- 1Shanxi Institute for Functional Food, Shanxi Agricultural University, Taiyuan, China
- 2College of Food Science and Engineering, Shanxi Agricultural University, Taigu, China
- 3Institute of Basic Medical Sciences, Khyber Medical University, Peshawar, Pakistan
- 4Chemistry Department, Faculty of Applied Sciences, Al-Leith University College, Umm Al-Qura University, Makkah, Saudi Arabia
- 5College of Animal Science and Technology, Shanxi Agricultural University, Taigu, China
This study investigated the effect of Flammulina velutipes polysaccharides (FVPs) on the myofibrillar protein (MP) oxidation protein and physicochemical properties of catfish surimi during 75 days of frozen storage at −18°C. FVP was added to surimi at 1%, 1.5%, and 2%, respectively; the degree of MP oxidation and the physicochemical properties of the surimi were investigated, and the microstructure of the surimi was observed by scanning electron microscopy (SEM). The results showed that the carbonyl content and the thiobarbituric acid reactive substances (TBARS) in the FVP groups were lower than those in the CK group (the blank surimi). In comparison, the total sulfhydryl content, solubility, and Ca2+-ATPase activity were higher than those in the CK group after 75 days of storage. The addition of FVP significantly increased the water-holding capacity (WHC), gel strength, elastic modulus (G'), and loss modulus (G“) of surimi, and made the gel of surimi have stronger continuity and a denser structure. Therefore, FVP has a better cryoprotective effect on surimi. It improves the quality of surimi, decreases MP oxidation, and reduces lipid and water loss during frozen storage. The anti-freezing effect of FVP added at 2% was similar to that of commercial protectants (4% sucrose and 4% sorbitol).
1. Introduction
Surimi is a wet concentrate of myofibrillar proteins prepared from the muscles of deboned fish after removing blood, lipids, and other impurities using cold water (1). Frozen storage is one of the most effective ways to preserve surimi products at home and on a commercial scale (2). Frozen storage inhibits the growth and reproduction of microorganisms, reduces the activity of enzymes, and effectively extends the shelf life of surimi products. However, frozen storage can cause the quality of surimi to deteriorate, especially at prolonged storage times. This is due to the oxidation of proteins and fats by oxidative enzymes and pro-oxidants during frozen storage. The direct oxidation of aliphatic amino acids (3), the direct cleavage of peptide backbone chains (4), the Michael addition reaction of unsaturated aldehydes and proteins, and the non-enzymatic catalytic reaction of reducing sugars lead to the formation of carbonyl compounds (5). The conversion of sulfhydryl groups into disulfide bonds leads to a decrease in the sulfhydryl content (6), which is a sign of protein oxidative damage. Studies have reported protein degradation and lipid peroxidation as the two mechanisms responsible for the deterioration in quality and increased perishability when surimi is stored for a prolonged period. The structural changes in surimi proteins, especially myofibrillar protein (MP), occur either due to changes in the α-helical structure of surimi MP (7), an increase in the relative content of disordered curls (8–10), or conformational changes in the main chain (from ordered to disordered units). Moreover, surimi is rich in unsaturated fatty acids (11) and thus highly susceptible to oxidation during the freezing process. Lipid peroxidation in surimi produces many different aldehydes, ketones, and acids, resulting in the oxidation and decrease in gelation of MP, hence quality deterioration. To preserve and protect surimi quality during frozen storage, food industries commonly add 4% sucrose and sorbitol as commercial preservatives to inhibit the oxidation of MP (12). However, the sweetness and caloric content of these cryoprotectants are relatively high and not in line with the current concept of pursuing a healthy diet. Therefore, the search for cryoprotectants that are low in sweetness and caloric content has recently become a hot topic of research in food industries across the world. In this context, polysaccharides may offer a cost-effective, healthy, and safe alternative to traditional cryoprotectants for surimi preservation and maintaining quality during frozen storage.
Polysaccharides are natural polymers of aldose or ketose linked together by glycosidic bonds and found in plants, algae, bacteria, and animals. The structural unit in these molecules is monosaccharides that are linked by either α-1,4-glycosidic, β-1,4-glycosidic, or α-1,6-glycosidic bonds (13). Research evidence suggests that polysaccharides may have a cryoprotective effect on MP during the frozen storage of surimi. For example, Zhou et al. (14) reported that 8% seaweed sugar could effectively delay the denaturation process of MP in tilapia surimi and maintain Ca2+ATPase activity and MP solubility. Similarly, Nopianti et al. (15) reported that lactitol, alginate, and dextran exert an anti-freezing effect and prevent the denaturation of MP during frozen storage. Research on polysaccharides obtained from natural sources as cryoprotective agents is also underway.
Flammulina velutipes is known as the constructed mushroom and the hairy stalked money mushroom, which has a beautiful form, a fresh taste, and a high economic value (16). Flammulina velutipes is a rich source of polysaccharides, triterpenes, sterols, and vitamins with proven antioxidant, anti-inflammatory, immune-modulating, and antimicrobial activities. These beneficial activities have mainly been attributed to the presence of polysaccharides. Flammulina velutipes polysaccharide (FVP) is a water-soluble polysaccharide extracted from Flammulina velutipes mainly through hot water, enzyme, or ultrasound/microwave-assisted extraction methods (17–19). Zheng et al. (20) showed that the protein oxidation of freeze–thawed large yellow croaker was inhibited by FVP treatment, as demonstrated by the sulfhydryl group and intrinsic fluorescence intensity. Xie et al. (21) studied the effect of FVP on the quality of Salmo salar during frozen storage, and the results showed the best effect on protein oxidation by adding 0.15 mg/mL of FVP. In summary, FVP can effectively improve protein denaturation and potentially protein oxidation in the frozen food field. However, to date, the majority of the research has focused on the functional evaluation of FVP and paid less attention to its production and industrial use, thus greatly limiting the translational aspects of FVP research results.
In the current study, we have extracted the polysaccharide from the roots of Flammulina velutipes, which were often discarded due to their difficulty to swallow when consumed, and evaluated the effect of Flammulina velutipes polysaccharides on the physicochemical properties of catfish surimi and myofibrillar protein oxidation during frozen storage, with the ultimate goals being to gain deeper insights and potential for using FVP as a natural cryoprotectant in frozen surimi.
2. Materials and methods
2.1. Materials and reagents
Flammulina velutipes were purchased from the Edible Mushroom Research Centre, Shanxi Agricultural University (Jinzhong, Shanxi, China). A total of 102 live catfish (C. gariepinus) with an average weight of 1,250 ± 25 g and a length of 30 ± 2 cm were purchased from a local aquaculture farm at Taigu Taoyuanbao (Jinzhong, Shanxi, China). Sucrose and sorbitol were purchased from Shandong Dingsheng Chemical Co., Ltd. (Shandong, China), while the chemicals including ether, KCl, MgCl2, NaCl, HCl, glycine, and ethylenediaminetetraacetic acid were purchased from Chengdu Chron Chemical Co., Ltd.; urea, DTNB, Kormas Brilliant Blue, 1,4-Piperazinediethanesulfonic acid (PIPES), sodium pyrophosphate, and Na2HPO4 were purchased from Tianjin Kaitong Reagent Co., Ltd. (Tianjin, China); all the above reagents were of analytical grade.
