- 1School of Medicine, Foshan University, Foshan, Guangdong, China
- 2Division of Cell, Developmental and Integrative Biology, School of Medicine, South China University of Technology, Guangdong, China
- 3Greater Bay Area Institute of Precision Medicine (Guangzhou), Fudan University, Guangzhou, Guangdong, China
- 4College of Letters and Science, University of California, Berkeley, Berkeley, CA, United States
- 5Reproductive Medicine Center, Department of Obstetrics and Gynecology, The First Affiliated Hospital of Anhui Medical University, Hefei, Anhui, China
- 6Department of Public Health and Nutrition, The University of Haripur, Haripur, Pakistan
Chickpea (Cicer arietinum L.), an annual plant of the family Fabaceae is mainly grown in semiarid and temperate regions. Among pulses, cultivated worldwide chickpeas are considered an inexpensive and rich source of protein. Chickpea is a good source of protein and carbohydrate, fiber, and important source of essential minerals and vitamins. The quality of protein is better among other pulses. Consumption of chickpeas is related to beneficial health outcomes. Dietary peptides from the protein of chickpeas gaining more attention. Peptides can be obtained through acid, alkali, and enzymatic hydrolysis. Among all these, enzymatic hydrolysis is considered safe. Various enzymes are used for the production of peptides, i.e., flavorzyme, chymotrypsin, pepsin, alcalase, papain, and trypsin either alone or in combinations. Chickpea hydrolysate and peptides have various bioactivity including angiotensin 1-converting enzyme inhibition, digestive diseases, hypocholesterolemic, CVD, antioxidant activity, type 2 diabetes, anti-inflammatory, antimicrobial, and anticarcinogenic activity. This review summarizes the nutritional composition and bioactivity of hydrolysate and peptides obtained from chickpea protein. The literature shows that chickpea peptides and hydrolysate have various functional activities. But due to the limited research and technology, the sequences of peptides are unknown, due to which it is difficult to conduct the mechanism studies that how these peptides interact. Therefore, emphasis must be given to the optimization of the production of chickpea bioactive peptides, in vivo studies of chickpea bioactivity, and conducting human study trials to check the bioactivity of these peptides and hydrolysate.
1. Introduction
Pulses are also called “meat for poor men,” because of the high content of their protein profile, especially in poor countries of the world, they occupy a unique place. Pulses are leguminous in nature and can be grown in all types of soil, they can help to utilize the surrounding nitrogen in the soil, help to improve the soil fertility, and significantly contribute to economic and environmental sustainability. Chickpeas are considered the most primitive legumes pulse crop cultivated around ~7500 years ago in the Middle East (1).
Chickpea (Cicer arietinum L.) which is also called Garbanzo beans is considered a good source of high-quality protein and has good nutritive value for human nutrition. There are mainly two varieties of chickpea (CP) “Desi” and “Kabuli” type. Around 80–85% of the total CP area is covered by the Desi type and the major producing countries are Africa and Asia, while for the Kabuli type the main production countries are Europe, North Africa, West Asia, and North America (2). For the production of chickpeas, India ranked 1st position, with around 6 million tons of production per year, followed by Pakistan. The other chickpea-producing countries are southern Europe, North Africa, Australia, and America. A total of 70% of the world’s chickpeas production comes from Turkey, Iran, Pakistan, and India. Worldwide chickpea is the third most key pulse crop, followed by field peas and dry beans (3). In 2020 the main importer countries for the CP were Pakistan, Bangladesh, and India, whereas Russia, Australia, and Canada were the top exporters (4) (Figure 1).
In developing countries, CP is the most important basket food. It is considered a highly consumed seed food, while the method of preparation depends on regional and ethnic factors. According to the WHO, CP contains a high level of non-starch polysaccharides and a low amount of calories. Lentils, beans, and CP are good sources of minerals, vitamins, and fiber and are recommended as an important part of a healthy diet for all types of populations. CP has good nutritional value and has the potential to be used as a functional food for the management of various diseases (5). Besides, the protein content and the dietary fiber content of the CP are highest among pulses, as demonstrated by various studies fiber rich food consumption is recommended for several diseases (3). Fiber plays an important role in weight reduction and is highly recommended for obese individuals. Moreover, dietary fiber can be helpful to reduce the risk of stroke, type 2 diabetes, coronary heart disease, gastrointestinal diseases, and hypertension (6). Furthermore, previous studies reported the antihypertensive effect of bioactive compounds from CP (7). Proteins obtained from CP are a good and balanced source of essential amino acids, with high bioavailability. Chickpea protein hydrolysate exhibit various biological activities such as reduction of antigenic activity and angiotensin I-converting enzyme (ACE) inhibition (8). Organic compounds such as isoflavones and polyphenols are helpful to reduce the risk of hypertension, inflammation, diabetes mellitus, and metabolic syndrome (1).
Chickpea is one of the major pulse crops and has been used intensively as food in developed countries. Chickpeas have nutritional value for humans and play important role in the management of various diseases. Publication about the nutritional components and bio-active peptide extraction and composition is limited. This review focused on the nutritional composition and bioactive peptide derived from CP, their method of production, and health benefits have been reviewed.
2. Composition of chickpeas
Desi and Kabuli are the two distinct varieties of chickpeas. The Desi variety has a wrinkled shape, thick seed coat, and colored flower angular seeds. The seed coat usually contains 14% of the total mass of the grain. The net seed weight for the desi variety ranges from 0.1 g to 0.3 g. the total chickpea production area is covered by the desi variety (80–85%), and the main producing countries are semi-arid areas, i.e., Africa and Asia. The Kabuli variety has a thin seed coat, white flowers, smooth seed surface, seed color is white, and seed weight ranges from 0.2 g to 0.6 g. The seed coat contains 5% of the total seed weight. Kabuli varieties are largely grown in temperate regions, i.e., Europe, North Africa, North America, and West Asia (9).
2.1. Chickpea protein content and digestibility
After cereals legumes are the most important food and are considered worldwide as a meat alternative because of their low price and sustainability. Chickpeas contain good-quality protein having higher bioavailability (10). In developing countries of Asia and Africa, protein energy malnutrition in young children and infants is common and can affect their health condition due to lack of energy and protein in the diet. In the Afro-Asian diet, pulses are a major part and can minimize the occurrence of malnutrition by providing a higher protein percentage (11).
Albumins and globulins are the predominant protein found in legumes. The globulin proteins of the legumes contain vicilin (7S), convicilin (15S), and legumin (11S). Other proteins such as prolamines and gluteins are present in small amounts. Prolamines contain high content of glutamine and proline and are soluble in alcohol. Cysteine and methionine concentrations are higher in gluteins, these two are more important amino acids for human health. Gluteins are soluble in reducing salt, base, acids, and detergents (12).
By comparing the protein content of various pulses, the content ranges from 15 to 30%. Among which content for great northern beans were (23–27%), kidney beans had (27%), navy beans and small white beans (26 and 22.7%) respectively (13). While red, pink and pinto beans were (25.3, 22.9, and 21.1%) (14). Cowpeas and black beans protein ranges from 22 to 28% and 22.9–27.7% (15). According to US pulse quality survey peas and lentil protein content were 19–30% (16). The environment and cultivar have a great influence on the overall content of protein in various pulses (17). While comparing the protein composition in edible beans, lentils, pinto beans, great northern beans and kidney beans is globulin (49–80%) followed by albumin (15–25%), glutelins (18–21%), promalins (2–4%) (18). Chickpea protein content varies significantly on dry mass base before (17–22%) and after (25–29%) dehulling. Kabuli and desi type’s protein concentrations show a significant difference in various studies (3). The protein content of the Kabuli chickpea (20.55%) was lower as compared to the desi type (29.2%) (19). While another study carried out by Singh et al. (20) showed that the protein content ranges from 15.7–31.5% (20). Kou et al. (21) revealed that the average protein content in desi and Kabuli was 22.2, and 23.4%, respectively (21). According to (22) the protein content of dried chickpea are (19–27%). Globulins (53–60%) is the major protein in chickpea while other proteins such as albumins (8–12%), promaline, and glutelins are (3–7%) and (18–24%), respectively (22). While another study investigated the protein content of six varieties of dried chickpeas, the content varies from 20.9 to 25.7%. The content of prolamine, glutein, albumin, and globulin were 19.38–24.40%, 3.12–6.89%, 8.39–12.31%, and 53.44–60.29%, respectively (12). As reported by da Silva et al. (23), globulin content in chickpeas was 41.79%, promaline, was 0.48%, gluteins 9.99% and albumin 16.18% (23).
The protein digestibility of the desi type is lower as compared to the Kabuli type. Singh et al. (24), also investigated that protein digestibility of the desi type was lower (63%) as compared to the Kabuli type (79%). According to (25) in vitro protein digestibility of raw chickpeas ranged from 34 to 76% (25). Increase in vitro protein digestibility was found by Chitra et al. for chickpea (79.4%) as compared to other pulses, i.e., soybean, mung bean, pigeon pea, and red beans (26). Various sources of research showed variability (48–89%) in the protein digestibility of chickpeas (27). The digestibility of chickpea flour can be increased by fermentation. Besides the digestibility fermentation can improve the flavor and textural properties of chickpea flour. The content of essential amino acids including phenylalanine, cysteine, threonine, tyrosine, and methionine was also higher in fermented chickpea flour (28). Geographic location, environmental variability, plant growing season, analysis method by different authors, and variety of chickpeas, all these factors are responsible for the protein composition and amount present in chickpeas (29).
2.2. Amino acids composition of chickpea
The nutritional quality of foods can be determined by the amino acids composition. The amino acid composition of great northern, black, kidney, navy, white beans pink and pinto are leucine, cysteine, aspartic acid, glutamic acid, methionine, Lysine and lysine. Navy beans and beans are poor source of histidine, methionine and cysteine (30). Chickpeas contain a high amount of amino acids, but some amino acids are deficient. Few studies show that the limited amino acids are arginine and aspartic acid (31). While other demonstrated that methionine and cysteine are found low in chickpeas (FAO/WHO 1985). However, the deficiency of these amino acids can be overcome to fulfill the dietary requirement of an individual by consuming pulses along with cereals (3). The content of essential amino acids is significantly higher in chickpea flour (39.89 g/100 g of protein) as compared to wheat flour (32.20 g/100 g of protein). Chickpea flour has a higher content of arginine, aspartic acid and glutamic acid, the sum of these three amino acids was 36.85 g/100 g of protein for Kabuli and 34.53 g/100 g of protein for desi variety. Tryptophan and cysteine two essential amino acids were not found in chickpeas in this study (20). The protein content is given in Table 1 while the content of amino acids is presented in Table 2.
