- 1Escuela de Tecnología Médica, Facultad de Salud, Universidad Santo Tomás, Osorno, Chile
- 2Departamento de Ingeniería Química, Facultad de Ingeniería y Ciencias, Universidad de La Frontera, Temuco, Chile
- 3Departamento de Ciencias Preclínicas, Facultad de Medicina, Universidad de La Frontera, Temuco, Chile
- 4Carrera de Tecnología Médica, Facultad de Medicina, Universidad de La Frontera, Temuco, Chile
- 5Laboratorio de Morfometría, Metabolismo y Enfermedades Cardiovasculares, Centro Biomédico, Instituto de Biología, Universidade do Estado do Rio de Janeiro, Rio de Janeiro, Brazil
Introduction: There exists a correlation between obesity and the consumption of an excessive amount of calories, with a particular association between the intake of saturated and trans fats and an elevated body mass index. Omega-3 fatty acids, specifically eicosapentaenoic and docosahexaenoic acids, have been identified as potential preventive nutrients against the cardiometabolic hazards that are commonly associated with obesity. The objective of this comprehensive review was to elucidate the involvement of long-chain polyunsaturated fatty acids, specifically eicosapentaenoic acid and docosahexaenoic acid, in the modulation of gene expression during the progression of obesity.
Methods: The present analysis focused on primary studies that investigated the association between long-chain polyunsaturated fatty acids, gene expression, and obesity in individuals aged 18 to 65 years. Furthermore, a comprehensive search was conducted on many databases until August 2023 to identify English-language scholarly articles utilizing MeSH terms and textual content pertaining to long-chain polyunsaturated fatty acids, gene expression, obesity, and omega-3. The protocol has been registered on PROSPERO under the registration number CRD42022298395. A comprehensive analysis was conducted on a total of nine primary research articles. All research collected and presented quantitative data.
Results and Discussion: The findings of our study indicate that the incorporation of eicosapentaenoic and docosahexaenoic acid may have potential advantages and efficacy in addressing noncommunicable diseases, including obesity. This can be attributed to their anti-inflammatory properties and their ability to regulate genes associated with obesity, such as PPARγ and those within the ALOX family.
Systematic Review Registration: https://www.crd.york.ac.uk/prospero/display_record.php?ID=CRD42022298395, CRD42022298395.
1. Introduction
Due to its immense effects on health, medical costs, and mortality, obesity is a public health concern. Over 30 % of adults have been impacted by obesity and overweight (1, 2). Obesity is a multifaceted and complex condition that is typically preventable; it is characterized by an excessive accumulation of adipose tissue that poses a health risk due to its association with type 2 diabetes, hypertension, and hyperlipidemia (3, 4).
The multidisciplinary field of study known as genomics was created to understand genomes’ structure, function, evolution, and mapping (5). In this way, nutrigenomics and nutri-epigenomics enable the chemical analysis of food and the investigation of the metabolites created during food oxidation to modify gene expression either directly or indirectly through epigenetic remodeling (6).
Every meal contains thousands of biologically active substances, many of which may benefit our health. Among these are those related to polyunsaturated fatty acids. Within the unsaturated fatty acids, the long-chain polyunsaturated fatty acids can be further split into the omega-3 and omega-6 groups (7), which are particularly significant. Eicosapentaenoic and docosahexaenoic acids, which are mainly found in fish, shellfish, and marine algae, and arachidonic acid, which is regularly found in chicken and eggs, are examples of common long-chain polyunsaturated fatty acids (8).
Consumption of indulgent foods, saturated lipids, and trans fats is associated with a higher body mass index. According to Swinburn et al. (4), excessive caloric intake and poor diet quality have also been linked to obesity. It is now known, however, that omega-3 fatty acids, such as eicosapentaenoic and docosahexaenoic acids, are adequate nutrients that protect against the cardiometabolic dangers associated with obesity (9). As a result, eicosapentaenoic and docosahexaenoic acids inhibit the innate immune response that TLR4 induces in adipose and trophoblast cells to reduce inflammation in obese pregnant women (10). Also, taking extra eicosapentaenoic and docosahexaenoic acid lowers the amount of triacylglycerol in the blood (11–13).
However, genetic variability and variables such as physical activity, drugs, and food pesticide residues, among other factors, produce multiple results (14). For these reasons, understanding the molecular effects of long-chain polyunsaturated fatty acids, e.g., eicosapentaenoic and docosahexaenoic acids, in regulating gene expression during the development of obesity is a promising goal that could strongly impact dietary choices, considering not only food composition but also its nutrigenomic and nutria-epigenomic properties. Thus, the aim of the study was to describe the role of long-chain polyunsaturated fatty acids, eicosapentaenoic, and docosahexaenoic acids, in the regulation of gene expression during the development of obesity in humans between 18 and 65 years old.
2. Materials and methods
The goal of this study was to do a systematic review of quantitative studies that look at how long-chain polyunsaturated fatty acids, especially eicosapentaenoic and docosahexaenoic acids, are implied in gene expression during obesity development in people between the ages of 18 and 65. The protocol was assigned the registration number CRD42022298395 in the PROSPERO database. The review has been performed according to PRISMA (15).
2.1. Search strategy and selection criteria
2.1.1. Search strategy
By using MeSH terms (such as “long-chain polyunsaturated fatty acids” AND “gene expression” AND “obesity” AND “omega-3”) and text terms associated with gene expression, nutrigenomics, and omega-3 in accordance with the research question, several databases (MEDLINE, EMBASE, Scopus, and Web of Science) were searched up to August 2023 for original articles and primary quantitative studies in English. The searches were a part of larger searches for several reviews examining a variety of health-related factors, including anthropometric factors, nutritional analysis, and physical analysis. The included studies and relevant reviews’ reference lists were also searched.
2.1.2. Identification of relevant studies
Two reviewers screened titles, abstracts, and papers for inclusion. Discussion with another reviewer helped to resolve differences between reviewers’ results.
2.1.3. Types of study and design
The specific inclusion criteria were: 1. primary quantitative studies or mixed methods studies with a quantitative component (using descriptive or inferential statistics methods, with parametric or non-parametric methods): cross-sectional studies or randomized controlled trials, which report the type of long-chain polyunsaturated fatty acids (eicosapentaenoic and docosahexaenoic acids), measurements of lean or fat-free mass, total body weight, body mass index and 2. studies in English. Research studies were excluded if they: 1. systematic reviews; 2. conference abstracts; 3. editor letters; 4. were not an original investigation published in full; 5. did not provide or specify numerical data; 6. studies realized just in postmenopausal women; 7. studies focused just in population older than 65 years old and 8. studies not focused on the role of long-chain polyunsaturated fatty acids in the regulation of gene expression during the development of obesity, or that do not describe anthropometric measurements, nutritional or physical analyzes.
