- 1Molecular Preventive Medicine, University Medical Center and Faculty of Medicine, University of Freiburg, Freiburg, Germany
- 2Institute of Food Chemistry, Hamburg School of Food Science, University of Hamburg, Hamburg, Germany
- 3Plant Quality and Food Security, Leibniz Institute of Vegetable and Ornamental Crops, Großbeeren, Germany
- 4Department of Public Health, Health Services Research and Health Technology Assessment, Institute of Public Health, Medical Decision Making and HTA, UMIT TIROL, University for Health Sciences and Health Technology, Hall in Tirol, Austria
- 5Institute of General Practice/Family Medicine, Faculty of Medicine and Medical Center, University of Freiburg, Freiburg, Germany
- 6Institute of Food Technology and Food Chemistry, Technische Universität Berlin, Berlin, Germany
Scope: As prostaglandin E2 (PGE2) has important roles in physiological and inflammatory functions, a double-blind randomized controlled crossover study to investigate the potential of nasturtium (Tropaeolum majus) for modulating PGE2 was conducted, aiming at clarifying the role of benzyl isothiocyanate (BITC). As secondary parameters leukotriene 4 (LTB4), and cytokine release (tumor necrosis factor alpha, TNF-α; interleukins IL-1β, IL-10, and IL-12) were quantified.
Methods and results: Thirty-four healthy female participants consumed 1.5 g nasturtium containing BITC, (verum) or no BITC (control) twice a day for 2 weeks each. Nasturtium intervention resulted in an increase in mean PGE2 levels in serum samples (verum: 1.76-fold, p ≤ 0.05; control: 1.78-fold, p ≤ 0.01), and ex vivo stimulated peripheral blood mononuclear cells (PBMC) (verum: 1.71-fold, p ≤ 0.01; control: 1.43-fold). Using a pre-to-post responder analysis approach, 18 of 34 subjects showed a > 25% PGE2 increase in serum, while it was >25% decreased for 9 subjects (stimulated PBMC: 14 and 8 of 28, respectively). Under the selected conditions, the BITC content of nasturtium did not affect the observed changes in PGE2. Verum intervention also increased mean LTB4 serum level (1.24-fold, p ≤ 0.01), but not in LPS stimulated PBMC, and significantly increased TNF-α release in stimulated PBMC after 3 h (verum: 1.65-fold, p = 0.0032; control: 1.22-fold, p = 0.7818). No change was seen in the anti-inflammatory cytokine IL-10, or the pro-inflammatory cytokines IL-1β, and IL-12.
Conclusion: In contrast to the previously reported in vitro results, on average, LPS activated PBMC and serum from both groups showed increased PGE2 levels. Further analyses suggest that PGE2 release after intervention could possibly depend on the baseline PGE2 level. Identification of phenotypes that respond differently to the nasturtium intervention could be useful to establish personalized approaches for dosing phytopharmaceuticals medicines.
1. Introduction
Nasturtium (Tropaeolum majus, T. majus, Indian cress) from the Brassicales plant order is widely used in culinary and as dietary supplement. The plant is also a component in phytopharmaceuticals for the prevention and treatment of urinary tract infections, and against acute sinusitis or bronchitis (1, 2). Nasturtium contains large amounts of the prodrug glucosinolate (GLS) glucotropaeolin (benzyl GLS), especially in the leaves, flowers and seeds (3). This compound breaks down to benzyl isothiocyanate (BITC) by enzymatic cleavage by the plant’s endogenous myrosinase when the cell matrix is destroyed. There is good evidence for the pharmacological efficacy of BITC from in vitro studies (4–6). However, there is a lack of clear evidence on the role of BITC in the health-promoting or therapeutic efficacy of nasturtium in humans. Besides BITC, nasturtium is also rich in polyphenols such as anthocyanins, which have also been described as anti-inflammatory or antioxidant (7, 8). In a previous study, we demonstrated a concentration-dependent inhibition of the cyclooxygenase (COX)/prostaglandin E2 (PGE2) signaling pathway in bacterial lipopolysaccharide (LPS) stimulated peripheral blood mononuclear cells (PBMC) from healthy human volunteers upon treatment with a water extract of nasturtium (8). PGE2 is one of the most abundant prostanoids in the human body. It mediates pathogenic mechanisms, including the inflammatory response, in which it contributes to the development of the cardinal signs of acute inflammation. Inhibition of COX and subsequent reduction in the generation of prostanoids is the most established mechanism of action of non-steroidal anti-inflammatory drugs (9). However, nowadays PGE2 is also known to sustain important homeostatic functions and is considered important for the resolution of inflammation (10–12).
In our recent in vitro study, heat inactivation of myrosinase prior to extraction did not abolish the inhibitory effect on PGE2, thus questioning the relevance of BITC in these experiments (8). In contrast, in a 5-day human randomized, controlled trial conducted with the plant Brassica carinata from the order Brassicales, we found a significant reduction in serum PGE2 levels after consumption of a plant powder drink containing allyl isothiocyanate (AITC) and not after drinking the preparation devoid of AITC (13). Based on these findings, the present study aimed to clarify whether the basic research results shown in vitro could be transferred in the same way to the situation in humans, when consuming nasturtium. Thus, we conducted a monocentric, randomized, doubleblind, controlled crossover trial in order to determine the effect of regular nasturtium intake on PGE2 levels as primary parameter. By using nasturtium containing preformed BITC, and also nasturtium with inactivated myrosinase from which BITC could not be enzymatically released, we aimed to clarify the role of BITC in acting on PGE2. Secondary parameters included the quantification of leukotriene 4 (LTB4) levels, and also cytokine release (tumor necrosis factor alpha, TNF-α, interleukins IL-1β, IL-10, and IL-12) from ex vivo LPS stimulated PBMC from healthy human volunteers.
2. Materials and methods
2.1. Chemicals
Fetal calf serum (FCS), L-glutamine and phosphate buffered saline (PBS, without Ca and Mg), penicillin–streptomycin (P/S) solution, L-glutamine solution, RPMI-1640 and penicillin/streptomycin solution (10,000 U/mL and 10,000 μg/mL) were purchased from Gibco™, Life Technologies GmbH (Darmstadt, Germany). LPS from Escherichia coli O11:B4, standard BSA solution (10 mg/mL), ammonium persulfate, methanol (>99%) and Tween 20 were from Sigma Aldrich (Taufkirchen, Germany), LymphoPrepTM gradient was purchased from Progen Biotechnik GmbH (Heidelberg, Germany). Colistin sulfate salt was purchased from Thermo Fisher Scientific Inc. (Waltham, Massachusetts, USA). Trifluoracetic acid (TFA, >99%) was from AppliChem GmbH (Darmstadt, Germany). Pronase E (from Streptomyces griseus) was obtained from Merck KGaA (Darmstadt, Germany). Sodium hydroxide, formic acid (FA; 98%), acetonitrile (LC–MS grade), methylene chloride (GC Ultra Grade), and water Rotisolv HPLC were purchased from Carl Roth GmbH+Co. KG (Karlsruhe, Germany). C18ec solid phase extraction cartridges (3 mL, 200 mg) were obtained from Machery-Nagel GmbH & Co. KG (Düren, Germany). Benzyl cyanide (≥98%), benzonitrile (≥99.9%), and BITC (≥98.5%) were obtained from Sigma-Aldrich Chemie GmbH (Steinheim, Germany) and Na2SO4 anhydrous (≥99%) was from VWR International GmbH (Darmstadt, Germany). β-Mercaptoethanol was obtained from Merck KGaA (Darmstadt Germany). Ethylene diamine tetraacetic acid (EDTA, 99%, p.a.) and sodium dodecyl sulfate (SDS, ≥99%) was from Serva GmbH (Heidelberg, Germany).