2.3. Carbohydrate and dietary fiber
Pulses contain carbohydrate as a major component about (60–70%) on dry weight bases. In plant-based food dietary fiber is the non-digestible part of the human small intestine. In whole grains, the dietary fiber is carbohydrate (non-digestible) which includes soluble (slowly digested in the colon) and insoluble (metabolically inactive and aids in bowel movement), where it goes under fermentation and helps in the intestinal bacteria growth, no starch polysaccharides such as β-glucans, gums, cellulose, pectin, and hemicelluloses. Other fibers such as lignin, fructans, and oligosaccharides are not recovered by alcohol precipitation (37). Tosh et al. (38) reported that the total content of dietary fiber in chickpea ranged from 18 to 22% of which soluble fiber were 4–8%, and insoluble fiber were 10–18%. The total starch content of chickpeas is also the highest at 60.3% among the legumes with a higher value of 32.3% of amylose. Chickpea oligosaccharides ranged from (5.54–8.82%) in the different cultivars. It included a small amounts of 0.25–0.73% of verbascose, 1.54–3.18% of stachyose, 2.04–5.26% of ciceritol, and 0.42–0.86% of raffinose (38).
Aguilera et al. (15) reported that the total dietary fiber content of chickpeas was the highest (18–22 g/100 g) among the other pulses. In which the insoluble part is about 10–18 g/100 g and the soluble part is 4–8 g/100 g (15). The Kabuli type of chickpea has a lower content of total dietary fiber and insoluble fiber as compared to the Desi type. This could be due to the thinner hulls and seed coat in the Kabuli type only 4·3–4·4% of total seed weight compared to the desi type having thicker hulls and seed coat 11·5% of total seed weight. Other researchers also demonstrated that the content of dietary fiber in chickpeas varies from 18.73–21.86% (39). a-galactoside in various pulses (cowpea, horse gram) include verbascose (11, 15%), raffinose (32, 26%), stachyose (56, 58%). While in great northern beans, pinto beans, kidney beans and navy beans the stachyose content is very low (2.2, 4.2, 3.6, and 2.8%) (40). The content of sucrose in peas, mung beans, great northern beans, pinto, navy, red beans and kidney beans are 1.8 2.6, 3.0, 4.8, 3.1 1.6 and 4.1%, respectively, (41). Content of dietary fiber in different pulses, i.e., lupin, cranberry, mung beans, cow peas and lentil are 39, 15, 5%, 11–24% (42).
Chickpea grains contain a high amounts of mono-di and oligosaccharides. According to Sánchez-Mata et al. (43), the concentration of mono-saccharides in chickpeas were ribose, 0·11 g/100 g, galactose, glucose, 0·7 g/100 g, 0·05 g/100 g and fructose, 0·25 g/100 g. Maltose (0·6%) and sucrose (1–2%) have been reported to be the most abundant free disaccharides in chickpeas. Disaccharides include sucrose 1–2% and maltose 0.6%, while the main oligosaccharides stachyose, ciceritol, raffinose, and a small amount of verbascose. The content of stachyose and Ciceritol in chickpeas were 25% and 36–43%, respectively (43). The content of total dietary fiber and carbohydrates in chickpeas by different researchers are presented in Table 3.
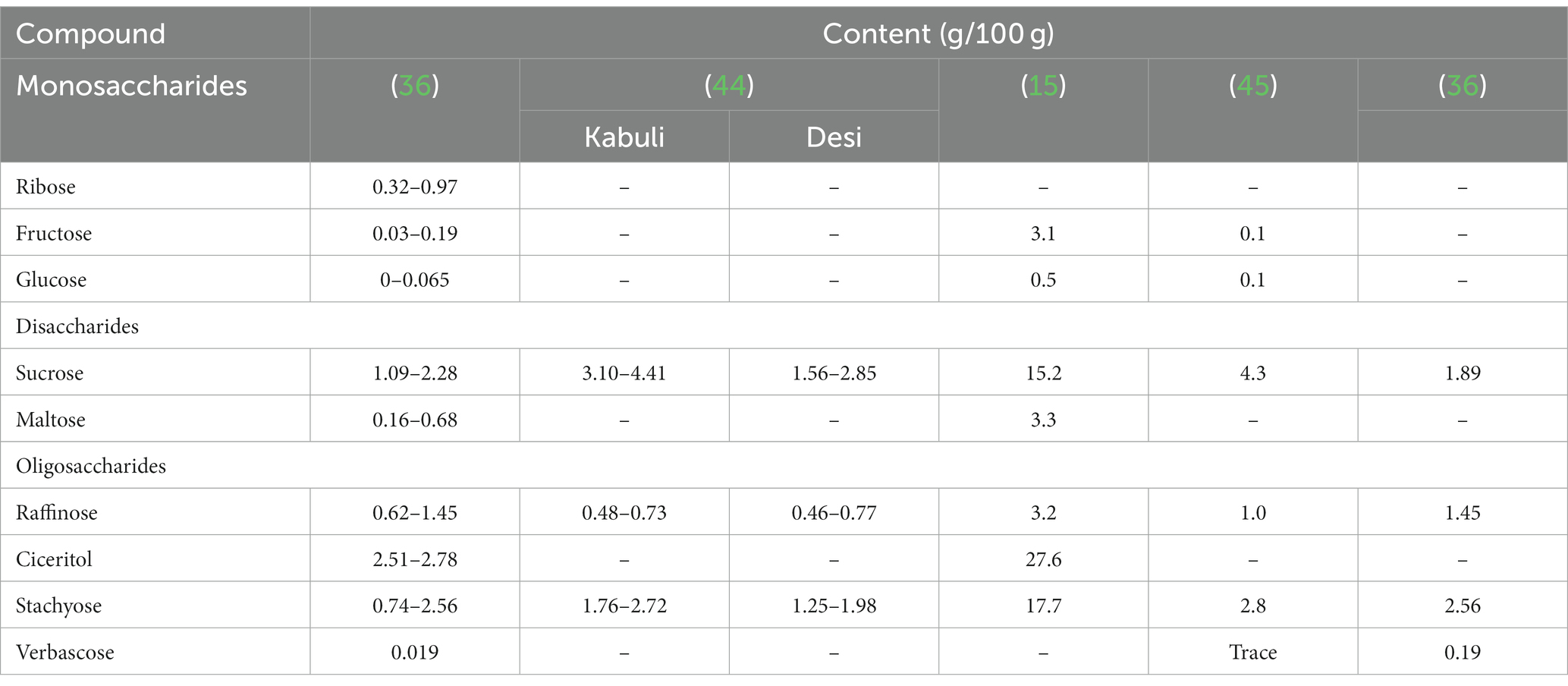
Table 3. The content of total dietary fiber and carbohydrates in chickpeas by different researchers.
2.4. Fat content and fatty acid composition of chickpea
Generally, the content of lipids is lower in pulses, i.e., 1–3% in peas, 2–3% in mung beans, and 2% in cowpea. The TAG and PhL content in kidney beans, peas and mung beans are (34–46%, 31–40, 31%) and (49–59%, 52–61, 31%), respectively (46). However, chickpea presents higher content of lipids content up to 7% (22). In pulses, lipids are usually present in the form of sterols, phospholipids (PhL), triacylglycerol (TAG), steryl esters, and free fatty acids (FFA). The phospholipids (PhL) content of chickpeas was around 17–20% and TAG content was 56–67% as reported by Zia-Ul-Haq et al. (29). The fatty acid profile of chickpeas revealed the content of linoleic and oleic acids (nutritionally essential fatty acids), both are present in high amounts. Linoleic acid content was highest followed by palmitic acid and oleic acid. Two important essential fatty acids required for physiological functions, growth, and maintenance are linolenic acid and Linoleic acid. Linoleic acid regulates smooth muscle contraction, reducing blood pressure through the production of prostaglandins. In chickpea composition majority of the fatty acids are unsaturated while saturated fatty acids contribution is minor. This makes the chickpea an exceptional legume from a nutritional point of view. Summo et al. (7) investigated three varieties of chickpeas. Among these, beige chickpea have the highest content of monounsaturated fatty acids as compared to black and brown chickpea. While the content of polyunsaturated fatty acid was the lowest (50.3%) in the beige variety. The content of polyunsaturated fatty acid was significantly higher in the brown (67.0%) and black (64.4%) varieties. This may be due to the higher concentration of linoleic and linolenic acid in brown and black chickpeas (7).
According to Barbana and Boye (31) the lipid content ranges from 4.5 to 6.0 g/100 g. In neutral lipids, the major component is triglycerides while in polar lipids the major component is lecithin. High levels of essential unsaturated fatty acids linolenic acid (0.5–0.9% in oil), linoleic acid (54.7–56.2% in oil), oleic acid (21.6–22.2% in oil), and low level of stearic acid (1.3–1.7% in oil) and palmitic acid (18.9–20.4% in oil) were observed. Other minor amounts of fatty alcohols, waxes, and sterols are also present in chickpea lipid composition. The lipid composition of chickpea is tabulated in Table 4.
2.5. Minerals content
High content of magnesium, potassium, manganese and iron are found in pulses. Other minerals include calcium, selenium, zinc and copper. According to (48), field peas have an average of 0.047, 1040, 301, 5.4, 117, and 1.3 (mg/100 g) of selenium, magnesium, manganese potassium, iron and zinc content. Mineral composition of lentil include iron (6–9 mg/100 g), calcium (59–69 mg/100 g) potassium (844–943 mg/100 g) of eight different cultivar (49).
Like other pulses, chickpeas not only provide variation in the daily diet of Asian and African people but also provide essential minerals and vitamins. As an important component of enzymes and cells, minerals involves in the majority of defense mechanisms. They act as activators, inhibitors, and regulators of metabolism. In chickpea, the content of zinc, phosphorous, and manganese is higher as compared to other pulses (10). As a good source of zinc and iron chickpeas can play a vital role to overcome the malnutrition of minerals. The content of iron and zinc in chickpeas ranged from 4.56 mg/100 g to 9.87 mg/100 g and 0.96 mg/100 g to 4.05 mg/100 g (10). The content of Fe and Zn ranged from 4.6 mg/100 g to 6.7 mg/100 g and 3.7 mg/100 g to 7.4 mg/100. Other minerals calcium, magnesium, manganese, and copper content ranged from 40.83 mg/100 g to 260.95 mg/100 g, 71.96 to 187.86 mg/100 g, 1.31 mg/100 g to 3.69 mg/100 g, and 0.13 mg/100 g to 1.07 mg/100 g, respectively. Wild species of chickpea have a higher content of manganese, magnesium, and calcium as compared to genotypes. Magnesium and calcium content ranged from 125 to 159 mg/100 g and 93 to 197 mg/100 g. Manganese and copper content in 28 chickpea genotypes ranging from (1.3 to 2.1 mg/100 g) and (0.66 to 1.04 mg/100 g), respectively. Zia-Ul-Haq et al. (29) investigated the minerals content of 4 desi chickpea varieties grown in different areas of Pakistan. The content of potassium ranged from 1109 mg/100 g to 1236 mg/100 g. All varieties have a notable amounts of copper, calcium, and zinc. According to these results, chickpeas can provide an adequate amount of minerals to fulfill the human body’s mineral requirement.
