- College of Life Science and Food Engineering, Inner Mongolia Minzu University, Tongliao, Inner Mongolia, China
Koumiss, a traditional fermented dairy product made from fresh mare milk, is a sour beverage that contains an abundance of microbial communities, including lactic acid bacteria, yeast and others. Firstly, probiotics such as Lacticaseibacillus in koumiss can induce the secretion of immunoglobulin G in serum and interleukin-2 in the spleen while beneficial Saccharomyces can secrete antibacterial compounds such as citric acid and ascorbic acid for specific immunopotentiation. Additionally, more isoflavone in koumiss can regulate estrogen levels by binding to its receptors to prevent breast cancer directly. Bile salts can be converted into bile acids such as taurine or glycine by lactic acid bacteria to lower cholesterol levels in vivo. Butyric acid secretion would be increased to improve chronic gastrotis by regulating intestinal flora with lactic acid bacteria. Finally, SCFA and lCFA produced by Lacticaseibacillus inhibit the reproduction of pathogenic microorganisms for diarrhea prevention. Therefore, exploring the mechanisms underlying multiple physiological functions through utilizing microbial resources in koumiss represents promising avenues for ameliorating chronic diseases.
Introduction
Koumiss, with a long history, is one of the favored traditional dairy products among nomadic people living in the grasslands of northern China. It is a fermented beverage and is also known as kumiss, chigo, chige, arrag, or airag. It has an acidic-alcoholic profile, a milky white appearance, and a subtle aroma and flavor. Koumiss is usually fermented naturally using traditional methods with fresh mare milk and can provide abundant nutrients for drinkers from June to September in Inner Mongolia, Xinjiang, Tibet, and other provinces in China (1–3). Traditional methods of making koumiss using natural fermentation are described here. Fresh mare milk is filtered and cooled to approximately 20°C; it is subsequently poured into a wooden barrel, leather bag, or plastic barrel that contains some koumiss residue as a fermentation starter. Next, a wooden stick is used to stir the fresh mare milk violently to form a mixture that then ferments naturally at ambient temperature for 1–3 days (4). The yeast, lactic acid bacteria, other microorganisms, and alcohol content are determined by fermentation time. Notably, the stirring operation is very important for homogenization during fermentation to allow for the more uniform distribution of acidity and to break casein clots in the gel formation of proteins. Finally, koumiss with good consistency and structure, as well as delicate taste, are obtained (5, 6). Therefore, the short shelf life of koumiss, only 3–5 days, coupled with the relatively low milk output of mares, has restricted opportunities for large-scale industrial production and consumer acceptance. However, the complex microbial communities present in koumiss, including benificial lactic acid bacteria, yeast, etc., have been found to help regulate gut microbiota, metabolites, and host immunity (5, 7). Koumiss also possesses preventive and therapeutic properties against chronic diseases such as pulmonary tuberculosis, hyperlipidemia, and cardiovascular and cerebrovascular diseases (5, 8, 9). At the same time, some potential harmful bacteria still survives in the fermentated koumiss.
Nutritional ingredients of koumiss
Fresh mare milk
Fresh mare milk with particular composition, hypoallergenicity, and nutraceutical properties (10), the raw material of koumiss, contains many nutrients essential for the human body. These include proteins, fats, lactose, galactose, vitamins, enzymes, and minerals, etc. (Table 1). The nutrients in mare milk are comparable to those found in human breast milk (HBM) and can be used as a substitute for infant formula (1, 11). Additionally, the contents of essential amino acids, unsaturated fatty acids, and lactose which can promote calcium absorption among humans, are greater in mare milk than in other domestic animal milks (12). Furthermore, fresh mare milk is more easily digested and absorbed by the human body. This is because digestible whey protein constitutes approximately 40% of the total protein, more than twice as much as found in cow milk. The indigestible casein is also relatively lower in contents (13). The lower fat content and smaller fat droplets in fresh mare milk are more easily absorbed by the human body (14, 15).
Fresh mare milk is rich in vitamins C, A, E, D, B1, B2, B12, and calcium, phosphorus, and other mineral elements, of which the ratio of calcium and phosphorus is 2:1 which is very similar to HBM (16). Mare milk also contains large numbers of essential trace elements such as Zn, Cu, V, Cr, Ni, Co, Mo, and other elements not essential but beneficial to the human body, such as Sr., Rb, Ba, and Li (11). An additional twelve rare elements that positively affect the human body have also been found in mare milk. The contents of rare elements from high to low are Sc, Ce, Nd, La, Y, Sm, Eu, Pr, Gd, Yb, Er, and Ho (11). Additionally, the content of lysozyme in mare milk is twice that of HBM and promotes recovery from gastric ulcers and upper respiratory infections, as well as healing wounds and postoperative scars. The combination of lysozyme and lactoferrin in mare milk is also a type of natural anti-infection substance with bactericidal effects (5, 17, 18).
Koumiss
The abundant nutrients and bioactive compounds found in koumiss are also metabolized by probiotic lactic acid bacteria, yeast, and other microorganisms in addition to those found in fresh mare milk. Unique tastes and high nutritional values in koumiss are the results of enzyme catalysis by lactic acid bacteria and yeast, such as the metabolism of carbohydrates and amino acids and the biosynthesis of fatty acids, which all benefit the pancreas and promote digestion (11, 12, 19). Gastrointestinal motility disorders can also be relieved because the acid amines and peptones converted from casein and albumin in koumiss can be absorbed easily and quickly by patients (13). Whey proteins rather than caseins are major proteins in the koumiss (20). Additionally, a variety of volatile organic compounds in koumiss, including lactic acid, ethanol, carbon dioxide, and other substances converted from lactose by lactic acid bacteria and yeast would prove beneficial for consumers who are lactose intolerant (Table 1) (3, 12). Essential fatty acids, such as α-linoleic acid and linolenic acid, are higher in koumiss than in other unsaturated fatty acids and can also be produced through natural fermentation (21).
Furthermore, organic acids, aromatic compounds, extracellular polysaccharides, peptides, enzymes, bacteriocins, and other special nutrients are also produced during natural fermentation. The organic acids and bacteriocins inhibit harmful microorganisms, and bioactive peptides have excellent anticancer and antioxidant properties (5, 12). Therefore, the high nutritional values and rich functional components identified in koumiss are primarily the results of the original fresh mare milk and microbiological metabolites found in it (Table 1) (11, 22).
Prevention of chronic diseases by drinking koumiss
Koumiss can effectively improve specific immunopotentiation
Numerous probiotics found in koumiss, especially lactic acid bacteria, can directly regulate the intestinal flora balance and microenvironment. As a result, the probiotics and their metabolites can improve specific immunopotentiation (23–25). Lacticaseibacillus casei Zhang isolated from koumiss can effectively inhibit the inflammatory response caused by polyinosinic:polycytidylic acid (Poly I:C). Poly I:C is often used as an analog double-stranded RNA (dsRNA) virus to simulate the viral infection process in macrophage cells (RAW264.7) (26). Research has shown that L. casei Zhang in koumiss can prevent or mitigate inflammatory reactions by reducing the amount of tumor necrosis factor-α (TNF-α) in serum (27, 28). Additionally, L. casei Zhang contributes to immunopotentiation by increasing the level of total immunoglobulin G (IgG) in serum and the content of interleukin-2 (IL-2) in the spleen. This induces the activation of T and B lymphocytes, enhances NK cell activity, and increases the activity of monocytes and macrophages against tumor cells or bacteria (Figure 1) (29–31). L. casei Zhang can stimulate the activation of T helper cells and NK cells, which then secrete the proinflammatory cytokine interferon γ (IFN-γ). IFN-γ can bind to the transmembrane glycoprotein receptor IFN-γR, inducing macrophage cells and T lymphocytes to flow to the inflammatory site and enhance immune reactions (28, 32, 33). Another strain isolated from koumiss, Lactobacillus acidophilus NCFM, can increase the murine dendritic cell expression of interferon β (IFN-β), interleukin-12 (IL-12), and interleukin-10 (IL-10). These observations demonstrate the strong antiviral ability of koumiss (26, 34).
