- Independent Researcher, Vilnius, Lithuania
It has been unexplained why chronic pain does not invariably accompany chronic pain-prone disorders. This question-driven, hypothesis-based article suggests that the reason may be varying occurrence of concomitant peripheral compressive proximal neural lesion (cPNL), e.g., radiculopathy and entrapment plexopathies. Transition of acute to chronic pain may involve development or aggravation of cPNL. Nociceptive hypersensitivity induced and/or maintained by cPNL may be responsible for all types of general chronic pain as well as for pain in isolated tissue conditions that are usually painless, e.g., neuroma, scar, and Dupuytren's fibromatosis. Compressive PNL induces focal neuroinflammation, which can maintain dorsal root ganglion neuron (DRGn) hyperexcitability (i.e., peripheral sensitization) and thus fuel central sensitization (i.e., hyperexcitability of central nociceptive pathways) and a vicious cycle of chronic pain. DRGn hyperexcitability and cPNL may reciprocally maintain each other, because cPNL can result from reflexive myospasm-induced myofascial tension, muscle weakness, and consequent muscle imbalance- and/or pain-provoked compensatory overuse. Because of pain and motor fiber damage, cPNL can worsen the causative musculoskeletal dysfunction, which further accounts for the reciprocity between the latter two factors. Sensitization increases nerve vulnerability and thus catalyzes this cycle. Because of these mechanisms and relatively greater number of neurons involved, cPNL is more likely to maintain DRGn hyperexcitability in comparison to distal neural and non-neural lesions. Compressive PNL is associated with restricted neural mobility. Intermittent (dynamic) nature of cPNL may be essential in chronic pain, because healed (i.e., fibrotic) lesions are physiologically silent and, consequently, cannot provide nociceptive input. Not all patients may be equally susceptible to develop cPNL, because occurrence of cPNL may vary as vary patients' predisposition to musculoskeletal impairment. Sensitization is accompanied by pressure pain threshold decrease and consequent mechanical allodynia and hyperalgesia, which can cause unusual local pain via natural pressure exerted by space occupying lesions or by their examination. Worsening of local pain is similarly explainable. Neuroma pain may be due to cPNL-induced axonal mechanical sensitivity and hypersensitivity of the nociceptive nervi nervorum of the nerve trunk and its stump. Intermittence and symptomatic complexity of cPNL may be the cause of frequent misdiagnosis of chronic pain.
Introduction
Why are some neuromas painful, while others are painless? Why do some small articular fractures result in a very painful osteoarthritis, while some disastrous articular injuries may go asymptomatic? Similar questions can be asked regarding common ganglia of the hand, rheumatoid arthritis, Dupuytren's fibromatosis, and scars. It is also puzzling why some patients with systemic diseases develop chronic pain, while others remain relatively pain free. Many patients are making long diagnostic and therapeutic odysseys due to relentless pain of unclear etiology. Although numerous risk factors for chronic pain have been found, there is no straightforward common answer to the above questions of persistent pain.
Chronic pain, i.e., pain that lasts more than 3 months (1), is thought to be principally due to central sensitization (2–4), which is driven by neuroinflammation in many chronic pain conditions (5–7). Nevertheless, the mechanism of transition of acute to chronic pain remains abstract despite arduous research (8–15) and amounting data on relevant molecular and physiologic factors. In terms of the evolutionary purpose of pain, the answer to the question why acute pain transforms into chronic pain is simple: because tissue damage, i.e., noxious input and resultant nociceptive stimulus (16), persists. While the importance of initial noxious trigger in the development of sensitization and chronic pain has been well recognized, the current theories of chronic pain tend to be focused on susceptibility to and central processing of pain (8, 17–19). A clinical category of nociplastic pain has been recently introduced to cover persistent pain supposedly driven by central sensitization without any peripheral input (20). However, experimental research that supports autonomous (continuing input-independent) central sensitization is relatively scanty (4, 5) and is outweighed by contrary evidence (21–24). Although central mechanisms of sensitization are important, they cannot completely account for such chronic pain features as ongoingness, spontaneity, and, most importantly, individual variability in occurrence. If central processes, such as, e.g., synaptic potentiation and disinhibition (5, 16, 25, 26), would invariably persist on their own after disappearance of noxious trigger, then chronic pain would likely afflict all patients with identical chronic pain-prone conditions.
However, where can nociceptive input come from when there is no obvious pathology? Could the cause be an occult tissue lesion generated by the initial noxious input? Compressive nerve lesions often arise in this way, specifically, because of changes of paraneural tissue. Peripheral nerve injury as one of the causes of chronic pain has been well acknowledged (5) but primarily linked to neuropathic pain (27–29). Yet, there is increasing evidence of neuropathic etiology of chronic pain in conditions commonly thought to be of non-neural origin, e.g., complex regional pain syndrome (CRPS) type I (30–33), fibromyalgia (34–36), and a number of other diseases found to be associated with small fiber neuropathy (SFN) (37–39). The unitary neural etiology of chronic pain is becoming particularly evident from the high prevalence of accompanying SFN, the causes of which involve peripheral neural damage of various origin (40, 41), including compressive neuropathies (42, 43). Signs of peripheral neural damage after non-neural tissue injury have also been observed in animal experiments (44–46). The peripheral nerves are very susceptible to overload: nerve function can be disturbed by pressures as low as 30 millimeters of mercury (47), and experimental behavioral neuropathy along with ongoing neuronal activity develops within three weeks of repetitive overuse (48, 49). The most prevalent anatomic sites of chronic pain include the extremities and lower back (50–54), which are mobile and subject to overload areas, tightly interconnected with the spinal plexuses. Endoneurial edema, which can cause nerve conduction block, may quickly develop because of repetitive compression (55) at these sites. Notably, it has been hypothesized that proximal neural tissue irritation by repetitive activities may be an ectopic cause of repetitive strain injury (56, 57) (also known as nonspecific arm pain).
This report (for synopsis see the Supplementary File “Overview”) introduces a working hypothesis to explore whether sensitization maintained by peripheral proximal neural lesion (PNL), specifically by compressive PNL (cPNL), could explain all types of general chronic pain. The following sections involve hypothesis-driven reasoning, which is supported by the literature concerning sensitization, chronic pain, and neural lesion, with particular reference to PNL. Citation preference was given to the most recent review studies and original research that includes reviews and/or discussions of the pertinent issues. However, historical precedence of research was also considered if directly applicable to the central concept of the current article. The view that sensitization and chronic pain can be maintained without persistent peripheral noxious input (4, 20, 58) is a key point of controversy addressed by the current article.