2.2. Study population and ethical approval
This study was conducted in accordance with the Declaration of Helsinki and approved by the Ethical Committee of the University of Freiburg (ethical vote number 322/18, 11/13/2018). This intervention trial was registered on German Clinical Trials Register (DRKS) with the ID: DRKS00016548. All participants provided written informed consent for study participation and received financial compensation approved by the ethics committee. A total of 46 healthy female subjects were recruited via posted flyers, social media, and personal communication in Freiburg, Germany. Subjects were screened for eligibility using a health questionnaire. As the immune system differs between men and women (14), the study was conducted with women only, so sex did not need to be considered as a confounding factor. The inclusion criteria for the study were as follows: age between 20 and 45 years, good health status and non-smoking. Exclusion criteria included: acute and chronic diseases, acute gastrointestinal ulcer, acute nephritis, pregnancy, breast feeding, intake of antibiotics (acute or during the last 3 months), severe allergies against vegetables of the order Brassicales and strong over- or underweight (BMI > 25 or < 18). Five subjects dropped out before randomization: two for health reasons (acute infection), and three had conflicting schedules with the planned study. During allocation, 7 participants dropped out: 6 due to acute illness and one due to conflicting schedules with the study. 34 participants completed the study. The flow diagram according to CONSORT guidelines for randomized trials is shown in Figure 1.
2.3. Nasturtium (T. majus) preparation
Standardized dried powder of T. majus flowers and leaves was provided by Repha GmbH (Langenhagen, Germany). A detailed phytochemical analysis of the plant powder was reported by us earlier (8). For consumption, every day subjects freshly prepared 1.5 g of plant powder plus a pinch of sugar (around 0.04 g), which was provided to them in a plastic medication cup with lid, by thoroughly suspending this mixture in 20 mL of drinking water and shaking for 20 s. Subsequently, the preparation was swallowed, with optional consumption of white bread. We used dried plant powder containing (1) the active enzyme myrosinase, which resulted in the formation of a defined amount BITC during the preparation step (verum preparation), and (2) containing heat-inactivated myrosinase, which did not produce ITC (control). In the verum 18.87 ± 4.5 μmol BITC and 2.33 ± 0.05 μmol benzyl nitrile (BCN) were detected, whereas the control contained 0.07 ± 0.06 μmol BITC and 4.22 ± 0.63 μmol BCN per serving. Prior to the intervention, aliquots of the plant powders were analyzed for the absence of relevant microbiological contamination following the guidelines of the German Society for Hygiene and Microbiology (DGHM, Münster, Germany) for dried products (herbs and spices) by Eurofins BioTesting Services Nord GmbH (Hamburg, Germany).
2.4. Study design and protocol
A monocentric, randomized, double-blind, controlled, crossover trial was conducted at the Medical Center of the University of Freiburg, Germany (for study design see Figure 2). The intervention was conducted between April and September 2019. Each subject participated in two 14-day intervention phases separated by a 14-day phase-out period during which subjects resumed their normal diet. Both intervention phases were preceded by a 7-day wash-out period. During the wash-out period and the intervention period, subjects were advised to not consume plants from the order Brassicales. A list of foods to avoid was provided. During each of the interventions the subjects consumed one serving of verum or control every morning and afternoon (with 12 h interval), optionally together with some white bread. The order of consumption (begin with verum or control) was assigned randomly using Microsoft® Excel using the RANDBETWEEN function 1;2. “1” was defined as “control” und “2” as “verum.” No certain block size was used and the randomization was done for each subject during enrollment by one unblinded investigator. The full intervention protocol can be viewed at the DRKS (DRKS00016548).
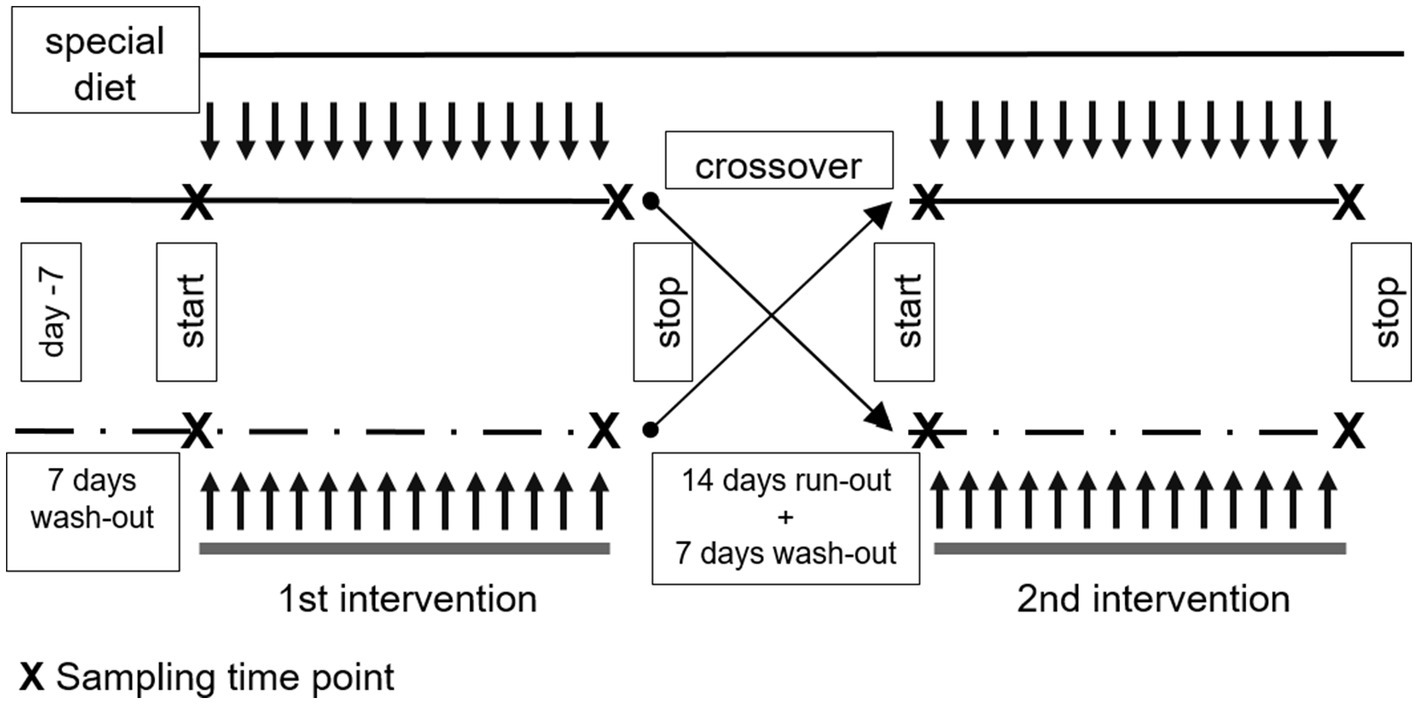
Figure 2. Study design, treatment and sampling time line. This study was conducted as a randomized, double-blind, controlled, crossover trial. Each subject participated in two 14-day intervention phases separated by a 14-day run-out phased during which subjects resumed their normal diet. Both intervention phases were preceded by a 7-day wash-out period. During the wash-out periods and the interventions period the consumption of plants from the order Brassicales was not allowed. During each of the intervention the subjects consumed prepatation of verum (containing active myrosinase) or control (containing no active myrosinase) every morning and afternoon. Before the first intake of nasturtium (verum or control) on day 1 and 14, the participants collect spot urine. Before the first intake and 4 h after the last intake, blood was taken.
2.5. Collection and preparation of blood and urine samples
On day one, subjects arrived fasting at the study center. They were asked to provide a spot urine sample, which was immediately placed on ice. Then, before intake of the first preparation, the initial blood samples were drawn into Li-Heparin vacutainers and serum vacutainers with coagulant (Sarstedt AG & Co. KG, Nümbrecht, Germany). Vacutainers for serum samples were placed on ice; Li-Heparin vacutainers for PBMC isolation were kept at room temperature. All samples were processed within 2 h after sampling. On day 14, 4 h after the last procedure in the morning, spot urine and blood were collected as on the first day. On day 14, no further intake took place in the evening. This schedule was chosen because the concentration of ITC metabolites in human serum reaches a maximum between 1 and 5 h after consumption (15).
Urine samples were stored in urine monovette (Sarstedt, Sarstedt AG & Co. KG, Nümbrecht, Germany) and reaction tubes at −80°C until analysis. Two 200 μL urine aliquots were mixed with 50 μL TFA for chemical analysis and stored at −80°C. Blood with coagulant was centrifuged at 4°C and 2000× g for 10 min to obtain serum, aliquoted in 200 or 300 μL portions, and stored at −80°C. One 300 μL aliquot was mixed with 100 μL TFA and stored at −80°C until chemical analysis.