2.2. Methodology
2.2.1. Preparation of FVP
The FVP was extracted from the roots of Flammulina velutipes by ultrasound-assisted hot water extraction method. For this purpose, the roots of Flammulina velutipes were first washed and then dried at 60°C for 24 h until they reached a constant weight. The roots were then mechanically crushed into the powder using a commercially available grinder (model RT-02A, Rong Tsong Precision Technology Co., Ltd., Taiwan, China) and passed through a 200 mesh sieve. The powder was added to distilled water (1:25 w/v) and ultrasonicated at 70°C for 45 min followed by centrifugation at 8,000 rpm for 10 min at 4 °C by a high-speed freezing centrifuge (EPPENDERF China Co., Ltd., Beijing, China). The resultant solution was concentrated to one-fifth volume on a rotary evaporator (model RE 52A, Yarong Co., Ltd., Shanghai, China) at 55°C for 14 h and then added anhydrous ethanol at a ratio of 1: 3 (v/v) and left overnight at 4°C in order to obtain a precipitate. The precipitate was collected by centrifugation, placed at 78°C for pre-freezing, and dried in a vacuum freeze-drying machine (Ningbo Xinzhi Biotechnology, China) for 48 h. The FVPs were sent to Borealis Biotechnology Ltd. for testing, and the results showed that the FVP consisted of glucose (Glc), galactose (Gal), mannose (Man), fucose (Fuc), and xylose (Xyl) at a molar ratio of 55.5:25.9:10:5.5:2.
2.2.2. Preparation of catfish surimi
The catfish were immediately transferred to the Meat Laboratory of Shanxi Agricultural University and kept in ice water (0–4°C) for 15 min to stun them. The head, gut, bones, and skin were removed, and a large piece of fish filet was obtained. The filet was washed two times with a 0.15% NaCl solution (0–4°C), dehydrated, and minced in a meat grinder. The minced fish were divided into five parts by adding FVP at 0%,1%, 1.5%, and 2% of fish meat (mass weight), respectively. As a positive control, no FVP or commercial protectant with 4% sucrose and 4% sorbitol was used, namely the CK group, 1% FVP group, 1.5% FVP group, 2% FVP group, and Y group. Five groups were chopped in a chopper mixer (Qingpu Food Packaging; China) for 3 min to obtain catfish surimi, and 20% ice water was added to maintain a low temperature during chopping. Finally, the five groups were packaged in polyethylene bags (75 millimeters in thickness, 20 x 30 cm in dimension) with an oxygen permeability of 0.8 mL m −2 24 h −1 and a water vapor permeability of 2.4 g m −2 24 h −1 at 23°C. The samples were frozen and stored at −18°C in a refrigerator (Beijing Fuyi Electric Appliance Co., Ltd., Beijing, China) and sampled at 15, 30, 45, 60, and 75 days for index measurements.
2.2.3. Extraction and concentration measurement of surimi MP
The myofibrillar proteins (MPs) were extracted from catfish according to the Zhu et al. method (22) and stored at 4°C for backup. The concentration of protein was measured using the biuret method.
2.2.4. Determination of total sulfhydryl content
The total sulfhydryl content was evaluated according to the method described by Cai et al. (23)
2.2.5. Determination of the carbonyl content
The carbonyl content was analyzed according to the Levine et al. (24) method.
2.2.6. Determination of MP solubility
A 5 mg/mL of MP solution was prepared with PIPES buffer solution (0.6 mol/L of NaCl, 0.02 mol/L of MgCl2, 0.1 mol/L of PIPES, and 0.01 mol/L of odium pyrophosphate, pH = 6.2). The solution was kept at 2°C for 4 h and shaken every 20 min during this period. After 4 h, the solution was centrifuged at 2°C at 2,000 g for 15 min, and 1 mL of the supernatant was taken to measure the MP concentration. MP solubility is calculated according to the following formula (1):
2.2.7. Determination of Ca2+-ATPase activity
Ca2+-ATPase activity was measured by a commercially available Ca2+-ATPase activity assay kit (Solabao Biotechnology; China). The phosphorus content was quantified following the manufacturer's instructions, and the enzyme activity unit (U) was defined as the μmole number of inorganic phosphorus produced by a milligram of protein for 1 h at 25°C (μmol Pi/mg protein/h).
2.2.8. Determination of water-holding capacity
The water-holding capacity (WHC) of surimi was evaluated using the centrifuge method. Briefly, a 2 g sample of surimi with two layers of Whatman filter paper was placed in a centrifuge tube and centrifuged at 2,000 g for 15 min at 4°C. The WHC is calculated as the percentage of the surimi weight after centrifugation divided by the surimi weight before centrifugation.
2.2.9. Determination of color
The color of surimi was determined using the Xia et al. (25) method described previously. A colorimeter (CM-5 Konica Minolta Office System Co., Ltd., Tokyo, Japan) was used to determine the L*, a*, and b* values. The whiteness of surimi was calculated using the following formula (2):
where L* represents lightness value, a* denotes greenness/redness values, and b* indicates blueness/yellowness values.
2.2.10. pH measurement
Surimi (5 g) was homogenized for 30 s at a higher speed with 15 mL of distilled water. The pH of all samples was determined using an acidity meter (FE28, Metter Toledo, Shanghai, China).
2.2.11. Determination of gel strength
The surimi gel was prepared according to the method of Yan et al. (26). Gel samples were analyzed with a spherical metal probe (P/5S) using a texture profile analyzer (SMATA.XTPlus, Stable Micro Systems, UK). Samples were analyzed at test speed (120 mm/min), trigger force (0.4 N), and compression distance (10 mm). Gel strength was calculated according to the formula (3)
2.2.12. Determination of rheological properties
All rheological measurements were carried out using a rheometer (DHR-2, TA Instruments-Waters LLC, New Castle, DE, USA) equipped with a Peltier temperature control unit. Surimi samples frozen for 75 days were thawed and placed on the platform of the rheometer with a parallel plate pp50 test, spacing 1 mm, and temperature scanned in vibration mode within the linear viscoelastic zone, increasing the temperature from 20°C to 70°C at a ramp rate of 2°C/min, oscillation frequency set to 1 Hz, and strain set to 0.1%. The frequency sweep and temperature ramp measurements were carried out in the linear viscoelastic range (0.05% strain). The viscosity curves were determined under the controlled shear rate in a range of 1–10 s−1.
2.2.13. Determination of TBARS values
TBARS values were analyzed by the method of Guan et al. (27).
2.2.14. Microstructure
The microstructure of surimi gels was determined using scanning electron microscopy (SEM). Briefly, the surimi gels were prepared by cutting them into 3 mm × 3 mm × 2 mm cuboids. The cuboids were then fixed with 2.5% (v/v) glutaraldehyde in 0.2 M phosphate buffer solution (pH 7.2). The samples were rinsed for 1 h in distilled water and then dehydrated with serial concentrations in ethanol: 50%, 70%, 80%, 90%, and 100% (v/v). The dried samples were put on the aluminum stub using carbon tape, and the samples were coated with gold for approximately 60 s. The specimens were observed using a SEM (Quanta 450 SEM, FEI Company, Hillsboro, America).
2.3. Statistical analysis
Each experimental procedure was performed on three replicates. The data obtained were expressed as the mean values ± standard deviations and analyzed using SPSS version 22.0 (SPSS Inc., USA). One-way ANOVA was used to analyze all the data by DUNCAN's test with significance values of P < 0.05. All figures were designed using Origin Pro 9 (Origin Lab Inc., USA).
3. Results
3.1. Effect of FVP on the total sulfhydryl group content of surimi
The sulfhydryl is one of the most reactive functional groups in proteins, and can stabilize the spatial structure of MP (28). The effect of different cryoprotectant treatments on sulfhydryl content is shown in Figure 1. Overall, the sulfhydryl content of all samples decreased with an increase in freezing storage time. These findings were consistent with those reported by Cai et al. (29). Under the condition of moderate oxidation of MP, the sulfhydryl of MP is converted into disulfide bonds during the frozen storage, thus resulting in a decrease in the total sulfhydryl content. After 75 days of frozen storage, the total sulfhydryl content of surimi in the 2% FVP group was the highest (60.99 nmol/mg) followed by the 1.5% FVP and 1% FVP groups which were 53.37 nmol/mg and 50.33 nmol/mg, respectively. All these concentrations were significantly higher than those in the CK group (34.72 nmol/mg) (P < 0.05).