2.1.4. Population
Men or women who lived in the community, between 18 and 65 years old, and healthy volunteers free of problems that could lead to subsequent illness, such as osteoarthritis, diabetes, insulin resistance, high blood pressure, or high cholesterol. Additionally, exclusion criteria included being pregnant, nursing, or postmenopausal (due to the effects of estrogen fluctuation on endothelial function and other parameters), having an immune system defect, having a current clinical disease (specifically diabetes and gastrointestinal, liver, kidney, stroke, mental, coronary heart, and thyroid disease), consuming medications that interact with serum lipid profiles and weight loss, taking anticoagulant and beta-blocking medications, and taking anti-HIV drugs.
2.1.5. Quality assessment/risk of bias
One reviewer evaluated the approach’s quality using the National Institute for Health and Care Excellence methodology for quantitative studies (16), while a second reviewer verified its accuracy. Discussions between reviewers helped to settle their differences. As a result, no studies were disqualified due to poor quality.
2.1.6. Data synthesis and extraction
Data relating to population and study characteristics of the included studies were extracted by one reviewer and checked by another reviewer (Table 1).
Two researchers systematically analyzed the whole text, meticulously examining the data pertaining to long-chain polyunsaturated fatty acids, gene expression, and obesity. Their objective was to identify relevant information concerning the variables associated with the influence of long-chain polyunsaturated fatty acids on the regulation of gene expression during the developmental stages of obesity in individuals aged 18 to 65 years. The content underwent a thorough examination and was subsequently reorganized into distinct subjects, as presented in Table 2. These were included if the study’s authors built their interpretation and concepts from the initial data.
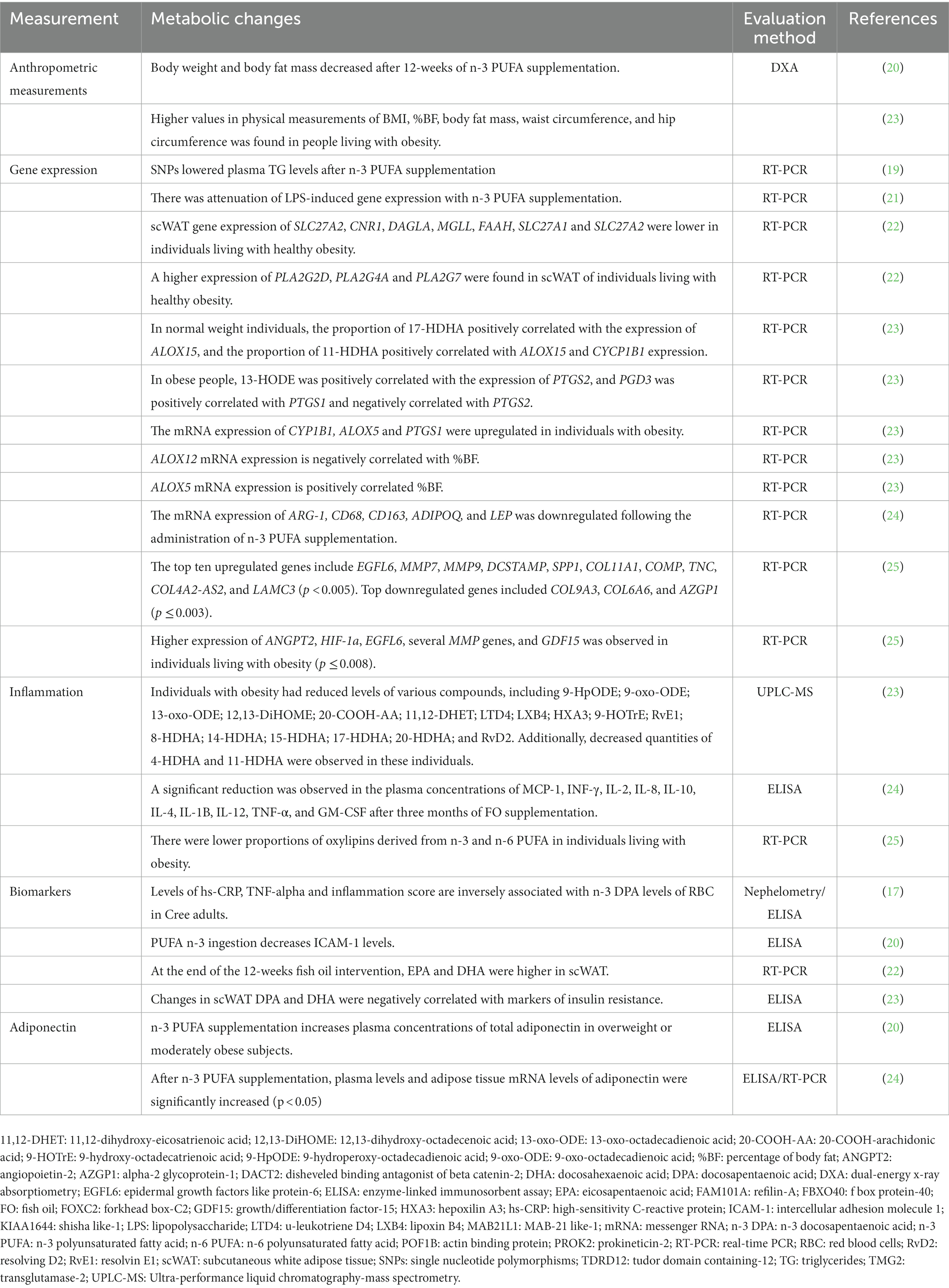
Table 2. Variables involved in role of long-chain polyunsaturated fatty acids in the regulation of gene expression during the development of obesity.
3. Results
Figure 1 illustrates the flow chart for the study selection process from the nine papers identified (17–25). A summary of the included studies and the populations, settings, and contexts in which they were conducted are shown in Table 1.
3.1. Description of included studies
Four publications from the primary studies were realized in the US, two in Canada, and three in the United Kingdom. Nine papers in all were examined. The papers collected and reported quantitative data through clinical trials or experimental studies (Table 1).
Participants from both sexes were included in every study (17–25). Additionally, seven investigations on white people (18, 20–25), one on the Cree population (17), and one on residents of Quebec City were carried out (19).
Details of the long-chain polyunsaturated fatty acids investigated in each study are shown in Table 1. Only omega-3 long-chain polyunsaturated fatty acid studies have been included (17–25).
3.2. Quality assessment
Table 3 displays the quality assessment outcomes and evaluation standards for thorough research. Studies’ overall internal and external validity quality was often high or moderate. No studies were removed due to poor quality.