Pre-hypothetical reasoning: ectopic nociceptive sensitization and definition of proximal neural lesion
General remarks on sensitization after nerve injury
Peripheral nerve lesion, including nerve compression, as a trigger of peripheral and consequent central sensitization [i.e., hyperexcitability and resultant hyperactivity of peripheral and central sensory pathway, respectively (5, 59)], has been documented by numerous studies (25, 27, 60–65). Nociceptive sensitization causes tissue hypersensitivity: hyperalgesia to noxious stimuli and painful sensations (allodynia) to non-noxious stimuli (16). Transient local tissue hypersensitivity is a common experience after traumas and in inflammatory conditions that involve skin. Clinically important central sensitization manifests as abnormal extraterritorial hypersensitivity of non-lesioned tissue and seems to be an inevitable result of peripheral sensitization [see, e.g., Satkeviciute and Dilley (66) and Torebjörk et al. (67)], which in turn can be induced by tissue injury, inflammation, and nerve injury (25, 68). Notably, nerve damage produces stronger sensitizing input than peripheral non-neural lesions: skin hypersensitivity lasts up to 24 h after capsaicin injection (69), ten days after incision (70), and over two months after nerve injury (65). Sensitizing effects of nerve injury obviously also apply to the nociceptive nervi nervorum, activity of which may account for peripheral nerve trunk pain (71–74). Clinically, sensitization-induced pain hypersensitivity can be measured as reduced pressure pain threshold (PPT) (75).
Symptomatic nociceptive sensitization can be induced by both distal neural lesions (63, 76) and proximal ones (63, 77) (as well as by non-neural insults). Why then should proximal neural injury be so important?
Ectopic ongoing (spontaneous) nociceptive neuronal activity and neuroinflammation
Hyperexcitability (i.e., sensitization) and consequent hyperactivity of sensory neurons enables continuing generation and discharge of neural impulses, which is necessary to be processed by the brain cortex as persistent pain. Increased excitability of sensory neurons is due to reduction of activation threshold of their ion channels, which may be caused by nerve injury, inflammation (arising from both neural and non-neural tissue), and by ion channel mutations (68, 78, 79). Although there are many mechanisms involved in sensitization, abnormal peripheral neuronal activity plays a key role as a triggering input (25, 26, 80). In this regard, nociceptive sensitization can electrophysiologically be understood as ongoing (spontaneous) activity and mechanical sensitivity of primary sensory fibers (81), which relates to neuronal hyperexcitability. The terms of neuronal ongoing activity and spontaneous activity have largely been used interchangeably (or rather as each other complementing terms): it is generally meant that ongoing generation and discharge of neuronal impulses occurs spontaneously. Ongoing activity can be induced with varying predominance in all types of sensory neurons (82, 83) and seems to be in part driven by neuroinflammatory mediator and cytokine mechanisms regardless of the triggering insult type (5, 59). Sensitization of nociceptive neurons involves complex molecular/ionic signaling cascades as well as activation of multiple receptors and ion channels. In-depth reviews of the nerve damage-related molecular mechanisms of nociceptor sensitization are available elsewhere (16, 68, 84, 85).
Neural impulses (action potentials) can be generated not only normally by stimulation of receptors in end organs (e.g., in the skin), but also ectopically by directly stimulating sensory nerve fibers (68, 85) as well as neuron cell bodies (86), which occurs in nerve injury and radiculopathy, respectively. After peripheral nerve damage, which causes loss of some dorsal root ganglion (DRG) neurons (62, 87–89), spontaneous ectopic activity arises in the surviving afferent neurons. Obviously, ectopic nerve trunk stimulation induces activity of the entire sensory neuron via normal orthodromic activation of the somata of the dorsal root ganglion neuron (DRGn) and antidromic (retrograde) nerve ending activation (81, 90).
It has been well established that hyperexcitability of peripheral sensory neurons is an ectopic cause of central sensitization and neuropathic pain (59, 60, 83, 91–94). This also applies to neurons of the trigeminal ganglion (60, 95) and, by analogy, possibly to sensory ganglions of other cranial nerves. Ectopic discharge (i.e., arising not from nerve ending receptors) can come both from the afferent fibers (25, 29, 86, 96–99) and from the somata of the DRGn (86). Recently, however, it has been discovered that spontaneous sensory activity in a chronic nerve constriction model is generated by a structure known as the axon initial segment that is located in the prebifurcation (stem) part of the sensory fibers within the DRG (100). Ectopic peripheral neural activity is explainable by nerve injury-induced DRG inflammation and consequent activation of glial cells (5, 59, 101, 102), which leads to sensory neuron hyperexcitability (5, 59, 102). Importantly, both ongoing ectopic activity and mechanical sensitivity of afferent fibers (both of the A- and C-type) can be induced not only with but also without structural nerve injury, specifically, by focal neuroinflammation (81, 103–106) that may presumably be produced by injury of paraneural tissues (82, 107). However, while axonal mechanical sensitivity can be induced by atraumatic non-inflammatory axonal transport disruption (73, 82, 107, 108), ongoing activity does not develop without focal neuritis (82, 107). In reality, though, disruption of axonal transport does not seem to occur without concomitant neuroinflammation, because signs of focal inflammatory reaction are evident within hours after nerve compression (47). Another explanation of nerve injury-induced sensitization of intact nociceptors is Wallerian myelinated fiber degeneration (98) and, specifically, its neuroinflammatory effects (109). Local peripheral neuroinflammation obviously results in DRG neuron body sensitization, because neuritis-induced ongoing nociceptive fiber activity can be recorded both distally and proximally to the DRG (81).