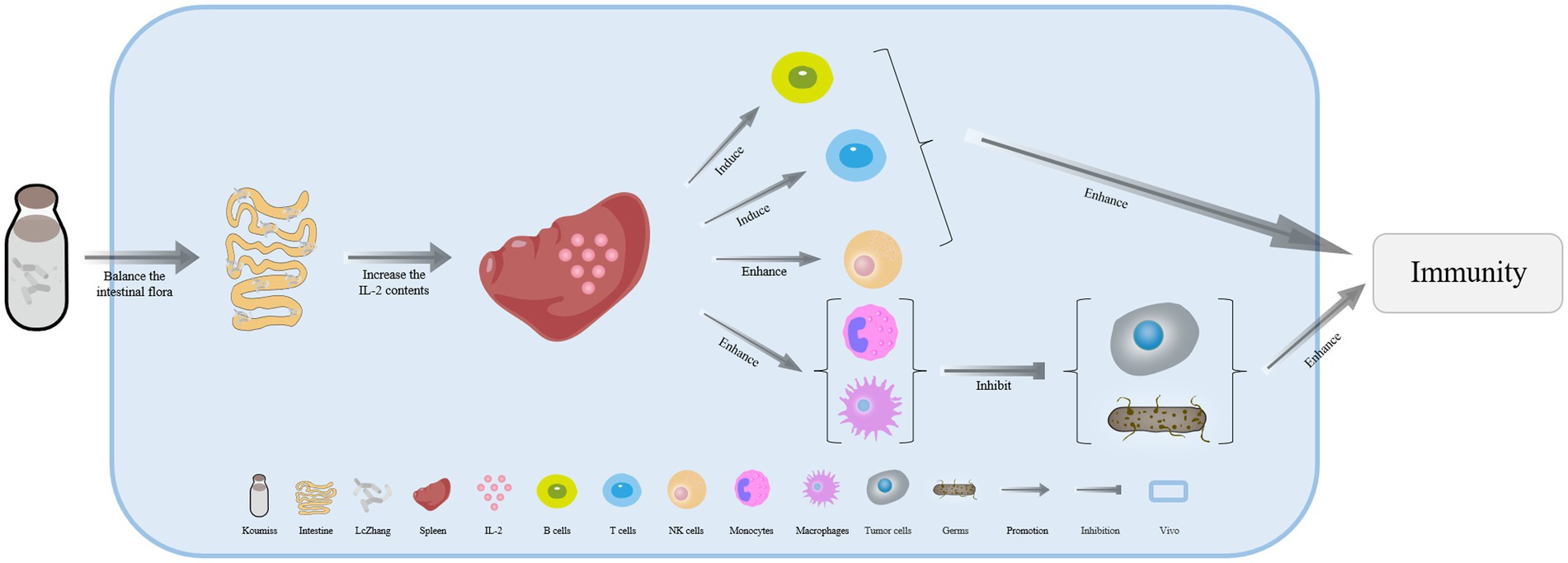
Figure 1. The mechanism of immunopotentiation in various ways with koumiss. Drinking koumiss with L. casei Zhang can induce the secretion of interleukin-2 (IL-2) in the spleen, which in turn increases the activation of T and B lymphocytes, enhances NK cell activity, and ultimately enhances immunopotentiation. Furthermore, the increased content of IL-2 in the spleen enhances the activation of monocytes and macrophages against tumor cells and bacteria.
Drinking koumiss which contains L. casei Zhang can promote the transcription of toll-like receptor 3 (TLR3) for double-stranded ribonucleic acid (dsRNA) in the pattern recognition receptor family. This is located on the surface of epithelial cells. Consequently, TLR3 upregulates the expression of proinflammatory factors by recognizing ligands and activates the nuclear factor kappa B (NF-κB) signaling pathway, thereby effectively promoting immune responses (26, 35). Additionally, the transcription of the immune receptor TLR9 by L. casei Zhang present in koumiss can activate plasmacytoid dendritic cells (pDCs) and B lymphocytes, thus enhancing immunological effects when the human body is stimulated by harmful bacteria carrying common unmethylated CpG motifs in their genomes (26, 36, 37).
Consuming koumiss probiotics has been shown to increase the expression of peroxisome proliferator-activated receptor α/β/δ (PPAR-α/β/δ) in mice kidney proximal tubule cells, thereby enhancing immune responses (38, 39). Among them, fatty acids β-oxidation (FAO) in renal proximal tubules is promoted by high-expression of PPAR-α in acute kidney injury (AKI) disease induced by cisplatin (CP) or ischemia/reperfusion (I/R). Thus, the lipoperoxides, including 4-hydroxy-2-hexenal, would be reduced by probiotics in koumiss, which help alleviate or hinder oxidative stress-mediated AKI. This also prevents cell apoptosis and proximal tubular cell death from occurring (40–42). Therefore, probiotics in koumiss can prevent kidney damage and protect kidney function (43, 44).
Saccharomyces cerevisiae isolated from koumiss can secrete antibacterial compounds, the main ingredients of which include citric acid, ascorbic acid, lactic acid, malic acid, and killer toxins (45, 46). These antibacterial compounds can effectively increase the concentration of immunoglobulin A (IgA), which can rapidly initiate the humoral immune response, and increase the number of beneficial bacteria while reducing the number of harmful bacteria or viruses in the gastrointestinal tract, together strengthening the resistance to various diseases (47–49). These antibacterial compounds in koumiss can also increase the concentrations of CD3+ and mature T lymphocytes in peripheral lymphoid organs to enhance the immune function of lymphoid organs or can decrease the concentrations of CD8+ in T lymphocytes to regulate the ratio of CD4+/CD8+ (50, 51). Humoral immunity is increased by antibacterial compounds and would be increased when infected by Escherichia coli O8 (51, 52). The antibacterial compounds can increase the numbers of Bifidobacterium and inhibit extraintestinal pathogenic E. coli in the cecum. Additionally, they can regulate the pH of the intestine and inhibit harmful bacteria by producing acetic acid. This helps maintain a normal microflora structure in the cecum, promoting physical health and preventing various diseases (51). Therefore, koumiss consumption can effectively strengthen the body’s immunocompetence and anti-inflammatory responses.
Koumiss can effectively reduce the occurrence of cancer
The probiotics found in koumiss have been shown to strengthen the immune system, suppress tumor growth, and prevent the accumulation of carcinogenic compounds. Lactic acid bacteria is considered the dominant probiotic involved in the process of koumiss fermentation (53, 54). Additionally, it has been found that lactic acid bacteria isolated from koumiss collected in Xinjiang, China effectively reduced the number of colon cancer HT-29 cells. When Limosilactobacillus reuteri (Lactobacillus reuteri) BCRC14625 (1.0 × 109 CFU/mL) was cultured together with HT-29 cells, there was a significant increase in lactate dehydrogenase (LDH) activity (p < 0.05), resulting in damage to the cell membrane. In contrast, L. reuteri BCRC14625 has been observed inducing HT-29 cancer cells to secrete nitric oxide (NO), leading to apoptosis of the cells. Therefore, koumiss may be both a potential preventive and therapeutic agent for treating colon cancer (55–57).
Long-term consumption of koumiss had been shown to reduce Bacteroides uniformis concentrations, which are responsible for degrading isoflavones in vivo (p = 0.016). This results in a greater concentration of isoflavones that can bind to estrogen receptors (ERα and ERβ) as estrogen-like compounds in patients. Therefore, the level of estrogen in the body can be regulated to prevent hormone-induced breast cancer and other cancers (Figure 2) (58–60). Drinking koumiss can potentially serve as an early predictor of gastric cancer, while also relieving the symptoms of chronic atrophic gastritis. Furthermore, consuming koumiss can significantly reduce blood platelet counts, which in turn, may prevent inflammation and cancer (60–63).
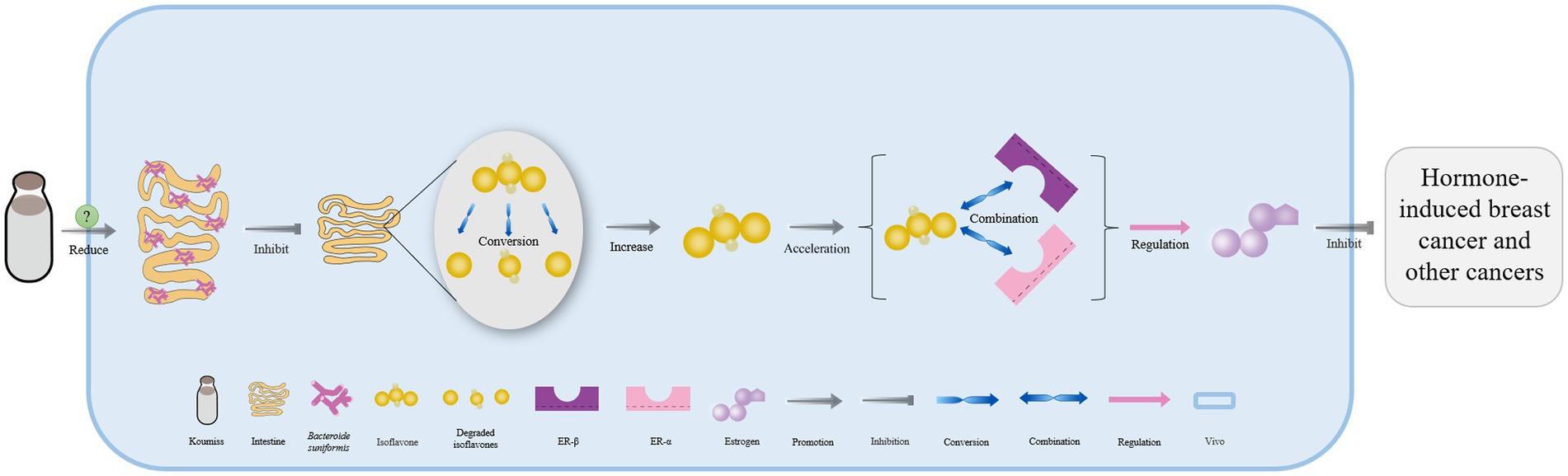
Figure 2. The mechanism of cancer prevention effectively with koumiss. Drinking koumiss can reduce the concentration of Bacteroides uniformis in vivo by some certain ways, which in turn inhibits the degradation of isoflavones in the intestine. This results in more isoflavones being available to accelerate binding to estrogen receptors (ER α and ER β) as estrogen-like compounds, directly regulating the level of estrogen in vivo to prevent hormone-induced breast cancer and other cancers.