According to (3) 100 g of raw chickpea seed provides approximately 160 mg/100 g of Ca, 138 mg/100 g of Mg, 4·1 mg/100 g of Zn, and 5·0 mg/100 g of Fe. The daily requirement of zinc, iron, and magnesium can be fulfilled through chickpea 100 g/day consumption (3). Desi and Kabuli chickpeas showed no significant difference and minerals content, only in the case of calcium Kabuli type have a lower concentration as compared to the desi type. The minerals composition of chickpea is presented in Table 5.
2.6. Vitamins content of chickpea
A minute amount of vitamins is required per day, a well-balanced combination of pulses, vegetables, meat, fruits, dairy products, and cereals can fulfill these requirements. Pulses are considered a good source of water-soluble vitamins especially vitamin B. In baked products, the use of pulses improves the baked quality of the food items and also provides a high folate content. The folate content of pulses varies from one type to another, in addition, it is influenced by cultivar, composition, and growing location (51). A notable difference is found among pulses folate content. Not only the pulse type, the type of cultivar and growing location also influence the folate concentration. The content of folate in lentils, field peas, yellow peas and green peas were 146–290 μg/100 g, 26–202 μg/100 g, (41–55 μg/100 g) and (50–202 μg/100 g), respectively (52). While in mung beans, cow peas and kidney beans the content was (141–169 μg/100 g) and 96 μg/100 g and 103 μg/100 g (53). Content of thiamine and riboflavin in lupin and lentil was (360–390 μg/100 g, 560 μg/100 g) and (610–650 μg/100 g, 280 μg/100 g) (54). Other vitamins such as niacin and vitamin C has been observed in very minute amount.
Chickpea is a good source of tocopherol and folic acid, other water-soluble vitamins (riboflavin, pyridoxine, and pantothenic acid). The concentration of these vitamins was observed highest as compared to other pulses. According to (22) the folate content in field peas was 26–202 μg/100 g, and in lentils the content was 146–290 μg/100 g. Various peas classes having variability on folate content, green peas (50–202 μg/100 g) having higher folate content as compared to yellow peas (41–55 μg/100 g) (22). Chickpeas have higher folate content of 42–537 μg/100 g. In pulses, riboflavin and thiamin are also present in high concentration. The content of thiamin in chickpeas was 453 μg/100 g, while riboflavin content was 173 μg/100 g. The content of niacin, riboflavin, pyridoxine, and thiamin was reduced during cooking. Leaching and chemical destruction lead to the loss of vitamins. Loss of vitamins by various cooking methods is different. High content of vitamin loss occurs due to boiling as compared to microwave cooking and autoclaving. Table 6 shows the vitamin content of chickpeas.
2.7. Phytochemical composition
Flavonoids, tannins, isoflavones and phenolic acids are the main phenolic compounds found in pulses. Polyphenol content of lentil, mung beans and anchor was 49.45, 98.02, and 48.41 of mg/100 g of GAEs. Tannins contents varies 213.97, 447.98, 212.52 mg/100 g of TEs. Flavonol and flavonoid content of lentil, mung beans and anchor were 0.05, 0.04, 0.02 mg/100 g of QEs and 475.07, 734.08, 771.35 mg/100 g of CEs (34).
Chickpeas have a good reservoir of bioactive compounds, and having health protective value (7). Flavonoids and polyphenols are two important phytochemical presents in chickpeas in higher quantities, these two compounds act as good antioxidants. The content of flavonoids and polyphenols depends on the chickpea color, the darker the color, the higher will be the concentration. Method of polyphenols extraction, reagents used in extraction, extraction time, and analysis methods all these factor affect the content of phenolic compounds in chickpea. Polyphenolic compound ranges from 0.72 to 1.81 mg/g, anthocyanin content was 14.9 mg/kg of beans. The content of anthocyanins and polyphenols is lower in chickpeas while phenolic acids such as hydroxycinnamic, anise, caffeic, cinnamic, p-coumaric, chlorogenic isoferulic, and piperonyl are present in high amounts. Strong antioxidant properties (reduce oxidative stress, chelate metal ions) is characterized by phenolic acids present in chickpea (60).
Zhao et al. (1) reported the content of total flavonoids, total phenolics, and anthocyanins in 6 different chickpea varieties (1). Results reveal that with coat pigmentation color intensity the content of flavonoids, total phenolics, and anthocyanins was higher as compared to the light-colored coat. In terms of flavonoids in six species, the content ranged from 0.021 ± 0.002 to 0.1 ± 0.01 mg Rutin/g. Content of carotenoids in black and brown chickpeas showed significantly higher values (36.4 mg/kg and 35.2 mg/kg, respectively) compared to beige type. Other researchers also reported that the content of carotenoid was higher in the desi type as compared to the Kabuli chickpea, due to the intensity of seed coat color (51). Anthocyanin content was higher in the beige type as compared to brown. The content in black chickpeas ranged from 23.3 to 159 mg/kg. In terms of total phenolic compounds interestingly no significant difference was found, in brown chickpeas the content was 0.8 mg/g and in black chickpeas was 0.7 mg/g. The daily intake of bioactive compounds and dietary fiber can be improved by the intake of brown and black chickpeas. Positive health benefits for human health can be obtained as chickpeas have a lower glycemic index, and help to lower the risk of diabetes and heart diseases (25.3 mg/kg) (7). Another study carried out by Kaur et al. (10), reported that major phenolic compounds presents in chickpeas are flavonoids, phenolic acids, and condensed tannins (10). These compounds play an important role in the prevention and elimination of existing ROS (reactive oxygen species) from blood. Phenolic compounds have other health benefits such as anti-inflammatory, anti-thrombotic, immune-modulating, anticarcinogenic, antioxidant, cardioprotective, anti-ulcer, anti-atherogenic, analgesic agents, anti-microbial, and anti-allergenic. The content of flavonol in chickpeas ranged from (0.80 mg/100 g to 24.20 mg/100 g). In the wild type, the content was highest (7.94 mg/100 g to 24.20 mg/100 g), followed by desi genotypes type from (5.04 mg/100 g to 13.18 mg/100 g) and desi type (95.04 mg/100 g to 13.18 mg/100 g). The TPC (total phenol content) in chickpeas in different varieties ranged from (27.48 mg/100 g to 113.30 mg/100 g). The content of TPC was lower in the Kabuli type 27.48 mg/100 g to 48.01 mg/100 g, while in the desi type the content ranged from 38.59 mg/100 g to 83.52 mg/100 g. In the wild type, the content varies from medium to high, ranging from 63.08 mg/100 g to 113.30 mg/100 g. Due to the dark-colored seed coat of wild and desi types the TPC was higher as compared to the Kabuli type. The details of bioactive compounds in chickpea is given in Table 7.
3. Bioactive peptides from chickpeas
Traditionally for the growth and maintenance of body functions, dietary protein is required which provides energy as well as a balanced source of essential amino acids. The consumption of different compounds, provides beneficial health effects, these compounds are stated as bioactive compounds (62). Plant and animal sources are considered could be good sources of bioactive peptides. In the parent protein sequence, bioactive peptides are specific protein fragments that are active, after enzyme, and acid/base hydrolysis they are released and have numerous biological functions. The molecular weight of these peptides is <6 kDa and usually contains 2–20 amino acids. The activity of these peptides is highly dependent on the sequence and composition of amino acids, these peptides possess various activities such as antioxidative, mineral binding, antimicrobial, hypocholesterolemia, immunomodulatory, and antihypertensive (63). In the past 20 years, various enzymes such as flavorzyme, pepsin, papain, chymotrypsin, and alcalase are used to produces chickpea bioactive peptides. Chickpea peptides have various activities such as hypocholesterolemic, antifungal, anti-inflammatory, anti-obesity, antioxidant, hypoglycemic, and antihypertensive (64).
3.1. Production of bioactive peptides
Chickpea is the second largest producing pulse crop worldwide. It is a good source of essential amino acids and contains a higher percentage of dietary protein 15–30%. For the production of chickpea hydrolysate and peptide, numerous methods have been applied, which improve the functional properties such as angiotensin I-converting enzyme (ACE) inhibitory activity, metal-chelating ability, antihyperlipidemic, antioxidant, antiproliferative, and antitumor activity (65). Flow chart for the peptide extraction, purification and identification is summarized in Figure 2. Through hydrolysis of protein, peptides can be produced, which can be done through acid–base or enzymatic hydrolysis (either by enzymes obtained from plants and microorganisms or digestive enzymes) and microbial fermentation. In South East Asian countries (Japan, Korea, and China) fermentation is the ancient way used for food preservation. Besides increasing the shelf life, fermentation can improve the nutraceutical value of food, this is because microbial proteases generate bioactive peptides from protein fragments (66). Usually, enzymatic hydrolysis is used for the production of peptides, but as compared to acid–base hydrolysis enzyme hydrolysis is costly. Enzymatic hydrolysis improves nutritional properties as well as functional properties such as emulsifying properties of hydrolyzed protein. Chickpea protein concentrate or isolate and chickpea flour can be used for the production of peptides. Chickpea protein isolate and protein concentrate are usually used instead of chickpea flour due to their higher percentage of protein. Chickpea flour contains carbohydrate and lipids molecules which can reduce the rate of hydrolysis (62, 67).