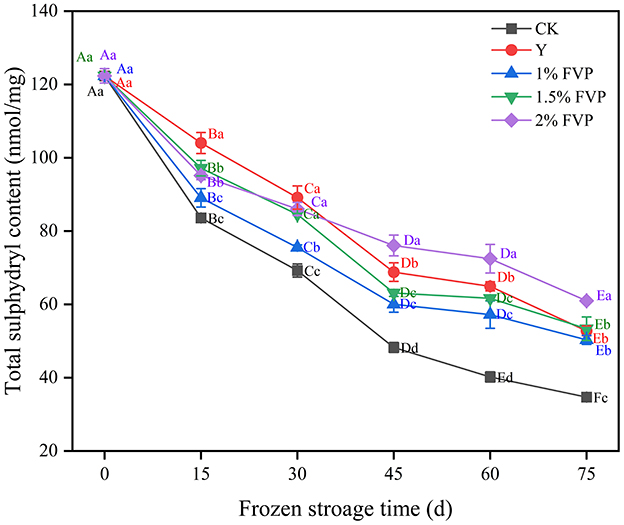
Figure 1. Effects of FVP on the sulfhydryl content of surimi MP. Data are expressed as mean ± standard deviation. Different lowercase letters indicated significant differences between different groups in the same freezing point (P < 0.05). Different capital letters indicated significant differences between different freezing sites in the same experimental group (P < 0.05).
3.2. Effect of FVP on the carbonyl content of surimi MP
The carbonyl content of surimi MP is produced by the direct oxidation of amino acid side chains (30), which is an important indicator of the oxidation of surimi MP. Chain groups on the amino acid side of MP are easy targets for oxygen radicals that break peptide bond, leading to an increase in the content of carbonyl compounds and their derivatives.
As shown in Figure 2, the carbonyl content of surimi MP increased in all groups as the frozen storage time extended, which was consistent with the results of Zhang et al. (31). This suggested that frozen storage enhanced the degree of protein oxidation and increased the protein carbonyl content, which may be related to the release of oxidase and prooxidants from ruptured organelles (32). Throughout the storage process, the MP carbonyl content of surimi in the CK group increased by 6.00 nmol/L, which was significantly higher than the FVP groups, and the MP carbonyl content of surimi in the 1%, 1.5%, and 2% FVP groups and the Y group increased by 4.17–4.74 nmol/L. The greater the concentration of FVP, the lower the content of carbonyl. However, the differences in carbonyl content between the Y group and the 1.5% and 2% FVP groups were not significant (P > 0.05).
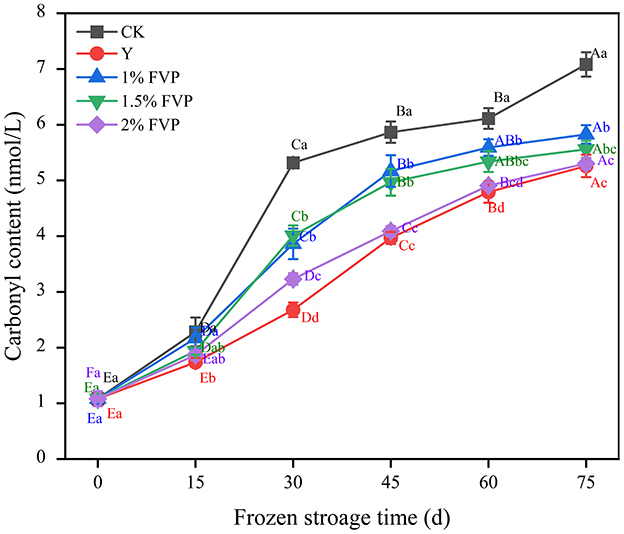
Figure 2. Effects of FVP on the carbonyl content of surimi MP. Data are expressed as mean ± standard deviation. Different lowercase letters indicated significant differences between different groups in the same freezing point (P < 0.05). Different capital letters indicated significant differences between different freezing sites in the same experimental group (P < 0.05).
3.3. Effect of FVP on the solubility of surimi MP
Protein solubility is a primary indicator of freezing-induced protein denaturation, which generally decreases due to hydrophobic interactions, hydrogen bonding, disulfide bonds, and ionic interactions during the frozen storage period (33). The effect of FVP on the solubility of surimi MP is shown in Figure 3, where the MP solubility of surimi decreased significantly (P < 0.05) in all groups before 30 days of frozen storage, thus indicating that a greater degree of MP denaturation occurs in the initial frozen period. These findings are in concordance with the previous studies [5]. At the end of frozen storage, the MP solubility of surimi decreased by 76.10%, 61.23%, 72.10%, 67.95%, and 63.31% in the CK, Y, 1%, 1.5%, and 2% FVP groups, respectively. MP solubility of surimi in the CK group was significantly lower (P < 0.05) than in the Y and FVP groups implying that FVP can effectively mitigate the decrease in protein solubility. On the other hand, hydroxyl groups can bind free water molecules and convert them into binding water, thus preventing the formation of ice. This conversion can result in fewer ice crystals and weaken the protein denaturation caused by the formation of ice crystals (34).
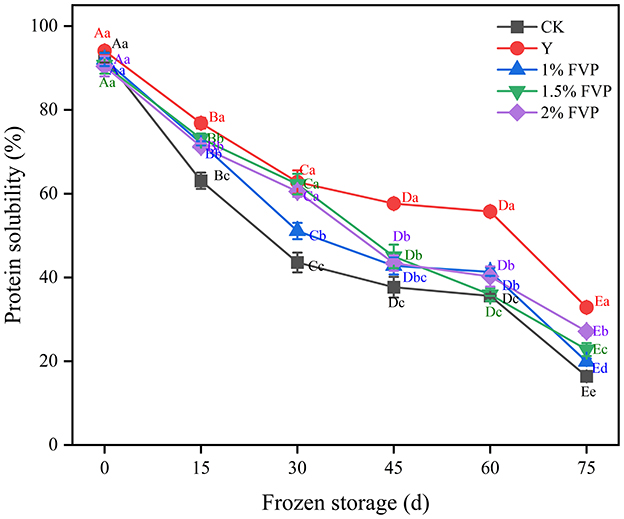
Figure 3. Effects of FVP on the solubility of surimi MP. Data are expressed as mean ± standard deviation. Different lowercase letters indicated significant differences between different groups in the same freezing point (P < 0.05). Different capital letters indicated significant differences between different freezing sites in the same experimental group (P < 0.05).
3.4. Effect of FVP on Ca2+-ATPase activity in surimi
Ca2+-ATPase activity is used as an indicator of myosin integrity and is widely used in the determination of the quality of frozen surimi (35). The Ca2+-ATPase activity of fresh surimi was 0.65 μmol/(mg min) and decreased continuously as the frozen storage time progressed, as shown in Figure 4. The Ca2+-ATPase activity of surimi in the CK group was lower than that of other experimental groups (Figure 4). A similar trend of decreased Ca2+-ATPase activity throughout frozen storage was also reported previously (36). At the end of frozen storage, Ca2+-ATPase activity was the highest in the Y group [0.22 μmol/(mg min) followed by the 2% FVP group (0.19 μmol/(mg min)], the 1.5% FVP group [0.11 μmol/(mg min)], and the 1% FVP group [0.05 μmol/(mg min)], and was the lowest in the CK group [0 μmol/(mg min)]. During frozen storage, the ice crystals change the structure of surimi proteins, resulting in a decrease in Ca2+-ATPase activity.
