- 1Department of Genetics, Cancer Research Institute, Biomedical Research Center of Slovak Academy of Sciences, Bratislava, Slovakia
- 22nd Department of Oncology, Faculty of Medicine, Bratislava and National Cancer Institute, Comenius University, Bratislava, Slovakia
Exploring the role of the gut microbiome in oncology is gaining more attention, mainly due to its ability to shape the immune system in cancer patients. A well-balanced microbial composition forms a symbiotic relationship with the host organism. Mounting evidence supports the potential of modifiable lifestyle factors, such as diet and physical activity, in restoring intestinal dysbiosis related to cancer development and treatment. In this Minireview, we describe the host-microbiome interplay following different dietary patterns, including a high-fat diet, fiber-rich diet, diet rich in rice and beans, Mediterranean diet, ketogenic diet, and physical activity in preclinical findings and clinical settings. According to the results, nutrition is a critical factor influencing the composition of gut microbial communities. Therefore, knowledge about the patient’s nutritional status in pre-treatment and treatment becomes crucial for further management. A combination of individualized dietary habits and professional training plans might help to maintain gut homeostasis, potentially improving the response to anti-cancer therapy and the quality of life in cancer survivors. However, a deep understanding of underlying mechanisms and large clinical trials are needed to uncover clinically relevant correlations for personalized treatment approaches leading to better outcomes for cancer patients.
1 Introduction
Tumor biology and the stage of the disease represent the key factors influencing the treatment response and outcomes for cancer patients (1). However, recent studies also highlight the critical impact of the gut microbiome, as well as microbes inhabiting tissues or organs, including mucosal surfaces of the body and tumor microenvironment, on the treatment efficacy (2–5). In 2022, polymorphic microbiomes were added to the newest version of “Hallmarks of cancer,” comprising common characteristics of tumors. As stated, microbiomes represent an enabling characteristic affecting other cancer hallmarks and modulating tumor phenotype (6).
The gut microbiome represents a complex ecosystem consisting of collective microbiota residing in the human gastrointestinal tract (GIT) with all the genetic material, metabolic functions, and interactions with the environment (7, 8). Dominant microbial phyla in the human intestinal tract are Firmicutes, Bacteroidetes, Proteobacteria, Fusobacteria, Actinobacteria, and Verrucomicrobia (9, 10). The Firmicutes/Bacteroidetes ratio might reflect potential microbiome-associated diseases (11). The production of microbiota-derived metabolites, including mainly short-chain fatty acids (SCFA), represents one of the key elements in host-microbiota crosstalk (12). This process is dependent on the concentration of fiber and other complex carbohydrates but also on intestinal composition and transit time.
Besides oncologic disease, anti-cancer and supportive treatment modalities and antibiotic prophylaxis contribute to major shifts in gut microbiome composition. Data emphasize the relevance of microbiota modulation in cancer survivors via lifestyle-related changes (Figure 1) (13–15).
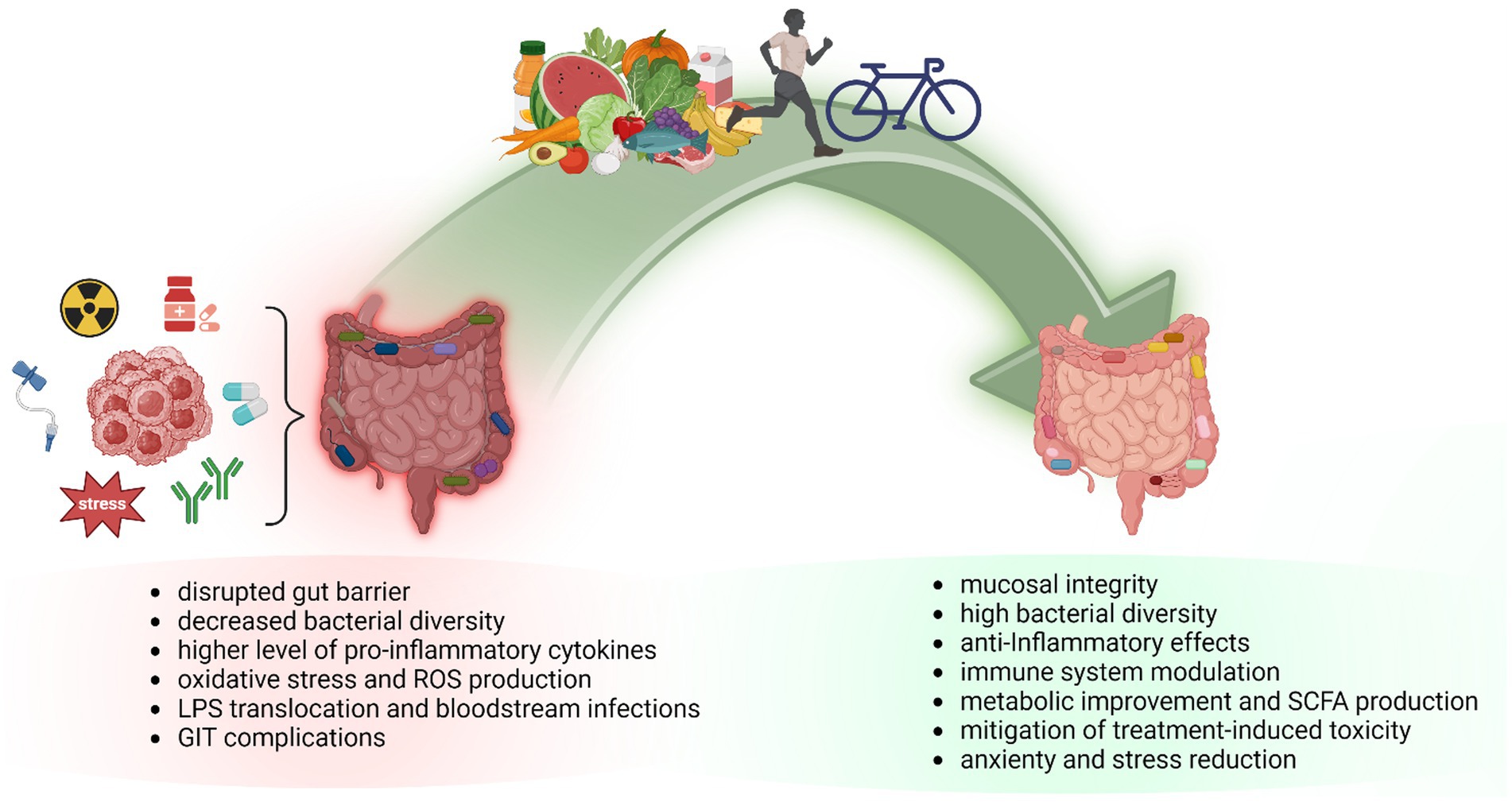
Figure 1. The impact of dietary intervention and exercise on gut microbiome composition in cancer patients. Gut dysbiosis is implicated in cancer development, and progression and negatively affects anti-cancer treatment resulting in worse patient prognosis. A well-balanced diet and regular exercise can modify the composition of the gut microbiome and elevate the levels of favorable microbes and microbiota-derived metabolites, leading to improved gut mucus layer integrity and decreased intestinal inflammation. Diet-microbiome interactions are involved in cancer prevention and development but also in response to anti-cancer treatment via gut-related anti-inflammatory and antioxidant activity. Physical activity guidelines aim to improve health conditions and quality of life in obese, overweight, or inactive cancer patients. As documented, exercise during cancer treatment might reduce mortality risk, and physical activity in cancer survivors decreases the risk of cancer recurrence. GIT, gastrointestinal tract; LPS, lipopolysaccharide; ROS, reactive oxygen species; SCFA, short-chain fatty acids.