During the past 20 years various enzymes pepsin, papain, flavorzyme, alcalase, trypsin, and α-chymotrypsin used alone or in combination for the production of chickpea peptides. Further research is needed for the optimization of the production of chickpea peptides from chickpea (64). Antioxidant activity of beta-conglycinin and glycinin showed higher activity after enzyme hydrolysis, after enzyme hydrolysis more active R-group of amino acid will release and produce higher activity. The unfolding of hydrophobic amino acids also leads to increase the activity. The protein nature, degree of hydrolysis, and molecular mass of the released peptides are responsible to increase or decrease the hydrophobicity. A peptide having molecular mass < 1 kDa showed improved activity and bitter taste (63). The antioxidant activity of hydrolysate is effected by enzyme specificity, amino acid sequence, and composition of peptides, peptide size widely depends on the type of enzyme used for hydrolysis. In other research used soy protein isolate, various enzymes were used to produce hydrolysate with different degrees of hydrolysis. A mixture of the peptide was produced and showed antioxidant activity according to the degree of hydrolysis (68). Hydrolysis of chickpea protein by alcalase showed higher antioxidant activity. Reducing power and hydroxyl radical scavenging activity of chickpea protein hydrolysate improved by combined treatment with alcalase and ultrasonic treatment with a degree of hydrolysis of 20.03%. Besides this chromatographic fractionation was performed to isolate a novel peptide from alcalase chickpea protein hydrolysate which showed hemolytic activity on bovine erythrocytes (69). It is stated that aclalase-derived peptides have the low molecular weight and various functional properties as compared to other enzymes (70). Barbana and Boye (31) reported that sequential hydrolysis of chickpea protein with alcalase and flavorzyme generates higher DH (58.89–78.58%). It reveal that the combine use of Exo and endo-proteases improve the degree of hydrolysis and N-terminal sides, alternately improving the functional properties of hydrolysate (Figure 3).
4. Health benefits of chickpea peptide and hydrolysate
4.1. Antioxidant activity
Food quality and human health both are affected by free radicals, in body macromolecules such as carbohydrate nucleic acids (DNA and RNA), polymers, and unsaturated lipids are damaged by unstable free radicals, causing oxidative stress. As a result many health problems such as arteriosclerosis, diabetes mellitus, neurodegenerative diseases, cancer, inflammatory diseases, and arteriosclerosis (71). While in food oxidation occurs in the presence of free radicals, which cause a change in food texture, color and flavor, and nutritive quality (1).
Several synthetic antioxidants, i.e., butylated hydroxytoluene, butylated hydroxyanisole, and propyl gallate have been used in the food industry for food preservation. However, use of synthetic antioxidants causes possible health hazard and food stability problems. Natural antioxidants could be the best option to replace synthetic antioxidants. Several natural compounds such as vitamin E, phenolic acids, flavonoids, ascorbic acid, carotenoids, protein and protein hydrolysates, and peptides retain antioxidant activity. Different protein sources have been used to generate peptides and hydrolysates having antioxidant activity, it present admirable options to be used to prevent oxidative stress, and used this protein sources as a natural antioxidants and nutritional supplement (72).
To upgrade the functional and nutritional characteristics of proteins, enzymatic hydrolysis is widely used to produce antioxidant peptides. A study reported that low molecular mass peptides having hydrophobic amino acids such as Val or Leu in their N-terminal showed higher antioxidant activity. Stronger antioxidant activity was observed in the presence of Tyr, Phe, and Trp (aromatic amino acids) and Met and Cys (sulfur-containing amino acids) (73). Chickpea protein and hydrolysates by various enzymes have been reported by various researchers. By the use of different biochemical assays, antioxidant activity of hydrolysate and peptides have been reported. Hydrolysates and peptides obtained from chickpeas showing antioxidant activity are presented in Table 8.
Quintero-Soto et al. (79) studied the antioxidant activity of chickpea protein (albumin and globulin) produced by alcalase. Obtained peak showed the highest activity was purified for the peptide sequence, three similar structure peptides have purified (FEI, FEL, FIE). Peptide fraction AH1-5 was evaluated for ABTS and DPPH activity (90.2 mg/mL) and activity ranged from 37.39 to 78.25% for ABTS and 73.65–74.14% for DPPH. The obtained values were higher than those obtained by Zhang et al. (81) who found antioxidant activity for soy hydrolysate synthetic peptides (81). The reason behind the highest ABTS activity is the presence of aromatic amino acids, the benzene ring is responsible to stabilize the free radicals by the donation of the electron. The antioxidant activity is also higher due to the presence of leucine at the C-terminus (82). In the case of DPPH activity higher activity was found in peptides having the highest hydrophobicity.
A study carried out by another researcher hydrolyzed chickpea sprout protein by various enzymes (trypsin, nutrase, alcalase, and papain). They found the nutrase fraction has the highest DPPH and OH activity. The hydrolysate was purified by IEC, GFC, RP-HPLC, and MALDI-TOF-MS/MS. The fraction having the highest DPPH and OH activity was identified for the amino acid sequence, the molecular weight of the peptide was 685.41 Da and the amino acid sequence was LTEIIP (Thr-Glu-IIe-IIe-Pro). Results suggested that peptides obtained from plant proteins having antioxidant activity are commonly short-chain amino acids (less than 15 amino acids) and contain Leu, Pro, Val, and Ile (hydrophobic amino acids) and Asn and Asp, Gln, and Glu (acidic amino acids) (75). Enzymatic hydrolysis of chickpea protein concentrate used as starting material, two peptides P3 and P8 purified showing higher antioxidant activity. The molecular mass of these peptides were 327, 33, and 402, 49, and the amino acid sequence was Asp-His-Gly and Val-Gly-Asp-Ile. As compared to P3, P8 exhibit higher antioxidant activity. In another study, four antioxidant peptides (ALEPDHR, TETWNPNHPEL, FVPH, and SAEHGSLH) from chickpea protein hydrolysate were reported and their antioxidant activity was studied in CaCo 2 cells. Three peptides SAEHGSLH, FVPH, and TETWNPNHPEL showed higher cellular antioxidant activity while one peptide ALEPDHR showed lower activity (83).
4.2. Diabetes
Diabetes mellitus can be defined as a chronic disease which results in an increase in blood sugar levels as a result of the pancreas producing insufficient insulin (84). Plant-based diet is widely used in the management and prevention of diabetes (85). Chronic metabolic disease diabetes represents a globally health problem with strong health and socioeconomic impact. About 90% of the world’s diabetic population is suffering from type 2 diabetes mellitus, the number is growing rapidly reaching up to 439 million by 2030 (86). Type 2 diabetes is also associated with other chronic diseases such as retinal damage, neuropathy, chronic renal failure, microangiopathy, and cardiovascular disease. The cost of diabetes mellitus will reach up to 2.5 million USD in 2030. This indicates that there is an urgent need to control and prevent type 2 diabetes (87).
Synthetic medicines like voglibose and acarbose are widely used for the control of type 2 diabetes, which inhibit the carbohydrate hydrolyzing enzymes (α-glucosidase, α- amylase) and suppress glucose absorption. These drugs have some side effects such as flatulence (78% of patients) and diarrhea (14% of patients) and the costs of these drugs are also high (88). Another approach to controlling type 2 diabetes is the inhibition of the dipeptidyl peptidase-IV enzyme. Several inhibitors such as sitagliptin, saxagliptin, linagliptin, and vildagliptin which are collectively called gliptins, cannot control glycemic load adequately and also have some side effects such as headaches, weight gain, urinary and upper respiratory tract infections, hypoglycemia and cardiovascular problems (89). There is a complex mechanism in the body to regulate insulin production and control the glucose level. Currently, the search for natural therapeutic products which have limited or no side effects for the treatment of disease is growing. For the management of type 2 diabetes food-derived compounds such as flavonoids, phenols, proteins and peptides, which have the potential for an antidiabetic activity is of research interest. Recent research reported that some proteins, amino acids, peptides, and protein hydrolysates play important role in blood glucose regulation.
GI (glycemic index) can be defined as the ability of various foods to raise the blood glucose level. The glycemic conditions must be controlled by the persons having diabetes. People must choose foods with carbohydrate sources which cause a slow release of glucose after consumption. Foods considered a good source of energy and carbohydrates for diabetic persons are beans and pulses having lower GI, which help in the regulation of insulin secretion and glycemic condition in type 2 diabetic persons (90). Significant reduction in triglyceride and glucose levels was observed in diabetic rats by feeding chickpea seeds with a dose of (400 mg/kg). The study reported that bromelain-producing chickpea hydrolysate has a peptide sequences containing glycine amino acid. The length of amino acid chains ranged between 7 and 18 amino acids. The peptide contains hydrophobic amino acids having the potential to inhibit type 2 diabetes.
Chandrasekaran and De Mejia (91) investigated peptide sequences obtained from germinated chickpea isolated protein produced by ficin enzyme. Three peptide sequences SPGAGKG, GLAR, and STSA were isolated by LC-ESI-MS/MS. The antidiabetic potential of peptides was evaluated through DPP-IV and α-glucosidase inhibition. Results showed that SPGAGKG is more active than GLAR to inhibit both α-glucosidase and DPP-IV inhibition. Chickpea peptide can be used as a potential source for the production of functional food ingredients. Chickpea peptides and hydrolysate having antidiabetic properties are depicted in Table 9.
4.3. Hypertension
Hypertension or high blood pressure is the leading risk factor for heart disease. Other diseases such as heart failure and renal disease, stroke, and myocardial infarction are closely related to hypertension. About 9.4 million people death occurs due to the aforementioned diseases. Cardiovascular function and blood pressure are regulated by dipeptidyl carboxypeptidase angiotensin I–converting enzyme (EC 3.4.15.1), which converts the inactive decapeptide angiotensin I into the potent vasoconstricting octapeptide angiotensin II, by removing dipeptide from the C-terminus, which can increase blood pressure. ACE inhibitors help to reduce the occurrence of hypertension, there is still confusion that which option is best either to inhibit the receptors that bind angiotensin II and signal vasoconstriction or ACE directly (93). Many drugs used for the treatment of blood pressure are related to the inhibition of angiotensin-I converting enzyme. Synthetic drugs (captopril, enalapril, and Lisinopril) are useful for a short time use, but these drugs have serious side effects. To overcome the disadvantages of synthetic drugs researchers are interested to find natural inhibitors for angiotensin I-converting enzymes. In this regards peptide have some inhibitory function for angiotensin I-converting enzyme gaining popularity (94).
Several peptides from food sources, i.e., legumes have the potential to inhibit angiotensin I-converting enzyme. Chickpea protein and hydrolysate showed ACE 50% activity is reported and the methods used for the study are well standardized. Chickpea hydrolysate produced by papain enzyme is the most potent sample for the inhibition of the ACE enzyme (IC50 = 0.010 μg/mL). ACE inhibitory activity of chickpea flour hydrolysate also showed activity (95). Previous research reported four ACE inhibitory peptides from enzymatic hydrolysate of chickpea protein having low molecular weight < 1 kDa, having ACE inhibitory activity IC50 0.1 mg/mL. Two of the peptides showed strong activity. Higher amino acid residue number of peptides shows the highest activity (96). In another study protein hydrolysate obtained from chickpea desi variety having molecular weight < 4 kDa exhibited strong ACE activity with the IC50 of 140 ± 1 μg/mL (31). To obtain the single chickpea peptide effect on ACE inhibition, studies are required to check the activity in animal models and clinical studies to confirm the discussed outcomes. Chickpea peptides and hydrolysate having antihypertensive properties are given in Table 10.