2.6. Isolation and cultivation of human PBMC
PBMC were isolated from Li-heparinized blood by centrifugation on a LymphoPrep™ gradient using SepMate™ centrifugation tubes (Stemcell™ Technologies Germany GmbH, Cologne, Germany). Cells were washed twice with PBS, and cell viability as well as cell concentration were determined using the trypan blue exclusion test. Cell suspensions of 1 × 106cells/mL were prepared with RPMI, supplemented with 10% FCS, 1% Pen/Strep and glutamine. 1.5 mL of cell suspension was stimulated with 100 ng/mL LPS and incubated at 37°C in a humidified incubator with 5% CO2/95% air atmosphere for 3, 24, or 48 h. Subsequently, the cells were centrifuged at 500× g for 5 min at 4°C. Cell free supernatants were collected, and stored at −80°C. Cell pellets were washed twice with ice cold PBS and stored at −80°C.
2.7. Quantification of PGE2 and LTB4 release by ELISA assay
Cell free supernatants and serum samples were used for quantification of PGE2 and LTB4 using the ELISA kits from Cayman (Hamburg, Germany) according to the manufacturer’s instructions.
2.8. Quantification of IL-10, IL-1 β, IL-12, and TNF-α by ELISA assay
The quantification of cytokines was done using the human IL-10, IL-1β, IL12, and TNF-α uncoated ELISA kits from Invitrogen (Carlsbad, California, USA) following the manufacturer’s protocol.
2.9. Analysis of glucosinolates and their hydrolysis products in nasturtium
Nasturtium plant powder was analyzed for GLS and the potential to release GLS hydrolysis products. For analysis of the GLS hydrolysis products, 1.5 g of the plant powder was prepared as described in 2.3. After 20 s, 500 μL of the mixture were placed into a solvent resistant extraction vessel, which was placed on an ice-water bath. Then, samples were extracted at 0°C and analyzed by GC–MS as described previously (16), except that an Agilent J&W GC VF-5 ms column (30 m × 0.25 mm × 0.25 μM) linked to a 10 m EZ-Guard P/N:CP9013 guard column (both Agilent Technologies, Waldbronn, Germany) was used for separation and the transfer line was set to 270°C. GLS were analyzed from the dry powder in their desulfo-form as described before (17). Three aliquots of active and inactivated plant powder material were analyzed (n = 3) for GLS and hydrolysis product formation.
2.10. Preparation of samples for determination of mercapturic acid metabolites
Urine and serum samples were prepared for analysis as described before (18). Frozen samples were thawed, vortexed for 1 min and centrifuged at 20,854× g, 4°C for 10 min. The supernatant was transferred to SPE cartridges. Prior to transferring, the SPE cartridges were conditioned with 3 mL acetonitrile (ACN) and equilibrated with 3 mL water. After the application of the samples, cartridges were washed with 3 mL formic acid (FA, 0.1% in water) and the analytes were eluted with 3 mL of FA (0.1% in ACN:water, 90:10, v/v). Afterwards, the eluates were evaporated to dryness with a steady stream of nitrogen. The urine and serum samples were reconstituted in 200 μL and 100 μL FA (0.1% in ACN:water, 90:10, v/v), respectively. Aliquots of the samples (5 μL) were then injected into the LC-ESI-MS/MS system.
2.11. LC-ESI-MS/MS analysis of the mercapturic acid metabolites
The mercapturic acid metabolites (BITC-glutathione, BITC-GSH; BITC-cysteine, BITC-Cys; BITC-cysteineglycine, BITC-CysGly; BITC-N-acetylcysteine, BITC-NAC) were analysed as described before (15) with minor modifications. The LC-ESI-MS/MS analysis was performed on a 4000 QTrap triple quadrupole MS/MS system (AB Sciex Germany GmbH, Darmstadt, Germany) coupled with an Agilent 1,200 series HPLC system (Agilent Technologies Deutschland GmbH & Co. KG, Waldbronn, Germany). The Software Analyst 1.6.1 (AB Sciex Germany GmbH, Darmstadt, Germany) was used for data acquisition and processing. The separation was performed on a Phenomenex Kinetex C18 column (5 μm, 100 Å, 150 × 2.1 mm). The set temperature for the oven was 20°C and the autosampler temperature was 4°C. The mobile phase consisted of 0.1% FA in water (A) and 0.1% FA in ACN (B) with a constant flow of 300 μL/min. Starting with 80% of A which was held for 1 min before B was raised from 20 to 90% within 11 min and held for 4 min. To equilibrate the system A was raised from 10 to 90% within 1 min and held for 5 min. The MS analysis was done in the negative ionization mode, with an entrance potential of −10 V, a desolvation gas temperature of 450°C, ion spray potential of −4.5 kV, gas 1 and gas 2 at 46 psi and a curtain gas pressure at 10 psi. Quantitation was done by an external calibration curve in a concentration range between 0.01 and 50 μM. The quantity of metabolites in urine was expressed as nmol/mg creatinine.
2.12. Preparation of serum samples for determination of protein conjugates
The sample preparation of serum samples was conducted as described before with minor modifications (18). The samples were thawed, vortexed for 1 min and aliquotes of 200 μL were mixed with 500 μL of PBS buffer (pH 7.4). Afterwards, 200 μL of protease solution was added (10 mg/mL in PBS) for protein digestion. The samples were incubated overnight (15 h, 37°C) and the enzyme inactivated by adding 100 μL TFA. After vortexing for 1 min and centrifugation at 20,854× g, 4°C for 10 min, the supernatant was applied to prepared SPE cartridges. Preparation of the SPE cartridges was done by conditioning with 3 mL methanol and equilibrating with 3 mL water. After sample application, the cartridges were washed with 3 mL FA (0.1% in water) and the analytes were eluted with 3 mL FA (0.1% in MeOH:water, 90:10, v/v). Subsequently, the serum samples were evaporated to dryness under a gentle stream of nitrogen and redissolved in 100 μL FA (0.1% in MeOH-water, 90:10, v/v). Sample aliquotes of (4 μL) were injected into the LC-ESI-MS/MS system.
2.13. LC-ESI-MS/MS analysis of protein conjugates
For the LC-ESI-MS/MS analysis the protocol performed by Kühn and colleagues was used (18). The 4000 QTrap triple quadrupole MS/MS system (AB Sciex Germany GmbH, Darmstadt, Germany) was coupled with an Agilent 1200 series HPLC system (Agilent Technologies Deutschland GmbH & Co. KG, Waldbronn, Germany). Data acquisition and processing was done using the Software Analyst 1.6.1 (AB Sciex Germany GmbH, Darmstadt, Germany). For quantification, an external calibration with BITC-lysine (BITC-Lys) and BITC-Cys synthesized by Kühn et al. (18) was used in a concentration range from 0.01 to 4 μmol/L (18).
2.14. Quantification of creatinine
Creatinine was used as a normalization factor to estimate mercapturic acid metabolites in urine. Quantification of creatinine in urine samples was done using the ELISA kit from Invitrogen AG (Carlsbad, CA, USA) following the manufacturer’s protocol.
2.15. Statistical study design and analysis
The sample size calculation was based on the primary outcome, namely change in PGE2 serum level upon nasturtium intervention. According to this, detection of a 25% difference between groups in PGE2 level, measured by ELISA, with 80% power, using a 2-tailed t-test at the 1% significance level and 30% standard deviation, requires 34 participants. The calculation was based on the assumption of a log-normal distribution of the values. Based on an estimated drop-out-rate of 20%, 41 subjects were recruited for the trial. For both intervention phases, the between-subject difference in outcome parameters was calculated. The two-sided Student’s paired t-test or Wilcoxon signed-rank test (due to parameter distribution) was carried out. Normal distribution was assessed using the Kolmogorov–Smirnov test and Shapiro–Wilk test. To analyze for a potential carry-over effect between the verum and control intervention phase, a pre-test was performed by calculating the sum of the measured values in the intervention trial for each participant and compared across the two groups by an unpaired t-test (19). This was done for the primary parameter PGE2 in serum and LPS stimulated PBMC. Statistical significance was set to α ≤ 0.05. Furthermore, an exploratory responder analysis was carried out to see how the intervention affected the individual subject. Data analysis was performed using the statistical software package SAS, version 9.4 (SAS Inc., Cary, NC, USA) or GraphPad Prism 6.0 software (LaJolla, California, USA).