Sensitized primary sensory neurons may cause ectopic activity of central nervous system (CNS) neurons and thus induce central sensitization (5, 21, 25), possibly via neurogenic CNS neuroinflammation that may presumably be generated by peripheral sensory neuron activity (110). Central sensitization can also be caused by direct CNS damage, which may lead to secondary peripheral sensitization by retrograde excitation of DRG neurons (5, 111). CNS involvement can account for bilateral neuropathy symptoms, which may be due to DRGn hyperactivity-induced hyperexcitability of wide dynamic range neurons and activation of commissural neurons of the spinal cord (66), or, also supposedly, due to systemic neuroinflammation-mediated mechanisms (42, 66). Extraterritorial hypersensitivity may be shaped by neuron crosstalk not only in the CNS (112, 113) but also in the DRG (83). Notably, allodynia involves functional interplay between nociceptive and non-nociceptive pathways (2, 16).
Intra- and para-neural fibrosis
The above reasoning allows formulating the question of transition to chronic pain as follows: Why should DRGn hyperexcitability persist after the causative lesion has long healed?
A permanent consequence of healing of damaged tissue is fibrosis. The strength of fibrous tissue reaches its maximum at about three months after cutaneous injury (114), which, along with accompanying avascularity and acellularity of the scar, suggests that resolution of fibrosis that continues beyond three months becomes unlikely. Probably not coincidentally, this accords well with the time point given in the definition of chronic pain (1). As a part of reparative process following injury, fibrosis occurs both in the CNS (115, 116) and in the peripheral nervous system (116, 117). Signs of intraneural and extraneural fibrosis can be seen as early as three weeks following experimental repetitive overuse injury (48). Intralesional or paraneural postinjury fibrosis can presumably cause persistent focal nerve compression (56) and consequent neuroinflammation that leads to DRGn hyperexcitability. Intraganglional fibrosis likely follows direct DRG injury, e.g., due to intervertebral disc herniation. A small study found DRG fibrosis only in persistent post-herpetic neuralgia cases but no DRG scarring in pain free patients (118). Two studies have reported ectopic (i.e., remote to the injury site) fibrosis of the DRG after nerve compression (119, 120). Nerve injury-induced intraganglional inflammation (59, 101, 102), DRGn death, and resultant elimination of cell debris via inflammatory processes may account for ectopic fibrosis of the DRG.
Intermittent (dynamic) partial compressive nerve lesion as an essential cause of persistent sensitization and pain
There are certain controversies in the above discussion of ectopic neural fibrosis. Natural cell death (apoptosis) occurs in almost all tissues without notable residual fibrosis and consequent functional deficits. Another doubt is whether ectopic postinflammatory structural neural changes alone could be sufficient to maintain DRG and consequent CNS sensory neuron hyperexcitability (i.e., chronic pain), because fibrotic tissue is physiologically silent. The excitatory effects of paraneural or intraneural fibrosis may fade out after eventual death of the involved neurons and resolution of associated inflammation. Importantly, experimental research has shown that sensitization effects resolve without continuing focal neuroinflammatory input (82, 121) (which implies existence of occult peripheral neural damage in unexplained chronic pain). This can be supported by, e.g., a carpal tunnel syndrome (CTS) study that indicates that an extreme degree of distal nerve compression, in contrast to that of a moderate grade, does not result in peripheral tissue hypersensitivity (122). The latter may be explained by complete resolution of the distal focal inflammation and its ectopic effects due to significant loss of the neural tissue. Some pain-related neuropeptides have been found to be more expressed after experimental partial nerve injury than after complete neurotomy (123, 124). Also, inflammatory changes in the DRG and spinal cord tend to be more pronounced after nerve chronic constriction than after complete transection (125). Therefore, persistent intermittent (dynamic) partial proximal peripheral nerve damage (via, e.g., external compression by myofascial tension) with resultant continuing focal neuroinflammation and consequent hyperexcitability of the DRGn could be a reasonable explanation of the above controversy.
However, although established fibrosis is physiologically silent, scarred paraneural tissue can cause intermittent neural damage by restricting mobility of nerves (48). Via this mechanism, intraneural fibrosis of peripheral nerves may also result in dynamic intraneural compression of intact nerve fibers and their bundles. These structures are relatively mobile: loose connective tissue allows movement of nerve fibers and fascicles in respect to each other (121, 126), which may lead to their internal entrapment in epiperineural scars during body motion. Thus, motion of the healthy part of the nerve may result in dynamic compression even in seemingly static neural scarring. Although such a subtle mechanism of dynamic intraneural nerve compression may probably produce only slight disturbance of nociceptive neuron function, this may be sufficient to maintain central sensitization via long-term potentiation, which involves augmentation of action potential output by recruitment of subthreshold inputs (25).
Furthermore, nerve compression may intermittently be produced not only by neuromuscular but also by neurovascular mechanisms, e.g., as in trigeminal neuralgia (127). The neurovascular mechanism (presumably involving reflexive blood vessel dilation or spasm and transient edema) may be responsible for both intraneural and extraneural compression. These modes of intermittent neural compression may act concurrently. In naturally immobile areas like the skull, dynamic neural compression can probably only be produced by the neurovascular mechanism.
It is noteworthy, however, that sensory nerves are partly motile because they usually traverse dynamic structures or terminate in them (e.g., trigeminus branches glide because of facial muscle activity). Therefore, both para- and intra-neural fibrosis probably always result in intermittent nerve compression. Mobility of the sensory nerves may be responsible for intermittence of compression and resultant sensitization in numerous entrapment syndromes.
The double crush concept and sensitization
As a bifocal nerve lesion, the double crush syndrome (128, 129) may act as a strong trigger of sensitization. As noted above nerve compression physically impairs intra-axonal circulation and induces focal neuroinflammation (47). Disruption of axoplasmic transport in turn causes axonal mechanical sensitivity (73, 82, 107, 108), while neuroinflammation induces both axonal mechanical sensitivity and ongoing nociceptor activity (103, 104). In double crush syndrome, secondary nerve compression is facilitated by impairment of axoplasmic transport due to primary entrapment (128, 130). The resultant neurological deficit has been found to be greater than the sum of the deficits produced by each of the lesions individually (131, 132). Therefore, theoretically, simultaneous action of two or more nerve lesions, which would be asymptomatic if separately applied, may produce clinical nerve injury (133) by synergy of their effects and eventual activation of the DRGn (Supplementary Figure 1S). Similarly, compressive nerve lesions and coexistent systemic neuroinflammation may produce a cumulative effect, because neuroinflammation also disrupts axoplasmic transport (108, 134) (probably because of compression consequent to inflammatory neural edema). On the other hand, nerve compression induces focal neuroinflammation on its own (47, 135), which is essential in development of ongoing activity of nociceptive neurons (82, 107). Systemic inflammatory non-neural tissue conditions may further contribute to sensory neuron hyperexcitability (78), possibly, via induction of neuroinflammation (5). Thus, in patients with background systemic neuroinflammation, a subclinical focal peripheral nerve injury may lead to sensitization or its enhancement (Supplementary Figure 2S). Also, peripheral nerves may become hyperexcitable via other systemic factors (128), including genetic mechanisms, as has been shown with mutation of Na(v)1.7 sodium channels in SFN (136, 137) (however, there is no congenital pain).