Nutrition critically shapes the human gut microbiome composition (16, 17). Wastyk et al. investigated the influence of a plant-based fiber-rich diet and fermented food on the intestinal microbiome and host-immune system in healthy individuals. As detected, a diet based on a high-fermented food diet elevated bacterial diversity while reducing inflammatory markers (18). Recent studies focusing on the diet-driven structure of bacterial communities in cancer patients outline the potential clinical benefits in the context of immunotherapy response modulation (14, 19, 20). Moreover, numerous data highlight the emerging impact of physical activity and exercise in combination with dietary interventions on the quality of life in cancer survivors (21–23).
Herein, we review current knowledge about the role of diet-related microbiome changes and physical activity in cancer patients. Specifically, we describe the host-microbiome interactions in various types of diets. In addition, ongoing clinical trials concerning the clinical implication of diet and exercise interventions in cancer patients are provided. According to the findings so far, targeting the gut microbiota through dietary habits in combination with regular physical activity might represent a potential trend in the future care of cancer patients. However, exact, clinically relevant correlations need to be evaluated in large clinical trials.
2 A diet-microbiome relationship in cancer patients
The impact of nutrition on the efficacy of anti-cancer therapy and patient outcomes is gaining more attention. Mueller et al. showed that pre-surgical administration of immunonutrition containing omega-3 fatty acids, arginine, RNA-nucleotides, and soluble guar fiber improved wound complications and decreased the length of hospital stay in patients with recurrent head and neck squamous cell carcinoma (24). A meta-analysis of ovarian cancer patients confirmed an inverse association between the total vegetable and fruit intake before diagnosis and overall mortality (25).
Different dietary patterns shape the gut microbiome composition and function (26–28). Clinical studies support the importance of a diet that would lead to a higher microbial diversity with limited inflammation-inducing microorganisms and molecules (18, 19, 29). The study on 115 colorectal cancer patients undergoing surgical intervention showed that a higher seaweed consumption correlated with a lower level of Rikenellaceae and Alistipes in the study cohort. On the other hand, data revealed an association between an increased proportion of Bacteroidetes, Bacteroida, and Bacteroidales and higher beverage consumption. The presence of butyrate-producing Clostridium symbiosum negatively correlates with fatty and amino acid intake (30).
2.1 High-fat diet
Ample data confirmed a correlation between high body mass index (BMI) and serious diseases, including cancer (31, 32). Obesity has been associated with a significant decrease in gut microbiome diversity (33). Fruge et al. found the impact of a diet on gut microbiome composition among 40 overweight and obese prostate cancer patients. Samples collected at baseline confirmed an elevated abundance of Proteobacteria. Increased poultry intake was associated with the prevalence of Clostridiales, while red meat consumption correlated with high Prevotella and Blautia (34).
A high-fat diet (HFD) induces microbial changes associated with dysbiosis (35). A meta-analysis of 27 dietary studies with 1,101 animal and human samples described significant microbiome alterations related to HFD, showing the most reproducible signals from the Lactococcus species (36). Currently, in vivo modulation of the gut microbiome by an HFD led to colorectal cancer development via gut microbiota dysbiosis, impaired metabolic pathways, and gut barrier disruption. The results showed an abundance of Alistipes and decreased Parabacteroides distasonis in HFD-fed animals (37). Matsushita et al. indicated the relationship between the elevated presence of systemic lipopolysaccharides related to HFD-induced microbiota disruption and the growth of inflammatory prostate cancer in vivo (38). Importantly, the Chinese traditional medicine extract Evodiamine helped to restore the gut microbiome, leading to a decreased amount of Enterococcus faecalis and Escherichia coli along with Bifidobacterium and Lactobacillus enrichment in the feces of HFD-fed mice with colorectal cancer (39).
2.2 Fiber-rich diet
A pooled analysis of 10 prospective cohorts with 1,445,850 million individuals documented a reduced risk of lung cancer after the consumption of dietary fiber with yogurt (40). In tumor-bearing mice, a high-fiber diet induced the IFN-1 production by intratumoral monocytes leading to a higher efficacy of immunotherapy (41). Clinical studies also confirmed positive correlations and improved response to immune checkpoint inhibitors in the group of patients with a high-fiber intake (42). High dietary fiber was associated with the consumption of fruit, vegetables, and calcium and improved progression-free survival (PFS) in 37 of 128 melanoma patients. A 5-gram increase in fiber intake correlated with a 30% decline in the risk of cancer progression or death. The results revealed the enrichment of the Ruminococcaceae family and Faecalibacterium genus in a group of patients supplemented with sufficient fiber. In an animal model, a diet rich in fiber led to a higher proportion of propionate in stool and correlated with delayed tumor growth (19). A lower consumption of fiber and omega-3 fatty acids was associated with poor response to immunotherapy in melanoma patients (43). Analysis of post-menopausal women with breast cancer confirmed that high total dietary fiber correlated with the decrease in Clostridium spp., showing a correlation between dietary fiber and bacterial taxons with β-glucuronidase activity (44). Fiber-rich diet increases the levels of microbiota-derived SFCA (45), while a low-fiber diet leads to bacterial utilization of amino acids and host mucins (46).
2.3 Mediterranean diet
Mediterranean diet (MD) is assumed to be involved in reduced cancer risk, but the mechanisms are still under discussion (47). A significant correlation between MD and a lower incidence of cancer was observed in the Greek (48), Afghanistan (49), and Italian populations (50). Accordingly, a meta-analysis of prospective cohort studies showed that MD reduced mortality from cancer or cardiovascular diseases (51). The European Prospective Investigation into Cancer and Nutrition (EPIC) study documented that vegetables and fruit protected against breast, colorectal, and lung cancer development. The consumption of alcohol, red, and processed meat correlated with colorectal cancer risk, while the MD and higher fish intake had a strong protective effect (52). However, the Netherland cohort study did not find an association between MD and reduced colorectal cancer risk in the Dutch population (53).
The positive impact of MD on inflammatory processes was observed in preclinical and clinical studies. MD improved azoxymethane-induced dysbiosis in the murine model of colorectal cancer on a high-fat Western diet. The prevalence of Lactobacillaceae was higher in the MD group of azoxymethane-treated animals following a low-fat diet (54). A combination of MD and a healthy lifestyle positively affected the quality of life in breast cancer survivors (55) and resulted in a reduction in overall mortality (56). The Bacteroidetes/Firmicutes ratio decreased in a group of breast cancer survivors supplemented with MD in combination with probiotic sachets containing Bifidobacterium longum BB536 and Lactobacillus rhamnosus HN001 (57). Another study reported reduced gastrointestinal complications in gynecologic cancer patients treated with platinum-based chemotherapy receiving MD (58). Moreover, a meta-analysis confirmed an association between the presence of anti-inflammatory microbiota and the consumption of MD in participants with colorectal cancer or gastrointestinal diseases (59).
