autism spectrum disorder. Int J Mol Sci. (2019) 20:2115. doi: 10.3390/ijms20092115
28. Weston B, Fogal B, Cook D, Dhurjati P. An agent-based modeling framework for evaluating hypotheses on risks for developing autism: effects of the gut microbial environment. Med Hypotheses. (2015) 84:395–401. doi: 10.1016/j.mehy.2015.01.027
29. Gomaa EZ. Human gut microbiota/microbiome in health and diseases: a review. Antonie Van Leeuwenhoek. (2020) 113:2019–40. doi: 10.1007/s10482-020-01474-7
30. Bolte ER. Autism and clostridium tetani. Med Hypotheses. (1998) 51:133–44. doi: 10.1016/S0306-9877(98)90107-4
31. Strati F, Cavalieri D, Albanese D, De Felice C, Donati C, Hayek J, et al. New evidences on the altered gut microbiota in autism spectrum disorders. Microbiome. (2017) 5:24. doi: 10.1186/s40168-017-0242-1
32. Ahmed SA, Elhefnawy AM, Azouz HG, Roshdy YS, Ashry MH, Ibrahim AE, et al. Study of the gut microbiome profile in children with autism spectrum disorder: a single tertiary hospital experience. J Mol Neurosci. (2020) 70:887–96. doi: 10.1007/s12031-020-01500-3
33. Rodiño-Janeiro BK, Vicario M, Alonso-Cotoner C, Pascua-García R, Santos J. A review of microbiota and irritable bowel syndrome: future in therapies. Adv Ther. (2018) 35:289–310. doi: 10.1007/s12325-018-0673-5
34. Pinto-Sanchez MI, Hall GB, Ghajar K, Nardelli A, Bolino C, Lau JT, et al. Probiotic bifidobacterium longum ncc3001 reduces depression scores and alters brain activity: a pilot study in patients with irritable bowel syndrome. Gastroenterology. (2017) 153:448–59.e8. doi: 10.1053/j.gastro.2017.05.003
35. De Palma G, Nadal I, Collado MC, Sanz Y. Effects of a gluten-free diet on gut microbiota and immune function in healthy adult human subjects. Br J Nutr. (2009) 102:1154–60. doi: 10.1017/S0007114509371767
36. Al-Ayadhi L, Zayed N, Bhat RS, Moubayed NMS, Al-Muammar MN, El-Ansary A. The use of biomarkers associated with leaky gut as a diagnostic tool for early intervention in autism spectrum disorder: a systematic review. Gut Pathogens. (2021) 13:54. doi: 10.1186/s13099-021-00448-y
37. Tomova A, Soltys K, Kemenyova P, Karhanek M, Babinska K. The influence of food intake specificity in children with autism on gut microbiota. Int J Mol Sci. (2020) 21:2797. doi: 10.3390/ijms21082797
38. Sgritta M, Dooling SW, Buffington SA, Momin EN, Francis MB, Britton RA, et al. Mechanisms underlying microbial-mediated changes in social behavior in mouse models of autism spectrum disorder. Neuron. (2019) 101:246–59.e6. doi: 10.1016/j.neuron.2018.11.018
39. Mangiola F, Ianiro G, Franceschi F, Fagiuoli S, Gasbarrini G, Gasbarrini A. Gut microbiota in autism and mood disorders. World J Gastroenterol. (2016) 22:361–8. doi: 10.3748/wjg.v22.i1.361
40. Briggs AD, Kehlbacher A, Tiffin R, Garnett T, Rayner M, Scarborough P. Assessing the impact on chronic disease of incorporating the societal cost of greenhouse gases into the price of food: an econometric and comparative risk assessment modelling study. BMJ Open. (2013) 3:e003543. doi: 10.1136/bmjopen-2013-003543
41. Parker BJ, Wearsch PA, Veloo ACM, Rodriguez-Palacios A. The genus alistipes: gut bacteria with emerging implications to inflammation, cancer, and mental health. Front Immunol. (2020) 11:906. doi: 10.3389/fimmu.2020.00906
42. Sakkas H, Bozidis P, Touzios C, Kolios D, Athanasiou G, Athanasopoulou E, et al. Nutritional status and the influence of the vegan diet on the gut microbiota and human health. Medicina. (2020) 56:88. doi: 10.3390/medicina56020088
43. Machiels K, Joossens M, Sabino J, De Preter V, Arijs I, Eeckhaut V, et al. A decrease of the butyrate-producing species Roseburia hominis and Faecalibacterium prausnitzii defines dysbiosis in patients with ulcerative colitis. Gut. (2014) 63:1275–83. doi: 10.1136/gutjnl-2013-304833
44. Kasai C, Sugimoto K, Moritani I, Tanaka J, Oya Y, Inoue H, et al. Comparison of the gut microbiota composition between obese and non-obese individuals in a Japanese population, as analyzed by terminal restriction fragment length polymorphism and next-generation sequencing. BMC Gastroenterol. (2015) 15:100. doi: 10.1186/s12876-015-0330-2