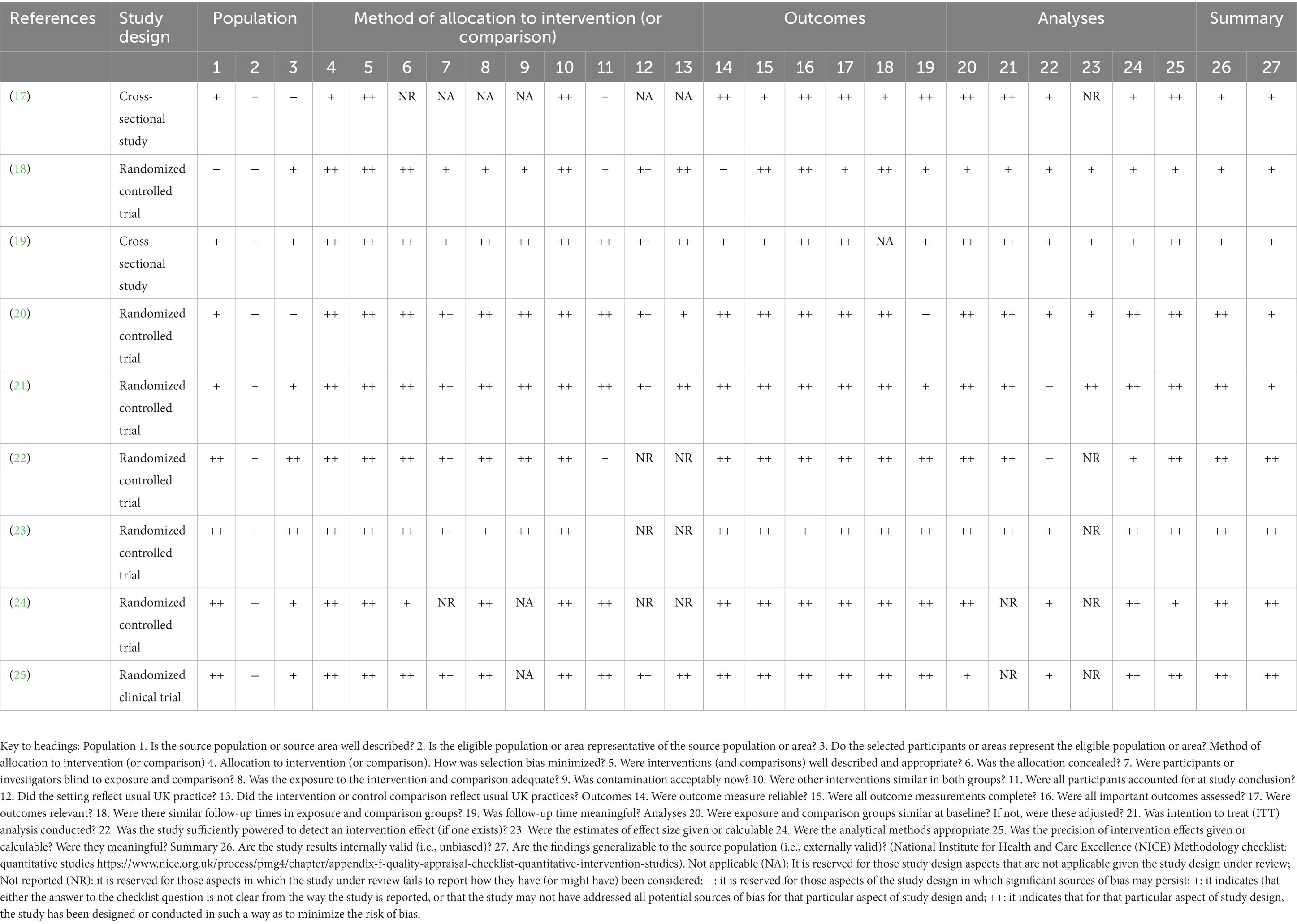
Table 3. National Institute for health and care excellence methodology checklist: quantitative studies.
3.3. Body size and composition
The effects of long-chain polyunsaturated fatty acids consumption on body composition can be addressed using anthropometric measurements such as waist circumference, fat mass, lean mass, and body mass index; however, other analyses related to the effects of long-chain polyunsaturated fatty acids consumption on gene expression through biomarkers, such as cytokines, enzyme activity, fatty acid composition, oxylipins, and biochemical markers, could be used too.
While studies had differing objectives, populations, and contexts, some themes were consistently repeated across several studies (Table 2). The main themes described have been identified in high-quality studies. None were identified in low-quality studies.
3.4. Relation between long-chain polyunsaturated fatty acids and obesity
The use of biomarkers or anthropometric measurements to evaluate the effects on body composition has been indicated in almost all studies (Table 2). In effect, Kratz et al. (18). found decreased body weight and body fat after omega-3 long-chain polyunsaturated fatty acid supplementation (p < 0.001 and p = 0.002, respectively).
3.5. Regulation of gene expression
Gene expression refers to the mechanism via which the genetic information contained within a gene is translated into a functional product or activity. Western blot, mRNA analysis, and enzyme-linked immunosorbent assays are commonly employed techniques for quantifying the expression levels of gene products.
Accordingly, PLA2G2A and PLA2G4A genes were up-regulated by omega-3 polyunsaturated fatty acids supplementation (19, 22), whereas SLC27A2, CNR1, DAGLA, MGLL, FAAH, SLC27A1, and SLC27A2 genes were found to be down-regulated in people living with healthy obesity (22). Additionally, the ALOX5 gene shows a negative correlation with body fat and fat mass, while the ALOX12 gene has a positive correlation with both of them (23).
3.6. Biomarkers
Fatty tissue is believed to function as a very sophisticated organ system (26). The primary cause of metabolic disorders is white adiposse tissue, particularly in the abdomen (27–29). In white adiposse tissue, adipocytes release various hormones and inflammatory substances, such as cytokines (24, 30). Leptin, adiponectin, resistin, and visfatin are the hormones typically linked to adipose tissue; nevertheless, adipocytes can also release IL-6 and TNF-α (31, 32).
In this regard, hs-CRP and IL-6 were directly associated with docosahexaenoic acid levels, whereas IL-6 and TNF-α were inversely associated with eicosapentaenoic acid and omega-3 long-chain polyunsaturated fatty acid levels (17). On the other hand, after eating a meal high in omega-3 fatty acids, plasma CRP increased over time, whereas TNF-α and VCAM-1 tended to decline (20). A meal richer in omega-3 fatty acids had a more significant postprandial effect on nuclear factor-κB over the following 4 h than a meal high in saturated fat. However, the cumulative impact of the meals was not statistically significant (20). Similarly, increased docosahexaenoic acid values were discovered following the omega-3 long-chain polyunsaturated fatty acid intervention (22, 23). Two genes that are significant for gene-nutrient expression, have been markedly up-regulated in the omega-3 long-chain polyunsaturated fatty acids supplemented group (21). Results are shown in detail in Tables 1, 2.
4. Discussion
This systematic review collates and synthesizes evidence from nine quantitative studies relating long-chain polyunsaturated fatty acid consumption (eicosapentaenoic and docosahexaenoic acids) with the regulation of gene expression and anti-inflammatory effects during the development of obesity in humans between 18 and 65 years old.
4.1. Summary of Key findings and interpretation
It is important to note that our searches aimed mainly at the evaluation of gene expression and anti-inflammatory activity after eicosapentaenoic and docosahexaenoic acids intake, because they are poorly investigated compared to other macro and micronutrients.
Long-chain polyunsaturated fatty acids have emerged as a potential protective nutrient against the cardiometabolic risks associated with obesity (33), where a higher body mass index has been linked with low omega-3 status among adults (34–36). In this sense, our study found a decrease in body composition and fat mass after eicosapentaenoic and docosahexaenoic acid intake (18). Indeed, previous studies described a decreased body mass index after consuming eicosapentaenoic acid; where a 4.35% weight loss was found after 12 weeks (37, 38), confirming its protective role.