3. Results
3.1. Study population characteristics and side effects
A total of 34 healthy female participants completed the intervention trial. Seven individuals dropped out before allocation (Figure 1). Reasons were infection(s) (n = 6) and conflicting time schedules (n = 1). Individuals were aged 23.32 ± 2.56 years, with a body weight of 60.98 ± 5.19 kg, height of 1.69 ± 0.05 m and a BMI of 21.42 ± 1.79 kg/m2. Individuals starting with verum were aged 23.48 ± 2.62 years, with a body weight of 60.98 ± 6.13 kg, height of 1.69 ± 0.05 m and a BMI of 21.33 ± 1.97 kg/m2. Individuals starting with control were aged 23.08 ± 2.37 years, with a body weight of 61.12 ± 3.39 kg, height of 1.68 ± 0.05 m and a BMI of 21.57 ± 1.57 kg/m2. Participants reported mainly mild side effects, which are given in Figure 3. As an example, six subjects in the BITC-containing nasturtium intervention group reported nausea, while this was two in the control group (nasturtium without preformed BITC). For flatulence, it was four and nine, respectively.
3.2. Traceability of BITC metabolites and BITC adducts in serum and urine
BITC metabolites from the mercapturic acid pathway were detected in urine and serum samples of subjects before and after both intervention phases. As shown in Figures 4A,B, no BITC metabolites could be detected in all urine and serum samples before intervention, which confirmed the efficacy of the wash-out and compliance of the subjects to the study protocol. BITC-NAC was detected in urine samples of subjects from the verum group at a mean level of 28.36 ± 14.12 nmol/mg creatinine, and from the control group at 5.36 ± 4.53 nmol/mg creatinine (Figure 4A). BITC-NAC was detected in serum samples of subjects from verum group at a level of 43.91 ± 44.58 nmol/mg, and from control group at 0.58 ± 1.5 nmol/mg (Figure 4B). Additional to BITC-NAC, other metabolites from the mercapturic acid pathway could be detected in serum samples of the verum group, albeit at lower levels than BITC-NAC (Figure 4B).
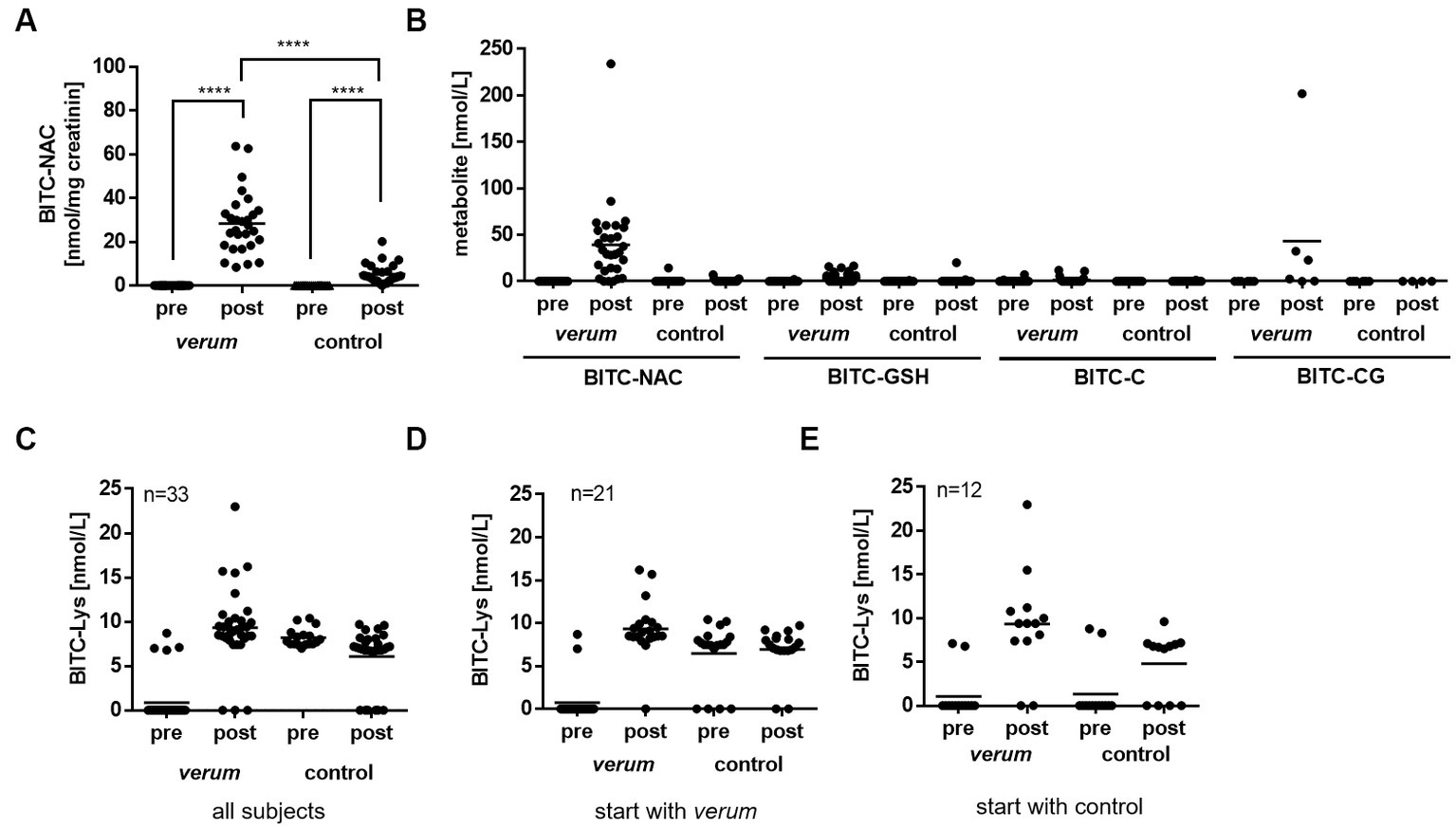
Figure 4. Detection of BITC metabolites and protein conjugates in serum and urine of participants using LC-ESI-MS/MS analysis. BITC-NAC was quantified in urine (A) and serum samples (B) from subjects before (pre) and after (post) of consumption of verum or control. (C) BITC-Lys was quantified in serum samples from subjects before (pre) and after (post) from intervention phases verum and control. (D,E) Concentration of BITC-Lys in serum samples dependent on which preparation was first consumed: verum (D) or control (E). Each dot represents results from one participant tested and the line represents the mean. Asterisk indicates statistically difference. ****p < 0.0001. BITC, benzyl isothiocyanate; NAC, N-acetylcystein; GSH, glutathione; C, cysteine; CysGly, cysteinylglycine; Lys, lysine.
BITC-Lys protein adducts could be detected in serum samples in both intervention groups (verum and control, Figure 4C). While in serum samples from only four subjects of the verum group BITC-Lys adducts were found before intervention start (mean of 7.4 nmol/L ± 0.88), this was the case in 17 subjects from the control group (mean 7.76 nmol/L ± 2.18), Figure 4C. In contrast to the mercapturic acid metabolites, ITC protein adducts seem to have a relatively long half-life (20). Therefore, it was possible that the measured adducts in the control group resulted from a previous verum intervention. We thus re-evaluated these data according to the sequence of the start of the intervention, i.e., verum or control (Figures 4D,E) and found that 15 of the 17 positive samples had in fact been preceded by a verum intervention.
3.3. Influence of nasturtium intervention on COX/PGE2 pathway activation
First, we analyzed the magnitude of difference in outcome in the verum and control group in terms of carrying out a pre to post analysis (Figure 5A). Intervention with verum significantly increased the mean PGE2 concentration in serum by 1.76-fold (p ≤ 0.05). This was comparable to the control (1.78-fold, p ≤ 0.01), which indicated an independence of the effect from the BITC content of the plant. Next, we investigated the effect of the intervention on PGE2 release in LPS stimulated PBMC in both phases to simulate an acute bacterial infection. Bacterial LPS is commonly used in such experiments to mimic inflammatory responses in cell and animal studies. After intervention, PGE2 release from 24 h stimulated PBMC increased to a mean of 1.71-fold (verum, p ≤ 0.01), and 1.43-fold (control), see Figure 5B. After 48 h of stimulation, PGE2 release was still upregulated upon intervention (verum: 1.49-fold, control: 1.43-fold). As seen for the serum PGE2 analysis, no significant superior treatment effect of verum compared to control was observed.