4.4. Cancer
Cancer is one of the leading causes of death, having high incidence worldwide, it is a chronic non-communicable disease described by invasion and metastasis, uncontrolled cell replication, increased proliferation, resistance to apoptosis, and evasion of tumor suppressor genes. 14.1 million cases of cancer were reported in 2012, while in 2030 it is estimated that 22 million new cases and 13.2 million deaths will occur (100). Initiation, promotion, and progression are the three stages of cancer genesis and evolution. Vascular invasion, survival of tumor cells, metastasis, and progression of malignancy are related to the overexpression of PCNA (101). Among all types of cancer colon cancer is the third most frequently occurring cancer type worldwide in both genders. Various factors (genetic and epigenetic) are related to its pathogenesis. Some case–control studies reveal the fact that hypercaloric diets rich in fats and carbohydrates are mainly responsible for the development of cancer (102). After hydrolyzing some foods especially protein generate some compounds (peptides) with various biological activities. Peptides obtained from soybean, beans, and chickpea protein hydrolysate having hypolipidemic, antioxidant, antiproliferative, metal chelating, an inhibitor of the angiotensin-converting enzyme, hypocholesterolemic and anticarcinogenic activities (100). Chickpea consumption is reported to have health benefits due to its composition of complex carbohydrates and protein. Real Hernandez et al. (64) reported two animal studies that reported the anticarcinogenic activity of hydrolysate of chickpea protein. Sequential hydrolysis of chickpea protein concentrate by pepsin followed by pancreatin did not significantly decrease the number of aberrant crypts in mice with cancer, but was helpful in the regulation of cholesterol homeostasis in mice. Chickpea albumin hydrolysate formed by alcalase followed by Flavourzyme fed to mice (100 mg per kilogram of body weight/day) has significantly inhibited and reduced the number of tumors in mic. However human studies are still required to clear that chickpea protein hydrolysates and peptides exhibit anticarcinogenic effects. The previously mentioned peptide (RQSHFANAQP) having hypolipidemic and antioxidant activity, was also reported to decrease the capability of cancer cells. Peptide (RQSHFANAQP) fragment ANAQ is also found to interact with protein p53 in silico, which is actively involved in the cancer progression. However to confirm to the role of chickpea peptide and hydrolysate in cancer prevention more interventional research is required.
4.5. Other health benefits
Antidiabetic, anticancer, antihypertensive, and antioxidant are not only the bioactivities of chickpea peptides that have been reported. Peptides and hydrolysate of chickpeas possess some other activities such as antimicrobial, anti-inflammatory, hypocholesterolemic, and some skin health-promoting activities. Pathogenic and spoilage bacteria spoil the food and decrease the shelf life as well damage the food safety in the food industry. The food industry tried natural and synthetic antibacterial agents to avoid such damage occurred by bacteria. Due to the negative effect of the chemicals on human health, the food industry tried to restrict these antibacterial agents (103). To avoid toxic effects there is a need to search natural biomolecules. In this case, bioactive peptides can be used as alternatives to the synthetic antibiotics in food and pharmaceutical industries due to their low toxicity and high specificity (104). Such bioactive peptides can be obtained from food protein sources such as vertebrates, fish, eggs, wheat, insects, plants, milk, and bacteria (105). In the last year some plant proteins have been identified to prevent the development of microorganisms (106). Chickpea peptides were isolated some years ago, these peptides were arietin (5.6 kDa) and cicerin (8.2 kDa), and having antifungal activity against Botrytis cinerea, Mycosphaerella arachidicola, and Fusarium oxysporum. Another study also reported that peptide having molecular mass 8 kDa (Val-Lys-Ser-Thr-Gly-Arg-Ala-Asp-Asp-Asp-Leu-Ala-Val-Lys-Thr-Lys-Tyr-Leu-Pro-Pro) purified from green chickpea showed activity against Mycosphaerella arachidicola, B. cinerea and Physalospora piricola (107).
A physiological process that starts in the response to biological, chemical, or physical injury is called inflammation. This response is the protection of the body against infection, some chronic diseases such as cancer obesity, rheumatoid arthritis, diabetes, osteoporosis, and cardiovascular diseases, occur if the inflammation becomes chronic and excessive production of mediators such as tumor necrosis factor (TNF-α) and interleukin (1 and 6) are the major cause. Several drugs are used for the treatment of these diseases, but drugs have some side effects. To control the production of these mediators and to overcome the side effects of the drugs, researchers are trying to search for alternative treatments from natural sources (108). Chickpea seeds have been found to have some molecules possess the ability to inhibit the process of inflammation through various mechanisms. Recently study reported that chickpea extract has the potential to reduce the activity of cyclooxygenase-2 (COX-2), nuclear factor kappa B (NF-ĸB), and TNF-α in rats (109). Another study reveal that peptides fraction and phenolic extract at the concentration of (5 mg/mL and 0.5 mg/mL) have the ability to inhibit the production of nitric oxide in RAW 264.7 macrophages induced by lipopolysaccharides (LPS) (110). Peptide (RQSHFANAQP) purified from chickpeas have significantly reduced the expression of tumor necrosis factor- α in rats that fed on 20 mg peptide/kg of body weight/day (64). Another study reported that germinated chickpea protein hydrolysate contains hydrophobic peptides which inhibit the production of NO (nitric oxide) an inflammatory signaling molecule, but the sequence of the peptide was unknown (110). The peptide (LHQNIGSSSSPDIYNPQAGR) significantly reduced human α-amylase, but these findings also need some further verification (111). Intensive research is required on the anti-inflammatory effect of peptide and hydrolysate obtained from chickpea.
The high consumption of saturated fat, low consumption of dietary fiber, and low physical activity, cause high concentrations of cholesterol and lipid in the blood. Various metabolic and cardiovascular disorders such as coronary cardiopathy, atherosclerosis, obesity, pancreatitis, and fatty liver are linked with hyperlipidemia. To reduce the blood lipid level in the blood researchers are interested to search for bioactive compounds which can be used for this purpose. Recent research reported that the consumption of chickpea has been associated with a reduction of blood lipid level due to their high fiber and low lipid composition (112). Hydrolysate obtained from chickpea albumin by alcalase-flavourzyme was fed (150 mg/kg) to mice with a high-fat diet. The result showed an increase in high-density lipoprotein (HDL) cholesterol levels by 46.75, 36.55, 48.53, and 15.34%, respectively, and a decrease in total triglycerides in serum, LDL cholesterol, and cholesterol levels (113). Another study reveal a 50% reduction in the cholesterol level by the consumption of chickpea hydrolysate obtained through flavourzyme and alcalase (96). A peptide having amino acid sequence Arg-Gln-Ser-His-Phe-Ala-Asn-Ala-Gln-Pro from chickpea protein demonstrated to Kunming rats with a high-fat diet for 4 weeks, results attained decrease in serum and hepatic triglycerides and cholesterol level (114). Hydrolysate obtained through pepsin-pancreatin enzyme from chickpea protein showed a reduction in the concentration of LDL, of triglycerides level in mice. This effect is due to the concentration of hydrophobic amino acids present in hydrolysate, which are helpful in the excretion of lipids in feces (100). Significantly increased HDL level and reduction in triglycerides, total cholesterol, and LDL contents in serum were observed in obese rats fed with a fat-rich diet with chickpea peptide (112).
5. Conclusion
This review summarized information about the nutritional benefits of chickpea and its role in the health improvement. Chickpea consumption not only provide basic nutrition but also have health benefits. It is a cheap and easily available source of health-promoting fatty acids, carbohydrates, minerals and vitamins, folate, protein and b-carotene. Chickpea provide potential health benefits and lowering the development and progression of numerous chronic diseases (CVD, type-2 diabetes, etc.). Chickpea also contain bioactive peptides, which can produced through enzymatic hydrolysis. Peptide can be produced with food approved enzymes such as alcalase, flavorzyme, chymotrypsin, pepsin, papain, trypsin and pancreatin. Chickpea hydrolysate and peptides also have been health outcomes such as anticarcinogenic, skin health promoting bioactivities, anti-inflammatory, hypolipidemic, antioxidant, antihypertensive, antifungal, and antidiabetic. Some peptides such as RQSHFANAQP, NRYHE and VFVRN purified from chickpea showed health benefits but human studies are still required to conform the biological activity of these peptides in humans. Chickpea peptide and hydrolysate and peptides can be produced enzymatically and added to food products to promote human health. However research should be carried out to provide evidence and unravel the mechanism of chickpea peptide and hydrolysate involved in the disease prevention and promoting human health. Processing methods required for the production of bioactive peptides and to reduce the production of harmful (antinutrients compounds) need attention.
Author contributions
NB and QK contributed to write the first draft of the manuscript, conception, and design of the study. IH organized the database and performed the statistical analysis. DL revised and supervised the work. All authors contributed to manuscript revision, read, and approved the submitted version.
Funding
This work was financially supported by the National Natural Science Foundation of China (grant nos. 82270413 and 81870307), the Natural Science Foundation of Guangdong Province of China (grant nos. 2022A1515011368 and 2023A1515011581), the Key Projects of Department of Education of Guangdong Province of China (grant nos. 2022ZDZX2057 and 2022ZXKC474). Guangdong Basic and Applied Basic Research Foundation of China (2022A1515111169 and 2022A1515110595), the Special Fund for Science and Technology Innovation Cultivation of Guangdong University Students (no. pdjh2023a0543), Comprehensive Application Research and Industrialization of “JinDengLong” Health Products (no. 2120197000214), and The Student Academic Fund of Foshan University (no. xsjj202212zra01). Guangzhou Basic Research Program 2023 (2023A04J0708).
Conflict of interest
The authors declare that the research was conducted in the absence of any commercial or financial relationships that could be construed as a potential conflict of interest.
Publisher’s note
All claims expressed in this article are solely those of the authors and do not necessarily represent those of their affiliated organizations, or those of the publisher, the editors and the reviewers. Any product that may be evaluated in this article, or claim that may be made by its manufacturer, is not guaranteed or endorsed by the publisher.