There exists a widespread association between obesity and inflammation (17, 21, 23, 25, 39). This correlation is characterized by an elevation in mRNA levels of IL-6, IL-12, IFN, and CXCL10 chemokines in individuals with obesity. Adiponectin activity exhibits a protective effect in multiple physiological processes, including energy metabolism, inflammation, and cell proliferation. Furthermore, it has been implicated in the mitigation of chronic non-communicable conditions such as diabetes mellitus (40–42). The present study has observed elevated levels of PPAR-γ and adiponectin subsequent to the intake of eicosapentaenoic and docosahexaenoic acid (18, 23, 25). These findings provide support for the beneficial effects of omega-3 long-chain polyunsaturated fatty acids. A noteworthy discovery pertaining to eicosapentaenoic and docosahexaenoic acids is their ability to mitigate inflammation triggered by lipopolysaccharide (21). This implies that eicosapentaenoic and docosahexaenoic acids serve as primary inhibitors of this inflammatory response (17, 43).
Both eicosapentaenoic and docosahexaenoic acids have the primary effect of lowering triglyceride levels by reducing the synthesis of very-low-density lipoprotein-triglycerides in the liver (44). In addition, women living with obesity were observed to have lower triglyceride levels after consuming eicosapentaenoic and docosahexaenoic acids (45, 46). However, even though consuming omega-3 long-chain polyunsaturated fatty acids led to lower levels of fatty acids (19, 22, 47), no definite effects on triglycerides have been reported (19, 20).
The American Heart Association has described the advantages of omega-3 long-chain polyunsaturated fatty acid consumption to lower hypertriglyceridemia and the variables that affect its measurement to examine these discrepancies (13). In this instance, phospholipase A2 polymorphisms were investigated, and triglyceride levels in humans living with obesity were found to be correlated with SNPs (19). In addition, alterations in the expression of certain enzymes, like PLA2G2D, would reduce eicosapentaenoic and docosahexaenoic acids in murine models (48), which could partially account for the disparity.
Dietary polyunsaturated fatty acid intake may control the parameters related to obesity through different epigenetic mechanisms. There is current information on the genetic modulation of ALOX5 and ALOX15 expression during obesity (23), where ALOX5 promotes leukotrienes, lipoxins, and resolvins production (46). However, ALOX12 and ALOX15 genes appear to have anti-inflammatory and inflammatory effects, respectively (49). Likewise, ALOX12 pro-inflammatory activity was decreased after omega-3 intake (23, 25, 37). Although ALOX12 and ALOX15 could have pro-inflammatory activity, our results might be explained because ALOX12 and ALOX15 are involved in eicosapentaenoic and docosahexaenoic acid metabolism (49). However, more studies are still required to understand the relationship between genomics, obesity, and polyunsaturated fatty acids.
After polyunsaturated fatty acid consumption, there would be a correlation between obesity and inflammation, decreasing pro-inflammatory genes and cytokines expression, such as VCAM-1, 8-epi-prostaglandin-F2α (8-EPI), and TNF-α (20). According to previous studies, polyunsaturated fatty acids decrease sVCAM-1 and TNF-α in people living with obesity (24, 40, 42, 46).
While inflammation serves a healthy function, uncontrolled inflammation can have negative consequences, leading to tissue damage and the development of many diseases. In these circumstances, inflammation has a self-limiting nature and the initiation of active resolution mechanisms. At the core of these processes lies the production of specialized pro-resolving lipid mediators (SPMs) derived from EPA and DHA. Resolvins, protectins, and maresins, which have been extensively characterized in cellular and animal models, are among the compounds under consideration (50, 51).
SPMs encompass a collection of anti-inflammatory mediators that can be categorized into four distinct families, namely lipoxins (LX), resolvins (Rv), protectins (PD), and marosins (MaR) (52). SPMs are produced from the same precursors as proinflammatory mediators; however, the mechanisms exhibit significant differences. These structures have the ability to selectively attach to particular receptors, thereby reinstating homeostasis through the reduction of cellular activity and inflammation (53). In recent times, there has been an increasing recognition of the potential therapeutic value of mediators in the treatment of inflammatory illnesses. Research on the physiology of resolution has led to the exploration of novel research domains, spanning from fundamental physiology and pharmacology to the identification of potential therapeutic targets (54).
Previous studies have demonstrated the efficacy of SPMs in attenuating the advancement of cardiovascular disease (CVD) through the modulation of many molecular pathways implicated in the pathogenesis of CVD (54–57). SPMs refer to specialized pro-resolving mediators, which are the metabolic byproducts derived from ω-3 and ω-6 polyunsaturated fatty acids (PUFAs) through the enzymatic actions of lipoxygenase (LOX), cyclooxygenase-2 (COX-2), and, to a lesser extent, cytochrome P450.
Animal studies have shown that not having enough lipooxygenase leads to more epoxyeicosatrienoic acid and SPMs being made. These variables have the ability to regulate the activation of proinflammatory pathways, promoting cardiac repair and minimizing cardiac remodeling in both acute and chronic heart failure conditions (58, 59). The cellular impacts of SPMs are derived from their interaction with distinct G protein-coupled transmembrane receptors (GPCRs), namely ALX/FPR2, GPR32/DRV1, ChemR23, BLT1, GPR37, and GPR18/DRV2 (60). In general, SPMs exhibit common signaling pathways such as intracellular phosphorylation cascades and gene regulation, with the exception of PD1, which induces intracellular calcium elevation and subsequently activates calcium-dependent signaling pathways (61).
The sole receptor previously believed to be accountable for the biological consequences of LX is the formyl peptide receptor 2 (FPR2), or ALX. In addition to LXA4, the ALX/FPR2 receptor is also stimulated by RvD1 and RvD3. However, as of now, no receptors that bind to LXB4 have been discovered (62). The influence of RvD1 on the phosphorylation of AKT through the PI3K pathway results in the inhibition of proinflammatory effects mediated by NF-kB. Additionally, it has been observed that it augments ERK1/2 phosphorylation through MEK1/2 activation, hence eliciting anti-inflammatory responses (63, 64). The activation of the nuclear factor erythroid 2-related factor 2 (NRF2) occurs through the interaction with LXA4, leading to the induction of phosphorylation at the Ser40 residue. This phosphorylation event subsequently facilitates the translocation of NRF2 into the nucleus. Phosphorylated NRF2 has the capability to establish a heterodimeric association with sMAF, leading to the formation of a complex that can bind to the antioxidant response element (ARE). This binding event subsequently triggers the transcriptional activation of various antioxidant genes, including HO-1, NQO-1, SOD, and TXN (65).