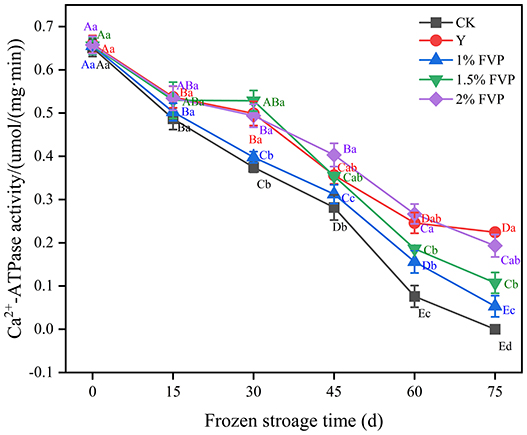
Figure 4. Effects of FVP on the Ca2+-ATPase activity of surimi MP. Data are expressed as mean ± standard deviation. Different lowercase letters indicated significant differences between different groups in the same freezing point (P < 0.05). Different capital letters indicated significant differences between different freezing sites in the same experimental group (P < 0.05).
3.5. Effect of FVP on the water-holding capacity of surimi
Water-holding capacity (WHC) is the ability to retain the original moisture content and add water when surimi suffers external forces that can visually evaluate the quality of surimi (37). As shown in Figure 5, the WHC of fresh surimi was 56.29% in the CK group and increased significantly with the addition of FVP (P < 0.05). Since FVP is hygroscopic, it can retain water content that can cross-link with surimi MP to become a stable complex (38). This is consistent with the results of Gao et al. (39), in which freezing storage led to the destruction of the secondary bonds that stabilize the MP structure. At 75 days of frozen storage, the WHC of surimi containing FVP was higher than that of the Y and CK groups, and the highest WHC was 53.42% in the 2% FVP group, followed by the 1.5% FVP group (51.44%). The lowest WHC was 47.43% in the CK group, which decreased by 8.86% compared with the fresh surimi. However, compared to the fresh fish surimi, the WHC of the 1%, 1.5%, and 2% FVP groups decreased by 7.52%, 6.48%, and 6.94%, respectively, thereby indicating that the addition of FVP can slow down the decrease of WHC in surimi during frozen storage.
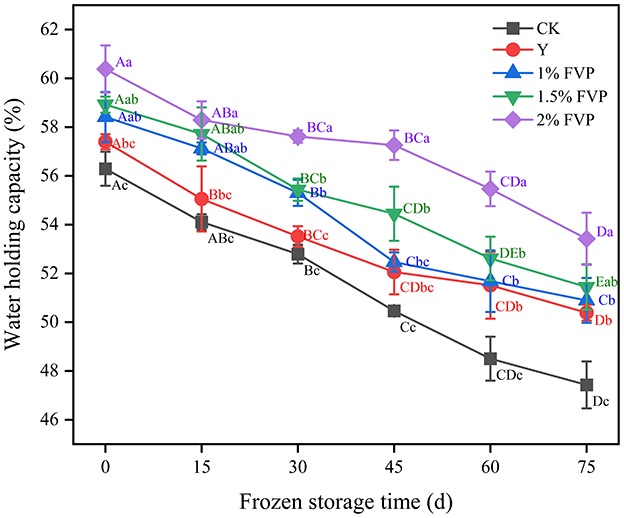
Figure 5. Effect of FVP on the water-holding capacity of surimi. Data are expressed as mean ± standard deviation. Different lowercase letters indicated significant differences between different groups in the same freezing point (P < 0.05). Different capital letters indicated significant differences between different freezing sites in the same experimental group (P < 0.05).
3.6. Effect of FVP on color and pH of surimi
The color of surimi is determined by both the myoglobin content and the color of the additives (40), and white is more popular among consumers. Different levels of FVP affected the color values (L*, a*, b*, and whiteness) of surimi during 75-day storage (Table 1). Overall, adding FVP results in a significant decrease in the L* and whiteness values of surimi compared with CK (P < 0.05). These changes were due to the yellowish color of FVP itself. However, the L*, a*, and b* values of surimi decreased after adding the commercial protectants, which also led to a decrease in whiteness values. As storage time was prolonged, the whiteness of surimi decreased gradually. At the end of frozen storage, the whiteness value of surimi dropped to 41.98 and 39.10 in the CK and Y groups, respectively, and the FVP group had a lower level (32.98–35.97).
A series of biochemical reactions in the surimi can lead to a change in pH during frozen storage, so the quality of the surimi can be estimated by measuring the change in pH (41). From Table 1, the pH of fresh surimi was 6.44, and the pH of FVP-added surimi decreased. This is due to the fact that the pH of FVP is lower than that of surimi itself. The pH of surimi showed an increasing trend during frozen storage, probably because of the degradation of proteins and production of alkaline substances such as amines (42), which is consistent with the trend of pH changes during freeze–thaw cycles of surimi products studied by Wilayat et al. (43). At 75 days of frozen storage, the pH of the CK group was lower than that of the FVP groups significantly (P < 0.05).
3.7. Effect of FVP on the gel strength of surimi
Gel strength is an essential index to evaluate the quality of surimi products, with higher gel strength values indicating better quality surimi products (44). From Figure 6, the gel strength of fresh surimi was 2352.87 g·mm, and the effect of adding commercial protectant (Y group) on the gel strength of surimi was not significant (P > 0.05), while the gel strength of FVP-added surimi increased significantly (P < 0.05). FVP is easily expanded by heat after absorbing water and fills in the gaps of the gel network structure of surimi, making the gel structure of surimi denser (45). The expanded FVP would exert a certain pressure on the surimi protein, which can further strengthen the gel strength of the surimi. The gel strength of surimi in the CK group was lower than that in the Y and FVP groups throughout the frozen period, and at 75 days of frozen storage, the maximum gel strength of surimi was 2428.92 g·mm in the 2% FVP group, which was 755.93 g·mm higher than that of the CK group.
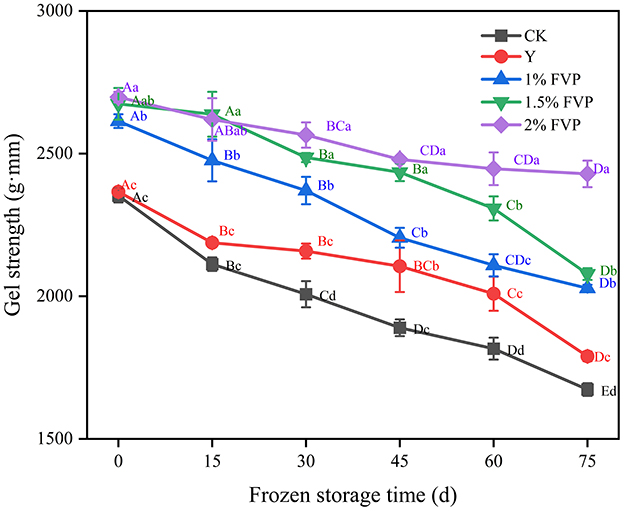
Figure 6. Effect of FVP on the gel strength of surimi. Data are expressed as mean ± standard deviation. Different lowercase letters indicated significant differences between different groups in the same freezing point (P < 0.05). Different capital letters indicated significant differences between different freezing sites in the same experimental group (P < 0.05).