2.4 Rice and beans diet
A higher intake of rice and beans was inversely associated with the risk of skin and esophageal cancer (60–62). Alterations in gut microbial composition leading to a higher diversity were identified in a randomized clinical trial evaluating the effects of heat-stabilized rice bran or cooked navy bean powder in colorectal cancer survivors. Both diet components similarly increased total dietary fiber. However, only rice bran intake decreased the Firmicutes/Bacteroidetes ratio and led to increased levels of fecal SCFA (63). Differences in a stool metabolome linked to gut microbial metabolism were observed between overweight and obese colorectal survivors supplemented with 35 g of cooked navy bean and navy bean-free snacks (64). Twenty eight-day diet program with heat-stabilized rice bran or navy bean powder in overweight or obese colorectal cancer survivors led to increased fiber intake. After 14 days, rice bran decreased Firmicutes/Bacteroidetes ratio and elevated fecal concentration of propionate and acetate. However, further longitudinal analyses are needed to evaluate the impact of heat-stabilized rice bran on cancer patient outcomes (63). Consumption of dietary rice bran for 24 weeks in participants with a high risk of colorectal cancer increased Bifidobacteria, Prevotella_9, and Lactobacillales in feces. However dietary intervention did not have a significant effect on fecal bacterial diversity (65). Zhang aimed to evaluate the impact of bean consumption on microbial composition in overweight or obese patients positive for precancerous polyps or colorectal cancer in a BE GONE study (66). The first results of this interventional study indicate that an eight-week boost in dry bean consumption potentially helps to enhance gut microbiome diversity in the study group (67).
2.5 Ketogenic diet
Numerous preclinical findings described the potential therapeutic impact of the ketogenic diet (KD) on tumor growth and enhanced immunity (68–70) and its positive correlation with the response to chemo- and radiotherapy (71, 72). KD, consisting of high fat, low carbohydrate, and low protein, causes metabolic changes via increased blood ketones and reduced blood glucose, leading to starvation of cancer cells. These metabolic changes suggest being implicated in improved survival of animals with malignant gliomas treated with chemotherapy (73). On the contrary, the results from the murine melanoma model did not confirm the association between KD and enhanced tumor growth (74). Shifts in microbial balance resulted in Lactobacillus and Coriobacteriaceae decline, while Romboutsia and Akkermansia enrichment in KD-fed mice bearing ovarian cancer (75). Ferrere et al. stated that KD led to an increase in Akkermansia muciniphila, Ruthenibacterium lactatiformans, and Pseudoflavonifractor capillosus together with a decrease in Lactobacillaceae in melanoma–tumor-bearing mice (76).
The effects of the KD and its impact on the gut microbiome need to be evaluated in clinical settings. In a study comprising breast cancer treated with chemotherapy, it did not bring benefits in terms of improved quality of life and physical activity (77). Recently, the Diet Restriction and Exercise-induced Adaptations in Metastatic Breast Cancer (DREAM) study investigates the impact of the calorie-restricted and KD with aerobic exercise during chemotherapy on tumor burden, quality of life, and treatment toxicity in patients with metastatic breast cancer (78).
2.6 Malnutrition and nutritional support
Investigating the role of the diet-microbiome relationship in malnutrition is in line with the findings that nutritional deficiencies significantly correlate with the response to anti-cancer therapy (79, 80). A high risk of malnutrition, observed in patients with head and neck cancer, is of high concern (81, 82), and early nutritional screening might help identify patients at risk (83). Nutritional support and prophylactic swallowing exercises, performed before dysphagia or problematic swallowing development, improved patient outcomes (84). Uncured malnutrition leads to reduced efficacy and tolerance of chemotherapy or radiotherapy, including elevated treatment toxicity, complications, and prolonged hospitality (85). According to the findings, malnutrition leads to death in almost 20% of cancer patients (86). Studies uncovered that whey protein isolates might be potential supplements that improve nutrition in cancer patients. Whey protein supplementation plus zinc and selenium led to increased levels of albumin and immunoglobulin G in 42 chemotherapy-treated cancer patients compared to the control group supplemented with maltodextrin oral snacks (87).
3 The role of physical activity and exercise in cancer patients
Growing evidence indicates that physical activity is safe for cancer patients undergoing cancer treatment having an impact on metabolic and inflammatory parameters (88, 89). However, intervention during and after treatment should be planned individually with modifications related to different cancer diagnoses (21, 90). Longer survival was observed in stage III colon cancer patients who were physically active and ate vegetables, fruits, and whole grains than participants with higher BMI who did not keep healthy behaviors (88). According to some findings, regular physical activity and exercise were shown to alter the gut microbiome independently from a diet (91). An analysis of ten cross-sectional and seven longitudinal studies documented an increased abundance of Eubacterium rectale, Akkermansia muciniphila, Faecalibacterium prausnitzii, Eubacterium hallii, and Bifidobacterium spp. in active individuals (92).
Beneficial outcomes of exercise-induced modifications in the murine gut microbiome and metabolome suggest being involved in the prevention and treatment of intestinal inflammation and cancer (93). The effect of diet and exercise in breast cancer patients, stage 0-II through a presurgical weight-loss plan (nutritionally adequate and energy-restricted diet with 30 min exercise/day) led to a higher bacterial richness and diversity. According to the level of Akkermansia muciniphila, the women were divided into the high and low Akkermansia muciniphila groups (HAM and LAM group, respectively). The analysis of stool samples from the HAM group showed an increased Prevotella and Lactobacillus and reduced Clostridium, Campylobacter, and Helicobacter. During the study course, women belonging to the HAM group lost a significant percentage of body fat, while the obtained results were not significant for the LAM group (94). Interestingly, the results of the study by Newton et al. will evaluate the effect of a 3-month exercise program on the expansion of favorable gut bacteria in men with prostate cancer receiving androgen deprivation therapy (95).
Paulsen et al. found an association between gut microbiota beta diversity, fatigue, depression, cardio-respiratory fitness, and exercise in breast cancer survivors (96). The term prehabilitation means increased physical fitness intending to reduce stress before esophagogastric surgery (97). The results of a randomized clinical trial showed that prehabilitation increased physical status, endurance, and walking in patients undergoing a surgical procedure for malignant gastroesophageal cancer (98). Uster et al. confirmed that a combination of nutritional sessions and physical exercise in patients with metastatic tumors of the GIT or lung tract contributed to adequate protein intake. As shown, nausea and vomiting were less presented in study participants. Individual nutritional plans for patients contained measures such as oral nutritional supplements, snacks rich in proteins, and snacks rich in energy. Medical history such as anti-cancer therapy, drugs, and blood parameters was documented by the dietician. The exercise program lasted 60 min twice a week with the participation of a physiotherapist (99).