The RvD1 receptor, known as GPR32/DRV1, belongs to the GPCR family and exhibits a similar binding affinity for RvD3 and RvD5 (25). The connection between Resolvin D1 (RvD1) and G-protein-coupled receptor 32 (GPR32)/DRV1 leads to enhanced production of several microRNAs (miRNAs) in macrophages. One such miRNA is miR-208a, which hinders the transcription process of tumor suppressor protein 4 (PDCD4) and consequently leads to an elevation in the levels of interleukin-10 (IL-10). Furthermore, it has been observed that there is an augmentation in the production of miR146b, a molecule that effectively suppresses the transcription of NF-κB (66). Furthermore, it is worth noting that GPR18/DRV2 belongs to the same family and has been identified as the sole receptor for RvD2. The activation of this entity induces the ERK1/2, PKA, or PLC pathway in order to facilitate resolution. In contrast, GPR37 exclusively interacts with PD1, hence inhibiting PKA and triggering calcium-dependent signaling pathways that ultimately result in the promotion of phagocytosis and the modulation of cytokine production (67).
The expression of ALX/FRP2, GPR32/DRV1, ChemR23, and GPR18/DRV2 is not restricted only to immune system cells. These receptors have also been detected in vascular smooth muscle cells (VSMC), endothelial cells, and atherosclerotic lesions (68–70). Moreover, when endothelial cells were exposed to docosahexaenoic acid (DHA), there was a notable decrease in the adhesion and migration of immune cells. This effect was significantly attenuated when the receptors for Resolvin D1 (RvD1), namely ALX/FPR2 and GPR32/DRV1, were blocked.
However, there are a number of pathogenic and therapeutic factors that can affect the intricate involvement of endogenous SPMs in the context of chronic inflammation and disrupt their typical functioning. For instance, multiple studies conducted on animal models have demonstrated that the resolving response may be altered by the combination of age and obesity generated by a diet rich in PUFAs (71, 72). The observed phenomenon could potentially be attributed to the increased movement of neutrophils and the presence of a significant number of proinflammatory cytokines and lipid mediators within the cardioplegic and cardiorenal networks (71, 72). Molecules such as doxorubicin (73), carprofen (74), and FPR2 inhibitors (75) have the ability to disrupt immunometabolic responses by decreasing the synthesis of SPMs and altering the maturation of leukocytes. Consequently, the resolution of the chronic inflammatory process is prolonged.
4.2. Scope and limitations
The goal was to seek an explanation that would reconcile prior conflicting findings concerning the role of long-chain polyunsaturated fatty acids, eicosapentaenoic, and docosahexaenoic, in the regulation of gene expression during the development of obesity. Our review confirms that eicosapentaenoic acid and docosahexaenoic acid participate in the regulation of gene expression by modifying components of fatty acid metabolism. Indeed, eicosapentaenoic acid and docosahexaenoic acid generate epigenetic changes in fatty acid metabolism, which are evidenced through changes in gene expression, anthropometric measurements, biochemical markers, and inflammation.
Unfortunately, our review had some limitations, i.e., a low quantity of articles linking omega-3 polyunsaturated fatty acids to gene expression and their influence on obesity. Also, the intervention times were highly variable between studies, with significant differences in the number of weeks and days. Finally, some studies did not provide sufficient data to compare the results obtained before and after the intervention, and some did not even incorporate the baseline measurements for the parameters studied, which limited the extraction of information.
5. Conclusion
Obesity is an alarmingly increasing public health issue. Obesity prevention is a critical factor in controlling obesity-related non-communicable diseases, including diabetes, cardiovascular disease, stroke, hypertension, cancer, and psychological problems.
Our results suggest that eicosapentaenoic and docosahexaenoic acids could be beneficial and effective against noncommunicable diseases, such as obesity, in people over 18 years old thanks to the anti-inflammatory role and the modulation of obesity-regulating genes such as PPARγ and those belonging to the ALOX family.
The preclinical evidence suggests that SPMs have potential as innovative and promising agents in the prevention and management of CVD. This can be achieved by either enhancing the endogenous synthesis of SPMs through supplementation of PUFAs and other molecules that promote their production, or by administering exogenous synthetic analogs of SPMs, either alone or in conjunction with other drugs that protect the heart. Moreover, it has been observed in both in vitro and in vivo preclinical investigations that SPMs enhance host defenses, which distinguishes them from existing anti-inflammatory therapies. Consequently, it is anticipated that these findings will lead to the development of novel guidelines pertaining to the utilization of SPMs in the management of cardiovascular disease.
Data availability statement
The original contributions presented in the study are included in the article/supplementary material, further inquiries can be directed to the corresponding authors.
Author contributions
CS: Conceptualization, Writing – original draft, Writing – review & editing. KN: Data curation, Formal analysis, Investigation, Writing – original draft, Writing – review & editing. LM: Data curation, Formal analysis, Investigation, Writing – original draft, Writing – review & editing. BR: Data curation, Formal analysis, Investigation, Writing – original draft, Writing – review & editing. VS-M: Supervision, Writing – original draft, Writing – review & editing. JF: Writing – original draft, Writing – review & editing, Conceptualization.
Funding
The author(s) declare financial support was received for the research, authorship, and/or publication of this article. This research was funded by [Programa de Formación de Investigadores Postdoctorales, Universidad de La Frontera] grant number [PDT22- 0001]; [DIUFRO, Universidad de La Frontera] grant number [DI22-0007], and [SmartC-BIOREN, Service Management Analytical Research and Training Center, Agencia Nacional de Investigación y Desarrollo de Chile] grant number [CCSS210005].
Conflict of interest
The authors declare that the research was conducted in the absence of any commercial or financial relationships that could be construed as a potential conflict of interest.
The author(s) declared that they were an editorial board member of Frontiers, at the time of submission. This had no impact on the peer review process and the final decision.
Publisher’s note
All claims expressed in this article are solely those of the authors and do not necessarily represent those of their affiliated organizations, or those of the publisher, the editors and the reviewers. Any product that may be evaluated in this article, or claim that may be made by its manufacturer, is not guaranteed or endorsed by the publisher.