3.8. Effect of FVP on the rheological properties of surimi
The energy storage modulus (G') is known as the elastic modulus, which reflects the formation of the protein gel network structure. The loss modulus (G”) is known as the viscous modulus, which reflects the viscous characteristics of the samples (46). Figure 7 shows the changes in G' and G” during the temperature scan from 20 to 70°C with different experimental groups. The responses for all samples follow a similar trend in which two stages can be distinguished. In the first stage, G′ and G″ decreased with temperature increasing to a minimum of ~50°C because the temperature at this point is the optimal activity temperature of endogenous hydrolase, the network structure of actin and myosin is broken, and some chemical bonds between protein molecules are broken, which makes protein molecules extend and surimi enter the gel deterioration stage. In the second stage, the changes, including the gel networks, hydrophobic bonds, disulfide bonds, and other chemical bonds, and the three-dimensional gel structure occurred (47), so that G′ and G″ increased rapidly to their maximum of ~70°C. G' and G” of the CK group were lower than those of the Y and FVP groups throughout the frozen process, which indicated that FVP and commercial protectants had a protective effect on the gel network structure of surimi. The FVP molecules improve the gel properties by filling the gaps of proteins and interacting with them, resulting in greater viscoelasticity when the FVP is added to the surimi. The best gel properties were found in the surimi of the 2% FVP group.
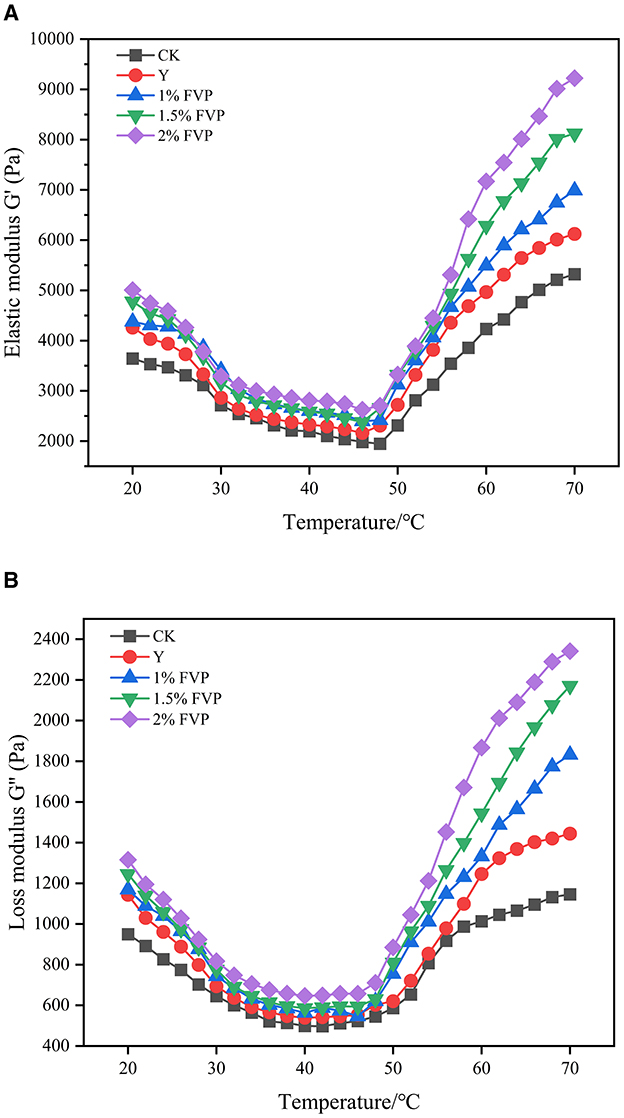
Figure 7. Effect of FVP on the energy storage modulus (G') (A) and the loss modulus (G”) (B) of surimi. Data are expressed as mean ± standard deviation. Different lowercase letters indicated significant differences between different groups in the same freezing point (P < 0.05). Different capital letters indicated significant differences between different freezing sites in the same experimental group (P < 0.05).
3.9. Effect of FVP on the TBARS value of surimi
Lipid oxidation produces complex reaction products such as ketones, aldehydes, hydrocarbons, and esters, which lead to off-flavors in surimi and negatively affect its quality (48). TBARS is widely used as an indicator to assess the degree of lipid oxidation (11). As shown in Figure 8, although the TBARS values increased slowly from 0 to 30 days of frozen storage, the TBARS values in all groups showed an increasing trend with the extension of frozen time. The CK groups were always higher than the FVP groups during the whole frozen storage time. After 75 days of frozen storage, the TBARS values of surimi in the CK group increased from 0.16 mg/kg to 0.54 mg/kg, an increase of 0.38 mg/kg, and the Y group was the lowest, only increasing by 0.17 mg/kg. When supplemented with 1%, 1.5%, and 2% FVP, the TBARS values increased by 0.25 mg/kg, 0.24 mg/kg, and 0.23 mg/kg, respectively, all of which were lower than the CK group. These results show that FVP exerts an inhibitory effect on the lipid oxidation of surimi (20). However, with the extension of frozen time, the antioxidant capacity of FVP diminishes, leading to a significant increase in the degree of oxidation of surimi in the later stages of freezing (P < 0.05).
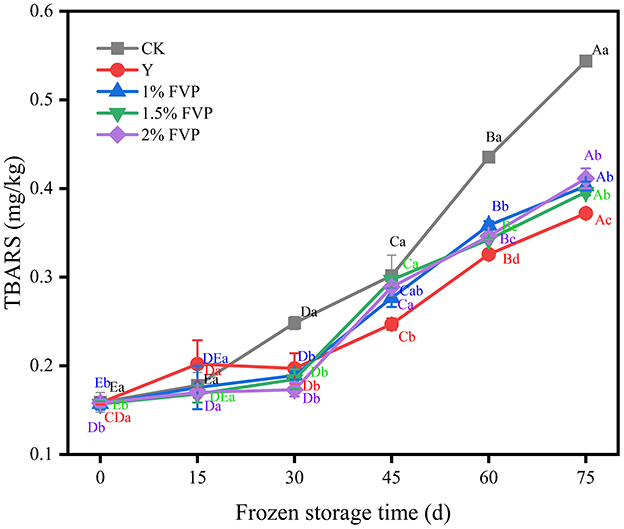
Figure 8. Effect of FVP on the TBARS of surimi. Data are expressed as mean ± standard deviation. Different lowercase letters indicated significant differences between different groups in the same freezing point (P < 0.05). Different capital letters indicated significant differences between different freezing sites in the same experimental group (P < 0.05).
3.10. Effect of FVP on the microstructure of surimi
The microstructures of surimi with different levels of FVP were visualized by SEM, as shown in Figure 9. There were more pores with larger pore sizes in the CK group, and the overall structure was rough and loose after 75 days of frozen storage. However, with the addition of FVP at 2%, the surface of the surimi gel was flat and smooth, and the network structure was tight, which was not significantly different from that of the Y group. Therefore, the pores of surimi gels in the CK group may be formed by water loss, while FVP can closely link with proteins to improve the gel quality. In addition, the multi-OH structure of FVP itself can absorb water lost during the thermal denaturation of surimi proteins to improve WHC and reduce pores. Zhuang et al. (49) also found that polysaccharides were beneficial for improving the structural properties of the composite gel.