Interferon γ (IFN- γ) levels can be increased greatly in vivo by L. casei Zhang isolated from koumiss (28). IFN-γ plays an important role in the antiproliferation of ovarian cancer, rectal cancer, and hepatocellular carcinoma. High doses of IFN-γ have been shown to promote the transcription and synthesis of protease caspase 3 and caspase 7. These activate the apoptosis process of cancer cells by initiating Janus kinase-signal transducers and activators of the transcription 1-caspase (JAK-STAT1-caspase) signal (32, 64, 65). Research has shown that IFN-γ can downregulate the expression of vascular endothelial growth factor A (VEGFA), which in turn disturbs the proliferation and survival of endothelial cells (66). This leads to blocking angiogenesis in the tumor microenvironment, ultimately preventing tumor growth. IFN-γ can also block the interleukin 8-chemokine receptor CXCR2 (CXCL8-CXCR2) axis, which prevents the timely transportation of CXCR2+ CD68+ immunosuppressive macrophages to the tumor microenvironment (TME). This enhances the therapeutic effects of programmed cell death protein 1 (PD-1) blockade therapy for pancreatic cancer (67).
Consuming koumiss can also significantly reduce the levels of lithocholic acid and bile acid in feces, which can help prevent intrahepatic cholestasis and reduce liver cancer incidence (68–71). These findings demonstrate that the probiotics found in koumiss should be effective in reducing the incidence rates of tumors and cancers.
Koumiss can effectively reduce in vivo cholesterol levels
The results of high throughput sequencing have shown that lactic acid bacteria is the dominant flora present in koumiss. Through assimilation of lactic acid bacteria, cholesterol in the cell membrane can be bound to the phospholipid tail, upper-phospholipid, and polar head regions, and thereby be converted to coprostanol and reduce cholesterol levels in vivo (60, 72–74). The binding of lactic acid bacteria with bile acids can inhibit its absorption by the small intestine and facilitate its excretion in feces. Endogenous cholesterol is then sequentially converted into bile acid, ultimately leading to a reduction in cholesterol levels (74, 75). Additionally, the contents of short-chain fatty acids (SCFAs) such as propionate and butyrate in the intestine increases in the presence of three lactic acid bacteria strains (Lactobacillus helveticus MG9-2, Lactiplantibacillus plantarum LIP-1, and Limosilactobacillus fermentum E7301) isolated from koumiss. The activity of pyruvate dehydrogenase in the liver is effectively restrained thereby leading to the inhibition of fatty acid synthesis and ultimately resulting in a reduction in the concentration of cholesterol in serum and the liver (74, 76). lactic acid bacteria in koumiss can also effectively increase high-density lipoprotein in the blood, which can reduce or block the flow of cholesterol into the liver (77, 78).
Lacticaseibacillus casei in koumiss collected from Xinjiang has been shown to secrete bile salt hydrolase, which catalyzes the deconjugation of bile salts in enterohepatic circulation (55). This leads to the release of glycine or taurine groups from the steroid’s core molecular structure, converting conjugated bile acid into free bile acid that is excreted in feces. As a result, the bile acid content in the body is reduced (Figure 3) (79, 80). Additionally, the expression of the farnesoid x receptor is significantly downregulated, while that of cholesterol 7-alpha hydroxylase (CYP7A1) is upregulated, both of which can accelerate the conversion of bile acid from endogenous cholesterol in the liver. This ultimately helps maintain normal metabolic activities (Figure 3) (81–83).
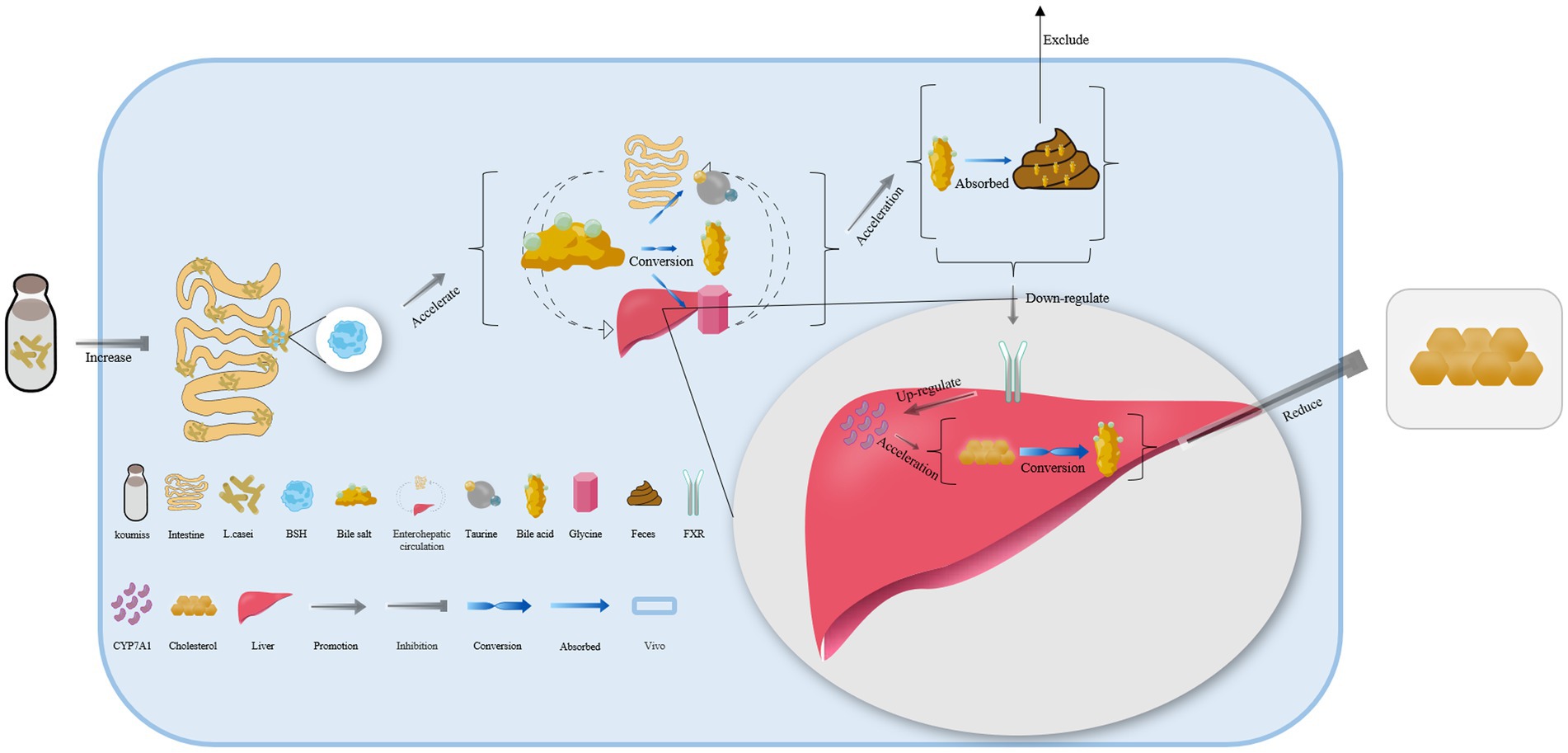
Figure 3. The mechanism of cholesterol content reduction with koumiss in vivo. Drinking koumiss can increase the content of bile salt hydrolase (BSH) secreted by Lacticaseibacillus casei, which can accelerate the conversion of bile salts into bile acid, taurine, or glycine in enterohepatic circulation. The excessive bile acid can be absorbed by the feces and excreted out of the body. Furthermore, the requirements of bile acid would down-regulate the expression level of farnesoid x receptor (FXR) and up-regulate CYP7A1, accelerating the conversion of cholesterol to bile acid. This, in turn, significantly lowers the content of cholesterol levels in vivo.
A total of seven metabolites naturally found in koumiss have been identified in the feces of patients with hyperlipidemia who had been drinking koumiss for an extended period. These metabolites include stearic acid, sphingosine, tyrosine, α-tocotrienol, γ-tocotrienol, butyric acid, and butyrate (84). Stearic acid, a saturated fatty acid, can increase the oxidation rate of low-density lipoprotein (LDL) cholesterol in the blood after being converted to oleic acid (85). Sphingosine is bound to cholesterol to form a complex that can limit intestinal cholesterol absorption. Sphingosine can also attenuate the affinity of transporter Niemann Pick C1 like 1 (NPC1L1) toward cholesterol (86). Additionally, tyrosine can be converted to adrenaline, which further promotes the process of lipid hydrolysis in vivo (87), and α-tocotrienol and γ-tocotrienol downregulate the expression of HMG CoA reductase to inhibit the synthesis of endogenous cholesterol and linoleic acid promotes β-oxidation, thus reducing the synthesis of endogenous triglycerides (TGs). Butyrate accelerates the hydrolysis of fatty acids, leading to a reduction in the concentration of TGs in the blood, and can also block the transportation of Very Low-Density Lipoprotein Cholesterol (VLDL-C) from the liver to the blood (84). Therefore, consuming koumiss can help reduce cholesterol through multiple processes.