Traditional MD, physical activity, and vitamin D supplementation improved health-related quality of life in breast cancer survivors due to reduced body weight and relieving symptoms of treatment-associated toxicity (55). The result of the survey 12 months after the adaptation of the lifestyle modification program, including higher dietary fiber, lower saturated fatty acids intake, increased exercise, and elevated levels of circulating vitamins, showed a decreased occurrence of symptoms such as nausea, fatigue, vomiting, and constipation in patients with healthy regimen (55). Ho et al. evaluated the effect of diet and physical activity on generic and cancer-specific quality of life, anxiety, and depression in a cohort of 223 patients with colorectal malignancies. According to the findings, the dietary intervention improved both quality of life and depression levels. However, physical activity did not lead to significant improvements (100).
The impact of diet- and exercise-driven changes on microbial diversity in cancer patients is challenging (Table 1) and depends on numerous factors, including cancer type, the stage of disease, specific training interventions, and the patient’s health status.
4 Conclusion and future directions
Targeting the gut microbiome in cancer patients represents an emerging approach that is clinically relevant, not only in terms of patient care but also as a preventive tool, mainly in gastrointestinal cancers. Mounting evidence emphasizes the potential of gut microbiota modulations in improving cancer treatment efficacy, mainly chemo- and immunotherapy. However, large clinical trials are needed to better elucidate the relationship between diet- and exercise-related microbiota modulations and cancer patient outcomes. Precise determination of treatment response-favorable bacterial taxons and communities could bring microbiota-based interventions into clinical practice. Importantly, studies evaluating the correlations between various types of diet, and the particular composition of the gut microbiome, may bring clinically relevant results. In this context, clinicians should collect as much information about the patient‘s nutritional status and eating habits as possible, even in the pre-treatment period.
Standardization of methods and outcome measures are necessary to address the safety and efficacy issues. Diet and fitness plans for patients should be created based on consultations with specialists. Multidisciplinary cooperation between clinicians, nutritionists, and physical therapists might help to increase patients’ response to anti-cancer treatment and improve quality of life while reducing treatment-associated side effects. Educational programs concerning the benefits of a healthy and high-quality diet might be helpful for patients’ engagement.
Moreover, it is crucial to design longitudinal trials that use not only surrogate endpoints for the determination of the effect of nutrition on microbiota but include clinically relevant endpoints as well. Multiple factors influencing microbial composition need to be taken into account when evaluating interactions between diet and microbiota. Especially in cancer patients with progressing disease, we need to distinguish the association from the causal relationship between changes in diet/physical activity and microbiota changes. On the other side, emerging evidence suggests how microbiota modulates treatment response to anti-cancer drugs, and this is most prominent in the era of new-generation immunotherapies. Therefore, strategies to maintain and/or positively modulate gut microbiota could improve cancer care outcomes.
Author contributions
SC: Conceptualization, Funding acquisition, Project administration, Visualization, Writing – original draft, Writing – review & editing. AS: Visualization, Writing – original draft. VS: Visualization, Writing – review & editing. MM: Supervision, Writing – review & editing.
Funding
The author(s) declare financial support was received for the research, authorship, and/or publication of this article. This study was supported by the Scientific Grant Agency of the Ministry of Education, Science, Research and Sport of the Slovak Republic and Slovak Academy of Sciences (VEGA), contract No. 2/0069/22. The funding source had no influence on the writing of the manuscript.
Acknowledgments
Figure 1 was created with BioRender.com.
Conflict of interest
The authors declare that the research was conducted in the absence of any commercial or financial relationships that could be construed as a potential conflict of interest.
The reviewer TB declared a past co-authorship with the author MM to the handling editor.
Publisher’s note
All claims expressed in this article are solely those of the authors and do not necessarily represent those of their affiliated organizations, or those of the publisher, the editors and the reviewers. Any product that may be evaluated in this article, or claim that may be made by its manufacturer, is not guaranteed or endorsed by the publisher.
References
1. Li, W, Deng, Y, Chu, Q, and Zhang, P. Gut microbiome and Cancer immunotherapy. Cancer Lett. (2019) 447:41–7. doi: 10.1016/j.canlet.2019.01.015
2. Alexander, JL, Wilson, ID, Teare, J, Marchesi, JR, Nicholson, JK, and Kinross, JM. Gut microbiota modulation of chemotherapy efficacy and toxicity. Nat Rev Gastroenterol Hepatol. (2017) 14:356–65. doi: 10.1038/nrgastro.2017.20
3. Sevcikova, A, Izoldova, N, Stevurkova, V, Kasperova, B, Chovanec, M, Ciernikova, S, et al. The impact of the microbiome on resistance to cancer treatment with chemotherapeutic agents and immunotherapy. Int J Mol Sci. (2022) 23:488. doi: 10.3390/ijms23010488
4. Chrysostomou, D, Roberts, LA, Marchesi, JR, and Kinross, JM. Gut microbiota modulation of efficacy and toxicity of cancer chemotherapy and immunotherapy. Gastroenterology. (2023) 164:198–213. doi: 10.1053/j.gastro.2022.10.018
5. Lythgoe, MP, Mullish, BH, Frampton, AE, and Krell, J. Polymorphic microbes: a new emerging hallmark of cancer. Trends Microbiol. (2022) 30:1131–4. doi: 10.1016/j.tim.2022.08.004
6. Hanahan, D. Hallmarks of cancer: new dimensions. Cancer Discov. (2022) 12:31–46. doi: 10.1158/2159-8290.CD-21-1059
7. Lozupone, CA, Stombaugh, JI, Gordon, JI, Jansson, JK, and Knight, R. Diversity, stability and resilience of the human gut microbiota. Nature. (2012) 489:220–30. doi: 10.1038/nature11550