Distal vs. proximal neural lesion
As discussed above, distal peripheral nerve lesions inevitably lead to proximal neural involvement in the form of the DRGn ectopic hyperexcitability and, possibly, DRG fibrosis. Furthermore, peripheral nerve injury results not only in loss of DRG neurons [proximal injuries being more detrimental (87, 88, 138)], but also in death of neurons in the dorsal horns of the spinal cord (62). Therefore, one can presume that distal nerve damage eventually generates a secondary (ectopic) PNL manifesting as structural and/or functional changes in the DRG and CNS. Logically, in such cases both distal nerve lesion and the ectopic PNL would coexist and shape the clinical picture of neuropathy.
The above elaboration on the ectopic consequences of distal peripheral nerve damage indirectly attests to the significance of direct proximal-level nerve injury. The closer the injury to the DRG, the more neurons are lost (87, 88, 138) and the greater overall excitation of DRG neurons is effected, because obviously more fibers are damaged in proximal nerve injuries than in distal ones. Similarly, Wallerian myelinated fiber degeneration, which induces spontaneous activity of the neighboring uninjured C-fibers (98, 109) and thus contributes to sensitization, involves a longer length of the nerve distal to the damage (and consequently can produce greater cumulative sensitizing effect) in proximal than in distal nerve lesions. Proximity of neural lesion to the DRG may also be important in that spontaneous neuron activity originates in the axon initial segment located in the DRG (83, 100).
Widespread nociceptive hypersensitivity, a sign of central sensitization, often accompanies carpal tunnel syndrome (71, 122, 139, 140). However, widespread hypersensitivity has been found absent in CTS patients without neck and arm pain (141), which implies that concomitant cPNL (a likely cause of the pain) may have been absent in those patients. This further implies that proximal-level neural damage may play a more significant role in the development of sensitization than distal peripheral nerve lesions alone. Widespread hypersensitivity in cervical radiculopathy (142), lumbar radiculopathy (143), whiplash injuries (142, 144), and plexopathies after breast cancer surgery and radiotherapy (145) also suggests the etiological importance of proximal compressive nerve injury. Of similar relevance may also be the occurrence of bilateral peripheral hypersensitivity in patients with non-neurogenic neck pain (146) and nonspecific arm pain (147).
A summarized definition of PNL, functional PNL, and compressive PNL
Summarizing the current section, and for the purpose of this article, PNL can be defined as a primary neural lesion in the proximity of spinal plexuses, sensory ganglia, and/or in the CNS, as well as a secondary (ectopic) ganglionic and/or CNS sensory neuron lesion induced by indirect injury. Also for the purpose of this paper, the functional PNL is understood as sensory neuron hyperexcitability and its consequent hyperactivity. Neuron hyperexcitability results from focal neuroinflammation, which in turn can be generated by proximal dynamic (intermittent) paraneural and intraneural compression, i.e., via peripheral cPNL. In this report, PNL primarily refers to peripheral lesions.
A working hypothesis of chronic pain maintained by dynamic compressive PNL
On the basis of the above considerations, it can be hypothesized that general chronic pain and pain in peripheral tissue conditions that are usually painless is due to nociceptive sensitization produced by hyperexcitability of sensory neurons (i.e., by functional PNL), which in turn is induced and/or maintained by persistent dynamic peripheral cPNL. For simplicity, this postulation will be elucidated below by initially addressing unusual local pain and then discussing chronic pain in general. This partition is only conditional because both pain categories refer to persistent pain driven by the same mechanism.
Compressive neuropathic etiology of pain in usually painless conditions
Unusual pain in space occupying lesions
Space occupying lesions generate intralesional pressure, exert certain pressure on the surrounding tissue, and are equally compressed by the latter, which in areas of nociceptive hypersensitivity can cause local pain because of pressure pain threshold drop and consequent mechanical allodynia and hyperalgesia (Figure 1) that commonly accompany sensitization (16, 75). Therefore, cPNL-induced and/or -maintained sensitization may be the cause of pain in usually painless surgical conditions, e.g., ganglion cyst (148), Dupuytren's fibromatosis (149), and scars (150). Obviously, such lesions can also be painful to palpation during clinical examination when background hypersensitivity coexists. Differently from general chronic pain (see below), persistent pain in local lesions may be caused not only by paraspinal cPNL but also by compression at any distal level proximal to the lesion. This concept of the hypothesis finds an indirect confirmation in a clinical study in which oral cancer patients with perineural invasion showed greater pain scores than patients without nerve involvement (151). The latter study also suggests that proximal peripheral nerve injury-induced sensitization can augment preexistent organic pain.