References
1. Zhao, X, Sun, L, Zhang, X, Wang, M, Liu, H, and Zhu, Y. Nutritional components, volatile constituents and antioxidant activities of 6 chickpea species. Food Biosci. (2021) 41:100964. doi: 10.1016/j.fbio.2021.100964
2. Pande, S, Siddique, K, Kishore, G, Bayaa, B, Gaur, P, Gowda, C, et al. Ascochyta blight of chickpea (Cicer arietinum L.): a review of biology, pathogenicity, and disease management. Aust J Agric Res. (2005) 56:317–32. doi: 10.1071/AR04143
3. Jukanti, AK, Gaur, PM, Gowda, C, and Chibbar, RN. Nutritional quality and health benefits of chickpea (Cicer arietinum L.): a review. Br J Nutr. (2012) 108:S11–26. doi: 10.1017/S0007114512000797
4. FAOSTAT . Top 20 countries production of chick peas. (2020). Available at: www.fao.org/home/en.
5. Kerem, Z, Lev-Yadun, S, Gopher, A, Weinberg, P, and Abbo, S. Chickpea domestication in the Neolithic Levant through the nutritional perspective. J Archaeol Sci. (2007) 34:1289–93. doi: 10.1016/j.jas.2006.10.025
6. Bhagyawant, SS, Narvekar, DT, Gupta, N, Bhadkaria, A, Gautam, AK, and Srivastava, N. Chickpea (Cicer arietinum L.) lectin exhibit inhibition of ace-I, α-amylase and α-glucosidase activity. Protein Pept Lett. (2019) 26:494–501. doi: 10.2174/0929866526666190327130037
7. Summo, C, De Angelis, D, Ricciardi, L, Caponio, F, Lotti, C, Pavan, S, et al. Nutritional, physico-chemical and functional characterization of a global chickpea collection. J Food Compos Anal. (2019) 84:103306. doi: 10.1016/j.jfca.2019.103306
8. Zhang, T, Li, Y, Miao, M, and Jiang, B. Purification and characterisation of a new antioxidant peptide from chickpea (Cicer arietium L.) protein hydrolysates. Food Chem. (2011) 128:28–33. doi: 10.1016/j.foodchem.2011.02.072
9. Ipekesen, S, Basdemir, F, Tunc, M, and Bicer, BT. Minerals, vitamins, protein and amino acids in wild Cicer species and pure line chickpea genotypes selected from a local population. J Elem. (2022) 27:127–40.
10. Kaur, K, Grewal, SK, Gill, PS, and Singh, S. Comparison of cultivated and wild chickpea genotypes for nutritional quality and antioxidant potential. J Food Sci Technol. (2019) 56:1864–76. doi: 10.1007/s13197-019-03646-4
11. Iqbal, A, Khalil, IA, Ateeq, N, and Khan, MS. Nutritional quality of important food legumes. Food Chem. (2006) 97:331–5. doi: 10.1016/j.foodchem.2005.05.011
12. Rachwa-Rosiak, D, Nebesny, E, and Budryn, G. Chickpeas—composition, nutritional value, health benefits, application to bread and snacks: a review. Crit Rev Food Sci Nutr. (2015) 55:1137–45. doi: 10.1080/10408398.2012.687418
13. Shah, W . Domestic processing effects on some insoluble dietary fibre components of various food legumes. Food Chem. (2004) 87:613–7.
14. Horax, R, Hettiarachchy, N, Chen, P, and Jalaluddin, M. Preparation and characterization of protein isolate from cowpea (Vigna unguiculata L. Walp.). J Food Sci. (2004) 69:fct114–8.
15. Aguilera, Y, Martín-Cabrejas, MA, Benítez, V, Mollá, E, López-Andréu, FJ, and Esteban, RM. Changes in carbohydrate fraction during dehydration process of common legumes. J Food Compos Anal. (2009) 22:678–83. doi: 10.1016/j.jfca.2009.02.012
16. You, A . Dietary guidelines for Americans, vol. 7. Washington, D.C: US Department of Health and Human Services and US Department of Agriculture (2015).
17. Wang, N, and Daun, JK. Effects of variety and crude protein content on nutrients and anti-nutrients in lentils (Lens culinaris). Food Chem. (2006) 95:493–502. doi: 10.1016/j.foodchem.2005.02.001
18. Rubio, LA, Perez, A, Ruiz, R, Guzmán, MÁ, Aranda-Olmedo, I, and Clemente, A. Characterization of pea (Pisum sativum) seed protein fractions. J Sci Food Agric. (2014) 94:280–7. doi: 10.1002/jsfa.6250
19. Gaur, PM, Singh, MK, Samineni, S, Sajja, SB, Jukanti, AK, Kamatam, S, et al. Inheritance of protein content and its relationships with seed size, grain yield and other traits in chickpea. Euphytica. (2016) 209:253–60. doi: 10.1007/s10681-016-1678-2
20. Singh, N, Kaur, S, Isono, N, and Noda, T. Genotypic diversity in physico-chemical, pasting and gel textural properties of chickpea (Cicer arietinum L.). Food Chem. (2010) 122:65–73. doi: 10.1016/j.foodchem.2010.02.015
21. Kou, X, Gao, J, Xue, Z, Zhang, Z, Wang, H, and Wang, X. Purification and identification of antioxidant peptides from chickpea (Cicer arietinum L.) albumin hydrolysates. LWT Food Sci Technol. (2013) 50:591–8. doi: 10.1016/j.lwt.2012.08.002
22. Hall, C, Hillen, C, and Garden Robinson, J. Composition, nutritional value, and health benefits of pulses. Cereal Chem. (2017) 94:11–31. doi: 10.1094/CCHEM-03-16-0069-FI
23. Da Silva, M, Neves, V, and Lourenço, E. Protein fractions and major globulin from chickpea (Cicer arietinum L.). Alimentose Nutriçăo. (2001) 12:131–49.
24. Singh, U, and Jambunathan, R. Studies on desi and kabull chickpea (Cicer arietinum L.) cultivars: levels of protease inhibitors, levels of polyphenolic compounds and in vitro protein digestibility. J Food Sci. (1981) 46:1364–7. doi: 10.1111/j.1365-2621.1981.tb04176.x
25. Khattak, AB, Zeb, A, and Bibi, N. Impact of germination time and type of illumination on carotenoidcontent, protein solubility and in vitro protein digestibility of chickpea (Cicer arietinum L.) sprouts. Food Chem. (2008) 109:797–801. doi: 10.1016/j.foodchem.2008.01.046
26. Chitra, U, Vimala, V, Singh, U, and Geervani, P. Variability in phytic acid content and protein digestibility of grain legumes. Plant Foods Hum Nutr. (1995) 47:163–72. doi: 10.1007/BF01089266
27. Han, IH, Swanson, BG, and Baik, BK. Protein digestibility of selected legumes treated with ultrasound and high hydrostatic pressure during soaking. Cereal Chem. (2007) 84:518–21. doi: 10.1094/CCHEM-84-5-0518
28. Angulo-Bejarano, PI, Verdugo-Montoya, NM, Cuevas-Rodríguez, EO, Milán-Carrillo, J, Mora-Escobedo, R, Lopez-Valenzuela, JA, et al. Tempeh flour from chickpea (Cicer arietinum L.) nutritional and physicochemical properties. Food Chem. (2008) 106:106–12. doi: 10.1016/j.foodchem.2007.05.049
29. Zia-Ul-Haq, M, Iqbal, S, Ahmad, S, Imran, M, Niaz, A, and Bhanger, M. Nutritional and compositional study of desi chickpea (Cicer arietinum L.) cultivars grown in Punjab, Pakistan. Food Chem. (2007) 105:1357–63. doi: 10.1016/j.foodchem.2007.05.004
30. Kesli, Y, and Adak, MS. Effects of different harvest time and sulfur fertilization on amino acid composition of lentil. J Plant Nutr. (2012) 35:1693–704. doi: 10.1080/01904167.2012.698350
31. Barbana, C, and Boye, JI. Angiotensin I-converting enzyme inhibitory activity of chickpea and pea protein hydrolysates. Food Res Int. (2010) 43:1642–9. doi: 10.1016/j.foodres.2010.05.003
32. Torres-Fuentes, C, Alaiz, M, and Vioque, J. Affinity purification and characterisation of chelating peptides from chickpea protein hydrolysates. Food Chem. (2011) 129:485–90. doi: 10.1016/j.foodchem.2011.04.103
33. Wallace, TC, Murray, R, and Zelman, KM. The nutritional value and health benefits of chickpeas and hummus. Nutrients. (2016) 8:766. doi: 10.3390/nu8120766
34. Ahmmed, T, Rahman, A, Salma, U, Akter, Z, Ansary, MMU, Khalil, MI, et al. Nutritional, phytochemicals and antioxidant properties of some popular pulse varieties of Bangladesh. J Agric Chem Environ. (2020) 9:343–68.