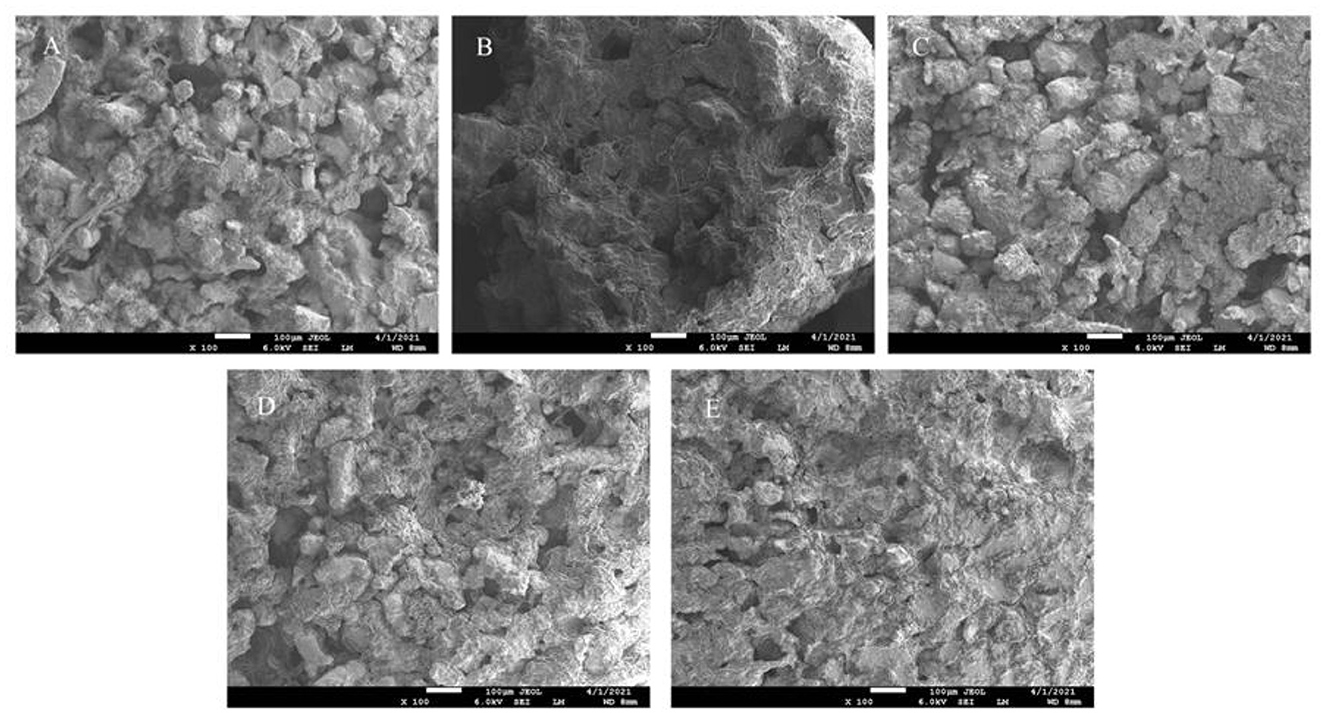
Figure 9. SEM photographs of CK group (A), Y group (B), 1% FVP group (C), 1.5% FVP group (D), and 2% FVP group (E). Data are expressed as mean ± standard deviation. Different lowercase letters indicated significant differences between different groups in the same freezing point (P < 0.05). Different capital letters indicated significant differences between different freezing sites in the same experimental group (P < 0.05).
4. Discussion
Flammulina velutipes is a popular mushroom species and has long been known for its nutritive and medicinal properties in folk medicine. While it is edible, the roots of Flammulina velutipes are often discarded due to difficulty in chewing, resulting in a waste of resources. However, the roots are rich in FVP, the most active ingredient found in Flammulina velutipes. In the current study, FVP was extracted from the roots of Flammulina velutipes using ultrasound-assisted hot water extraction. The molecular weight of FVP was found to be in the range of 27 KDa−9,178 KDa and mainly consists of glucose (Glc), galactose (Gal), mannose (Man), fucose (Fuc), and xylose (Xyl) molecules linked by α-1,4- and α-1,6-glycosidic chains.
MP is an important component of myofibrils, and ~85% of water is retained in the MP structure. Research evidence has shown that freezing causes disruption of secondary bonds that stabilize the MP structure. Thus, the hydrophobic groups of MP are exposed and interact with adjacent protein regions, resulting in MP aggregation denaturation and decreased water retention. As a macromolecule polysaccharide, FVP may also form a glassy system, in which the water in the tissues reaches the freezing concentration state maximally, and the migration rate of water molecules is greatly reduced, which can effectively avoid aggregation denaturation and ice recrystallization (50).
The gel strength can well reflect the ability of proteins to form a gel, which is a primary index to evaluate the quality of surimi products. During the frozen storage process, trimethylamine demethylase is released into muscle tissue, then oxidizes trimethylamine to form formaldehyde, which always destroys the integrity of myosin (51), thus leading to the decrease of gel ability of surimi protein. On the other hand, the oxidation of fat during frozen storage also adversely affects the structure and function of surimi protein, leading to a decrease in surimi gel strength. The hydroxyl groups in the FVP can replace the water binding site and form strong hydrogen bonds with the polar residues of protein molecules to stabilize the protein structure of surimi, thus effectively improving the gel strength of surimi and slowing down the decrease in gel strength during frozen storage.
The FVP is easily expanded by heat after absorbing water and then fills in the gaps of the gel network structure in surimi, making the surimi gel structure denser. The expanded FVP will exert a certain pressure on the surimi protein, and the surimi network structure will become stronger. Electron microscopy images showed that the microstructure of surimi gel had a flat and smooth surface and a tight network structure, which was because FVP molecules would improve the gel properties by filling gaps between proteins and interacting with proteins to improve the compactness and denseness of the gel structure.
5. Conclusion
The research suggests that, as a novel cryoprotectant, FVP can significantly enhance the quality of surimi products while avoiding an excessively sweet taste and high-calorie value. Subsequently, the frozen storage of surimi indicated that adding FVP could alleviate the decline in MP solubility, Ca2+-ATPase activity, total sulfhydryl content, and water-holding capacity of catfish surimi. In addition, the addition of FVP could inhibit the increase in TBARS values, improve the rheological properties of surimi gel, and enhance the gel strength of surimi, and the microstructure showed that the gel structure of surimi with the addition of FVP was denser. Using the above indicators, 2% FVP effectively mitigated the protein denaturation of catfish surimi during frozen storage. Therefore, FVP is expected to be used as a new type of cryoprotectant in the frozen storage of surimi products.
Data availability statement
The raw data supporting the conclusions of this article will be made available by the authors, without undue reservation.
Author contributions
LL: Conceptualization, Data curation, Resources, Validation, Writing—original draft, Writing—review and editing. YL: Conceptualization, Writing—review and editing. XZ: Investigation, Writing—review and editing. TA: Writing—review and editing. MS: Writing—review and editing. MYS: Writing—review and editing. YW: Writing—review and editing. CC: Writing—review and editing. YZ: Funding acquisition, Project administration, Supervision, Writing—review and editing.
Funding
The author(s) declare financial support was received for the research, authorship, and/or publication of this article. This research was funded by the National Key Research and Development Program of China (Grant No. 2021YFD1901111), the Science and Technology Major Project of Shanxi Province, China (Grant No. 202101140601026), The Modern Agro-industry Technology Research System in Shanxi Province (Grant No. 2022HX080), the Special and Excellent Agricultural High-quality Development Science and Technology Support Project of the Shanxi Agricultural University in 2022 (Grant No. TYGC-28), and the Major Agricultural Technology Collaborative Promotion Project of Shanxi Province (Grant No. 2023XTTG01).
Acknowledgments
The authors thank BoRui Saccharide Biotech (YZ) Co. Ltd. for the molecular determination of polysaccharides.
Conflict of interest
The authors declare that the research was conducted in the absence of any commercial or financial relationships that could be construed as a potential conflict of interest.
Publisher's note
All claims expressed in this article are solely those of the authors and do not necessarily represent those of their affiliated organizations, or those of the publisher, the editors and the reviewers. Any product that may be evaluated in this article, or claim that may be made by its manufacturer, is not guaranteed or endorsed by the publisher.