8. Berg, G, Rybakova, D, Fischer, D, Cernava, T, Verges, MC, Charles, T, et al. Microbiome definition re-visited: old concepts and new challenges. Microbiome. (2020) 8:103. doi: 10.1186/s40168-020-00875-0
9. Arumugam, M, Raes, J, Pelletier, E, Le Paslier, D, Yamada, T, Mende, DR, et al. Enterotypes of the human gut microbiome. Nature. (2011) 473:174–80. doi: 10.1038/nature09944
10. Eckburg, PB, Bik, EM, Bernstein, CN, Purdom, E, Dethlefsen, L, Sargent, M, et al. Diversity of the human intestinal microbial Flora. Science. (2005) 308:1635–8. doi: 10.1126/science.1110591
11. Stojanov, S, Berlec, A, and Strukelj, B. The influence of probiotics on the Firmicutes/Bacteroidetes ratio in the treatment of obesity and inflammatory bowel disease. Microorganisms. (2020) 8:1715. doi: 10.3390/microorganisms8111715
12. Chalova, P, Tazky, A, Skultety, L, Minichova, L, Chovanec, M, Ciernikova, S, et al. Determination of short-chain fatty acids as putative biomarkers of Cancer diseases by modern analytical strategies and tools: a review. Front Oncol. (2023) 13:1110235. doi: 10.3389/fonc.2023.1110235
13. George, SM, Neuhouser, ML, Mayne, ST, Irwin, ML, Albanes, D, Gail, MH, et al. Postdiagnosis diet quality is inversely related to a biomarker of inflammation among breast cancer survivors. Cancer Epidemiol Biomark Prev. (2010) 19:2220–8. doi: 10.1158/1055-9965.EPI-10-0464
14. Sampsell, K, Hao, D, and Reimer, RA. The gut microbiota: a potential gateway to improved health outcomes in breast cancer treatment and survivorship. Int J Mol Sci. (2020) 21:9239. doi: 10.3390/ijms21239239
15. Ciernikova, S, Mego, M, Hainova, K, Adamcikova, Z, Stevurkova, V, and Zajac, V. Modification of microflora imbalance: future directions for prevention and treatment of colorectal cancer? Neoplasma. (2015) 62:345–52. doi: 10.4149/neo_2015_042
16. Kolodziejczyk, AA, Zheng, D, and Elinav, E. Diet-microbiota interactions and personalized nutrition. Nat Rev Microbiol. (2019) 17:742–53. doi: 10.1038/s41579-019-0256-8
17. Valdes, AM, Walter, J, Segal, E, and Spector, TD. Role of the gut microbiota in nutrition and health. BMJ. (2018) 361:k2179. doi: 10.1136/bmj.k2179
18. Wastyk, HC, Fragiadakis, GK, Perelman, D, Dahan, D, Merrill, BD, Yu, FB, et al. Gut-microbiota-targeted diets modulate human immune status. Cells. (2021) 184:e14:4137–4153.e14. doi: 10.1016/j.cell.2021.06.019
19. Spencer, CN, McQuade, JL, Gopalakrishnan, V, McCulloch, JA, Vetizou, M, Cogdill, AP, et al. Dietary Fiber and probiotics influence the gut microbiome and melanoma immunotherapy response. Science. (2021) 374:1632–40. doi: 10.1126/science.aaz7015
20. Zhou, CB, and Fang, JY. Cross communication of diet-microbiome-immune interactions in Cancer immunotherapy. Cell Rep Med. (2022) 3:100806. doi: 10.1016/j.xcrm.2022.100806
21. Rock, CL, Thomson, CA, Sullivan, KR, Howe, CL, Kushi, LH, Caan, BJ, et al. American Cancer Society nutrition and physical activity guideline for Cancer survivors. CA Cancer J Clin. (2022) 72:230–62. doi: 10.3322/caac.21719
22. Jochems, SHJ, Van Osch, FHM, Bryan, RT, Wesselius, A, van Schooten, FJ, Cheng, KK, et al. Impact of dietary patterns and the Main food groups on mortality and recurrence in Cancer survivors: a systematic review of current epidemiological literature. BMJ Open. (2018) 8:e014530. doi: 10.1136/bmjopen-2016-014530
23. Kim, JY, Lee, MK, Lee, DH, Kang, DW, Min, JH, Lee, JW, et al. Effects of a 12-week home-based exercise program on quality of life, psychological health, and the level of physical activity in colorectal cancer survivors: a randomized controlled trial. Support Care Cancer. (2019) 27:2933–40. doi: 10.1007/s00520-018-4588-0
24. Mueller, SA, Mayer, C, Bojaxhiu, B, Aeberhard, C, Schuetz, P, Stanga, Z, et al. Effect of preoperative immunonutrition on complications after salvage surgery in head and neck cancer. J Otolaryngol Head Neck Surg. (2019) 48:25. doi: 10.1186/s40463-019-0345-8
25. Hurtado-Barroso, S, Trius-Soler, M, Lamuela-Raventos, RM, and Zamora-Ros, R. Vegetable and fruit consumption and prognosis among cancer survivors: a systematic review and meta-analysis of cohort studies. Adv Nutr. (2020) 11:1569–82. doi: 10.1093/advances/nmaa082
26. Rinninella, E, Cintoni, M, Raoul, P, Lopetuso, LR, Scaldaferri, F, Pulcini, G, et al. Food components and dietary habits: keys for a healthy gut microbiota composition. Nutrients. (2019) 11:2393. doi: 10.3390/nu11102393
27. Zmora, N, Suez, J, and Elinav, E. You are what you eat: diet, health and the gut microbiota. Nat Rev Gastroenterol Hepatol. (2019) 16:35–56. doi: 10.1038/s41575-018-0061-2
28. Beam, A, Clinger, E, and Hao, L. Effect of diet and dietary components on the composition of the gut microbiota. Nutrients. (2021) 13:2795. doi: 10.3390/nu13082795
29. Noh, H, Jang, HH, Kim, G, Zouiouich, S, Cho, SY, Kim, HJ, et al. Taxonomic composition and diversity of the gut microbiota in relation to habitual dietary intake in Korean adults. Nutrients. (2021) 13:366. doi: 10.3390/nu13020366
30. Hoang, T, Kim, MJ, Park, JW, Jeong, SY, Lee, J, and Shin, A. Nutrition-wide association study of microbiome diversity and composition in colorectal Cancer patients. BMC Cancer. (2022) 22:656. doi: 10.1186/s12885-022-09735-6
31. Dai, H, Alsalhe, TA, Chalghaf, N, Riccò, M, Bragazzi, NL, and Wu, J. The global burden of disease attributable to high body mass index in 195 countries and territories, 1990-2017: an analysis of the global burden of disease study. PLoS Med. (2020) 17:e1003198. doi: 10.1371/journal.pmed
32. Recalde, M, Davila-Batista, V, Díaz, Y, Leitzmann, M, Romieu, I, Freisling, H, et al. Body mass index and waist circumference in relation to the risk of 26 types of cancer: a prospective cohort study of 3.5 million adults in Spain. BMC Med. (2021) 19:10. doi: 10.1186/s12916-020-01877-3
33. Turnbaugh, PJ, Hamady, M, Yatsunenko, T, Cantarel, BL, Duncan, A, Ley, RE, et al. A core gut microbiome in obese and lean twins. Nature. (2009) 457:480–4. doi: 10.1038/nature07540
34. Fruge, AD, Ptacek, T, Tsuruta, Y, Morrow, CD, Azrad, M, Desmond, RA, et al. Dietary changes impact the gut microbe composition in overweight and obese men with prostate cancer undergoing radical prostatectomy. J Acad Nutr Diet. (2018) 118:e1:714–723.e1. doi: 10.1016/j.jand.2016.10.017
35. Murphy, EA, Velazquez, KT, and Herbert, KM. Influence of high-fat diet on gut microbiota: a driving force for chronic disease risk. Curr Opin Clin Nutr Metab Care. (2015) 18:515–20. doi: 10.1097/MCO.0000000000000209