Koumiss treats chronic gastritis and regulates intestinal flora
Koumiss is a functional drink that can be used to treat chronic gastritis. Studies have shown that when mice consumed koumiss, their intestinal numbers of Eubacterium rectale and Faecalibacterium prausnitzii significantly increased (60). These two intestinal microorganisms secrete butyric acid, which can regulate intestinal flora disorders and effectively promote gut content isolation and hypodermis among intestinal epithelial cells, thus stabilizing colon cells. These actions can inhibit the growth of cancer cells and reduce inflammation, making koumiss an effective treatment for chronic gastritis caused by Prevotella copri (enterotype 2) in the gut (Figure 4) (60, 88).
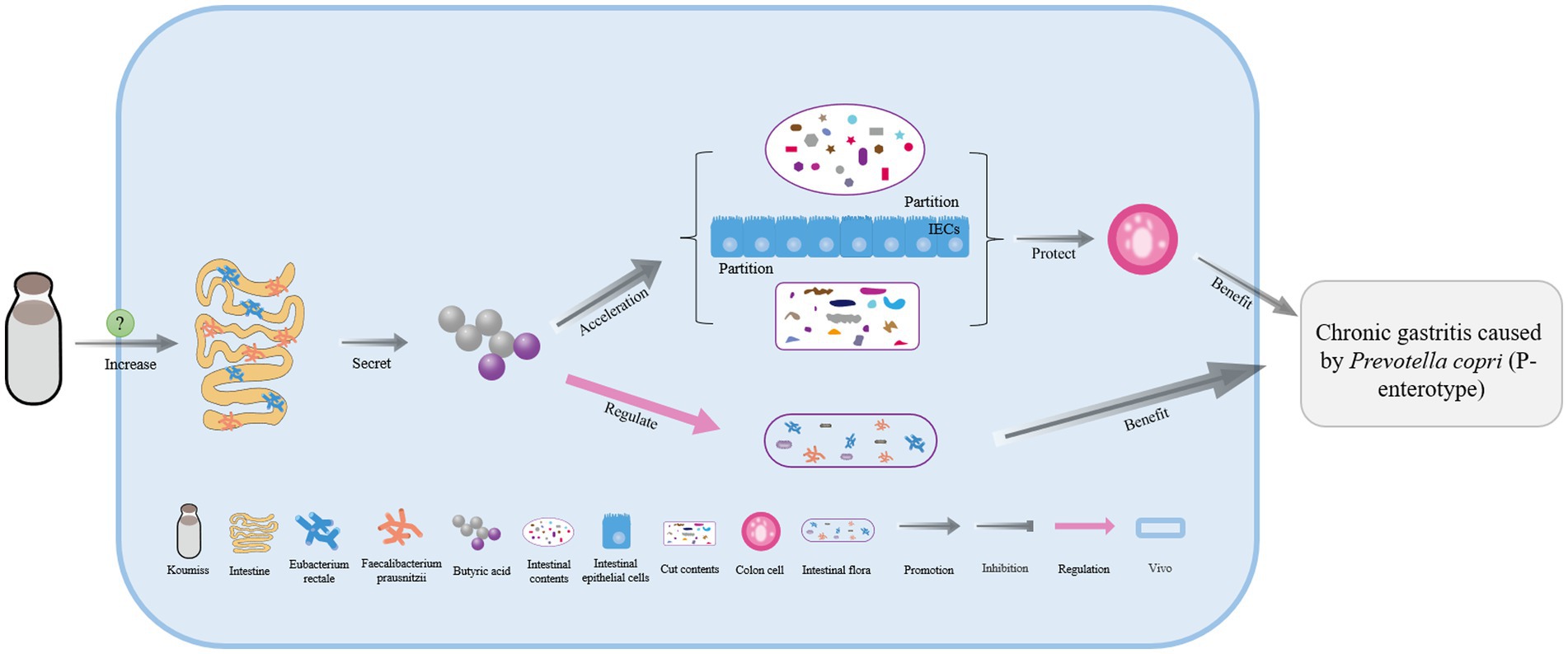
Figure 4. The mechanism of chronic gastrotis improvement with koumiss. Drinking koumiss can significantly increase the numbers of Eubacterium rectale and Faecalibacterium prausnitzii in the intestine by some certain ways, which can secrete more butyric acid to regulate the intestinal flora and help the intestinal and gut contents separated by intestinal epithelial cells (IECs) and then protect the colonic epithelial cells. As a result, chronic gastritis caused by Prevotella copri (P-enterotype) can be effectively treated.
Koumiss had also been shown to be effective in treating gastrointestinal inflammation caused by Salmonella typhimurium ATCC 14028 (89). Consuming koumiss can boost the relative abundance of Akkermania muciniphila and Lachnospiraceae in the intestine. Autonomic control of systemic glucose metabolism and energy balance of A. muciniphila can inhibit the growth and reproduction of Toxoplasma gondii in the intestinal tract. SCFAs produced by Lachnospiraceae not only stimulated the proliferation of colon epithelial cells and reduce colonic transit time but also provide nutrients for the colon mucosa, preventing mucosal atrophy. By maintaining primal gastrointestinal morphology and normal physiological functioning, koumiss provides an effective treatment option (90–92).
Other health functions of koumiss
A large number of Lacticaseibacillus paracasei and abundant paracaseins are also found in koumiss (Figure 5). Some substances, such as short-chain or long-chain fatty acids produced by L. paracasei can effectively inhibit the reproduction of pathogenic microorganisms (Figure 5). Paracaseins can induce endogenous mucin secretion, promote the expression of tight junction proteins, and inhibit the transmission of the NF-kB MLCK signal pathways (Figure 5). As a result, the intestinal damage caused by E. coli can be reduced and the intestinal mucosal barrier can be strengthened to effectively treat diarrhea (Figure 5) (93).
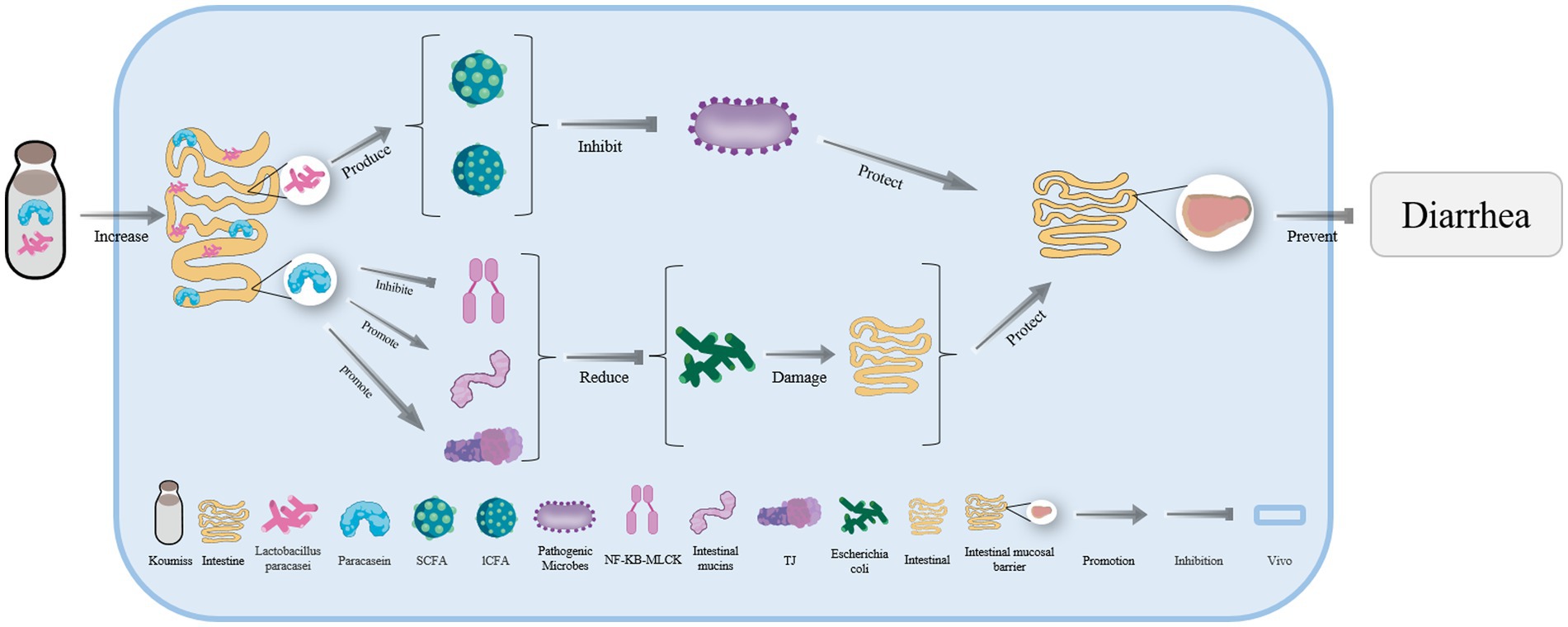
Figure 5. The mechanism of diarrhea treatment with koumiss. Drinking koumiss can enhance the number of Lacticaseibacillus paracasei and paracasein in vivo. SCFA and lCFA are produced by L. paracasei to inhibit the reproduction of pathogenic microorganisms. On the other hand, paracasein can catalyze the secretion of intestinal mucins and TJ while inhibiting the transmission of NF-KB-MLCK, reducing the damage of the intestine by Escherichia coli. This, in turn, helps maintain the integrity of the intestinal mucosal barrier (IMB) and prevents diarrhea.