References
1. Park SH, Lee HJ, Yoon IS, Lee G-W, Kim J-S, Heu MS. Protein functionality of concentrates prepared from yellowfin tuna (Thunnus albacares) roe by cook-dried process. Food Sci Biotechnol. (2016) 25:1569–75. doi: 10.1007/s10068-016-0242-0
2. Sun Q, Kong B, Liu S, Zheng O, Zhang C. Ultrasound-assisted thawing accelerates the thawing of common carp (Cyprinus carpio) and improves its muscle quality. LWT. (2021) 141:111080. doi: 10.1016/j.lwt.2021.111080
3. Floor E, Maples AM, Rankin CA, Yaganti VM, Shank SS, Nichols GS, et al. A one-carbon modification of protein lysine associated with elevated oxidative stress in human substantia nigra. J Neurochem. (2006) 97:504–14. doi: 10.1111/j.1471-4159.2006.03768.x
4. Thompson LV, Durand D, Fugere NA, Ferrington DA. Myosin and actin expression and oxidation in aging muscle. J Appl Physiol. (2006) 101:1581–7. doi: 10.1152/japplphysiol.00426.2006
5. Adeyemi KD, Sabow AB, Shittu RM, Karim R, Karsani SA, Sazili AQ. Impact of chill storage on antioxidant status, lipid and protein oxidation, color, drip loss and fatty acids of semimembranosus muscle in goats. CyTA-Journal of Food. (2016) 14:405–14. doi: 10.1080/19476337.2015.1114974
6. Lund M. N, Hviid M S, Claudi-Magnussen C, Skibsted L H. Effects of dietary soybean oil on lipid and protein oxidation in pork patties during chill storage. Meat Sci. (2008) 79:727–33. doi: 10.1016/j.meatsci.2007.11.008
7. Xie J, Yan Y, Pan QN, et al. Effect of frozen time on Ctenopharyngodon idella surimi: With emphasis on protein denaturation by Tri-step spectroscopy. J Mol Struct. (2020) 1217:128421. doi: 10.1016/j.molstruc.2020.128421
8. Wang B, Kong B, Li F, Liu Q, Zhang H, Xia X. Changes in the thermal stability and structure of protein from porcine longissimus dorsi induced by different thawing methods. Food Chem. (2020) 316:126375. doi: 10.1016/j.foodchem.2020.126375
9. Chen X, Wu J, Li X, Yang F, Yu L, Li X, et al. Investigation of the cryoprotective mechanism and effect on quality characteristics of surimi during freezing storage by antifreeze peptides. Food Chem. (2022) 371:131054. doi: 10.1007/978-981-19-4796-4
10. Abe S, Asada T, Kajiwara K. Effects of freeze-thaw cycles on gel-forming ability and protein denaturation in Alaska pollock frozen surimi. J Food Qual. (2019) 2019:368. doi: 10.1155/2019/3760368
11. Zhou F, Zhao M, Zhao H, Sun W, Cui C. Effects of oxidative modification on gel properties of isolated porcine myofibrillar protein by peroxyl radicals. Meat Sci. (2014) 96:1432–9. doi: 10.1016/j.meatsci.2013.12.001
12. Santana P, Zilda D, Huda, N. Physicochemical properties of surimi powder made fromthreadfin bream (Nemipterus Japonicus) with various dryoprotectants added. J Fundament Appl Sci. (2017) 9:866–74. doi: 10.4314/jfas.v9i2s.54
13. Wang Y, Zhang H. Advances in the extraction, purification, structural-property relationships and bioactive molecular mechanism of Flammulina velutipes polysaccharides: a review. Int J Biol Macromol. (2021) 167:528–38. doi: 10.1016/j.ijbiomac.2020.11.208
14. Zhou A, Benjakul S, Pan K, Gong J, Liu X. Cryoprotective effects of trehalose and sodium lactate on tilapia(Sarotherodon nilotica) surimi during frozen storage. Food Chem. (2006) 96:96–103. doi: 10.1016/j.foodchem.2005.02.013
15. Nopianti R, Huda N, Noryati I, Fazilah A, Easa AM. Cryoprotective effect of low-sweetness additives on protein denaturation of threadfin bream surimi (Nemipterus spp) during frozen storage. CyTA J Food. (2012) 10:243–50. doi: 10.1080/19476337.2011.639033
16. Leung MYK, Fung KP, Choy YM. The isolation and characterization of an immunomodulatory and anti-tumor polysaccharide preparation from Flammulina velutipes. Immunopharmacology. (1997) 35:255–63. doi: 10.1016/S0162-3109(96)00157-9
17. Liu Y, Zhang B, Ibrahim SA, et al. Purification, characterization and antioxidant activity of polysaccharides from Flammulina velutipes residue. Carbohydr Polym. (2016) 145:71–7. doi: 10.1016/j.carbpol.2016.03.020
18. Yang W, Pei F, Shi Y, et al. Purification, characterization and anti-proliferation activity of polysaccharides from Flammulina velutipes. Carbohydr Polym. (2012) 88:474–80. doi: 10.1016/j.carbpol.2011.12.018
19. Zhang Z, Lv G, He W, Shi L, Pan H, Fan L. Effects of extraction methods on the antioxidant activities of polysaccharides obtained from Flammulina velutipes. Carbohydr Polym. (2013) 98:1524–31. doi: 10.1016/j.carbpol.2013.07.052
20. Zheng Y, Shi Y, Yang X, Guo Q. Flammulina velutipes polysaccharide improves the water-holding capacity in the dorsal muscle of freeze-thawed cultured large yellow croaker (Larimichthys crocea). Food Chem. (2023) 403:134401. doi: 10.1016/j.foodchem.2022.134401
21. Qin L, Fu Y, Yang F, Chang Z, Zou C, Gao H, et al. Effect of Flammulina velutipes polysaccharide on the quality of salmon fillets during frozen storage. Food Ferment Ind. (2021) 47:178–83. doi: 10.13995/j.cnki.11-1802/ts.027123
22. Zhu W, Guo H, Han M, Shan C, Bu Y, Li J, et al. Evaluating the effects of nanoparticles combined ultrasonic-microwave thawing on water holding capacity, oxidation, and protein conformation in jumbo squid (Dosidicus gigas) mantles. Food Chem. (2023) 402:134250. doi: 10.1016/j.foodchem.2022.134250
23. Cai L, Nian L, Cao A, Zhang Y, Li X. Effect of carboxymethyl chitosan magnetic nanoparticles plus herring antifreeze protein on conformation and oxidation of myofibrillar protein from red sea bream (Pagrosomus major) after freeze-thaw treatment. Food Bioproc Technol. (2020) 13:355–66. doi: 10.1007/s11947-019-02384-x
24. Levine RL, Wehr N, Williams JA, Stadtman ER, Shacter E. Determination of carbonyl groups in oxidized proteins. Meth Mol Biol. (2000) 99:15–24. doi: 10.1385/1-59259-054-3:15
25. Xia X, Kong B, Liu Q, Liu J. Physicochemical change and protein oxidation in porcine longissimus dorsi as influenced by different freeze-thaw cycles. Meat Sci. (2009) 83:239–45 doi: 10.1016/j.meatsci.2009.05.003
26. Yan W, Yin T, Xiong S, You J, Hu Y, Huang Q. Gelling properties of silver carp surimi incorporated with konjac glucomannan: effects of deacetylation degree. Int J Biol Macromol. (2021) 191:925–33. doi: 10.1016/j.ijbiomac.2021.09.167
27. Guan W, Ren X, Li Y, Mao L. The beneficial effects of grape seed, sage and oregano extracts on the quality and volatile flavor component of hairtail fish balls during cold storage at 4°C. LWT. (2019) 101:25–31. doi: 10.1016/j.lwt.2018.11.024