35. Ghribi, AM, Sila, A, Przybylski, R, Nedjar-Arroume, N, Makhlouf, I, Blecker, C, et al. Purification and identification of novel antioxidant peptides from enzymatic hydrolysate of chickpea (Cicer arietinum L.) protein concentrate. J Funct Foods. (2015) 12:516–25. doi: 10.1016/j.jff.2014.12.011
36. Alajaji, SA, and El-Adawy, TA. Nutritional composition of chickpea (Cicer arietinum L.) as affected by microwave cooking and other traditional cooking methods. J Food Compos Anal. (2006) 19:806–12. doi: 10.1016/j.jfca.2006.03.015
37. Simpson, HL, and Campbell, BJ. Dietary fibre–microbiota interactions. Aliment Pharmacol Ther. (2015) 42:158–79. doi: 10.1111/apt.13248
38. Tosh, SM, and Yada, S. Dietary fibres in pulse seeds and fractions: characterization, functional attributes, and applications. Food Res Int. (2010) 43:450–60. doi: 10.1016/j.foodres.2009.09.005
39. Rincón, F, Martínez, B, and Ibáñez, MV. Proximate composition and antinutritive substances in chickpea (Cicer arietinum L) as affected by the biotype factor. J Sci Food Agric. (1998) 78:382–8. doi: 10.1002/(SICI)1097-0010(199811)78:3<382::AID-JSFA128>3.0.CO;2-J
40. Sreerama, YN, Neelam, DA, Sashikala, VB, and Pratape, VM. Distribution of nutrients and antinutrients in milled fractions of chickpea and horse gram: seed coat phenolics and their distinct modes of enzyme inhibition. J Agric Food Chem. (2010) 58:4322–30. doi: 10.1021/jf903101k
41. Fan, PH, Zang, MT, and Xing, J. Oligosaccharides composition in eight food legumes species as detected by high-resolution mass spectrometry. J Sci Food Agric. (2015) 95:2228–36. doi: 10.1002/jsfa.6940
42. Fouad, AA, and Rehab, F. Effect of germination time on proximate analysis, bioactive compounds and antioxidant activity of lentil (Lens culinaris Medik.) sprouts. Acta Sci Pol Technol Aliment. (2015) 14:233–46. doi: 10.17306/J.AFS.2015.3.25
43. Sánchez-Mata, MC, Peñuela-Teruel, MJ, Cámara-Hurtado, M, Díez-Marqués, C, and Torija-Isasa, ME. Determination of mono-, di-, and oligosaccharides in legumes by high-performance liquid chromatography using an amino-bonded silica column. J Agric Food Chem. (1998) 46:3648–52. doi: 10.1021/jf980127w
44. Wang, N, and Daun, JK. Effect of variety and crude protein content on nutrients and certain antinutrients in field peas (Pisum sativum). J Sci Food Agric. (2004) 84:1021–9. doi: 10.1002/jsfa.1742
45. Åman, P . Carbohydrates in raw and germinated seeds from mung bean and chick pea. J Sci Food Agric. (1979) 30:869–75. doi: 10.1002/jsfa.2740300907
46. Yoshida, H, Tomiyama, Y, Kita, S, and Mizushina, Y. Lipid classes, fatty acid composition and triacylglycerol molecular species of kidney beans (Phaseolus vulgaris L.). Eur J Lipid Sci Technol. (2005) 107:307–15. doi: 10.1002/ejlt.200401078
47. Baker, B, Papaconstantinou, J, Cross, C, and Khan, N. Protein and lipid constitution of some Pakistani pulses. J Sci Food Agric. (1961) 12:205–7. doi: 10.1002/jsfa.2740120308
48. Ray, H, Bett, K, Tar'an, B, Vandenberg, A, Thavarajah, D, and Warkentin, T. Mineral micronutrient content of cultivars of field pea, chickpea, common bean, and lentil grown in Saskatchewan, Canada. Crop Sci. (2014) 54:1698–708. doi: 10.2135/cropsci2013.08.0568
49. Wang, N, Hatcher, D, Toews, R, and Gawalko, E. Influence of cooking and dehulling on nutritional composition of several varieties of lentils (Lens culinaris). LWT Food Sci Technol. (2009) 42:842–8. doi: 10.1016/j.lwt.2008.10.007
50. Rao, R, Costa, A, Croy, R, Boulter, D, and Gatehouse, J. Variation in polypeptides of the major albumin protein of pea (Pisum sativum L.): inheritance and molecular analysis. Mol Gen Genet MGG. (1989) 219:277–81. doi: 10.1007/BF00261188
51. Ashokkumar, K, Diapari, M, Jha, AB, Tar’an, B, Arganosa, G, and Warkentin, TD. Genetic diversity of nutritionally important carotenoids in 94 pea and 121 chickpea accessions. J Food Compos Anal. (2015) 43:49–60. doi: 10.1016/j.jfca.2015.04.014
52. Sen Gupta, D, Thavarajah, D, Knutson, P, Thavarajah, P, McGee, RJ, Coyne, CJ, et al. Lentils (Lens culinaris L.), a rich source of folates. J Agric Food Chem. (2013) 61:7794–9. doi: 10.1021/jf401891p
53. Hefni, M, and Witthöft, CM. Folate content in processed legume foods commonly consumed in Egypt. LWT Food Sci Technol. (2014) 57:337–43. doi: 10.1016/j.lwt.2013.12.026
54. Erbaş, M, Certel, M, and Uslu, M. Some chemical properties of white lupin seeds (Lupinus albus L.). Food Chem. (2005) 89:341–5. doi: 10.1016/j.foodchem.2004.02.040
55. Chavan, J, Kadam, S, Salunkhe, D, and Beuchat, LR. Biochemistry and technology of chickpea (Cicer arietinum L.) seeds. Crit Rev Food Sci Nutr. (1987) 25:107–58. doi: 10.1080/10408398709527449
56. Ciftci, H, Ozkaya, A, Cevrimli, BS, and Bakoglu, A. Levels of fat-soluble vitamins in some foods. Asian J Chem. (2010) 22:1251–6.
57. Langyan, S., Yadava, P., Khan, F. N., Bhardwaj, R., Tripathi, K., Bhardwaj, V., et al. (2022). Nutritional and food composition survey of major pulses toward healthy, sustainable, and biofortified diets. Frontiers in Sustainable Food Systems, 6, 878269.
58. Margier, M., George, S., Hafnaoui, N., Remond, D., Nowicki, M., Du Chaffault, L., et al. (2018). Nutritional composition and bioactive content of legumes: Characterization of pulses frequently consumed in France and effect of the cooking method. Nutrients, 10, 1668.
59. Yegrem, L., Mengestu, D., Legesse, O., Abebe, W., and Girma, N. (2022). Nutritional compositions and functional properties of New Ethiopian chickpea varieties: Effects of variety, grown environment and season. International Journal of Food Properties, 25, 1485–1497.
60. Segev, A, Badani, H, Kapulnik, Y, Shomer, I, Oren-Shamir, M, and Galili, S. Determination of polyphenols, flavonoids, and antioxidant capacity in colored chickpea (Cicer arietinum L.). J Food Sci. (2010) 75:S115–9. doi: 10.1111/j.1750-3841.2009.01477.x
61. Fares, C, Suriano, S, Codianni, P, Marciello, U, Russo, M, and Menga, V. Phytochemical profile of chickpea cultivars grown in conventional and organic farms in Southern, Italy. Org Agric. (2021) 11:589–600. doi: 10.1007/s13165-021-00365-z
62. Daliri, EB-M, Oh, DH, and Lee, BH. Bioactive peptides. Foods. (2017) 6:32. doi: 10.3390/foods6050032
63. Sarmadi, BH, and Ismail, A. Antioxidative peptides from food proteins: a review. Peptides. (2010) 31:1949–56. doi: 10.1016/j.peptides.2010.06.020
64. Real Hernandez, LM, and Gonzalez De Mejia, E. Enzymatic production, bioactivity, and bitterness of chickpea (Cicer arietinum) peptides. Compr Rev Food Sci Food Saf. (2019) 18:1913–46. doi: 10.1111/1541-4337.12504
65. Matemu, A, Nakamura, S, and Katayama, S. Health benefits of antioxidative peptides derived from legume proteins with a high amino acid score. Antioxidants. (2021) 10:316. doi: 10.3390/antiox10020316
66. Rajapakse, N, Mendis, E, Jung, W-K, Je, J-Y, and Kim, S-K. Purification of a radical scavenging peptide from fermented mussel sauce and its antioxidant properties. Food Res Int. (2005) 38:175–82. doi: 10.1016/j.foodres.2004.10.002
67. Álvarez, C, Rendueles, M, and Díaz, M. Alkaline hydrolysis of porcine blood haemoglobin: applications for peptide and amino acid production. Anim Prod Sci. (2012) 53:121–8.
68. Peñta-Ramos, E, and Xiong, Y. Antioxidant activity of soy protein hydrolysates in a liposomal system. J Food Sci. (2002) 67:2952–6. doi: 10.1111/j.1365-2621.2002.tb08844.x
69. Tacias-Pascacio, VG, Morellon-Sterling, R, Siar, E-H, Tavano, O, Berenguer-Murcia, A, and Fernandez-Lafuente, R. Use of Alcalase in the production of bioactive peptides: a review. Int J Biol Macromol. (2020) 165:2143–96. doi: 10.1016/j.ijbiomac.2020.10.060
70. Qian, Z-J, Jung, W-K, and Kim, S-K. Free radical scavenging activity of a novel antioxidative peptide purified from hydrolysate of bullfrog skin, Rana catesbeiana Shaw. Bioresour Technol. (2008) 99:1690–8. doi: 10.1016/j.biortech.2007.04.005
71. Jang, HL, Liceaga, AM, and Yoon, KY. Purification, characterisation and stability of an antioxidant peptide derived from sandfish (Arctoscopus japonicus) protein hydrolysates. J Funct Foods. (2016) 20:433–42. doi: 10.1016/j.jff.2015.11.020
72. Chi, C-F, Hu, F-Y, Wang, B, Ren, X-J, Deng, S-G, and Wu, C-W. Purification and characterization of three antioxidant peptides from protein hydrolyzate of croceine croaker (Pseudosciaena crocea) muscle. Food Chem. (2015) 168:662–7. doi: 10.1016/j.foodchem.2014.07.117
73. Nwachukwu, ID, and Aluko, RE. Structural and functional properties of food protein-derived antioxidant peptides. J Food Biochem. (2019) 43:e12761. doi: 10.1111/jfbc.12761
74. Karaś, M, Baraniak, B, Rybczyńska, K, Gmiński, J, Gaweł-Bęben, K, and Jakubczyk, A. The influence of heat treatment of chickpea seeds on antioxidant and fibroblast growth-stimulating activity of peptide fractions obtained from proteins digested under simulated gastrointestinal conditions. Int J Food Sci Technol. (2015) 50:2097–103. doi: 10.1111/ijfs.12872
75. Wali, A, Mijiti, Y, Yanhua, G, Yili, A, Aisa, HA, and Kawuli, A. Isolation and identification of a novel antioxidant peptide from chickpea (Cicer arietinum L.) sprout protein hydrolysates. Int J Pept Res Ther. (2021) 27:219–27. doi: 10.1007/s10989-020-10070-2
76. Li, Y, Jiang, B, Zhang, T, Mu, W, and Liu, J. Antioxidant and free radical-scavenging activities of chickpea protein hydrolysate (Cph). Food Chem. (2008) 106:444–50. doi: 10.1016/j.foodchem.2007.04.067
77. Torres-Fuentes, C, Del Mar Contreras, M, Recio, I, Alaiz, M, and Vioque, J. Identification and characterization of antioxidant peptides from chickpea protein hydrolysates. Food Chem. (2015) 180:194–202. doi: 10.1016/j.foodchem.2015.02.046
78. Gupta, N, and Bhagyawant, SS. Impact of hydrolysis on functional properties, antioxidant, ace-I inhibitory and antiproliferative activity of Cicer arietinum and Cicer reticulatum hydrolysates. Forum Nutr. (2019) 44:5. doi: 10.1186/s41110-019-0095-4
79. Quintero-Soto, MF, Chávez-Ontiveros, J, Garzón-Tiznado, JA, Salazar-Salas, NY, Pineda-Hidalgo, KV, Delgado-Vargas, F, et al. Characterization of peptides with antioxidant activity and antidiabetic potential obtained from chickpea (Cicer arietinum L.) protein hydrolyzates. J Food Sci. (2021) 86:2962–77. doi: 10.1111/1750-3841.15778
80. Guo, Y, Zhang, T, Jiang, B, Miao, M, and Mu, W. The effects of an antioxidative pentapeptide derived from chickpea protein hydrolysates on oxidative stress in Caco-2 and Ht-29 cell lines. J Funct Foods. (2014) 7:719–26. doi: 10.1016/j.jff.2013.12.013
81. Zhang, Q, Tong, X, Li, Y, Wang, H, Wang, Z, Qi, B, et al. Purification and characterization of antioxidant peptides from alcalase-hydrolyzed soybean (Glycine max L.) hydrolysate and their cytoprotective effects in human intestinal Caco-2 cells. J Agric Food Chem. (2019) 67:5772–81. doi: 10.1021/acs.jafc.9b01235
82. Sánchez, A, and Vázquez, A. Bioactive peptides: a review. Food Quality Safety. (2017) 1:29–46. doi: 10.1093/fqs/fyx0006
83. Gupta, RK, Gupta, K, Sharma, A, Das, M, Ansari, IA, and Dwivedi, PD. Health risks and benefits of chickpea (Cicer arietinum) consumption. J Agric Food Chem. (2017) 65:6–22. doi: 10.1021/acs.jafc.6b02629
84. Chandrasekaran, S, Luna-Vital, D, and De Mejia, EG. Identification and comparison of peptides from chickpea protein hydrolysates using either bromelain or gastrointestinal enzymes and their relationship with markers of type 2 diabetes and bitterness. Nutrients. (2020) 12:3843. doi: 10.3390/nu12123843
85. Mcmacken, M, and Shah, S. A plant-based diet for the prevention and treatment of type 2 diabetes. J Geriatric Cardiol. (2017) 14:342. doi: 10.11909/j.issn.1671-5411.2017.05.009
87. Bommer, C, Sagalova, V, Heesemann, E, Manne-Goehler, J, Atun, R, Bärnighausen, T, et al. Global economic burden of diabetes in adults: projections from 2015 to 2030. Diabetes Care. (2018) 41:963–70. doi: 10.2337/dc17-1962
88. Artasensi, A, Pedretti, A, Vistoli, G, and Fumagalli, L. Type 2 diabetes mellitus: a review of multi-target drugs. Molecules. (2020) 25:1987. doi: 10.3390/molecules25081987
89. Usman, B, Sharma, N, Satija, S, Mehta, M, Vyas, M, Khatik, GL, et al. Recent developments in alpha-glucosidase inhibitors for management of type-2 diabetes: an update. Curr Pharm Des. (2019) 25:2510–25. doi: 10.2174/1381612825666190717104547
90. Osorio-Diaz, P, Agama-Acevedo, E, Mendoza-Vinalay, M, Tovar, J, and Bello-Perez, LA. Pasta added with chickpea flour: chemical composition, in vitro starch digestibility and predicted glycemic index pasta Adicionada con Harina De garbanzo: Composición Química, Digestibilidad in vitro Del Almidóny Predicción Del Índice Glucémico. Cyta J Food. (2008) 6:6–12.