Consumption of koumiss can increase the numbers of Vibrio desulfovibrio in mouse guts and generate more H2S. This can lead to the disordered oxidation pathway of butyric acid in cells, resulting in DNA chain breakage, inhibition of ATP-dependent K+ channels, and the release of cytochrome C which can help reduce both pulmonary and extrapulmonary tuberculosis in hosts (94, 95). It has also been reported that drinking koumiss can prevent tuberculosis caused by the pathogen Mycobacterium tuberculosis H37RV (89).
Consuming koumiss has been shown to effectively repair glomerular damage and prevent chronic liver and kidney diseases by increasing platelet-derived growth factor c (PDGF-c) and platelet-derived growth factor receptor alpha (PDGFR-α) expression (38, 96). Additionally, L. casei Zhang in koumiss downregulates the expression of toll-like receptors 4 (TLR4) in the liver, promotes the production of TNF-α and oxidative stress responses, and effectively protects the liver (97). Furthermore, koumiss contains angiotensin-converting enzyme inhibitors, which can reduce Angiotensin II (Ang II) generation and reduce hypertension (98–100).
Microbial community alternation during koumiss fermentation
It has been found that total of 12 phyla, 124 genera, and 227 species were found across 29 koumiss samples while 18 phyla, 286 genera, and 491 species across 13 fresh mare milk samples (101). Alghough fresh mare milk is rich in LAB, such as Lactobacillus helveticus, Lactiplantibacillus plantarum, L. kefiranofaciens and Lactococcus lactis, potential microbiological hazards can not be ignored as well. Variable levels of total microbial count (TMC) are 3.40–5.87 (log CFU/ml) in the fresh mare milk, including some potentially pathogens such as Acinetobacter, Klebsiella, Escherichia, Brucellosis, Campylobacter, Mycobacteriosis, Bacillus, Staphylococcus, Streptococcus Cronobacter, and others (10). However, the koumiss microbiota mainly comprised LAB instand of potential pathogens, and sequences representing pathogenic bacteria were not detected (101). In koumiss, Lactobacillus (such as Lentilactobacillus diolivorans, Lactobacillus acidophilus, Lacticaseibacillus casei, Latilactobacillus curvatus), Streptococcus, and Saccharomyces cerevisiae are still the dominated the microbial community as the starter (102, 103). Therefore, the bacterial microbiota diversity of the koumiss was more simplex than the fresh mare milk. In spite of these researches, lower abundance of potential pathogens would be also living in the koumiss during the natural fermentation process and would become the microbiological hazards that should be further assessed with accurate methods.
Conclusions and future perspectives
Koumiss, a traditional fermented dairy product with a long production history, is rich in nutrients such as vitamins C and E, has a balanced calcium-phosphorus ratio, and contains various beneficial trace elements. The natural fermentation process of koumiss leads to the formation of a unique flora structure consisting of a large variety of probiotic compounds. These produce organic acids, extracellular polysaccharides, functional peptides, and other metabolites. Therefore, koumiss can be regarded as a medicinal food that provides a series of special health functions, including immunity enhancement, cancer and tumor prevention, cholesterol reduction, chronic gastritis treatment, and intestinal flora regulation, among others. But, we should be pay attention to the potential pathogens in koumiss. So, standardized koumiss fermentation process should be established with the excellent probiotics as the starter to control the growth and reproduction of harmful bacteria in the future.
Although the special health functions of koumiss have been confirmed through limited animal testing, further studies are needed to elucidate the molecular mechanisms that enable its preventive and therapeutic effects on human diseases. This involves isolating and identifying many unique probiotics present in koumiss for more specialized applications. Furthermore, the molecular regulatory mechanisms underlying the alteration of intestinal flora, as well as other treatments using the probiotics and their metabolites found in koumiss, must be further explored by researchers. In contrast, harmful metabolites produced by these potential pathogens in koumiss should be distinguished by metabolomics technology. And the clinical allergies, intolerances, or contraindications of koumiss should be studied furtherly.
Author contributions
WX: Writing – original draft. XY: Funding acquisition, Conceptualization, Writing – review & editing. ZJ: Funding acquisition, Writing – review & editing. HL: Funding acquisition, Writing – review & editing. YY: Conceptualization, Writing – original draft.
Funding
The author(s) declare financial support was received for the research, authorship, and/or publication of this article. This study was supported by the National Natural Science Foundation of China (32170020), Science and Technology Planning Project of Inner Mongolia (2021GG0005), Natural Science Foundation of Inner Mongolia (2022QN03030 and 2023MS03053), Foundation of Inner Mongolia Minzu University (NMDYB20039), Program for Young Talents of Science and Technology in Universities of Inner Mongolia Autonomous Region (NJYT-19-A10).
Conflict of interest
The authors declare that the research was conducted in the absence of any commercial or financial relationships that could be construed as a potential conflict of interest.
Publisher’s note
All claims expressed in this article are solely those of the authors and do not necessarily represent those of their affiliated organizations, or those of the publisher, the editors and the reviewers. Any product that may be evaluated in this article, or claim that may be made by its manufacturer, is not guaranteed or endorsed by the publisher.
References
1. Malacarne, M, Martuzzi, F, Summer, A, and Mariani, P. Protein and fat composition of mare's milk: some nutritional remarks with reference to human and cow's milk. Int Dairy J. (2002) 12:869–77. doi: 10.1016/S0958-6946(02)00120-6
2. Tang, H, Ma, H, Hou, Q, Li, W, Xu, H, Liu, W, et al. Profiling of koumiss microbiota and organic acids and their effects on koumiss taste. BMC Microbiol. (2020) 20:85. doi: 10.1186/s12866-020-01773-z
3. Afzaal, M, Saeed, F, Anjum, F, Waris, N, Husaain, M, Ikram, A, et al. Nutritional and ethnomedicinal scenario of koumiss: a concurrent review. Food Sci Nutr. (2021) 9:6421–8. doi: 10.1002/fsn3.2595
4. Hu, Y, Zhang, L, Wen, R, Chen, Q, and Kong, B. Role of lactic acid bacteria in flavor development in traditional Chinese fermented foods: a review. Crit Rev Food Sci Nutr. (2022) 62:2741–55. doi: 10.1080/10408398.2020.1858269
5. Kirdar, SS. Therapeutics effects and health benefits of the caucasus koumiss: a review. Annu Res Rev Biol. (2021) 36:47–56. doi: 10.9734/arrb/2021/v36i1130450
6. Nina, AEM. To the question on the technology of koumiss production. Bull Sci Pract. (2017) 10:138–44. doi: 10.5281/zenodo.1012273
7. Fotschki, J, Szyc, AM, Laparra, JM, Markiewicz, LH, and Wróblewska, B. Immune-modulating properties of horse milk administered to mice sensitized to cow milk. J Dairy Sci. (2016) 99:9395–404. doi: 10.3168/jds.2016-11499
8. Wu, R, Wang, L, Wang, J, Li, H, Menghe, B, Wu, J, et al. Isolation and preliminary probiotic selection of lactobacilli from koumiss in Inner Mongolia. J Basic Microbiol. (2009) 49:318–26. doi: 10.1002/jobm.200800047
9. Ishii, SKY. Beneficial role of kumiss intake of Mongolian nomads. J Jpn Soc Food Sci. (2002) 55:281–5. doi: 10.4327/JSNFS.55.281