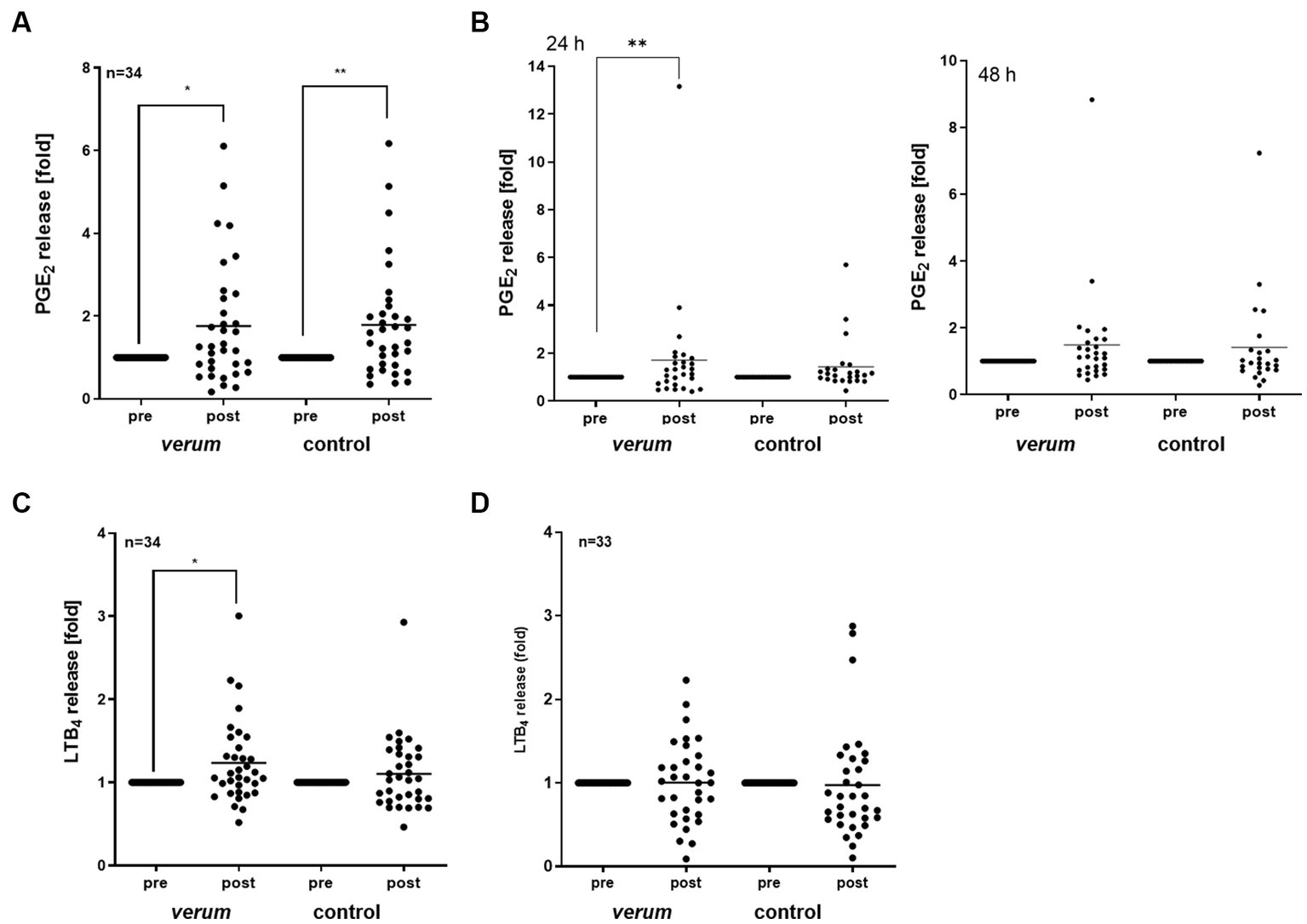
Figure 5. Effects of nasturtium intervention on COX/LOX pathway parameters in serum and LPS stimulated PBMC. PGE2 was quantified in serum samples (A). Isolated PBMC were stimulated with 100 ng/mL LPS for 24 or 48 h (B). LTB4 was quantified in serum samples (C) or 1 h LPS/fMLP stimulated PBMC (D). Data are shown as fold control post/pre. Each dot represents results from one participant tested and the line represents the mean. Asterisk indicates statistically difference. *p < 0.05, **p < 0.01.
We additionally analyzed the data for a potential carryover effect between the two intervention phases, but a p-value of 0.7498 indicated that this was not the case.
While breakdown of arachidonic acid through the enzymes COX-2 and microsomal prostaglandin E synthase-1 results in PGE2 production, it can also lead to LTB4 formation through 5-lipooxygenase (5-LOX) (21). Thus, we also analyzed the effect of intervention with nasturtium on LTB4 in both serum as well as LPS/N-formylmethionine-leucyl-phenylalanine (fMLP) stimulated PBMC. We observed that the mean LTB4 level was significantly increased in serum upon verum intervention (1.24-fold, p ≤ 0.01, Figure 5C). This was not evident in stimulated PBMC (1.00-fold, p = 0.835, Figure 5D).
The response in PGE2 to the intervention was quite heterogeneous, although the present study population was similar in many baseline parameters such as age, sex, weight, or height. To determine the effect of the intervention at the individual level, we next categorized subjects with a > 25% decrease in PGE2 serum level as “responders,” and those with an increase in PGE2 > 25% as “adverse responders.” Following this, 26% of the verum group could be classified as “responders,” 52% as “adverse responders,” and 22% showed no relevant change. For the control group, 24% of subjects could be classified as “responders,” 58% as “adverse responders,” and 18% showed no relevant change.
Previous studies addressed the question of whether an individual’s outcome could depend on the baseline level of the parameter of interest, (e.g., 22). Following this idea, we then first calculated the pre-to-post changes in PGE2 levels from each volunteer and correlated these to the corresponding PGE2 baseline levels. The results are presented in Figure 6 (A: PGE2 serum levels, B: PGE2 release from 24 h stimulated PBMC). An inverse correlation could be seen between the baseline PGE2 levels and the magnitude of change upon verum intervention in serum samples (verum: r = −0.3299, p = 0.0608; control: r = −0.02841, p = 0.8753), and in stimulated PBMC from both intervention groups (verum: r = −0.6404, p = 0.0002; control: r = −0.5696, p = 0.003). However, there are some statistical pitfalls in correlating the baseline value with the change in a factor, such as the artifact of regression to the mean or random variation within subjects, as described by Atkinson and Batterham (23). We thus calculated the change and standard deviation (SD) in PGE2 levels between the two baselines and compared this with the change upon verum or control intervention. In serum samples, the mean change from baseline was 189.5 pg/mL (SD: 345.9, CI: 68.80; 310.2), the change in the verum group was 241.9 pg/mL (SD: 252, CI: 154; 329.8), and the change in the control group was 244.3 pg/mL (SD: 311.5, CI: 135.6; 353). Since the mean SD in the baseline change was larger than the mean values in the intervention groups, a regression artifact cannot be excluded. In 24 h stimulated PBMC the mean change from baseline was 5496 pg/mL (SD: 4891, CI: 3381; 7611), the change in the verum group was 7862 pg/mL (SD: 7637 CI: 4900; 10,923) and the change in the control group was 4725 pg/mL (SD: 5725, CI: 2308; 7142). In this case, a pseudo effect is unlikely. However, having a relatively small sample size and no comparator arm without any treatment, the validity of this analysis is limited.