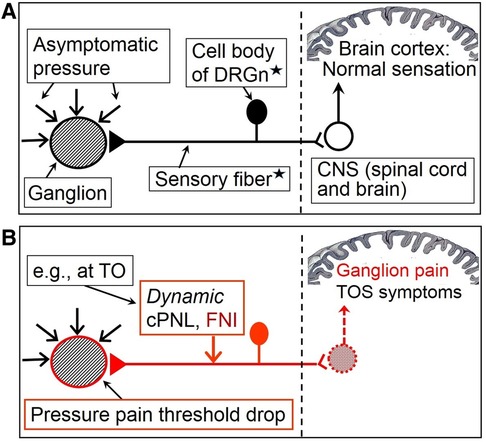
Figure 1. Hypothetical simplified mechanism of isolated pain in the case of a usually painless ganglion cyst. (A) Painless ganglion in the absence of sensitization. (B) Sensitization induced by compressive proximal neural lesion (cPNL), which is here clinically presented as TOS, induces focal neuroinflammation and consequent reduction of excitation threshold of afferent neurons. This results in PPT decrease of peripheral tissue and ganglion pain. This figure can serve as an explanation of neuroma pain as well; the ganglion then would represent a nerve stump including receptors of sensitized nervi nervorum (represented here by the DRGn). Filled in triangle = receptor of the sensory neuron; red arrow = force that is sufficient to produce symptomatic compression of otherwise unaffected nerve; black triangle with black horizontal line and black DRGn = peripheral nociceptive pathway in a normal state; red triangle with red horizontal line and red DRGn = hyperexcited peripheral nociceptive pathway; white circle = neuron of the dorsal horn of the spinal cord (arrow shows impulse direction); red pattern-filled circle = hyperactive dorsal horn neuron (arrow shows impulse direction). DRGn, dorsal root ganglion neuron; CNS, central nervous system; TO, thoracic outlet; cPNL, compressive proximal neural lesion; FNI, focal neuroinflammation; TOS, thoracic outlet syndrome. *Diagrammatically represents a mixture of primary sensory neurons (both of A- and C-type), functional interplay of which is involved in producing complex neural symptomatics. The complex involvement of central sensitization and neuron crosstalk, responsible for mechanical hyperalgesia and allodynia, is not shown. For more comprehensive depiction of nociceptive pathways and processes, see figures in the supplement file “Overview”.
Compressive PNL as an etiology of neuroma pain
There are no clear histological criteria to distinguish between painful and painless neuroma (152). Therefore, painful neuroma as a local condition with unexplained pain (153, 154) can be taken as a special case of application of the cPNL hypothesis. Logically, as a chronic pain condition, painful neuroma should have a source of nociceptive sensitization. Compressive forces naturally occur in and around neuroma itself because of the space-occupying nature of this lesion and fibrosis. Consequently, neuroma pain may be a result of cPNL-induced and/or -maintained sensitization of nociceptive nervi nervorum of the nerve stump (analogously as shown in Figure 1) and, importantly, a consequence of axonal mechanosensitivity that may also be induced and/or maintained by cPNL. This concept is based on the following neurophysiological logic, which is in a close context to the above prehypothetical reasoning.
A typical manifestation of symptomatic neuroma includes the Hoffmann-Tinel sign (155) that reflects nerve trunk mechanical sensitivity (107). Mechanical load of ectopically excited intact nociceptive nerve fibers is thought to produce neuropathy symptoms like radiating pain (82, 103, 105, 108), which is also characteristic of painful neuroma. In this regard, it is known that mechanical excitability of nociceptive axons can be induced by direct axonal injury [e.g., compression (156) or transection (97, 99)] and by focal neuroinflammation (73, 103, 105, 121), which is likely to accompany all nerve injuries (101). On the other hand, axonal mechanical sensitivity disappears with fading of the noxious input, as has been shown in an experimental model of non-inflammatory axonal transport disruption (82, 107). From a neuritis model, the same could be said about both mechanosensitivity (103, 105, 121) and spontaneous ongoing nociceptive fiber activity (82). [In these models, dissipation of noxious stimulus activity resulted in disappearance of cutaneous hypersensitivity as well (66, 105, 157, 158).] Increased abnormal afferent activity of transected peripheral nerve fibers continually decreases after the third postinjury week (97).
There is no active neuroinflammation or other pathophysiological activity within healed nerve stumps, which could contribute to ongoing sensory neuronal activity. Consequently, neuroma alone as a healed nerve injury cannot act as a permanent noxious stimulus and, therefore, cannot maintain clinically manifest sensitization accompanied by axonal mechanosensitivity (otherwise, all neuromas would probably be painful). Coexistence of neuroma and of cPNL-induced localized neuroinflammation (i.e., of a sensitizing input) may be crucial in the pathophysiology of neuroma pain. This logic practically explains why only some neuromas are painful: not all neuroma patients have significant background cPNL that induces and/or maintains nerve trunk hypersensitivity. Notably, nociceptive nervi nervorum can be sensitized by mechanical and chemical stress (74, 159), factors which may occur in nerve compression and consequent focal neuroinflammation.
Proximal neural lesion can be well expected (but may easily be overlooked, as discussed below) in painful neuroma that is often a result of a nerve injury sustained during trauma, because traumas can result in cPNL that clinically may present as, e.g., thoracic outlet syndrome (TOS), traction plexopathy with neural fibrosis, and cervical or lumbar radiculopathy. Likewise, coincidence of neuroma and pre-existing non-traumatic radiculopathy or TOS may also result in neuroma pain. Notably, painful neuromas have been mentioned to accompany the double crush syndrome (153). Also of relevance, the presence of concomitant cPNL may explain why surgical treatment of painful neuroma mostly produces only partial improvement (160, 161).
Intermittent nature of nerve compression may be more important in maintaining sensitization than paraneural or intraneural fibrosis as such. Therefore, indirect support of the above neuroma concept could also be the rarity of painful traumatic neuromas in areas innervated by naturally less mobile nerves, e.g., by the trigeminus (162) and other cranial nerves, because these nerves are less likely to be affected by overuse and intermittent cPNL. Probably, because of the same reason painful neuromas occur relatively rarely in the head (163). Of note, while head skin lacerations are common but rarely result in painful neuromas, these occur relatively often in extremities (154) that are extremely mobile. Furthermore, the rare symptomatic neuromas of the oral cavity occur predominantly in the distribution of the mandibular nerve (164), the most mobile division of the trigeminal nerve (see also the relevant discussion below).
Increased accumulation of ion channels has been observed in painful neuromas (165–167). This may also be due to effects of cPNL, because nerve compression is accompanied by expression of novel ion channels (135). Furthermore, migration of ion channels has been observed in an experimental nerve constriction injury (168).