36. Bisanz, JE, Upadhyay, V, Turnbaugh, JA, Ly, K, and Turnbaugh, PJ. Meta-analysis reveals reproducible gut microbiome alterations in response to a high-fat diet. Cell Host Microbe. (2019) 26:e4:265–272.e4. doi: 10.1016/j.chom.2019.06.013
37. Yang, J, Wei, H, Zhou, Y, Szeto, CH, Li, C, Lin, Y, et al. High-fat diet promotes colorectal tumorigenesis through modulating gut microbiota and metabolites. Gastroenterology. (2022) 162:e2:135–149.e2. doi: 10.1053/j.gastro.2021.08.041
38. Matsushita, M, Fujita, K, Hatano, K, Hayashi, T, Kayama, H, Motooka, D, et al. High-fat diet promotes prostate cancer growth through histamine signaling. Int J Cancer. (2022) 151:623–36. doi: 10.1002/ijc.34028
39. Zhu, LQ, Zhang, L, Zhang, J, Chang, GL, Liu, G, Yu, DD, et al. Evodiamine inhibits high-fat diet-induced colitis-associated cancer in mice through regulating the gut microbiota. J Integr Med. (2021) 19:56–65. doi: 10.1016/j.joim.2020.11.001
40. Yang, JJ, Yu, D, Xiang, YB, Blot, W, White, E, Robien, K, et al. Association of dietary fiber and yogurt consumption with lung cancer risk: a pooled analysis. JAMA Oncol. (2020) 6:e194107. doi: 10.1001/jamaoncol.2019.4107
41. Lam, KC, Araya, RE, Huang, A, Chen, Q, Di Modica, M, Rodrigues, RR, et al. Microbiota triggers sting-type I Ifn-dependent monocyte reprogramming of the tumor microenvironment. Cells. (2021) 184:e21:5338–5356.e21. doi: 10.1016/j.cell.2021.09.019
42. Matson, V, and Gajewski, TF. Dietary modulation of the gut microbiome as an Immunoregulatory intervention. Cancer Cell. (2022) 40:246–8. doi: 10.1016/j.ccell.2022.02.014
43. Simpson, RC, Shanahan, ER, Batten, M, Reijers, ILM, Read, M, Silva, IP, et al. Diet-driven microbial ecology underpins associations between cancer immunotherapy outcomes and the gut microbiome. Nat Med. (2022) 28:2344–52. doi: 10.1038/s41591-022-01965-2
44. Zengul, AG, Demark-Wahnefried, W, Barnes, S, Morrow, CD, Bertrand, B, Berryhill, TF, et al. Associations between dietary fiber, the fecal microbiota and estrogen metabolism in postmenopausal women with breast cancer. Nutr Cancer. (2021) 73:1108–17. doi: 10.1080/01635581.2020.1784444
45. Fu, Y, Moscoso, DI, Porter, J, Krishnareddy, S, Abrams, JA, Seres, D, et al. Relationship between dietary fiber intake and short-chain fatty acid-producing Bacteria during critical illness: a prospective cohort study. JPEN J Parenter Enteral Nutr. (2020) 44:463–71. doi: 10.1002/jpen.1682
46. Schroeder, BO, Birchenough, GMH, Stahlman, M, Arike, L, Johansson, MEV, Hansson, GC, et al. Bifidobacteria or fiber protects against diet-induced microbiota-mediated colonic mucus deterioration. Cell Host Microbe. (2018) 23:e7:27–40.e7. doi: 10.1016/j.chom.2017.11.004
47. Ostan, R, Lanzarini, C, Pini, E, Scurti, M, Vianello, D, Bertarelli, C, et al. Inflammaging and cancer: a challenge for the Mediterranean diet. Nutrients. (2015) 7:2589–621. doi: 10.3390/nu7042589
48. Benetou, V, Trichopoulou, A, Orfanos, P, Naska, A, Lagiou, P, Boffetta, P, et al. Conformity to traditional Mediterranean diet and cancer incidence: the Greek EPIC cohort. Br J Cancer. (2008) 99:191–5. doi: 10.1038/sj.bjc.6604418
49. Amiry, F, Mousavi, SM, Barekzai, AM, and Esmaillzadeh, A. Adherence to the Mediterranean diet in relation to gastric cancer in Afghanistan. Front Nutr. (2022) 9:830646. doi: 10.3389/fnut.2022.830646
50. Bravi, F, Spei, ME, Polesel, J, Di Maso, M, Montella, M, Ferraroni, M, et al. Mediterranean diet and bladder cancer risk in Italy. Nutrients. (2018) 10:1061. doi: 10.3390/nu10081061
51. Sofi, F, Cesari, F, Abbate, R, Gensini, GF, and Casini, A. Adherence to Mediterranean diet and health status: meta-analysis. BMJ. (2008) 337:a1344. doi: 10.1136/bmj.a1344
52. Ubago-Guisado, E, Rodriguez-Barranco, M, Ching-Lopez, A, Petrova, D, Molina-Montes, E, Amiano, P, et al. Evidence update on the relationship between diet and the most common cancers from the European prospective investigation into cancer and nutrition (EPIC) study: a systematic review. Nutrients. (2021) 13:3582. doi: 10.3390/nu13103582
53. Schulpen, M, and van den Brandt, PA. Mediterranean diet adherence and risk of colorectal cancer: the prospective Netherlands cohort study. Eur J Epidemiol. (2020) 35:25–35. doi: 10.1007/s10654-019-00549-8
54. Piazzi, G, Prossomariti, A, Baldassarre, M, Montagna, C, Vitaglione, P, Fogliano, V, et al. A Mediterranean diet mix has chemopreventive effects in a murine model of colorectal cancer modulating apoptosis and the gut microbiota. Front Oncol. (2019) 9:140. doi: 10.3389/fonc.2019.00140
55. Montagnese, C, Porciello, G, Vitale, S, Palumbo, E, Crispo, A, Grimaldi, M, et al. Quality of life in women diagnosed with breast Cancer after a 12-month treatment of lifestyle modifications. Nutrients. (2020) 13:136. doi: 10.3390/nu13010136
56. Castro-Espin, C, Bonet, C, Crous-Bou, M, Nadal-Zaragoza, N, Tjonneland, A, Mellemkjaer, L, et al. Association of Mediterranean diet with survival after breast cancer diagnosis in women from nine European countries: results from the EPIC cohort study. BMC Med. (2023) 21:225. doi: 10.1186/s12916-023-02934-3
57. Pellegrini, M, Ippolito, M, Monge, T, Violi, R, Cappello, P, Ferrocino, I, et al. Gut microbiota composition after diet and probiotics in overweight breast Cancer survivors: a randomized open-label pilot intervention trial. Nutrition. (2020) 74:110749. doi: 10.1016/j.nut.2020.110749
58. Ghisoni, E, Casalone, V, Giannone, G, Mittica, G, Tuninetti, V, and Valabrega, G. Role of Mediterranean diet in preventing platinum based gastrointestinal toxicity in gynecolocological malignancies: a single institution experience. World J Clin Oncol. (2019) 10:391–401. doi: 10.5306/wjco.v10.i12.391
59. Illescas, O, Rodriguez-Sosa, M, and Gariboldi, M. Mediterranean diet to prevent the development of colon diseases: a meta-analysis of gut microbiota studies. Nutrients. (2021) 13:2234. doi: 10.3390/nu13072234
60. Kune, GA, Bannerman, S, Field, B, Watson, LF, Cleland, H, Merenstein, D, et al. Diet, alcohol, smoking, serum beta-carotene, and vitamin a in male nonmelanocytic skin cancer patients and controls. Nutr Cancer. (1992) 18:237–44. doi: 10.1080/01635589209514224
61. Hu, J, Qi, Q, and Zhang, Y. Comparative research for the dietary pattern of patients with esophageal Cancer at different developing stages and the daily intake of vitamin a, E and beta-carotene. Pak J Pharm Sci. (2014) 27:1093–8.