28. Nopianti R, Huda N, Ismail N, Ariffin F, Easa AM. Effect of polydextrose on physicochemical properties of threadfin bream (Nemipterus spp) surimi during frozen storage. J Food Sci Technol. (2013) 50:739–46. doi: 10.1007/s13197-011-0394-0
29. Cai L, Wan J, Li X, Li J. Effects of different thawing methods on conformation and oxidation of myofibrillar protein from largemouth bass (Micropterus salmoides). J Food Sci. (2020) 85:2470–80. doi: 10.1111/1750-3841.15336
30. Zhu M, Zhang J, Peng Z, Kang Z, Ma H, Zhao S, et al. Fluctuated low temperature combined with high-humidity thawing to retain the physicochemical properties and structure of myofibrillar proteins from porcine longissimus dorsi. LWT. (2021) 142:111001. doi: 10.1016/j.lwt.2021.111001
31. Zhang X, Zhang Y, Dong Y, Ding H, Chen K, Lu T, et al. Study on the mechanism of protein hydrolysate delaying quality deterioration of frozen surimi. LWT. (2022) 167:113767. doi: 10.1016/j.lwt.2022.113767
32. Zhao Y, Kong H, Zhang X, Hu X, Wang M. The effect of Perilla (Perilla frutescens) leaf extracts on the quality of surimi fish balls. Food Science & Nutrition. (2019) 7:2083–90. doi: 10.1002/fsn3.1049
33. Sultanbawa Y, Li-Chan ECY. Structural changes in natural actomyosin and surimi from ling cod (Ophiodon elongatus) during frozen storage in the absence or presence of cryoprotectants. J Agric Food Chem. (2001) 49:4716–25. doi: 10.1021/jf001281x
34. Gao W, Huang Y, Zeng X-A, Brennan MA. Effect of soluble soybean polysaccharides on freeze-denaturation and structure of myofibrillar protein of bighead carp surimi with liquid nitrogen freezing. Int J Biol Macromol. (2019) 135:839–44. doi: 10.1016/j.ijbiomac.2019.05.186
35. Dey SS, Dora KC. Suitability of chitosan as cryoprotectant on croaker fish (Johnius gangeticus) surimi during frozen storage. J Food Sci Technol. (2011) 48:699–705. doi: 10.1007/s13197-010-0197-8
36. Jiang L, Wu S. Pullulan suppresses the denaturation of myofibrillar protein of grass carp (Ctenopharyngodon idella) during frozen storage. Int J Biol Macromol. (2018) 112:1171–4. doi: 10.1016/j.ijbiomac.2018.02.094
37. James C, Purnell G, James SJ. A review of novel and innovative food freezing technologies. Food Bioproc Technol. (2015) 8:1616–34. doi: 10.1007/s11947-015-1542-8
38. Qin L, Fu Y, Yang F, et al. Effects of polysaccharides autoclave extracted from Flammulina velutipes mycelium on freeze-thaw stability of surimi gels. LWT. (2022) 169:113941. doi: 10.1016/j.lwt.2022.113941
39. Gao W, Hou R, Zeng X. Synergistic effects of ultrasound and soluble soybean polysaccharide on frozen surimi from grass carp. J Food Eng. (2019) 240:1–8. doi: 10.1016/j.jfoodeng.2018.07.003
40. Zhao X, Wu T, Xing T, et al. Rheological and physical properties of O/W protein emulsions stabilized by isoelectric solubilization/precipitation isolated protein: The underlying effects of varying protein concentrations. Food Hydrocoll. (2019) 95:580–9. doi: 10.1016/j.foodhyd.2018.03.040
41. Chai JM. Physicochemical changes of tilapia (Oreochromis niloticus) muscle during salting. Food Chem. (2011) 129:1201–10. doi: 10.1016/j.foodchem.2011.05.110
42. Tang S, Feng G, Cui W, Gao R, Bai F, Wang J, et al. Effect of α-Tocopherol on the physicochemical properties of sturgeon surimi during frozen storage. Molecules. (2019) 24:710. doi: 10.3390/molecules24040710
43. Walayat N, Tang W, Nawaz A, Ding Y, Liu J, Lorenzo JM. Influence of Konjac oligo-glucomannan as cryoprotectant on physicochemical and structural properties of silver carp surimi during fluctuated frozen storage. LWT. (2022) 3:113641. doi: 10.1016/j.lwt.2022.113641
44. Liu J, Fang C, Luo Y, Ding Y, Liu S. Effects of konjac oligo-glucomannan on the physicochemical properties of frozen surimi from red gurnard (Aspitrigla cuculus). Food Hydrocoll. (2019) 89:668–73. doi: 10.1016/j.foodhyd.2018.10.056
45. Guo X, Shi L, Xiong S, Hu Y, You J, Huang Q, et al. Gelling properties of vacuum-freeze dried surimi powder as influenced by heating method and microbial transglutaminase. LWT. (2018) 99:105–11. doi: 10.1016/j.lwt.2018.09.050
46. Cao G, Chen X, Wang N, Tian J, Song S, Wu X, et al. Effect of konjac glucomannan with different viscosities on the quality of surimi-wheat dough and noodles. Int J Biol Macromol. (2022) 221:1228–37. doi: 10.1016/j.ijbiomac.2022.09.024
47. Alakhrash F, Anyanwu U, Tahergorabi R. Physicochemical properties of Alaska pollock (Theragra chalcograma) surimi gels with oat bran, LWT-FoodSci. Technol. (2016) 66:41–7. doi: 10.1016/j.lwt.2015.10.015
48. Hur SJ, Ye BW, Lee JL, Ha YL, Park GB, Joo ST. Effects of conjugated linoleic acid on color and lipid oxidation of beef patties during cold storage. Meat Sci. (2004) 66:771–5. doi: 10.1016/S0309-1740(03)00104-9
49. Zhuang X, Jiang X, Zhou H, Chen Y, Zhao Y, Yang H, et al. Insight into the mechanism of physicochemical influence by three polysaccharides on myofibrillar protein gelation. Carbohydr Polym. (2020) 229:115449. doi: 10.1016/j.carbpol.2019.115449
50. Sei T, Gonda T, Arima Y. Growth rate and morphology of ice crystals growing in a solution of trehalose and water. J Cryst Growth. (2002) 240:218–29. doi: 10.1016/S0022-0248(02)00875-8
Keywords: Flammulina velutipes polysaccharides, surimi, frozen storage, oxidation, cryoprotectant, myofibrillar protein
Citation: Ling L, Liu Y, Zhang X, Aziz T, Shahzad M, Sameeh MY, Wang Y, Cai C and Zhu Y (2023) Effect of Flammulina velutipes polysaccharides on the physicochemical properties of catfish surimi and myofibrillar protein oxidation during frozen storage. Front. Nutr. 10:1268580. doi: 10.3389/fnut.2023.1268580
Received: 28 July 2023; Accepted: 29 August 2023;
Published: 25 September 2023.
Edited by:
Ahmed A. Zaky, National Research Centre, EgyptReviewed by:
Mahmood A. Hashim, University of Helsinki, FinlandJin Guofeng, Beijing Technology and Business University, China
Copyright © 2023 Ling, Liu, Zhang, Aziz, Shahzad, Sameeh, Wang, Cai and Zhu. This is an open-access article distributed under the terms of the Creative Commons Attribution License (CC BY). The use, distribution or reproduction in other forums is permitted, provided the original author(s) and the copyright owner(s) are credited and that the original publication in this journal is cited, in accordance with accepted academic practice. No use, distribution or reproduction is permitted which does not comply with these terms.
*Correspondence: Yingchun Zhu, eWluZ2NodW4wNDE3QDE2My5jb20=