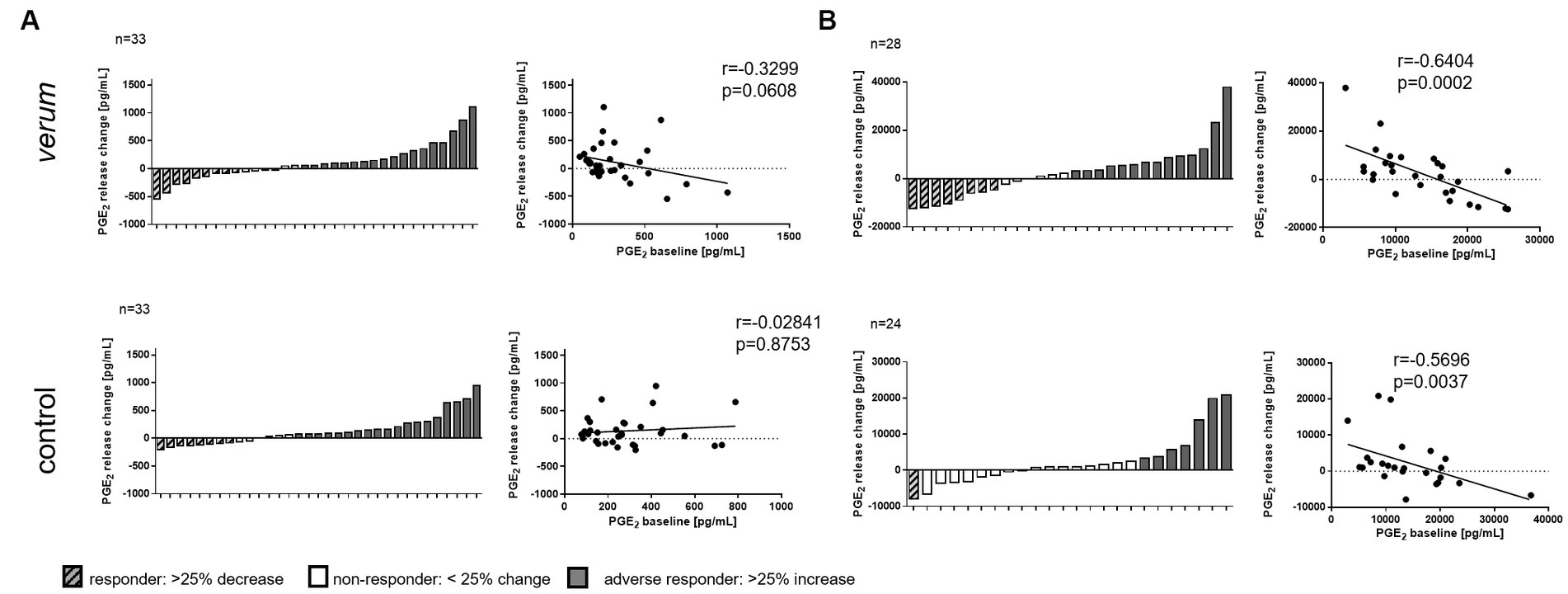
Figure 6. Individual responses to nasturtium intervention. Waterfall diagrams depict the individual change in release of PGE2 upon intervention with verum or control in serum (A) or LPS stimulated PBMC for 24 h (B). Each bar represents one participant. Dot plots show the correlation between baseline PGE2 and change in PGE2 level after intervention. Each dot represents one participant. Data for the correlation coefficient (r) and significancy (p) are given.
3.4. Influence of nasturtium intervention on TNF-α and interleukins
Upon invasion of foreign pathogens such as LPS-generating gram-negative bacteria, an inflammatory cascade is immediately activated. It produces, besides lipid mediators, pro-inflammatory cytokines, such as TNF-α, or IL-1β. As a significant upregulation in PGE2 was seen in intervention groups, we also studied the release of cytokines from LPS-activated cells. As given in Figure 7A, intervention with verum significantly increased TNF-α release in LPS stimulated PBMC after 3 h (1.65-fold, p = 0.0032; pre: mean 780.8 ± 316.4 pg/mL vs. post: mean 1019.62 ± 452.3 pg/mL). Intervention with control preparation had no effect on LPS-stimulated PBMC (1.22-fold, p = 0.7818; pre: mean 1051 ± 524.2 pg/mL vs. post: mean 1108 ± 503.4 pg/mL). After 24 h, the TNF-α level was back to baseline. No change was seen in the anti-inflammatory cytokine IL-10, or the pro-inflammatory cytokines IL-1β, and IL-12 (Figures 7B–D).
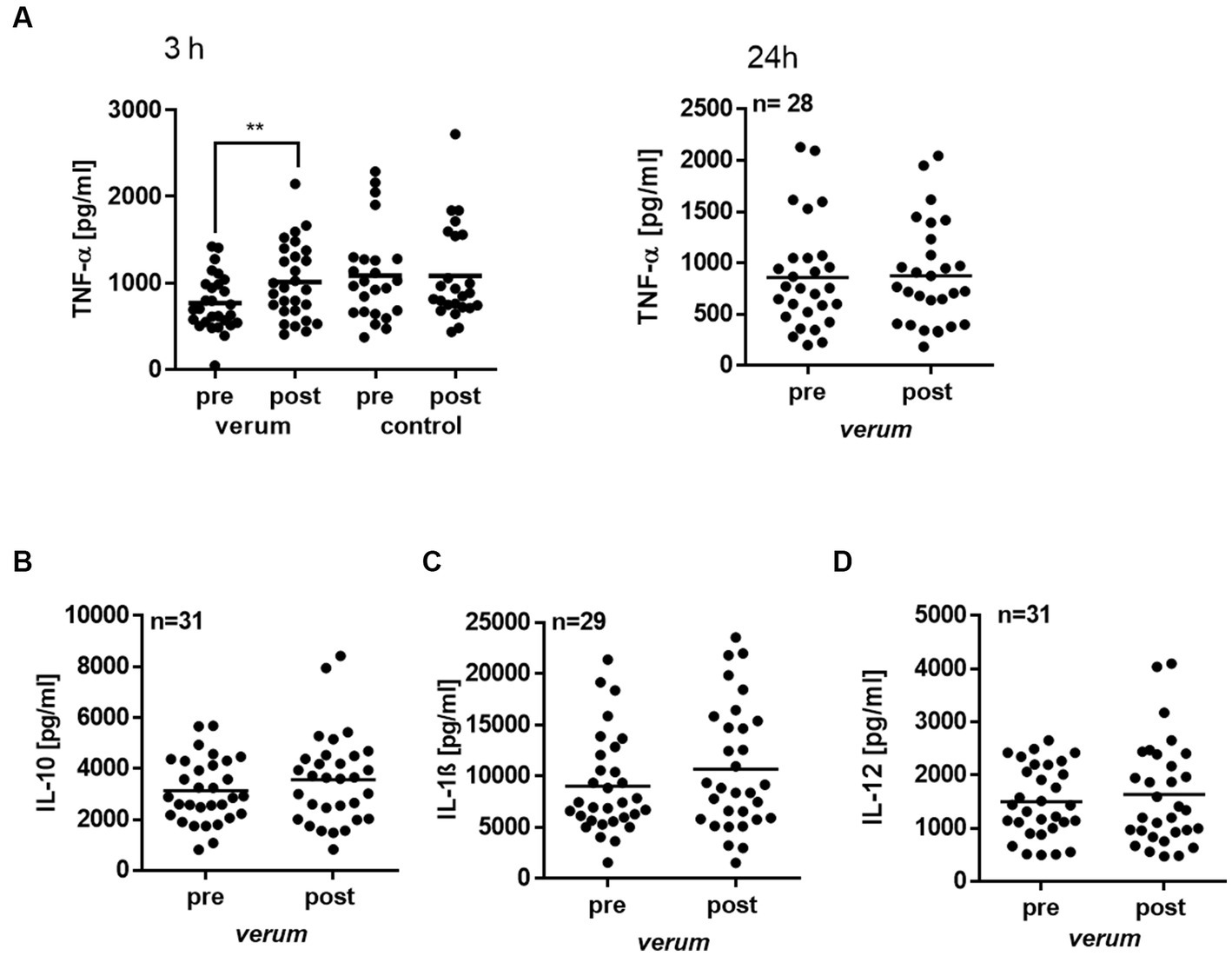
Figure 7. Effect of nasturtium intervention on cytokine release in LPS stimulated PBMC. Isolated PBMC were stimulated with 100 ng/mL LPS for 3 or 24 h before (pre) or after (post) intervention. TNF-α (A), IL-10 (B), IL-1β (C) and IL-12 (D) cytokines were analyzed by ELISA. Each dot represents the result from one participant tested in duplicates, and the line represents the mean. Asterisk indicates statistically difference. **p < 0.01.
4. Discussion
Increased consumption of ITC-containing foods such as nasturtium may improve human health to a certain extent (24). However, randomized, controlled human trials are currently lacking to provide a solid basis for recommending targeted consumption of these foods to protect health.