62. Tang, L, Xu, F, Zhang, T, Lei, J, Binns, CW, and Lee, AH. White rice consumption and risk of esophageal cancer in Xinjiang Uyghur autonomous region, Northwest China: a case-control study. J Health Popul Nutr. (2015) 33:4. doi: 10.1186/s41043-015-0019-8
63. Sheflin, AM, Borresen, EC, Kirkwood, JS, Boot, CM, Whitney, AK, Lu, S, et al. Dietary supplementation with Rice bran or navy bean alters gut bacterial metabolism in colorectal Cancer survivors. Mol Nutr Food Res. (2017) 61:201500905. doi: 10.1002/mnfr.201500905
64. Baxter, BA, Oppel, RC, and Ryan, EP. Navy beans impact the stool metabolome and metabolic pathways for colon health in cancer survivors. Nutrients. (2018) 11:28. doi: 10.3390/nu11010028
65. So, WKW, Chan, JYW, Law, BMH, Choi, KC, Ching, JYL, Chan, KL, et al. Effects of a rice bran dietary intervention on the composition of the intestinal microbiota of adults with a high risk of colorectal cancer: a pilot randomised-controlled trial. Nutrients. (2021) 13:526. doi: 10.3390/nu13020526
66. Zhang, X, Browman, G, Siu, W, Basen-Engquist, KM, Hanash, SM, Hoffman, KL, et al. The be Gone trial study protocol: a randomized crossover dietary intervention of dry beans targeting the gut microbiome of overweight and obese patients with a history of colorectal polyps or cancer. BMC Cancer. (2019) 19:1233. doi: 10.1186/s12885-019-6400-z
67. Zhang, X, Hoffman, KL, Li, F, Irajizad, E, Browman, G, Basen-Engquist, K, et al. Abstract LB223: beans to enrich the gut microbiome vs. obesity’s negative effects: first results from the BE GONE trial in high-risk colorectal patients. Cancer Res. (2021) 81:LB223-LB223. doi: 10.1158/1538-7445.AM2021-LB223
68. Otto, C, Kaemmerer, U, Illert, B, Muehling, B, Pfetzer, N, Wittig, R, et al. Growth of human gastric cancer cells in nude mice is delayed by a ketogenic diet supplemented with Omega-3 fatty acids and medium-chain triglycerides. BMC Cancer. (2008) 8:122. doi: 10.1186/1471-2407-8-122
69. Lussier, DM, Woolf, EC, Johnson, JL, Brooks, KS, Blattman, JN, and Scheck, AC. Enhanced immunity in a mouse model of malignant glioma is mediated by a therapeutic ketogenic diet. BMC Cancer. (2016) 16:310. doi: 10.1186/s12885-016-2337-7
70. Weber, DD, Aminazdeh-Gohari, S, and Kofler, B. Ketogenic diet in Cancer therapy. Aging. (2018) 10:164–5. doi: 10.18632/aging.101382
71. Yang, L, TeSlaa, T, Ng, S, Nofal, M, Wang, L, Lan, T, et al. Ketogenic diet and chemotherapy combine to disrupt pancreatic Cancer metabolism and growth. Med. (2022) 3:119–136.e8. doi: 10.1016/j.medj.2021.12.008
72. Abdelwahab, MG, Fenton, KE, Preul, MC, Rho, JM, Lynch, A, Stafford, P, et al. The ketogenic diet is an effective adjuvant to radiation therapy for the treatment of malignant glioma. PLoS One. (2012) 7:e36197. doi: 10.1371/journal.pone.0036197
73. Woolf, EC, and Scheck, AC. The ketogenic diet for the treatment of malignant glioma. J Lipid Res. (2015) 56:5–10. doi: 10.1194/jlr.R046797
74. Xia, S, Lin, R, Jin, L, Zhao, L, Kang, HB, Pan, Y, et al. Prevention of dietary-fat-fueled Ketogenesis attenuates Braf V600e tumor growth. Cell Metab. (2017) 25:358–73. doi: 10.1016/j.cmet.2016.12.010
75. Tewari, S, Esakov, E, Chau, D, Sangwan, N, Reizes, O, and Alhilli, M. The impact of ketogenic diet on the gut microbiome and tumor growth in an in vivo epithelial ovarian cancer model (105). Gynecol Oncol. (2022) 166:S70. doi: 10.1016/S0090-8258(22)01332-4
76. Ferrere, G, Alou, TM, Liu, P, Goubet, AG, Fidelle, M, Kepp, O, et al. Ketogenic diet and ketone bodies enhance the anticancer effects of Pd-1 blockade. JCI Insight. (2021) 6:e145207. doi: 10.1172/jci.insight.145207
77. Khodabakhshi, A, Seyfried, TN, Kalamian, M, Beheshti, M, and Davoodi, SH. Does a ketogenic diet have beneficial effects on quality of life, physical activity or biomarkers in patients with breast cancer: a randomized controlled clinical trial. Nutr J. (2020) 19:87. doi: 10.1186/s12937-020-00596-y
78. Kirkham, AA, King, K, Joy, AA, Pelletier, AB, Mackey, JR, Young, K, et al. Rationale and design of the diet restriction and exercise-induced adaptations in metastatic breast cancer (Dream) study: a 2-arm, parallel-group, phase ii, randomized control trial of a short-term, calorie-restricted, and ketogenic diet plus exercise during intravenous chemotherapy versus usual care. BMC Cancer. (2021) 21:1093. doi: 10.1186/s12885-021-08808-2
79. Greathouse, KL, Wyatt, M, Johnson, AJ, Toy, EP, Khan, JM, Dunn, K, et al. Diet-microbiome interactions in cancer treatment: opportunities and challenges for precision nutrition in Cancer. Neoplasia. (2022) 29:100800. doi: 10.1016/j.neo.2022.100800
80. Gouez, M, Delrieu, L, Bouleuc, C, Girard, N, Raynard, B, and Marchal, T. Association between nutritional status and treatment response and survival in patients treated with immunotherapy for lung Cancer: a retrospective French study. Cancers. (2022) 14:3439. doi: 10.3390/cancers14143439
81. Langius, JA, van Dijk, AM, Doornaert, P, Kruizenga, HM, Langendijk, JA, Leemans, CR, et al. More than 10% weight loss in head and neck cancer patients during radiotherapy is independently associated with deterioration in quality of life. Nutr Cancer. (2013) 65:76–83. doi: 10.1080/01635581.2013.741749
82. Orell-Kotikangas, H, Osterlund, P, Makitie, O, Saarilahti, K, Ravasco, P, Schwab, U, et al. Cachexia at diagnosis is associated with poor survival in head and neck cancer patients. Acta Otolaryngol. (2017) 137:778–85. doi: 10.1080/00016489.2016.1277263
83. Simon, SR, Pilz, W, Hoebers, FJP, Leeters, IPM, Schols, A, Willemsen, ACH, et al. Malnutrition screening in head and neck Cancer patients with oropharyngeal dysphagia. Clin Nutr ESPEN. (2021) 44:348–55. doi: 10.1016/j.clnesp.2021.05.019