Compressive neuropathic etiology of chronic pain in general: vicious cycle of chronic pain
Chronic pain may be caused by compensatory overuse-related cPNL. This can be judged, e.g., from the occurrence of painful musculoskeletal conditions in amputees (169–171). Initial posttraumatic pain may result in postural protection of the painful area and consequent compensatory musculoskeletal overuse. This may start a cycle of muscle weakening of the involved area and compensatory overuse of uninvolved muscles with resultant damage of the both muscle groups. Weakened muscles are naturally more susceptible to overuse injury. Muscle weakness and compensatory imbalance may cause myofascial tension, which can result in compression of proximal nerves in many systemic and posttraumatic conditions. This mechanism may explain high prevalence of chronic pain in patients with disabilities (172, 173). Notably, risk factors for fibromyalgia such as older age, high body mass index, and pre-existing medical disorders (174) could be associated with risk for muscle weakness. On the other hand, there may be reciprocity between loss of muscle strength and cPNL, because the latter is often accompanied by motor fiber damage. Wrong posture, ligament laxity, and muscle shortening may also contribute to development of cPNL, as has been implied by a biomechanical explanation of TOS pathogenesis (175). Another TOS study has suggested that abnormal postures due to initial nerve compression may lead to neck muscle tightness, imbalance, compensatory overuse, and consequent secondary worsening of the neural entrapment (133). Intermittent nerve compression via muscle spasm, which is common in proximal neural lesions, e.g., radiculopathy (176), may play an important role in this cycle. It is also of note that, as earlier suggested, nerve irritation by injured paraneural tissue may maintain focal neuroinflammation, which can serve as a persistent noxious input that causes nociceptive fiber hyperexcitability (82, 107).
Considering the above, chronic pain may be a consequence of a vicious cycle that involves continuing aggravation of PNL. This cycle may start with hyperexcitability of DRG neurons due to, e.g., peripheral tissue damage or radiculopathy, then proceed to consequent proximal reflexive muscle spasm and myofascial tightness, and eventually produce (or aggravate) focal neuroinflammatory injury of the proximal nerve trunk due to its compression at anatomically narrow spaces. In other words, functional PNL (i.e., DRGn hyperexcitability) may induce cPNL and may be maintained by the latter via focal neuroinflammation at the compression site. The reciprocity between DRGn hyperexcitability and cPNL can drive the vicious cycle even when the initial etiological trigger has healed (Figures 2, 3A). Importantly, the cycle may be perpetuated by reciprocity between cPNL-caused motor fiber dysfunction and muscle weakness that leads to muscle imbalance and compensatory overuse (Figure 3B). Primary CNS lesions may ignite the vicious cycle via possible antidromic activation of the DRGn (5, 111). Sensitization may play a role of a catalyst of this cycle, because sensitized nerve trunks become more susceptible to further damage (see discussion of the double crush concept and neuroma pain above). Thus, transition of acute to chronic pain may be due to the negative postural and compensatory overuse-related impact of acute pain (Figures 2, 3A). Because of the latter two factors and sensitization-catalyzed nerve vulnerability, a single-site cPNL may develop into a multiple-site cPNL, which could be another explanation of bilateral widespread chronic pain. It is essential that this cycle cannot function without persistent proximal neural lesion of intermittent character, which is discussed in the prehypothetical reasoning section above.
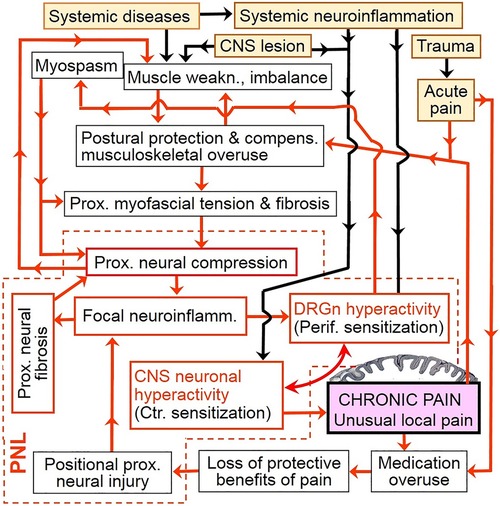
Figure 2. Chronic pain as a vicious cycle of persistent aggravation of proximal neural lesion. Only general etiological events (yellow filled-in boxes) are shown. Note the key role of focal neuroinflammation and DRGn hyperexcitability (i.e., functional PNL). Because of cPNL-maintained focal neural inflammation, ectopic firing from hyperactive DRG neurons can continue and evoke pain even after the initial etiological event has healed. This figure includes several cycles that can be discovered by following any arrow until the text box “Chronic pain” is reached; from there any arrow would eventually lead back to the latter textbox. (See also simplified versions of this illustration in Figure 3 and in Overview Figure 5ov in the Supplementary File “Overview”). Yellow filled-in text boxes = initial etiological events; redlined boxes = major drivers of the vicious cycle; violet filled-in box = processing of nociceptive impulses by the brain cortex; black flow arrow = triggering input which is not permanently involved in the vicious cycle; red flow one-headed arrow = input which serves as a link of the vicious cycle; bowed double-headed arrow = reciprocal enhancement but not an independent cycle. weakn., weakness; compens., compensatory; Prox., Proximal; neuroinflamm., neuroinflammation; DRGn, dorsal root ganglion neuron; Perif., Peripheral; CNS, central nervous system; Ctr., Central; PNL, proximal neural lesion.
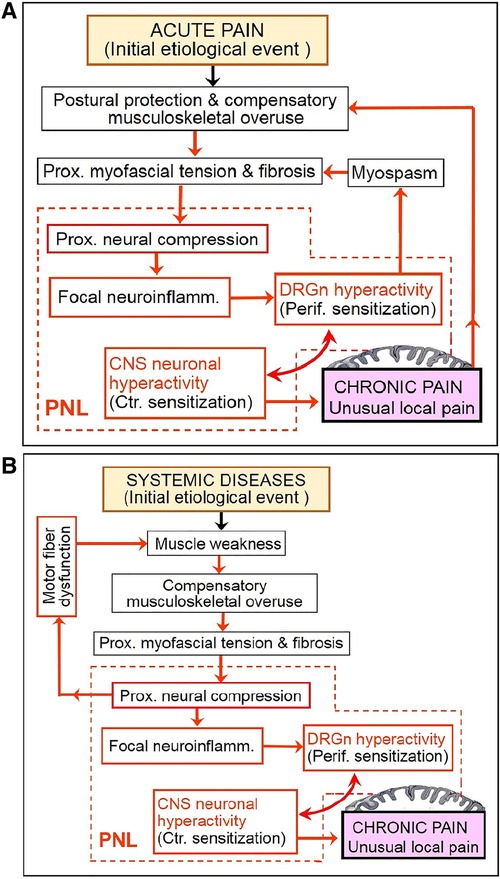
Figure 3. Partial deconstruction of the vicious cycle shown in Figure 2. (A) Two subcycles initiated by acute pain. (B) A subcycle initiated by systemic musculoskeletal impairment reveals the role of cPNL-induced motor fiber dysfunction (which is not shown in Figure 2). See Figure 2 legend for explanation of abbreviations and pictograms.