PGE2 is a multifunctional molecule that orchestrates a wide array of biological responses, homeostasis and inflammation in the human body. The ability of PGE2 to either activate or suppress biological processes, including inflammation, is context-dependent which reflects the complexity of this receptor system (25). It is involved in temperature regulation (11), crucial for muscle regeneration, or osteoblast bone formation (26, 27). In the early phases of a bacterial infection, PGE2 and related prostanoids act as vasodilators. Tissue influx of neutrophils, macrophages, and mast cells from the bloodstream is thereby facilitated, resulting in swelling and edema at the site of infection or tissue injury (28). Furthermore, PGE2 controls a number of processes that result in the clearance of the inflammation and subsequent tissue repair (25, 29). Using LPS-activated PBMC from humans, we found in vitro that aqueous nasturtium extract concentration-dependently decreased PGE2 levels at concentration corresponding to BITC 0.15 to 4.2 nmol/mL (8), suggesting that nasturtium might suppress an acute inflammatory response upon bacterial infection. A recent human crossover intervention trial (n = 19) with single intake of fresh watercress (Nasturtium officinale) reported a mild pro-inflammatory reaction in LPS-stimulated whole blood cultures from healthy subjects upon consumption of watercress as compared to control group, though. This was shown by significantly increased mean levels of TNF-α, IL-1β, and IL-6 (30). In the present study, we found that mean PGE2 serum levels increased upon nasturtium intervention as compared to baseline. In LPS stimulated PBMC, this was seen for PGE2, as well as for TNF-α. In the intervention group, we detected low level of BITC-NAC (43.91 ± 44.58 nmol/L) in serum after the verum intervention. Compared to a recent in vitro study, BITC concentration achieved in serum was very low. This could also be an explanation for the different effect on PGE2. However, we observed considerable variation in response between individuals, with some subjects showing an increase in PGE2 response while in another part it was decreased upon intervention. This concurs with other studies, in which usually only up to 40% of subjects responded to intervention, (e.g., 22). Reasons may include individual factors, like genetic differences or factors such as the composition of the gut microbiome (31). Further analysis of the present data indicated that individuals with relatively high baseline PGE2 levels may be more likely to respond with suppression of the prostanoid to nasturtium intake and vice versa. However, the hypothesis of such adaptogenic properties of the plant needs further validation, because these additional analyses were only of exploratory nature.
In the present study, it was investigated whether BITC significantly accounts for the biological effects of nasturtium. Thus, nasturtium with inactivated myrosinase as control was used. The absence of BITC formation during nasturtium preparation could be confirmed by chemical analysis. No relevant BITC metabolites were detected in serum samples of the subjects after intake of the control preparation. In urine, the level of BITC in the control group was only 19% of that found in the verum group, which concurs with a low, unavoidable GLS conversion by intestinal bacteria (32). Consequently, present results here suggest that BITC does not play a crucial role for the observed effect of nasturtium on PGE2.
While baseline serum and urine samples confirmed the absence of BITC before intervention, we found BITC-Lys protein conjugates in a number of the serum samples. ITC protein adducts are considered to be more stable biomarkers reflecting a larger time span of ITC exposure history. Stable albumin adducts have a reported half-life of 20–25 days (20, 33). In the present intervention study, we reprocessed these data according to the respective onset of the first round of intervention. This revealed that BITC-Lys adducts were already mainly present in the baseline sample of the control group that had previously received the verum intervention. Thus, it can be assumed that the time between the two intervention phases was not sufficient to eliminate all ITCs. The extent to which these remaining adducts may have influenced the results reported here is impossible to say. Protein adducts have been discovered to be involved in the chemopreventive effects of ITCs (34). Statistical data analysis gave, however, no clear evidence for a relevant carry-over effect between intervention groups. To ensure that contamination does not occur between study phases, we recommend that future experiments consider even greater separation of intervention phases.
In contrast to the previously reported in vitro results, on average LPS activated PBMC from verum or control group showed increased PGE2 levels. This was also true for subjects’ serum samples, indicating that the results were independent from the BITC content of nasturtium. Using the mean values, individual differences are ignored though, and we observed a high variability in individual responses as reaction to the treatment. The responder analysis performed here suggests that PGE2 formation after intervention depends whether or not baseline PGE2 was high, but this needs to be further verified in larger studies. Such a relationship could help predict the effectiveness of nasturtium for modulating PGE2 on the level of the individual in the future. It also provides clues for the subsequent design and analysis of studies on this medicinal plant, which may allow us to better determine clinically significant effects in patient outcomes. In the long term, identifying phenotypes that respond differently to an intervention may thus improve the delivery of personalized nutrition or phytopharmaceutical drugs and optimize the outcome for the individual.
Data availability statement
The original contributions presented in the study are included in the article/supplementary material, further inquiries can be directed to the corresponding author.
Ethics statement
The studies involving human participants were reviewed and approved by the Ethical Committee of the University of Freiburg (ethical vote number 322/18, 11/13/2018). This study was conducted in accordance with the Declaration of Helsinki. This intervention trial was registered on German Clinical Trials Register (DRKS) with the ID: DRKS00016548. The patients/participants provided their written informed consent to participate in this study.
Author contributions
EL: conceptualization. LF, HT, JS, MK, and FH: methodology. CH, LF, HT, SC, JS, MK, FH, MA, NB, and EL: formal analysis. CH, LF, HT, SC, JS, MK, and FH: investigation. RS, MS, and EL: resources. CH, LF, and EL: writing—original draft preparation. FH, SR, and MS: writing—review and editing. All authors have read and agreed to the published version of the manuscript. The listing order of the authors is without weighting.
Funding
The intervention trial was partly supported by a grant from Repha GmbH, Langenhagen, Germany. Repha GmbH was not involved in the design, conduction, interpretation, or publishing of the results. FH was funded by the Leibniz Association (J16/2017). The manuscript processing fee was partly funded by the University of Freiburg in the funding program Open Access Publishing.
Acknowledgments
Andrea Jankowsky and Jessica Eichhorn are thanked for excellent technical assistance.
Conflict of interest
The authors declare that the research was conducted in the absence of any commercial or financial relationships that could be construed as a potential conflict of interest.
Publisher’s note
All claims expressed in this article are solely those of the authors and do not necessarily represent those of their affiliated organizations, or those of the publisher, the editors and the reviewers. Any product that may be evaluated in this article, or claim that may be made by its manufacturer, is not guaranteed or endorsed by the publisher.