84. Kristensen, MB, Isenring, E, and Brown, B. Nutrition and swallowing therapy strategies for patients with head and neck cancer. Nutrition. (2020) 69:110548. doi: 10.1016/j.nut.2019.06.028
85. Muscaritoli, M, Lucia, S, Farcomeni, A, Lorusso, V, Saracino, V, Barone, C, et al. Prevalence of malnutrition in patients at first medical oncology visit: the Premio study. Oncotarget. (2017) 8:79884–96. doi: 10.18632/oncotarget.20168
86. Muscaritoli, M, Arends, J, Bachmann, P, Baracos, V, Barthelemy, N, Bertz, H, et al. Espen practical guideline: clinical nutrition in cancer. Clin Nutr. (2021) 40:2898–913. doi: 10.1016/j.clnu.2021.02.005
87. Bumrungpert, A, Pavadhgul, P, Nunthanawanich, P, Sirikanchanarod, A, and Adulbhan, A. Whey protein supplementation improves nutritional status, glutathione levels, and immune function in cancer patients: a randomized, double-blind controlled Trial. J Med Food. (2018) 21:612–6. doi: 10.1089/jmf.2017.4080
88. Van Blarigan, EL, Fuchs, CS, Niedzwiecki, D, Zhang, S, Saltz, LB, Mayer, RJ, et al. Association of Survival with adherence to the American Cancer Society nutrition and physical activity guidelines for cancer survivors after colon cancer diagnosis: the CALGB 89803/Alliance trial. JAMA Oncol. (2018) 4:783–90. doi: 10.1001/jamaoncol.2018.0126
89. Christensen, JF, Simonsen, C, and Hojman, P. Exercise training in Cancer control and treatment. Compr Physiol. (2018) 9:165–205. doi: 10.1002/cphy.c180016
90. Campbell, KL, Winters-Stone, KM, Wiskemann, J, May, AM, Schwartz, AL, Courneya, KS, et al. Exercise guidelines for cancer survivors: consensus statement from international multidisciplinary roundtable. Med Sci Sports Exerc. (2019) 51:2375–90. doi: 10.1249/MSS.0000000000002116
91. Mailing, LJ, Allen, JM, Buford, TW, Fields, CJ, and Woods, JA. Exercise and the gut microbiome: a review of the evidence, potential mechanisms, and implications for human health. Exerc Sport Sci Rev. (2019) 47:75–85. doi: 10.1249/JES.0000000000000183
92. Aya, V, Florez, A, Perez, L, and Ramirez, JD. Association between physical activity and changes in intestinal microbiota composition: a systematic review. PLoS One. (2021) 16:e0247039. doi: 10.1371/journal.pone.0247039
93. Allen, JM, Mailing, LJ, Cohrs, J, Salmonson, C, Fryer, JD, Nehra, V, et al. Exercise training-induced modification of the gut microbiota persists after microbiota colonization and attenuates the response to chemically-induced colitis in Gnotobiotic mice. Gut Microbes. (2018) 9:115–30. doi: 10.1080/19490976.2017.1372077
94. Fruge, AD, Van der Pol, W, Rogers, LQ, Morrow, CD, Tsuruta, Y, and Demark-Wahnefried, W. Fecal Akkermansia muciniphila is associated with body composition and microbiota diversity in overweight and obese women with breast Cancer participating in a Presurgical weight loss trial. J Acad Nutr Diet. (2020) 120:650–9. doi: 10.1016/j.jand.2018.08.164
95. Newton, RU, Christophersen, CT, Fairman, CM, Hart, NH, Taaffe, DR, Broadhurst, D, et al. Does exercise impact gut microbiota composition in men receiving androgen deprivation therapy for prostate cancer? A single-blinded, two-armed, randomised controlled trial. BMJ Open. (2019) 9:e024872. doi: 10.1136/bmjopen-2018-024872
96. Paulsen, JA, Ptacek, TS, Carter, SJ, Liu, N, Kumar, R, Hyndman, L, et al. Gut microbiota composition associated with alterations in cardiorespiratory fitness and psychosocial outcomes among breast cancer survivors. Support Care Cancer. (2017) 25:1563–70. doi: 10.1007/s00520-016-3568-5
97. Carli, F, and Zavorsky, GS. Optimizing functional exercise capacity in the elderly surgical population. Curr Opin Clin Nutr Metab Care. (2005) 8:23–32. doi: 10.1097/00075197-200501000-00005
98. Minnella, EM, Awasthi, R, Loiselle, SE, Agnihotram, RV, Ferri, LE, and Carli, F. Effect of exercise and nutrition prehabilitation on functional capacity in esophagogastric cancer surgery: a randomized clinical trial. JAMA Surg. (2018) 153:1081–9. doi: 10.1001/jamasurg.2018.1645
99. Uster, A, Ruehlin, M, Mey, S, Gisi, D, Knols, R, Imoberdorf, R, et al. Effects of nutrition and physical exercise intervention in palliative cancer patients: a randomized controlled trial. Clin Nutr. (2018) 37:1202–9. doi: 10.1016/j.clnu.2017.05.027
100. Ho, M, Ho, JWC, Fong, DYT, Lee, CF, Macfarlane, DJ, Cerin, E, et al. Effects of dietary and physical activity interventions on generic and cancer-specific health-related quality of life, anxiety, and depression in colorectal Cancer survivors: a randomized controlled trial. J Cancer Surviv. (2020) 14:424–33. doi: 10.1007/s11764-020-00864-0
Keywords: gut microbiome, cancer, dietary interventions, treatment response, physical activity
Citation: Ciernikova S, Sevcikova A, Stevurkova V and Mego M (2023) Diet-driven microbiome changes and physical activity in cancer patients. Front. Nutr. 10:1285516. doi: 10.3389/fnut.2023.1285516
Edited by:
Julio Plaza-Diaz, Children’s Hospital of Eastern Ontario (CHEO), CanadaReviewed by:
Lucilla Crudele, University of Bari Aldo Moro, ItalyHira Rafi, Northwestern University, United States
Tomas Buchler, Charles University, Czechia
Copyright © 2023 Ciernikova, Sevcikova, Stevurkova and Mego. This is an open-access article distributed under the terms of the Creative Commons Attribution License (CC BY). The use, distribution or reproduction in other forums is permitted, provided the original author(s) and the copyright owner(s) are credited and that the original publication in this journal is cited, in accordance with accepted academic practice. No use, distribution or reproduction is permitted which does not comply with these terms.
*Correspondence: Sona Ciernikova, c29uYS5jaWVybmlrb3ZhQHNhdmJhLnNr