References
1. Chooi, YC, Ding, C, and Magkos, F. The epidemiology of obesity. Metabolism. (2019) 92:6–10. doi: 10.1016/j.metabol.2018.09.005
2. NCD Risk Factor Collaboration (NCD-RisC). Worldwide trends in body-mass index, underweight, overweight, and obesity from 1975 to 2016: a pooled analysis of 2416 population-based measurement studies in 128·9 million children, adolescents, and adults. Lancet. (2017) 390:2627–42. doi: 10.1016/S0140-6736(17)32129-3
3. Spiegelman, BM, and Flier, JS. Obesity and the regulation of energy balance. Cells. (2001) 104:531–43. doi: 10.1016/s0092-8674(01)00240-9
4. Swinburn, BA, Caterson, I, Seidell, JC, and James, WPT. Diet, nutrition and the prevention of excess weight gain and obesity. Public Health Nutr. (2004) 7:123–46. doi: 10.1079/phn2003585
5. Bogyo, M, and Rudd, PM. New technologies and their impact on ‘omics’ research. Curr Opin Chem Biol. (2013) 17:1–3. doi: 10.1371/journal.pone.0144996
6. Bordoni, L, and Gabbianelli, R. Primers on nutrigenetics and nutri(epi)genomics: origins and development of precision nutrition. Biochimie. (2019) 160:156–71. doi: 10.1016/j.biochi.2019.03.006
7. Abedi, E, and Ali Sahari, M. Long-chain polyunsaturated fatty acid sources and evaluation of their nutritional and functional properties. Food Sci Nutr. (2014) 2:443–63. doi: 10.1002/fsn3.121
8. Al-Khalaifah, H. Modulatory effect of dietary polyunsaturated fatty acids on immunity, represented by phagocytic activity. Front Vet Sci. (2020) 7:569939. doi: 10.3389/fvets.2020.569939
9. Swanson, D, Block, R, and Mousa, SA. Omega-3 fatty acids EPA and DHA: health benefits throughout life. Adv Nutr (Bethesda). (2012) 3:1–7. doi: 10.3945/an.111.000893
10. Haghiac, M, Yang, XH, Presley, L, Smith, S, Dettelback, S, Minium, J, et al. Dietary Omega-3 fatty acid supplementation reduces inflammation in obese pregnant women: a randomized double-blind controlled clinical trial. PLoS One. (2015) 10:e0137309. doi: 10.1371/journal.pone.0137309
11. Miller, M, Stone, NJ, Ballantyne, C, Bittner, V, Criqui, MH, Ginsberg, HN, et al. Triglycerides and cardiovascular disease: a scientific statement from the American Heart Association. Circulation. (2011) 123:2292–333. doi: 10.1161/CIR.0b013e3182160726
12. Shearer, GC, Savinova, OV, and Harris, WS. Fish oil – how does it reduce plasma triglycerides? Biochim Biophys Acta. (2012) 1821:843–51. doi: 10.1016/j.bbalip.2011.10.011
13. Skulas-Ray, AC, West, SG, Davidson, MH, and Kris-Etherton, PM. Omega-3 fatty acid concentrates in the treatment of moderate hypertriglyceridemia. Expert Opin Pharmacother. (2008) 9:1237–48. doi: 10.1517/14656566.9.7.1237
14. Petracci, I, Gabbianelli, R, and Bordoni, L. The role of Nutri(epi)genomics in achieving the Body’s full potential in physical activity. Antioxidants. (2020) 9:498. doi: 10.3390/antiox9060498
15. Moher, D, Liberati, A, Tetzlaff, J, and Altman, DG, PRISMA Group. Preferred reporting items for systematic reviews and meta-analyses: the PRISMA statement. PLoS Med. (2009) 339:e1000097. doi: 10.1136/bmj.b2535
16. National Institute for Health and Care Excellence. Appendix F quality appraisal checklist – Quantitative intervention studies methods for the development of NICE Public health guidance. London: National Institute for Health and Care Excellence (2012).
17. Labonté, ME, Dewailly, E, Lucas, M, Couture, P, and Lamarche, B. Association of red blood cell n-3 polyunsaturated fatty acids with plasma inflammatory biomarkers among the Quebec Cree population. Eur J Clin Nutr. (2014) 68:1042–7. doi: 10.1038/ejcn.2014.125
18. Kratz, M, Swarbrick, MM, Callahan, HS, Matthys, CC, Havel, PJ, and Weigle, DS. Effect of dietary n-3 polyunsaturated fatty acids on plasma total and high-molecular-weight adiponectin concentrations in overweight to moderately obese men and women. Am J Clin Nutr. (2008) 87:347–53. doi: 10.1093/ajcn/87.2.347
19. Tremblay, BL, Cormier, H, Rudkowska, I, Lemieux, S, Couture, P, and Vohl, MC. Association between polymorphisms in phospholipase A2 genes and the plasma triglyceride response to an n-3 PUFA supplementation: a clinical trial. Lipids Health Dis. (2015) 14:12. doi: 10.1186/s12944-015-0009-2
20. Peairs, AD, Rankin, JW, and Lee, YW. Effects of acute ingestion of different fats on oxidative stress and inflammation in overweight and obese adults. Nutr J. (2011) 10:122. doi: 10.1186/1475-2891-10-122
21. Ferguson, JF, Xue, C, Hu, Y, Li, M, and Reilly, MP. Adipose tissue RNASeq reveals novel gene-nutrient interactions following n-3 PUFA supplementation and evoked inflammation in humans. J Nutr Biochem. (2016) 30:126–32. doi: 10.1016/j.jnutbio.2015.12.010
22. Fisk, HL, Childs, CE, Miles, EA, Ayres, R, Noakes, PS, Paras-Chavez, C, et al. Dysregulation of endocannabinoid concentrations in human subcutaneous adipose tissue in obesity and modulation by omega-3 polyunsaturated fatty acids. Clin Sci (Lond). (2021) 135:185–00. doi: 10.1042/CS20201060
23. Fisk, HL, Childs, CE, Miles, EA, Ayres, R, Noakes, PS, Paras-Chavez, C, et al. Modification of subcutaneous white adipose tissue inflammation by omega-3 fatty acids is limited in human obesity-a double blind, randomised clinical trial. EBioMedicine. (2022a) 77:103909. doi: 10.1016/j.ebiom.2022.103909
24. Hernandez, JD, Li, T, Rau, CM, LeSuer, WE, Wang, P, Coletta, DK, et al. ω-3PUFA supplementation ameliorates adipose tissue inflammation and insulin-stimulated glucose disposal in subjects with obesity: a potential role for apolipoprotein E. Int J Obes. (2021) 45:1331–41. doi: 10.1038/s41366-021-00801-w
25. Fisk, HL, Childs, CE, Miles, EA, Ayres, R, Noakes, PS, Paras-Chavez, C, et al. Dysregulation of subcutaneous white adipose tissue inflammatory environment modelling in non-insulin resistant obesity and responses to Omega-3 fatty acids - a double blind. Random Clin Trial Front Immunol. (2022b) 13:922654. doi: 10.3389/fimmu.2022.922654
26. Mathieu, P, Poirier, P, Pibarot, P, Lemieux, I, and Despres, JP. Visceral obesity: the link among inflammation, hypertension, and cardiovascular disease. Hypertension. (2009) 53:577–84. doi: 10.1161/HYPERTENSIONAHA.108.110320
27. Calabro, P, and Yeh, ET. Intra-abdominal adiposity, inflammation, and cardiovascular risk: new insight into global cardiometabolic risk. Curr Hypertens Rep. (2008) 10:32–8. doi: 10.1007/s11906-008-0008-z