Disorders of the CNS can also cause muscle weakness, imbalance, compensatory overuse, and resultant cPNL. However, CNS lesions, as well as distal nerve lesions, cannot be maintained and aggravated by the above neuromuscular cyclic mechanism, hence again the importance of the intermittent compressive PNL.
Application of the cPNL hypothesis to chronic pain in general
Why does occurrence of chronic pain vary from patient to patient?
The vicious cycle of chronic pain suggests that occurrence of cPNL, and thus of sensitizing input, may vary as vary postural muscular consequences of traumas and diseases among patients. Susceptibility to pain may in fact represent a predisposition for cPNL. This may explain why chronic pain incidence increases with age (177, 178), i.e., with gradual decline of muscle strength. Another strong epidemiologic tendency is a high prevalence of chronic pain among women (177, 179), which may be due to the relatively high susceptibility of their musculoskeletal system to overload injury (180) and thus to consequent cPNL. Genetic, neurophysiological, and other factors may play an additional role in the development of cPNL.
Why does pain predicts pain?
Having in mind the possibility of background compressive PNL may help to explain unclear aggravation of pain in many clinical situations. Examples could be the reports that have shown that preoperative regional pain correlates with acute pain after repeat surgical deliveries in women with painful post-Caesarean scars (181) and predicts chronic pain in limb amputees (182) as well as in patients after breast cancer surgery (183, 184); pre-operative/pre-amputation pain is also a risk factor for phantom limb pain (185). In the context of the current hypothesis, one could speculate that surgical trauma may have worsened a coexistent cPNL (e.g., plexopathy or radiculopathy that are likely in the above clinical situations) and its sensitizing effects. It is also considerable that risk factors for chronic pain include trauma (186–190) and surgery (187, 190), in particular extensive surgeries (191) that may be associated with a higher risk of positional nerve injury (192). Furthermore, according to the double crush concept, background peripheral nerve damage makes the involved nerves more susceptible to further positional injury during surgery (for analogy see Supplementary Figure 2S). Noteworthy is also that trauma and surgery may share common pathogenesis of chronic pain and its risk factors, such as acute pain intensity, preinjury pain, and level of disability (190), which may be due to coincidental cPNL. Hypothetically, psychology-related predictors may be associated with occult cPNL as well. For example, familial pain may be associated with certain musculoskeletal predispositions for cPNL. On the other hand, cPNL-induced chronic pain may cause psychological disorders, the negative musculoskeletal-postural impact of which (193) may in turn aggravate cPNL.
Explanation of chronic pain in spinal cord injury (SCI)
While hyperexcitability of both central and peripheral nociceptive neurons has been shown in SCI (DRG neurons being excited antidromically) (111), it remains unaccounted for why not all spinal cord injuries result in chronic pain [see, e.g., Hunt et al. (194)] and, specifically, why some SCIs result in “only at-level” or “only below-level” chronic pain (195). Furthermore, why does below-level SCI pain tend to occur more often than above-level pain (196)?
These conundrums could be explained by variability in occurrence of symptomatic cPNL above, at, and below SCI level. Chronic pain above SCI level may be due to cPNL that is produced by compensatory overuse, as earlier discussed. At and below SCI level, cPNL probably occurs in all or most patients, since, because of denervation, SCI likely results in a secondary musculoligamentous instability of the spinal skeleton at and below the injury, which may facilitate positional cPNL in the affected paraspinal regions. In addition, traumatization of spinal nerves, due to involuntary muscle contractions that accompany SCI as well as due to postural changes performed without patient's voluntary muscle control, may contribute to cPNL at and below SCI level. However, cPNL may not always become symptomatic because of variability in severity of SCI as well as in degree of recovery of dorsal horn neuron function and spinothalamic transmission. For example, if dorsal horn neurons and their connections are relatively well functioning at the injury level but there is completely damaged spinothalamic transmission from the lower segments, then one is likely to observe “only at-level” chronic pain. Similar logic can also explain “only below-level” and unilateral SCI pain. Notably, sensory and motor preservation as a risk factor for progression of neuropathic pain in SCI patients (197) may be associated with a higher risk of cPNL due to greater potential for physical activity of those patients, because they may overload proximal nerves by performing tasks that are unnaturally overdemanding for intact muscles.
The unclear mechanism of lag in the onset of chronic pain after spinal cord injury (198, 199) is explainable by gradual development of cPNL via positional and compensatory overuse-related trauma. Time is also required for partial reestablishment of function of the damaged sensory pathways. Here again, healed lesions (in this case, SCI) alone cannot serve as a continuing noxious input, as discussed above. Obviously, postural traumatization of healed (fibrotic) spinal cord lesions may produce both direct triggering input (which still fits into the cPNL concept) and secondary (antidromic) hyperactivation of DRG neurons.
Explanation of trigeminal neuralgia, temporomandibular pain, and other orofacial pain
Because of relative shortness of the trigeminal nerve, its lesion at any level can be regarded as PNL. In trigeminal neuralgia, the trigeminal nerve is compressed (most often via a neurovascular mechanism) proximal to its ganglion (200), while in temporomandibular disorders the preganglionic part of the nerve is involved (201, 202). Trigeminal neuralgia is a rarity in comparison to temporomandibular chronic pain (203, 204). This may be due to relative immobility of the postganglionic (prepontine) trigeminal nerve compared to its mobile preganglionic division V3 (mandibular nerve). The latter anatomofunctional feature creates predisposition for motion-maintained intermittent cPNL (discussed in the prehypothetical reasoning section) of the mandibular nerve at its exit from the skull (which is very close to the trigeminal ganglion). Notably, lesions of a single peripheral division of the trigeminus may lead to hyperexcitability of uninvolved sensory neurons because of lesion-induced inflammation in the trigeminal ganglion (201) and possibly because of excitatory effects of Wallerian degeneration. Consequently, lesions of, e.g., mandibular nerve may lead to sensitization of uninjured V1 and V2 trigeminal divisions.