45. Mukherjee A, Lordan C, Ross RP, Cotter PD. Gut microbes from the phylogenetically diverse genus Eubacterium and their various contributions to gut health. Gut Microbes. (2020) 12:1802866. doi: 10.1080/19490976.2020.1802866
46. Walters WA, Xu Z, Knight R. Meta-analyses of human gut microbes associated with obesity and IBD. FEBS Lett. (2014) 588:4223–33. doi: 10.1016/j.febslet.2014.09.039
47. Quévrain E, Maubert MA, Michon C, Chain F, Marquant R, Tailhades J, et al. Identification of an anti-inflammatory protein from Faecalibacterium prausnitzii, a commensal bacterium deficient in Crohn's disease. Gut. (2016) 65:415–25. doi: 10.1136/gutjnl-2014-307649
48. Haro C, Villatoro M, Vaquero L, Pastor J, Giménez MJ, Ozuna CV, et al. The dietary intervention of transgenic low-gliadin wheat bread in patients with non-celiac gluten sensitivity (NCGS) showed no differences with gluten free diet (GFD) but provides better gut microbiota profile. Nutrients. (2018) 10:1964. doi: 10.3390/nu10121964
49. Jiang H, Ling Z, Zhang Y, Mao H, Ma Z, Yin Y, et al. Altered fecal microbiota composition in patients with major depressive disorder. Brain Behav Immun. (2015) 48:186–94. doi: 10.1016/j.bbi.2015.03.016
50. Garcia-Mantrana I, Selma-Royo M, Alcantara C, Collado MC. Shifts on gut microbiota associated to mediterranean diet adherence and specific dietary intakes on general adult population. Front Microbiol. (2018) 9:890. doi: 10.3389/fmicb.2018.00890
51. Mikami A, Ogita T, Namai F, Shigemori S, Sato T, Shimosato T. Oral administration of flavonifractor plautii, a bacteria increased with green tea consumption, promotes recovery from acute colitis in mice via suppression of IL-17. Front Nutr. (2020) 7:610946. doi: 10.3389/fnut.2020.610946
52. Tsafrakidou P, Michaelidou AM, C GB. Fermented cereal-based products: nutritional aspects, possible impact on gut microbiota and health implications. Foods. (2020) 9:734. doi: 10.3390/foods9060734
53. Caesar R, Tremaroli V, Kovatcheva-Datchary P, Cani PD, Bäckhed F. Crosstalk between gut microbiota and dietary lipids aggravates WAT inflammation through TLR signaling. Cell Metab. (2015) 22:658–68. doi: 10.1016/j.cmet.2015.07.026
54. Urwin HJ, Miles EA, Noakes PS, Kremmyda LS, Vlachava M, Diaper ND, et al. Effect of salmon consumption during pregnancy on maternal and infant faecal microbiota, secretory IgA and calprotectin. Br J Nutr. (2014) 111:773–84. doi: 10.1017/S0007114513003097
55. Patrone V, Minuti A, Lizier M, Miragoli F, Lucchini F, Trevisi E, et al. Differential effects of coconut versus soy oil on gut microbiota composition and predicted metabolic function in adult mice. BMC Genomics. (2018) 19:808. doi: 10.1186/s12864-018-5202-z
Keywords: autism spectrum disorder, gut microbiota, nutritional status, gluten- and casein-free diet, dietary intake, children, adolescents
Citation: Mendive Dubourdieu P and Guerendiain M (2023) Understanding the link between gut microbiota, dietary intake, and nutritional status in children with autism and typical development. Front. Nutr. 10:1202948. doi: 10.3389/fnut.2023.1202948
Received: 09 April 2023; Accepted: 28 June 2023;
Published: 20 July 2023.
Edited by:
Tomás Cerdó, Maimonides Biomedical Research Institute of Cordoba (IMIBIC), SpainReviewed by:
Ana Nieto-Ruiz, University of Granada, SpainKiran Veer Sandhu, University College Cork, Ireland
Copyright © 2023 Mendive Dubourdieu and Guerendiain. This is an open-access article distributed under the terms of the Creative Commons Attribution License (CC BY). The use, distribution or reproduction in other forums is permitted, provided the original author(s) and the copyright owner(s) are credited and that the original publication in this journal is cited, in accordance with accepted academic practice. No use, distribution or reproduction is permitted which does not comply with these terms.
*Correspondence: Paula Mendive Dubourdieu, bXBtZW5kaXZlQG51dHJpY2lvbi5lZHUudXk=