10. Colavita, G, Amadoro, C, Rossi, F, Fantuz, F, and Salimei, E. Hygienic characteristics and microbiological hazard identification in horse and donkey raw milk. Vet Ital. (2016) 52:21–9. doi: 10.12834/VetIt.180.545.1
11. Liu, J, and Liu, Y. Determination of nutrients and trace metal elements of koumiss in west wuzhumuqin in xilinguole in Inner mongolia and the analysis of environmental influence factor. Adv Mater Res. (2012) 518–523:957–60. doi: 10.4028/www.scientific.net/AMR.518-523.957
12. Lv, J, and Wang, L. Bioactive Components in Milk and Dairy Products. In: YW Park, editor: Wiley Online Library. Ames, Iowa and Oxford, England: Wiley- Blackwell Publishers. (2009)
13. Barreto, IL, Rangel, AHDN, Urbano, SA, Bezerra, JDS, and Oliveira, CADA. Equine milk and its potential use in the human diet. Food Sci Technol. (2019) 39:1–7. doi: 10.1590/fst.11218
14. Wu, Y, Li, Y, Gesudu, Q, Zhang, J, Sun, Z, Halatu, H, et al. Bacterial composition and function during fermentation of Mongolia koumiss. Food Sci Nutr. (2021) 9:4146–55. doi: 10.1002/fsn3.2377
15. Marconi, E, and Panfili, G. Chemical composition and nutritional properties of commercial products of mare milk powder. J Food Compost Anal. (1998) 11:178–87. doi: 10.1006/jfca.1998.0573
16. Dönmez, N, Kısadere, İ, Balaban, C, and Kadiralieva, N. Effects of traditional homemade koumiss on some hematological and biochemical characteristics in sedentary men exposed to exercise. Biotech Histochem. (2014) 89:558–63. doi: 10.3109/10520295.2014.915428
17. Cieslak, J, Wodas, L, Borowska, A, Sadoch, J, Pawlak, P, Puppel, K, et al. Variability of lysozyme and lactoferrin bioactive protein concentrations in equine milk in relation to LYZ and LTF gene polymorphisms and expression. J Sci Food Agric. (2017) 97:2174–81. doi: 10.1002/jsfa.8026
18. Barłowska, J, Polak, G, Janczarek, I, and Tkaczyk, E. The influence of selected factors on the nutritional value of the milk of cold-blooded mares: the example of the sokólski breed. Animals (Basel). (2023) 13:1152. doi: 10.3390/ani13071152
19. Li, Q, Zhang, C, Xilin, T, Ji, M, Meng, X, Zhao, Y, et al. Effects of koumiss on intestinal immune modulation in immunosuppressed rats. Front Nutr. (2022) 9:765499. doi: 10.3389/fnut.2022.765499
20. Choi, SH. Characterization of airag collected in Ulaanbaatar, Mongolia with emphasis on isolated lactic acid bacteria. J Anim Sci Technol. (2016) 58:10. doi: 10.1186/s40781-016-0090-8
21. Salamon, RV, Salamon, S, Csapó-Kiss, Z, and Csapó, J. Composition of mare's colostrum and milk. Fat content, fatty acid composition and vitamin contents. Acta Univ Sapientiae Aliment. (2009) 5:393–402. doi: 10.1016/0958-6946(94)00008-D
22. Liu, W, Wang, J, Zhang, J, Mi, Z, Gesudu, Q, and Sun, T. Dynamic evaluation of the nutritional composition of homemade koumiss from Inner Mongolia during the fermentation process. J Food Process Preserv. (2019) 43:e14022. doi: 10.1111/jfpp.14022
23. Bergonzelli, GE, Blum, S, Brussow, H, and Corthésy-Theulaz, I. Probiotics as a treatment strategy for gastrointestinal diseases? Digestion. (2005) 72:57–68. doi: 10.1159/000087638
24. Vinderola, G, Matar, C, Palacios, J, and Perdigón, G. Mucosal immunomodulation by the non-bacterial fraction of milk fermented by Lactobacillus helveticus R389. Int J Food Microbiol. (2007) 115:180–6. doi: 10.1016/j.ijfoodmicro.2006.10.020
25. Hou, Q, Li, C, Liu, Y, Li, W, Chen, Y, Siqinbateer, et al. Koumiss consumption modulates gut microbiota, increases plasma high density cholesterol, decreases immunoglobulin G and albumin. J Funct Foods. (2019) 52:469–78. doi: 10.1016/j.jff.2018.11.023
26. Wang, Y, Xie, J, Wang, N, Li, Y, Sun, X, Zhang, Y, et al. Lactobacillus casei Zhang modulate cytokine and toll-like receptor expression and beneficially regulate poly I:C-induced immune responses in RAW264.7 macrophages. Microbiol Immunol. (2013) 57:54–62. doi: 10.1111/j.1348-0421.516.x
27. Kaburagi, T, Yamano, T, Fukushima, Y, Yoshino, H, Mito, N, and Sato, K. Effect of Lactobacillus johnsonii La1 on immune function and serum albumin in aged and malnourished aged mice. Nutrition. (2007) 23:342–50. doi: 10.1016/j.nut.2007.02.001
28. Ya, T, Zhang, Q, Chu, F, Merritt, J, Bilige, M, Sun, T, et al. Immunological evaluation of Lactobacillus casei Zhang: a newly isolated strain from koumiss in Inner Mongolia, China. BMC Immunol. (2008) 9:68. doi: 10.1186/1471-2172-9-68
29. Kirjavainen, PV, El-Nezami, HS, Salminen, SJ, Ahokas, JT, and Wright, PF. The effect of orally administered viable probiotic and dairy lactobacilli on mouse lymphocyte proliferation. FEMS Immunol Med Microbiol. (1999) 26:131–5. doi: 10.1111/j.1574-695X.1999.tb01380.x
30. Takeda, K, and Okumura, K. Effects of a fermented milk drink containing Lactobacillus casei strain Shirota on the human NK-cell activity. J Nutr. (2007) 137:791s–3s. doi: 10.1093/jn/137.3.791S
31. Targosz, M, Labuda, A, Czuba, P, Biedroń, R, Strus, M, Gamian, A, et al. Influence of macrophage activation on their capacity to bind bacterial antigens studied with atomic force microscopy. Nanomedicine. (2006) 2:82–8. doi: 10.1016/j.nano.2006.04.006
32. Kursunel, MA, and Esendagli, G. The untold story of IFN-γ in cancer biology. Cytokine Growth Factor Rev. (2016) 31:73–81. doi: 10.1016/j.cytogfr.2016.07.005
33. Dufour, JH, Dziejman, M, Liu, MT, Leung, JH, Lane, TE, and Luster, AD. IFN-gamma-inducible protein 10 (IP-10; CXCL10)-deficient mice reveal a role for IP-10 in effector T cell generation and trafficking. J Immunol. (2002) 168:3195–204. doi: 10.4049/jimmunol.168.7.3195
34. Weiss, G, Rasmussen, S, Zeuthen, LH, Nielsen, BN, Jarmer, H, Jespersen, L, et al. Lactobacillus acidophilus induces virus immune defence genes in murine dendritic cells by a toll-like receptor-2-dependent mechanism. Immunology. (2010) 131:268–81. doi: 10.1111/j.1365-2567.2010.03301.x
35. Westendorf, AM, Fleissner, D, Hansen, W, and Buer, J. T cells, dendritic cells and epithelial cells in intestinal homeostasis. Int J Med Microbiol. (2010) 300:11–8. doi: 10.1016/j.ijmm.2009.08.009
36. Akira, S, Uematsu, S, and Takeuchi, O. Pathogen recognition and innate immunity. Cells. (2006) 124:783–801. doi: 10.1016/j.cell.2006.02.015
37. Kumagai, Y, Takeuchi, O, and Akira, S. TLR9 as a key receptor for the recognition of DNA. Adv Drug Deliv Rev. (2008) 60:795–804. doi: 10.1016/j.addr.2007.12.004
38. Bakir, B, Sari, EK, Aydin, BD, and Yildiz, SE. Immunohistochemical examination of effects of kefir, koumiss and commercial probiotic capsules on platelet derived growth factor-c and platelet derived growth factor receptor-alpha expression in mouse liver and kidney. Biotech Histochem. (2015) 90:190–6. doi: 10.3109/10520295.2014.976841
39. Sari, EK, Bakir, B, Aydin, BD, and Sozmen, M. The effects of kefir, koumiss, yogurt and commercial probiotic formulations on PPARα and PPAR-β/δ expressions in mouse kidney. Biotech Histochem. (2014) 89:287–95. doi: 10.3109/10520295.2013.844274
40. Nishikawa, M, Nagatomi, H, Chang, BJ, Sato, E, and Inoue, M. Targeting superoxide dismutase to renal proximal tubule cells inhibits mitochondrial injury and renal dysfunction inuduced by cisplatin. Arch Biochem Biophys. (2001) 387:78–84. doi: 10.1006/abbi.2000.2237
41. Deigner, HP, and Hermetter, A. Oxidized phospholipids: emerging lipid mediators in pathophysiology. Curr Opin Lipidol. (2008) 19:289–94. doi: 10.1097/MOL.0b013e3282fe1d0e
42. Niki, E, Yoshida, Y, Saito, Y, and Noguchi, N. Lipid peroxidation: mechanisms, inhibition, and biological effects. Biochem Biophys Res Commun. (2005) 338:668–76. doi: 10.1016/j.bbrc.2005.08.072