91. Chandrasekaran, S, and De Mejia, EG. Optimization, identification, and comparison of peptides from germinated chickpea (Cicer arietinum) protein hydrolysates using either papain or ficin and their relationship with markers of type 2 diabetes. Food Chem. (2022) 374:131717. doi: 10.1016/j.foodchem.2021.131717
92. Martínez, KAA, and Mejia, EG. Comparison of five chickpea varieties, optimization of hydrolysates production and evaluation of biomarkers for type 2 diabetes. Food Res Int. (2021) 147:110572. doi: 10.1016/j.foodres.2021.110572
93. Messerli, FH, Bangalore, S, Bavishi, C, and Rimoldi, SF. Angiotensin-converting enzyme inhibitors in hypertension: to use or not to use? J Am Coll Cardiol. (2018) 71:1474–82. doi: 10.1016/j.jacc.2018.01.058
94. Hicks, B, Filion, K, Yin, H, Sakr, L, Udell, J, and Azoulay, L. Angiotensin converting enzyme inhibitors and risk of lung cancer: population based cohort study. BMJ. (2018) 363:k4209. doi: 10.1136/bmj.k4209
95. Balgir, PP, Kaur, T, and Sharma, M. Antihypertensive peptides derived from food sources. MOJ Food Process Technol. (2016) 2:1–6. doi: 10.15406/mojfpt.2016.02.00024
96. Pedroche, J, Yust, MM, Girón-Calle, J, Alaiz, M, Millán, F, and Vioque, J. Utilisation of chickpea protein isolates for production of peptides with angiotensin I-converting enzyme (ace)-inhibitory activity. J Sci Food Agric. (2002) 82:960–5. doi: 10.1002/jsfa.1126
97. Yust, MAM, Pedroche, J, Giron-Calle, J, Alaiz, M, Millán, F, and Vioque, J. Production of ace inhibitory peptides by digestion of chickpea legumin with alcalase. Food Chem. (2003) 81:363–9. doi: 10.1016/S0308-8146(02)00431-4
98. Jamdar, SN, Deshpande, R, and Marathe, SA. Effect of processing conditions and in vitro protein digestion on bioactive potentials of commonly consumed legumes. Food Biosci. (2017) 20:1–11. doi: 10.1016/j.fbio.2017.07.007
99. Medina-Godoy, S, Ambriz-Pérez, DL, Fuentes-Gutiérrez, CI, Germán-Báez, LJ, Gutiérrez-Dorado, R, Reyes-Moreno, C, et al. Angiotensin-converting enzyme inhibitory and antioxidative activities and functional characterization of protein hydrolysates of hard-to-cook chickpeas. J Sci Food Agric. (2012) 92:1974–81. doi: 10.1002/jsfa.5570
100. Sánchez-Chino, XM, Jiménez Martínez, C, León-Espinosa, EB, Garduño-Siciliano, L, Álvarez-González, I, Madrigal-Bujaidar, E, et al. Protective effect of chickpea protein hydrolysates on colon carcinogenesis associated with a hypercaloric diet. J Am Coll Nutr. (2019) 38:162–70.
101. Guzińska-Ustymowicz, K, Pryczynicz, A, Kemona, A, and Czy Żewska, J. Correlation between proliferation markers: Pcna, Ki-67, Mcm-2 and antiapoptotic protein Bcl-2 in colorectal cancer. Anticancer Res. (2009) 29:3049–52.
102. Azzeh, FS, Alshammari, EM, Alazzeh, AY, Jazar, AS, Dabbour, IR, El-Taani, HA, et al. Healthy dietary patterns decrease the risk of colorectal cancer in the Mecca region, Saudi Arabia: a case-control study. BMC Public Health. (2017) 17:607. doi: 10.1186/s12889-017-4520-4
103. Osman, A, Goda, HA, Abdel-Hamid, M, Badran, SM, and Otte, J. Antibacterial peptides generated by Alcalase hydrolysis of goat whey. LWT Food Sci Technol. (2016) 65:480–6. doi: 10.1016/j.lwt.2015.08.043
104. Najafian, L, and Babji, A. A review of fish-derived antioxidant and antimicrobial peptides: their production, assessment, and applications. Peptides. (2012) 33:178–85. doi: 10.1016/j.peptides.2011.11.013
105. Song, W, Kong, X, Hua, Y, Chen, Y, Zhang, C, and Chen, Y. Identification of antibacterial peptides generated from enzymatic hydrolysis of cottonseed proteins. LWT. (2020) 125:109199. doi: 10.1016/j.lwt.2020.109199
106. Kan, A, Özçelik, B, Kartal, M, Özdemir, Z, and Özgen, S. In vitro antimicrobial activities of Cicer arietinum L (chickpea). Trop J Pharm Res. (2010) 9. doi: 10.4314/tjpr.v9i5.68386
107. Faridy, J-CM, Stephanie, C-GM, Gabriela, M-MO, and Cristian, J-M. Biological activities of chickpea in human health (Cicer arietinum L.). a review. Plant Foods Hum Nutr. (2020) 75:142–53. doi: 10.1007/s11130-020-00814-2
108. Hassan, LEA, Dahham, SS, Fadul, SM, Umar, MI, Majid, ASA, Khaw, KY, et al. Evaluation of in vitro and in vivo anti-inflammatory effects of (−)-pseudosemiglabrin, a major phytoconstituent isolated from Tephrosia apollinea (Delile) Dc. J Ethnopharmacol. (2016) 193:312–20. doi: 10.1016/j.jep.2016.08.023
109. Wahby, M, Mohammed, D, Newairy, A, Abdou, H, and Zaky, A. Aluminum-induced molecular neurodegeneration: the protective role of genistein and chickpea extract. Food Chem Toxicol. (2017) 107:57–67. doi: 10.1016/j.fct.2017.05.044
110. Milán-Noris, AK, Gutiérrez-Uribe, JA, Santacruz, A, Serna-Saldívar, SO, and Martínez-Villaluenga, C. Peptides and isoflavones in gastrointestinal digests contribute to the anti-inflammatory potential of cooked or germinated desi and kabuli chickpea (Cicer arietinum L.). Food Chem. (2018) 268:66–76. doi: 10.1016/j.foodchem.2018.06.068
111. Hao, X, Li, J, Shi, Q, Zhang, J, He, X, and Ma, H. Characterization of a novel legumin α-amylase inhibitor from chickpea (Cicer arietinum L.) seeds. Biosci Biotechnol Biochem. (2009) 73:1200–2.
112. Shi, W, Hou, T, Guo, D, and He, H. Evaluation of hypolipidemic peptide (Val-Phe-Val-Arg-Asn) virtual screened from chickpea peptides by pharmacophore model in high-fat diet-induced obese rat. J Funct Foods. (2019) 54:136–45. doi: 10.1016/j.jff.2019.01.001
113. Xue, Z, Gao, J, Zhang, Z, Yu, W, Wang, H, and Kou, X. Antihyperlipidemic and antitumor effects of chickpea albumin hydrolysate. Plant Foods Hum Nutr. (2012) 67:393–400. doi: 10.1007/s11130-012-0311-3
Keywords: chickpeas, bioactive compounds, antioxidant, anticancer, Cicer arietinum
Citation: Begum N, Khan QU, Liu LG, Li W, Liu D and Haq IU (2023) Nutritional composition, health benefits and bio-active compounds of chickpea (Cicer arietinum L.). Front. Nutr. 10:1218468. doi: 10.3389/fnut.2023.1218468
Edited by:
María Janeth Rodríguez-Roque, Autonomous University of Chihuahua, MexicoReviewed by:
Nirmal Mazumder, Manipal Academy of Higher Education, IndiaGi Hyung Ryu, Kongju National University, Republic of Korea
Copyright © 2023 Begum, Khan, Liu, Li, Liu and Haq. This is an open-access article distributed under the terms of the Creative Commons Attribution License (CC BY). The use, distribution or reproduction in other forums is permitted, provided the original author(s) and the copyright owner(s) are credited and that the original publication in this journal is cited, in accordance with accepted academic practice. No use, distribution or reproduction is permitted which does not comply with these terms.
*Correspondence: Dahai Liu, c2VhbnNlYW4yMDE0QDEyNi5jb20=