28. Despres, JP, Arsenault, BJ, Cote, M, Cartier, A, and Lemieux, I. Abdominal obesity: the cholesterol of the 21st century? Can J Cardiol. (2008) 24:7D–12D. doi: 10.1016/s0828-282x(08)71043-2
29. Hevener, AL, and Febbraio, MA. The 2009 stock conference report: inflammation, obesity and metabolic disease. Obes Rev. (2010) 11:635–44. doi: 10.1111/j.1467-789X.2009.00691.x
30. Al-Mansoori, L, Al-Jaber, H, Prince, MS, and Elrayess, MA. Role of inflammatory cytokines, growth factors and Adipokines in Adipogenesis and insulin resistance. Inflammation. (2022) 45:31–44. doi: 10.1007/s10753-021-01559-z
31. Tilg, H, and Moschen, AR. Adipocytokines: mediators linking adipose tissue, inflammation and immunity. Nat Rev Immunol. (2006) 6:772–83. doi: 10.1038/nri1937
32. Tilg, H, and Moschen, AR. Inflammatory mechanisms in the regulation of insulin resistance. Mol Med. (2008) 14:222–31. doi: 10.2119/2007-00119.Tilg
33. Young, IE, Parker, HM, Cook, RL, O’Dwyer, NJ, Garg, ML, Steinbeck, KS, et al. Association between obesity and Omega-3 status in healthy Young women. Nutrients. (2020) 12:1480. doi: 10.3390/nu12051480
34. Cazzola, R, Rondanelli, M, Russo-Volpe, S, Ferrari, E, and Cestaro, B. Decreased membrane fluidity and altered susceptibility to peroxidation and lipid composition in overweight and obese female erythrocytes. J Lipid Res. (2004) 45:1846–51. doi: 10.1194/jlr.M300509-JLR200
35. Micallef, M, Munro, I, Phang, M, and Garg, M. Plasma n-3 polyunsaturated fatty acids are negatively associated with obesity. Br J Nutr. (2009) 102:1370–4. doi: 10.3390/ijms17101689
36. Mingay, E, Veysey, M, Lucock, M, Niblett, S, King, K, Patterson, A, et al. Sex-dependent association between omega-3 index and body weight status in older Australians. J Nutr Intermed Metab. (2016) 5:70–7. doi: 10.1016/j.jnim.2016.04.001
37. Berthelot, CC, Kamita, SG, Sacchi, R, Yang, J, Nording, ML, Georgi, K, et al. Changes in PTGS1 and ALOX12 gene expression in peripheral blood mononuclear cells are associated with changes in arachidonic acid, Oxylipins, and Oxylipin/fatty acid ratios in response to Omega-3 fatty acid supplementation. PLoS One. (2015) 10:e0144996
38. Munro, IA, and Garg, ML. Dietary supplementation with long chain omega-3 polyunsaturated fatty acids and weight loss in obese adults. Obes Res Clin Pract. (2013) 7:e173–81. doi: 10.1016/j.orcp.2011.11.001
39. Dobrian, AD, Lieb, DC, Ma, Q, Lindsay, JW, Cole, BK, Ma, K, et al. Differential expression and localization of 12/15 lipoxygenases in adipose tissue in human obese subjects. Biochem Biophys Res Commun. (2010) 403:485–90. doi: 10.1016/j.bbrc.2010.11.065
40. D'Archivio, M, Scazzocchio, B, Giammarioli, S, Fiani, ML, Varì, R, Santangelo, C, et al. ω3-PUFAs exert anti-inflammatory activity in visceral adipocytes from colorectal cancer patients. PLoS One. (2013) 8:e77432. doi: 10.1371/journal.pone.0077432
41. Mostowik, M, Gajos, G, Zalewski, J, Nessler, J, and Undas, A. Omega-3 polyunsaturated fatty acids increase plasma adiponectin to leptin ratio in stable coronary artery disease. Cardiovasc Drugs Ther. (2013) 27:289–95. doi: 10.1007/s10557-013-6457-x
42. Song, J, Li, C, Lv, Y, Zhang, Y, Amakye, WK, and Mao, L. DHA increases adiponectin expression more effectively than EPA at relative low concentrations by regulating PPARγ and its phosphorylation at Ser273 in 3T3-L1 adipocytes. Nutr Metab (Lond). (2017) 14:52. doi: 10.1186/s12986-017-0209-z
43. Lee, JY, Plakidas, A, Lee, WH, Heikkinen, A, Chanmugam, P, Bray, G, et al. Differential modulation of toll-like receptors by fatty acids: preferential inhibition by n-3 polyunsaturated fatty acids. J Lipid Res. (2003) 44:479–86. doi: 10.1194/jlr.M200361-JLR200
44. Oscarsson, J, and Hurt-Camejo, E. Omega-3 fatty acids eicosapentaenoic acid and docosahexaenoic acid and their mechanisms of action on apolipoprotein B-containing lipoproteins in humans: a review. Lipids Health Dis. (2017) 16:149. doi: 10.1186/s12944-017-0541-3
45. Koh, KK, Quon, MJ, Shin, KC, Lim, S, Lee, Y, Sakuma, I, et al. Significant differential effects of omega-3 fatty acids and fenofibrate in patients with hypertriglyceridemia. Atherosclerosis. (2018) 220:537–44. doi: 10.1016/j.atherosclerosis.2011.11.018
46. Polus, A, Zapala, B, Razny, U, Gielicz, A, Kiec-Wilk, B, Malczewska-Malec, M, et al. Omega-3 fatty acid supplementation influences the whole blood transcriptome in women with obesity, associated with pro-resolving lipid mediator production. Biochim Biophys Acta. (2016) 1861:1746–55. doi: 10.1016/j.bbalip.2016.08.005
47. Barber, E, Sinclair, AJ, and Cameron-Smith, D. Comparative actions of omega-3 fatty acids on in-vitro lipid droplet formation. Prostaglandins Leukot Essent Fat Acids. (2013) 89:359–66. doi: 10.1016/j.plefa.2013.07.006
48. Sato, H, Taketomi, Y, Miki, Y, Murase, R, Yamamoto, K, and Murakami, M. Secreted phospholipase PLA2G2D contributes to metabolic health by mobilizing ω3 polyunsaturated fatty acids in WAT. Cell Rep. (2020) 31:107579. doi: 10.1016/j.celrep.2020.107579
49. Singh, NK, and Rao, GN. Emerging role of 12/15-lipoxygenase (ALOX15) in human pathologies. Prog Lipid Res. (2019) 73:28–45. doi: 10.1016/j.plipres.2018.11.001
50. Chen, L, Deng, H, Cui, H, Fang, J, Zuo, Z, Deng, J, et al. Inflammatory responses and inflammation-associated diseases in organs. Oncotarget. (2017) 9:7204–18. doi: 10.18632/oncotarget.23208
51. Salazar, J, Pirela, D, Nava, M, Castro, A, Angarita, L, Parra, H, et al. Specialized Proresolving lipid mediators: a potential therapeutic target for atherosclerosis. Int J Mol Sci. (2022) 23:3133. doi: 10.3390/ijms23063133
52. Buckley, CD, Gilroy, DW, and Serhan, CN. Pro-resolving lipid mediators and mechanisms in the resolution of acute inflammation. Immunity. (2014) 40:315–27. doi: 10.1016/j.immuni.2014.02.009
53. Basil, MC, and Levy, BD. Specialized pro-resolving mediators: endogenous regulators of infection and inflammation. Nat Rev Immunol. (2016) 16:51–67. doi: 10.1038/nri.2015.4
54. Park, J, Langmead, CJ, and Riddy, DM. New advances in targeting the resolution of inflammation: implications for specialized pro-resolving mediator GPCR drug discovery. ACS Pharmacol Transl Sci. (2020) 3:88–06.