43. Li, S, Nagothu, KK, Desai, V, Lee, T, Branham, W, Moland, C, et al. Transgenic expression of proximal tubule peroxisome proliferator-activated receptor-alpha in mice confers protection during acute kidney injury. Kidney Int. (2009) 76:1049–62. doi: 10.1038/ki.2009.330
44. Doi, K, Suzuki, Y, Nakao, A, Fujita, T, and Noiri, E. Radical scavenger edaravone developed for clinical use ameliorates ischemia/reperfusion injury in rat kidney. Kidney Int. (2004) 65:1714–23. doi: 10.1111/j.1523-1755.2004.00567.x
45. Chen, Y, Wang, C, Hou, W, Wang, X, Gali, B, Huasai, S, et al. Effects of antibacterial compounds produced by Saccharomyces cerevisiae in koumiss on pathogenic Escherichia coli Os and its cell surface characteristics. J Integr Agric. (2017) 16:742–8. doi: 10.1016/S2095-3119(16)61516-2
46. León, ED, and Francino, MP. Roles of secretory immunoglobulin a in host-microbiota interactions in the gut ecosystem. Front Microbiol. (2022) 13:880484. doi: 10.3389/fmicb.2022.880484
47. Fan, HY, Wang, L, Luo, J, and Long, BG. Protection against Escherichia coli O157:H7 challenge by immunization of mice with purified Tir proteins. Mol Biol Rep. (2012) 39:989–97. doi: 10.1007/s11033-011-0824-0
48. Kirjavainen, PV, ElNezami, HS, Salminen, SJ, Ahokas, JT, and Wright, PF. Effects of orally administered viable Lactobacillus rhamnosus GG and Propionibacterium freudenreichii subsp. shermanii JS on mouse lymphocyte proliferation. Clin Diagn Lab Immunol. (1999) 6:799–802. doi: 10.1128/cdli.6.6.799-802.1999
49. Childers, NK, Bruce, MG, and McGhee, JR. Molecular mechanisms of immunoglobulin a defense. Annu Rev Microbiol. (1989) 43:503–36. doi: 10.1146/annurev.mi.43.100189.002443
50. Sharpe, AH, and Abbas, AK. T-cell costimulation--biology, therapeutic potential, and challenges. N Engl J Med. (2006) 355:973–5. doi: 10.1056/NEJMp068087
51. Chen, Y, Aorigele, C, Wang, C, Hou, W, Zheng, Y, and Simujide, H. Effects of antibacterial compound of Saccharomyces cerevisiae from koumiss on immune function and caecal microflora of mice challenged with pathogenic Escherichia coli O8. Acta Vet Brno. (2019) 88:233–41. doi: 10.2754/avb201988020233
52. Guo, L, Sun, YL, Wang, AH, Xu, CE, and Zhang, MY. Effect of polysaccharides extract of rhizoma atractylodis macrocephalae on thymus, spleen and cardiac indexes, caspase-3 activity ratio, Smac/DIABLO and HtrA2/Omi protein and mRNA expression levels in aged rats. Mol Biol Rep. (2012) 39:9285–90. doi: 10.1007/s11033-012-1677-x
53. De Oliveira, LAM, Miguel, MA, Peixoto, RS, Rosado, AS, Silva, JT, and Paschoalin, VM. Microbiological, technological and therapeutic properties of kefir: a natural probiotic beverage. Braz J Microbiol. (2013) 44:341–9. doi: 10.1590/s1517-83822013000200001
54. Amati, L, Marzulli, G, Martulli, M, Tafaro, A, Jirillo, F, Pugliese, V, et al. Donkey and goat milk intake and modulation of the human aged immune response. Curr Pharm Des. (2010) 16:864–9. doi: 10.2174/138161210790883651
55. Guo, C, Zhang, S, Yuan, Y, Yue, T, and Li, J. Comparison of lactobacilli isolated from Chinese suan-tsai and koumiss for their probiotic and functional properties. J Funct Foods. (2015) 12:294–302. doi: 10.1016/j.jff.2014.11.029
56. Xu, W, Liu, LZ, Loizidou, M, Ahmed, M, and Charles, IG. The role of nitric oxide in cancer. Cell Res. (2002) 12:311–20. doi: 10.1038/sj.cr.7290133
57. Chen, Z, Hsieh, Y, Huang, C, and Tsai, C. Inhibitory effects of probiotic lactobacillus on the growth of human colonic carcinoma cell line HT-29. Molecules. (2017) 22:107. doi: 10.3390/molecules22010107
58. Messina, M, McCaskill-Stevens, W, and Lampe, JW. Addressing the soy and breast cancer relationship: review, commentary, and workshop proceedings. J Natl Cancer Inst. (2006) 98:1275–84. doi: 10.1093/jnci/djj356
59. Renouf, M, and Hendrich, S. Bacteroides uniformis is a putative bacterial species associated with the degradation of the isoflavone genistein in human feces. J Nutr. (2011) 141:1120–6. doi: 10.3945/jn.111.140988
60. Li, C, Hou, C, Duolana, Laga, W, Liu, H, Siqinbateer, et al. Koumiss consumption alleviates symptoms of patients with chronic atrophic gastritis: a possible link to modulation of gut microbiota. J Nutr Oncol. (2018) 2:48–67. Available at: https://www.researchgate.net/publication/327646932
61. Dovizio, M, Alberti, S, Guillem-Llobat, P, and Patrignani, P. Role of platelets in inflammation and cancer: novel therapeutic strategies. Basic Clin Pharmacol Toxicol. (2014) 114:118–27. doi: 10.1111/bcpt.12156
62. Matowicka-Karna, J, Kamocki, Z, Polińska, B, Osada, J, and Kemona, H. Platelets and inflammatory markers in patients with gastric cancer. Clin Dev Immunol. (2013) 2013:401623. doi: 10.1155/2013/401623
63. Korniluk, A, Koper-Lenkiewicz, OM, Kamińska, J, Kemona, H, and Dymicka-Piekarska, V. Mean platelet volume (MPV): new perspectives for an old marker in the course and prognosis of inflammatory conditions. Mediat Inflamm. (2019) 2019:9213074. doi: 10.1155/2019/9213074
64. Song, M, Ping, Y, Zhang, K, Yang, L, Li, F, Zhang, C, et al. Low-dose IFNγ induces tumor cell stemness in tumor microenvironment of non-small cell lung cancer. Cancer Res. (2019) 79:3737–48. doi: 10.1158/0008-5472.Can-19-0596
65. Jorgovanovic, D, Song, M, Wang, L, and Zhang, Y. Roles of IFN-γ in tumor progression and regression: a review. Biomark Res. (2020) 8:49. doi: 10.1186/s40364-020-00228-x
66. Giroux, M, Schmidt, M, and Descoteaux, A. IFN-gamma-induced MHC class II expression: transactivation of class II transactivator promoter IV by IFN regulatory factor-1 is regulated by protein kinase C-alpha. J Immunol. (2003) 171:4187–94. doi: 10.4049/jimmunol.171.8.4187
67. Zhang, M, Huang, L, Ding, G, Huang, H, Cao, G, Sun, X, et al. Interferon gamma inhibits CXCL8-CXCR2 axis mediated tumor-associated macrophages tumor trafficking and enhances anti-PD1 efficacy in pancreatic cancer. J Immunother Cancer. (2020) 8:e000308. doi: 10.1136/jitc-2019-000308
68. Hofmann, AF. Detoxification of lithocholic acid, a toxic bile acid: relevance to drug hepatotoxicity. Drug Metab Rev. (2004) 36:703–22. doi: 10.1081/dmr-200033475
69. Ridlon, JM, Harris, SC, Bhowmik, S, Kang, DJ, and Hylemon, PB. Consequences of bile salt biotransformations by intestinal bacteria. Gut Microbes. (2016) 7:22–39. doi: 10.1080/19490976.2015.1127483
70. Li, C, Liu, X, Wang, H, Fan, H, Mi, Z, Kwok, L-y, et al. Koumiss consumption induced changes in the fecal metabolomes of chronic atrophic gastritis patients. J Funct Foods. (2019) 62:103522. doi: 10.1016/j.jff.2019.103522
71. Beilke, LD, Besselsen, DG, Cheng, Q, Kulkarni, S, Slitt, AL, and Cherrington, NJ. Minimal role of hepatic transporters in the hepatoprotection against LCA-induced intrahepatic cholestasis. Toxicol Sci. (2008) 102:196–204. doi: 10.1093/toxsci/kfm287
72. Lye, HS, Rusul, G, and Liong, MT. Removal of cholesterol by lactobacilli via incorporation and conversion to coprostanol. J Dairy Sci. (2010) 93:1383–92. doi: 10.3168/jds.2009-2574
73. Chiu, CH, Lu, TY, Tseng, YY, and Pan, TM. The effects of Lactobacillus-fermented milk on lipid metabolism in hamsters fed on high-cholesterol diet. Appl Microbiol Biotechnol. (2006) 71:238–45. doi: 10.1007/s00253-005-0145-0
74. Wang, J, Zhang, H, Chen, X, Chen, Y, Menghebilige, and Bao, Q. Selection of potential probiotic lactobacilli for cholesterol-lowering properties and their effect on cholesterol metabolism in rats fed a high-lipid diet. J Dairy Sci. (2012) 95:1645–54. doi: 10.3168/jds.2011-4768