References
1. Conrad, A, Kolberg, T, Engels, I, and Frank, U. In vitro study to evaluate the antibacterial activity of a combination of the haulm of nasturtium (Tropaeoli majoris herba) and of the roots of horseradish (Armoraciae rusticanae radix). Arzneimittelforschung. (2006) 56:842–9. doi: 10.1055/s-0031-1296796
2. Goos, KH, Albrecht, U, and Schneider, B. Efficacy and safety profile of a herbal drug containing nasturtium herb and horseradish root in acute sinusitis, acute bronchitis and acute urinary tract infection in comparison with other treatments in the daily practice/results of a prospective cohort study. Arzneimittelforschung. (2006) 56:249–57. doi: 10.1055/s-0031-1296717
3. Barba, FJ, Nikmaram, N, Roohinejad, S, Khelfa, A, Zhu, Z, and Koubaa, M. Bioavailability of glucosinolates and their breakdown products: impact of processing. Front Nutr. (2016) 3:24. doi: 10.3389/fnut.2016.00024
4. Herz, C, Márton, M, Tran, H, Gründemann, C, Schell, J, and Lamy, E. Benzyl isothiocyanate but not benzyl nitrile from Brassicales plants dually blocks the COX and LOX pathway in primary human immune cells. J Funct. (2016) 23:135–43. doi: 10.1016/j.jff.2016.02.034
5. Lee, YM, Seon, MR, Cho, HJ, Kim, JS, and Park, JH. Benzyl isothiocyanate exhibits anti-inflammatory effects in murine macrophages and in mouse skin. J Mol Med (Berl). (2009) 87:1251–61. doi: 10.1007/s00109-009-0532-6
6. Nakamura, Y. Chemoprevention by isothiocyanates: molecular basis of apoptosis induction. Forum Nutr. (2009) 61:170–81. doi: 10.1159/000212749
7. Cartea, ME, Francisco, M, Soengas, P, and Velasco, P. Phenolic compounds in Brassica vegetables. Molecules. (2010) 16:251–80. doi: 10.3390/molecules16010251
8. Tran, H, Márton, M, Herz, C, Maul, R, Baldermann, S, Schreiner, M, et al. Nasturtium (Indian cress, Tropaeolum majus nanum) dually blocks the COX and LOX pathway in primary human immune cells. Phytomedicine. (2016) 23:611–20. doi: 10.1016/j.phymed.2016.02.025
9. Díaz-González, F, and Sánchez-Madrid, F. NSAIDs: learning new tricks from old drugs. Eur J Immunol. (2015) 45:679–86. doi: 10.1002/eji.201445222
10. Ricciotti, E, and FitzGerald, GA. Prostaglandins and inflammation. Arterioscler Thromb Vasc Biol. (2011) 31:986–1000. doi: 10.1161/atvbaha.110.207449
11. Shin, YO, Lee, JB, Min, YK, and Yang, HM. Heat acclimation affects circulating levels of prostaglandin E2, COX-2 and orexin in humans. Neurosci Lett. (2013) 542:17–20. doi: 10.1016/j.neulet.2013.03.017
12. Funk, CD. Prostaglandins and leukotrienes: advances in eicosanoid biology. Science. (2001) 294:1871–5. doi: 10.1126/science.294.5548.1871
13. Schlotz, N, Odongo, GA, Herz, C, Waßmer, H, Kühn, C, Hanschen, FS, et al. Are raw Brassica vegetables healthier than cooked ones? A randomized, controlled crossover intervention trial on the health-promoting potential of Ethiopian kale. Nutrients. (2018) 10:1622. doi: 10.3390/nu10111622
14. Giefing-Kröll, C, Berger, P, Lepperdinger, G, and Grubeck-Loebenstein, B. How sex and age affect immune responses, susceptibility to infections, and response to vaccination. Aging Cell. (2015) 14:309–21. doi: 10.1111/acel.12326
15. Platz, S, Kühn, C, Schiess, S, Schreiner, M, Kemper, M, Pivovarova, O, et al. Bioavailability and metabolism of benzyl glucosinolate in humans consuming Indian cress (Tropaeolum majus L.). Mol Nutr Food Res. (2016) 60:652–60. doi: 10.1002/mnfr.201500633
16. Hanschen, FS, and Schreiner, M. Isothiocyanates, nitriles, and epithionitriles from glucosinolates are affected by genotype and developmental stage in Brassica oleracea varieties. Front Plant Sci. (2017) 8:1095. doi: 10.3389/fpls.2017.01095
17. Wermter, NS, Rohn, S, and Hanschen, FS. Seasonal variation of glucosinolate hydrolysis products in commercial white and red cabbages (Brassica oleracea var. capitata). Foods. (2020) 9:1682. doi: 10.3390/foods9111682
18. Kühn, C, Kupke, F, Baldermann, S, Klopsch, R, Lamy, E, Hornemann, S, et al. Diverse excretion pathways of benzyl glucosinolate in humans after consumption of nasturtium (Tropaeolum majus L.)-a pilot study. Mol Nutr Food Res. (2018) 62:e1800588. doi: 10.1002/mnfr.201800588
19. Wellek, S, and Blettner, M. On the proper use of the crossover design in clinical trials: part 18 of a series on evaluation of scientific publications. Dtsch Arztebl Int. (2012) 109:276–81. doi: 10.3238/arztebl.2012.0276
20. Kumar, A, and Sabbioni, G. New biomarkers for monitoring the levels of isothiocyanates in humans. Chem Res Toxicol. (2010) 23:756–65. doi: 10.1021/tx900393t
21. Wang, B, Wu, L, Chen, J, Dong, L, Chen, C, Wen, Z, et al. Metabolism pathways of arachidonic acids: mechanisms and potential therapeutic targets. Signal Transduct Target Ther. (2021) 6:94. doi: 10.1038/s41392-020-00443-w
22. de Roos, B, and Brennan, L. Personalised interventions-a precision approach for the next generation of dietary intervention studies. Nutrients. (2017) 9:847. doi: 10.3390/nu9080847
23. Atkinson, G, and Batterham, AM. True and false interindividual differences in the physiological response to an intervention. Exp Physiol. (2015) 100:577–88. doi: 10.1113/ep085070
24. Connolly, EL, Sim, M, Travica, N, Marx, W, Beasy, G, Lynch, GS, et al. Glucosinolates from cruciferous vegetables and their potential role in chronic disease: investigating the preclinical and clinical evidence. Front Pharmacol. (2021) 12:767975. doi: 10.3389/fphar.2021.767975
25. Sreeramkumar, V, Fresno, M, and Cuesta, N. Prostaglandin E2 and T cells: friends or foes? Immunol Cell Biol. (2012) 90:579–86. doi: 10.1038/icb.2011.75
26. Ho, ATV, Palla, AR, Blake, MR, Yucel, ND, Wang, YX, Magnusson, KEG, et al. Prostaglandin E2 is essential for efficacious skeletal muscle stem-cell function, augmenting regeneration and strength. Proc Natl Acad Sci U S A. (2017) 114:6675–84. doi: 10.1073/pnas.1705420114
27. Chen, H, Hu, B, Lv, X, Zhu, S, Zhen, G, Wan, M, et al. Prostaglandin E2 mediates sensory nerve regulation of bone homeostasis. Nat Commun. (2019) 10:181. doi: 10.1038/s41467-018-08097-7
28. Nakanishi, M, and Rosenberg, DW. Multifaceted roles of PGE2 in inflammation and cancer. Semin Immunopathol. (2013) 35:123–37. doi: 10.1007/s00281-012-0342-8
29. Cheng, H, Huang, H, Guo, Z, Chang, Y, and Li, Z. Role of prostaglandin E2 in tissue repair and regeneration. Theranostics. (2021) 11:8836–54. doi: 10.7150/thno.63396
30. Schulze, H, Hornbacher, J, Wasserfurth, P, Reichel, T, Günther, T, Krings, U, et al. Immunomodulating effect of the consumption of watercress (Nasturtium officinale) on exercise-induced inflammation in humans. Foods. (2021) 10:1774. doi: 10.3390/foods10081774
31. Manach, C, Milenkovic, D, Van de Wiele, T, Rodriguez-Mateos, A, de Roos, B, Garcia-Conesa, MT, et al. Addressing the inter-individual variation in response to consumption of plant food bioactives: towards a better understanding of their role in healthy aging and cardiometabolic risk reduction. Mol Nutr Food Res. (2017) 61. doi: 10.1002/mnfr.201600557
32. Sikorska-Zimny, K, and Beneduce, L. The metabolism of glucosinolates by gut microbiota. Nutrients. (2021) 13. doi: 10.3390/nu13082750
33. Skipper, PL, and Tannenbaum, SR. Protein adducts in the molecular dosimetry of chemical carcinogens. Carcinogenesis. (1990) 11:507–18. doi: 10.1093/carcin/11.4.507
Keywords: Tropaeolum majus, benzyl isothiocyanate, Brassicaceae, nutrition-based intervention, personalized nutrition, responder analysis
Citation: Herz C, Frei L, Tran HTT, Claßen S, Spöttel J, Krell M, Hanschen FS, Arvandi M, Binder N, Schreiner M, Rohn S and Lamy E (2023) A monocentric, randomized, double-blind, controlled crossover trial of nasturtium (Tropaeolum majus) on the lipid regulator prostaglandin E2. Front. Nutr. 10:1223158. doi: 10.3389/fnut.2023.1223158
Edited by:
Ângela Batista, Juiz de Fora Federal University, BrazilReviewed by:
Lauane Gomes Moreno, Universidade Federal dos Vales do Jequitinhonha e Mucuri (UFVJM), BrazilZora Djuric, University of Michigan, United States
Copyright © 2023 Herz, Frei, Tran, Claßen, Spöttel, Krell, Hanschen, Arvandi, Binder, Schreiner, Rohn and Lamy. This is an open-access article distributed under the terms of the Creative Commons Attribution License (CC BY). The use, distribution or reproduction in other forums is permitted, provided the original author(s) and the copyright owner(s) are credited and that the original publication in this journal is cited, in accordance with accepted academic practice. No use, distribution or reproduction is permitted which does not comply with these terms.
*Correspondence: Evelyn Lamy, ZXZlbHluLmxhbXlAdW5pa2xpbmlrLWZyZWlidXJnLmRl
†These authors share first authorship
‡ORCID: Nadine Binder, https://orcid.org/0000-0002-1919-4154