55. Fredman, G, and Spite, M. Specialized pro-resolving mediators in cardiovascular diseases. Mol Asp Med. (2017) 58:65–71. doi: 10.1016/j.mam.2017.02.003
56. Kasikara, C, Doran, AC, Cai, B, and Tabas, I. The role of non-resolving inflammation in atherosclerosis. J Clin Investig. (2018) 128:2713–23. doi: 10.1172/JCI97950
57. Viola, JR, Lemnitzer, P, Jansen, Y, Csaba, G, Winter, C, Neideck, C, et al. Resolving lipid mediators Maresin 1 and Resolvin D2 prevent Atheroprogression in mice. Circ Res. (2016) 119:1030–8. doi: 10.1161/CIRCRESAHA.116.309492
58. Halade, GV, Kain, V, Ingle, KA, and Prabhu, SD. Interaction of 12/15-lipoxygenase with fatty acids alters the leukocyte kinetics leading to improved postmyocardial infarction healing. Am J Physiol Heart Circ Physiol. (2017) 313:H89–H102. doi: 10.1152/ajpheart.00040.2017
59. Halade, GV, Kain, V, Tourki, B, and Jadapalli, JK. Lipoxygenase drives lipidomic and metabolic reprogramming in ischemic heart failure. Metabolism. (2019) 96:22–32. doi: 10.1016/j.metabol.2019.04.011
60. Chiang, N, and Serhan, CN. Structural elucidation and physiologic functions of specialized pro-resolving mediators and their eceptors. Mol Asp Med. (2017) 58:114–29. doi: 10.1016/j.mam.2017.03.005
61. Bang, S, Xie, Y-K, Zhang, Z-J, Wang, Z, Xu, Z-Z, et al. GPR37 regulates macrophage phagocytosis and resolution of inflammatory pain. J Clin Investig. (2018) 128:3568–82. doi: 10.1172/JCI99888
62. Dalli, J, and Serhan, CN. Identification and structure elucidation of the pro-resolving mediators provides novel leads for resolution pharmacology. Br J Pharmacol. (2019) 176:1024–37. doi: 10.1111/bph.14336
63. Doyle, R, Sadlier, DM, and Godson, C. Pro-resolving lipid mediators: agents of anti-ageing? Semin Immunol. (2018) 40:36–48. doi: 10.1016/j.smim.2018.09.002
64. Han, Y-H, Lee, K, Saha, A, Han, J, Choi, H, Noh, M, et al. Specialized Proresolving mediators for therapeutic interventions targeting metabolic and inflammatory disorders. Biomol Ther. (2021) 29:455–64. doi: 10.4062/biomolther.2021.094
65. Kang, GJ, Kim, EJ, and Lee, CH. Therapeutic effects of specialized pro-resolving lipids mediators on cardiac fibrosis via NRF2 activation. Antioxidants. (2020) 9:1259. doi: 10.3390/antiox9121259
66. Recchiuti, A. Resolvin D1 and its GPCRs in resolution circuits of inflammation. Prostaglandins Other Lipid Mediat. (2013) 107:64–76. doi: 10.1016/j.prostaglandins.2013.02.004
67. McCrary, MR, Jiang, MQ, Giddens, MM, Zhang, JY, Owino, S, Wei, ZZ, et al. Protective effects of GPR37 via regulation of inflammation and multiple cell death pathways after ischemic stroke in mice. FASEB J. (2019) 33:10680–91. doi: 10.1096/fj.201900070R
68. Carracedo, M, Artiach, G, Arnardottir, H, and Bäck, M. The resolution of inflammation through omega-3 fatty acids in atherosclerosis, intimal hyperplasia, and vascular calcification. Semin Immunopathol. (2019) 41:757–66. doi: 10.1007/s00281-019-00767-y
69. Chattopadhyay, R, Mani, AM, Singh, NK, and Rao, GN. Resolvin D1 blocks H2O2-mediated inhibitory crosstalk between SHP2 and PP2A and suppresses endothelial-monocyte interactions. Free Radic Biol Med. (2018) 117:119–31. doi: 10.1016/j.freeradbiomed.2018.01.034
70. Chattopadhyay, R, Raghavan, S, and Rao, GN. Resolvin D1 via prevention of ROS-mediated SHP2 inactivation protects endothelial adherens junction integrity and barrier function. Redox Biol. (2017) 12:438–55. doi: 10.1016/j.redox.2017.02.023
71. Halade, GV, Kain, V, Black, LM, Prabhu, SD, and Ingle, KA. Aging dysregulates D- and E-series resolvins to modulate cardios- plenic and cardiorenal network following myocardial infarction. Aging. (2016) 8:2611–34. doi: 10.18632/aging.101077
72. Lopez, EF, Kabarowski, JH, Ingle, KA, Kain, V, Barnes, S, Crossman, D, et al. Obesity superimposed on aging magnifies inflammation and delays the resolving response after myocardial infarction. Am. J. Physiol. Circ. Physiol. (2015) 308:H269–80. doi: 10.1152/ajpheart.00604.2014
73. Jadapalli, JK, Wright, GW, Kain, V, Sherwani, MA, Sonkar, R, Yusuf, N, et al. Doxorubicin triggers splenic contraction and irreversible dysregulation of COX and LOX that alters the inflammation-resolution program in the myocardium. Am J Physiol Circ Physiol. (2018) 315:H1091–100. doi: 10.1152/ajpheart.00290.2018
74. Halade, GV, Kain, V, Wright, GM, and Jadapalli, JK. Subacute treatment of carprofen facilitate splenocardiac resolution deficit in cardiac injury. J Leukoc Biol. (2018) 104:1173–86. doi: 10.1002/JLB.3A0618-223R
Keywords: gene expression, nutrigenomics, omega-3, epigenetics, molecular biology
Citation: Sandoval C, Nahuelqueo K, Mella L, Recabarren B, Souza-Mello V and Farías J (2023) Role of long-chain polyunsaturated fatty acids, eicosapentaenoic and docosahexaenoic, in the regulation of gene expression during the development of obesity: a systematic review. Front. Nutr. 10:1288804. doi: 10.3389/fnut.2023.1288804
Edited by:
Shuang Song, Dalian Polytechnic University, ChinaReviewed by:
Anandita Pal, University of North Carolina at Chapel Hill, United StatesBrian M. Shewchuk, East Carolina University, Greenville, United States
Copyright © 2023 Sandoval, Nahuelqueo, Mella, Recabarren, Souza-Mello and Farías. This is an open-access article distributed under the terms of the Creative Commons Attribution License (CC BY). The use, distribution or reproduction in other forums is permitted, provided the original author(s) and the copyright owner(s) are credited and that the original publication in this journal is cited, in accordance with accepted academic practice. No use, distribution or reproduction is permitted which does not comply with these terms.
*Correspondence: Cristian Sandoval, cristian.sandoval@ufrontera.cl; Jorge Farías, jorge.farias@ufrontera.cl