75. Singh, TP, Malik, RK, Katkamwar, SG, and Kaur, G. Hypocholesterolemic effects of Lactobacillus reuteri LR6 in rats fed on high-cholesterol diet. Int J Food Sci Nutr. (2015) 66:71–75. doi: 10.3109/09637486.2014.953450
76. Nishina, PM, and Freedland, RA. Effects of propionate on lipid biosynthesis in isolated rat hepatocytes. J Nutr. (1990) 120:668–73. doi: 10.1093/jn/120.7.668
77. Rader, DJ. High-density lipoproteins and atherosclerosis. Am J Cardiol. (2002) 90:62–70. doi: 10.1016/s0002-9149(02)02635-8
78. Shin, HS, Park, SY, Lee, DK, Kim, SA, An, HM, Kim, JR, et al. Hypocholesterolemic effect of sonication-killed Bifidobacterium longum isolated from healthy adult Koreans in high cholesterol fed rats. Arch Pharm Res. (2010) 33:1425–31. doi: 10.1007/s12272-010-0917-7
79. Ryan, PM, Ross, RP, Fitzgerald, GF, Caplice, NM, and Stanton, C. Functional food addressing heart health: do we have to target the gut microbiota? Curr Opin Clin Nutr Metab Care. (2015) 18:566–71. doi: 10.1097/mco.0000000000000224
80. Madjd, A, Taylor, MA, Mousavi, N, Delavari, A, Malekzadeh, R, Macdonald, IA, et al. Comparison of the effect of daily consumption of probiotic compared with low-fat conventional yogurt on weight loss in healthy obese women following an energy-restricted diet: a randomized controlled trial. Am J Clin Nutr. (2016) 103:323–9. doi: 10.3945/ajcn.115.120170
81. Wang, G, Huang, W, Xia, Y, Xiong, Z, and Ai, L. Cholesterol-lowering potentials of Lactobacillus strain overexpression of bile salt hydrolase on high cholesterol diet-induced hypercholesterolemic mice. Food Funct. (2019) 10:1684–95. doi: 10.1039/c8fo02181c
82. Khare, A, and Gaur, S. Cholesterol-lowering effects of Lactobacillus species. Curr Microbiol. (2020) 77:638–44. doi: 10.1007/s00284-020-01903-w
83. Michael, DR, Moss, JW, Calvente, DL, Garaiova, I, Plummer, SF, and Ramji, DP. Lactobacillus plantarum CUL66 can impact cholesterol homeostasis in Caco-2 enterocytes. Benef Microbes. (2016) 7:443–51. doi: 10.3920/bm2015.0146
84. Li, B, Hui, F, Yuan, Z, Shang, Q, Shuai, G, Bao, Y, et al. Untargeted fecal metabolomics revealed biochemical mechanisms of the blood lipid-lowering effect of koumiss treatment in patients with hyperlipidemia. J Funct Foods. (2021) 78:104355. doi: 10.1016/j.jff.2021.104355
85. Hunter, JE, Zhang, J, and Kris-Etherton, PM. Cardiovascular disease risk of dietary stearic acid compared with trans, other saturated, and unsaturated fatty acids: a systematic review. Am J Clin Nutr. (2010) 91:46–63. doi: 10.3945/ajcn.2009.27661
86. Garmy, N, Taïeb, N, Yahi, N, and Fantini, J. Interaction of cholesterol with sphingosine: physicochemical characterization and impact on intestinal absorption. J Lipid Res. (2005) 46:36–45. doi: 10.1194/jlr.M400199-JLR200
87. Kunihara, M, and Oshima, T. Effects of epinephrine on plasma cholesterol levels in rats. J Lipid Res. (1983) 24:639–44. doi: 10.1016/S0022-2275(20)37969-4
88. Duncan, SH, Louis, P, and Flint, HJ. Lactate-utilizing bacteria, isolated from human feces, that produce butyrate as a major fermentation product. Appl Environ Microbiol. (2004) 70:5810–7. doi: 10.1128/aem.70.10.5810-5817.2004
89. Yahya, R H, Maheswari, R R A, and Mulyono, R H (2012). Microbiological characteristic and antimicrobial activity of koumiss against salmonella typhimurium and Mycobacterium tuberculosis. In: Proceeding of the 2nd international seminar on animal industry. Jakarta: eRepo.
90. Yan, X, Han, W, Jin, X, Sun, Y, Gao, J, Yu, X, et al. Study on the effect of koumiss on the intestinal microbiota of mice infected with toxoplasma gondii. Sci Rep. (2022) 12:1271. doi: 10.1038/s41598-022-05454-x
91. Soret, R, Chevalier, J, De Coppet, P, Poupeau, G, Derkinderen, P, Segain, JP, et al. Short-chain fatty acids regulate the enteric neurons and control gastrointestinal motility in rats. Gastroenterology. (2010) 138:1772–1782.e4. doi: 10.1053/j.gastro.2010.01.053
92. Hamer, HM, Jonkers, D, Venema, K, Vanhoutvin, S, Troost, FJ, and Brummer, RJ. Review article: the role of butyrate on colonic function. Aliment Pharmacol Ther. (2008) 27:104–19. doi: 10.1111/j.1365-2036.2007.03562.x
93. Ren, S, Chen, A, Tian, Y, Bai, Z, and Wang, C. Lactobacillus paracasei from koumiss ameliorates diarrhea in mice via tight junctions modulation. Nutrition. (2022) 98:111584. doi: 10.1016/j.nut.2021.111584
94. Rowan, FE, Docherty, NG, Coffey, JC, and O'Connell, PR. Sulphate-reducing bacteria and hydrogen sulphide in the aetiology of ulcerative colitis. Br J Surg. (2009) 96:151–8. doi: 10.1002/bjs.6454
95. Wang, H, Hussain, T, Yao, J, Li, J, Sabir, N, Liao, Y, et al. Koumiss promotes Mycobacterium bovis infection by disturbing intestinal flora and inhibiting endoplasmic reticulum stress. FASEB J. (2021) 35:e21777. doi: 10.1096/fj.202002485RR
96. Eitner, F, Bücher, E, van Roeyen, CRC, Kunter, U, Rong, S, Seikrit, C, et al. PDGF-C is a proinflammatory cytokine that mediates renal interstitial fibrosis. J Am Soc Nephrol. (2008) 19:281–9. doi: 10.1681/ASN.2007030290
97. Wang, Y, Li, Y, Xie, J, Zhang, Y, Wang, J, Sun, X, et al. Protective effects of probiotic Lactobacillus casei Zhang against endotoxin- and d-galactosamine-induced liver injury in rats via anti-oxidative and anti-inflammatory capacities. Int Immunopharmacol. (2013) 15:30–7. doi: 10.1016/j.intimp.2012.10.026
98. Yu, Z, Yin, Y, Zhao, W, Chen, F, and Liu, J. Antihypertensive effect of angiotensin-converting enzyme inhibitory peptide RVPSL on spontaneously hypertensive rats by regulating gene expression of the renin-angiotensin system. J Agric Food Chem. (2014) 62:912–7. doi: 10.1021/jf405189y
99. Ferrario, CM, Jessup, J, Chappell, MC, Averill, DB, Brosnihan, KB, Tallant, EA, et al. Effect of angiotensin-converting enzyme inhibition and angiotensin II receptor blockers on cardiac angiotensin-converting enzyme 2. Circulation. (2005) 111:2605–10. doi: 10.1161/circulationaha.104.510461
100. Chen, Y, Wang, Z, Chen, X, Liu, Y, Zhang, H, and Sun, T. Identification of angiotensin I-converting enzyme inhibitory peptides from koumiss, a traditional fermented mare's milk. J Dairy Sci. (2010) 93:884–92. doi: 10.3168/jds.2009-2672
101. Zhang, M, Dang, N, Ren, D, Zhao, F, Lv, R, Ma, T, et al. Comparison of bacterial microbiota in raw Mare's Milk and koumiss using PacBio single molecule real-time sequencing technology. Front Microbiol. (2020) 11:581610. doi: 10.3389/fmicb.2020.581610
102. Zhu, L, Zeng, C, Yang, S, Hou, Z, Wang, Y, Hu, X, et al. Diversity and specificity of the bacterial community in Chinese horse milk cheese. Microbiology. (2020) 9:e1066. doi: 10.1002/mbo3.1066
Keywords: koumiss, lactic acid bacteria, nutritional ingredients, chronic disease prevention, Saccharomyces
Citation: Xue W, Yuan X, Ji Z, Li H and Yao Y (2023) Nutritional ingredients and prevention of chronic diseases by fermented koumiss: a comprehensive review. Front. Nutr. 10:1270920. doi: 10.3389/fnut.2023.1270920
Edited by:
Christian Coelho, VetAgro Sup, FranceReviewed by:
Marta Laranjo, University of Evora, PortugalHaiwei Lou, Henan University of Technology, China
Copyright © 2023 Xue, Yuan, Ji, Li and Yao. This is an open-access article distributed under the terms of the Creative Commons Attribution License (CC BY). The use, distribution or reproduction in other forums is permitted, provided the original author(s) and the copyright owner(s) are credited and that the original publication in this journal is cited, in accordance with accepted academic practice. No use, distribution or reproduction is permitted which does not comply with these terms.
*Correspondence: Zhaojun Ji, anpqODA4QDE2My5jb20=
†These authors have contributed equally to this work and share first authorship