The enigmatic etiology of orofacial pain can be further elucidated by applying the double crush concept. Various combinations of subclinical and/or symptomatic lesions of the trigeminus within and outside the skull may result in a variety of painful symptoms (for an analogy, see Supplementary Figures 1S, 2S). For example, only neurovascular compromise of trigeminus function within the skull may manifest as the classical trigeminal neuralgia, while combination of subclinical proximal and distal involvement of the nerve may cumulate to diffuse orofacial pain.
Overlapping pain and cPNL
Development of overlapping pain may require many months after the onset of the first pain (205). This is in line with the introduced concept, because development of an established cPNL obviously takes time, especially when there is no initial proximal neural injury. Furthermore, the neuromuscular mechanism of chronic pain cycle suggests that occurrence of cPNL at one site may lead to another cPNL in a different body region. However, single cPNL may also cause spread of symptoms because of continuing aggravation of PNL (Figures 2 and 3) and resultant involvement of commissural neurons of the spinal cord.
For example, the overlap of the jaw/face, head, and neck pain (206) may be due to an entrapment lesion at the neck (Supplementary Figure 3S-A), which can be supported by a recent suggestion that occipital nerve compression causes unremitting head and neck pain (207). Relevantly, the beneficial effects of botulinum toxin in some cases of migraine (208) may be due to resolution of cranial muscle spasm (and thus due to nerve decompression) by botulinum. The overlap between CRPS and fibromyalgia pain (209) may also be caused by a single or multiple-site cPNL, because neuropathic features are characteristic of both CRPS (210) and fibromyalgia (36) (Supplementary Figure 3S-B).
Implications for chronic pain management
Why should compressive PNL go so often undiagnosed?
One may argue that cPNL has distinct diagnostic signs that can only be associated with the classical neuropathic pain. However, peripheral nerve lesions are often overlooked in practical management of general chronic pain. A compelling example is the CRPS type I that historically has not been associated with nerve injury. Despite the evidence of the latter misconception (30–33), the diagnosis of CRPS type I continues to be in clinical and academic use.
Recognition of nerve involvement at the paraspinal and plexus level may be especially problematic due to the complexity of differential diagnosis. The diversity of clinical symptoms and signs of chronic pain conditions may mask cPNL as an etiology. The intermittence of myospasm-induced cPNL may present a special difficulty. Mild focal intraneural fibrotic changes can result in permanent neurological deficits, but may be undetectable by either electrodiagnostic or imaging techniques. In fact, instrumental approaches may not reveal any specific pathologic features even when proximal neural damage is clinically obvious, e.g., as in neuralgic amyotrophy (211). A specific example of diagnostic difficulty of cPNL could be the neurogenic thoracic outlet syndrome (133, 212–215) that may present with extraterritorial symptoms, e.g., headaches (133, 212), as the only concern of the patient. Local pain may also be the only complaint revealed to the doctor in the cases of unusually painful peripheral conditions caused by cPNL. While previous traumatic events are known to be a risk factor for chronic pain (186–190), common uncomplicated traumas like falls may go unreported (216). Seemingly trivial injuries may initially result in subsymptomatic proximal paraneural tissue damage and lead to serious neurogenic deficits years later because of an additive effect of paraneural fibrosis, repeated traumas, overuse injury, and age-related neural lesion. On the other hand, local musculoskeletal consequences of extensive injuries may veil concomitant peripheral neural lesions (217).
In general, chronic pain conditions very often go unrecognized (218). One study has found that the diagnosis of chronic pain was overlooked by referring physicians in 66.7% out of 60 cases, which included peripheral nerve entrapment, radiculopathy, and TOS (219). Similar chronic pain studies revealed that 40% to 80% of patients were misdiagnosed (220). Misdiagnosis in chronic pain is a challenging, complex problem (221, 222) that may be influenced by etiological uncertainty of this disorder as well as by relevant diagnostic bias and inertia. For example, over 18% out of the surveyed 804 clinical practitioners would not examine the neck in pain conditions of the shoulder (223). Given the proximity of the latter two anatomic areas, one may expect even a higher proportion of overlooked examinations of proximal paraneural areas in patients with more distal pain. The diagnostic errors may also be due to incomplete anamnesis caused by miscommunication. It has been found that up to 59% of the patients with chronic pain think they had difficulty in conveying their symptoms (224), which is not surprising considering the clinical complexity of this condition. Gathering a cPNL-based anamnesis may improve this situation.
Referral of patients with unclear pain to the right specialist may be of decisive diagnostic importance. Unfortunately, chronic pain does not fit into any single discipline of medicine, which may be why proximal neural lesions and distal painful conditions are often regarded as areas of different specialties. For example, the thoracic outlet syndrome, which produces abundant signs and symptoms in the hand, may not belong to the scope of upper extremity surgeons, but, depending on the clinical institution, may be managed by neurosurgeons, thoracic surgeons, or vascular surgeons. In this regard, the specialized treatment of general chronic pain is even more problematic, because this condition is mostly managed by primary care providers (224, 225) who have little experience in peripheral nerve diagnostics and treatment. Obviously, primary care doctors are aware to consult a large spectrum of relevant specialists. However, should diagnostic errors occur at the specialist level, then they are likely to continue long after the patients return to the primary care. The cPNL hypothesis implies that peripheral nerve surgeon's involvement may be of key significance in chronic pain management. A review by Poppler and Mackinnon (153) seems to be one of very few sources to specify peripheral nerve surgeon in the pain team context. Neurosurgeons are often mentioned in terms of interdisciplinary management of chronic pain. However, neurosurgery is primarily understood as CNS surgery. Although encompassed by the specialty of neurosurgery, peripheral nerve surgery is a multidisciplinary field that requires anatomofunctionally related expertise in musculoskeletal and other peripheral tissue conditions (226, 227), which are within the scope of orthopedics and traumatology, plastic and reconstructive surgery, and hand surgery.
Chronic pain treatment in the light of the cPNL hypothesis
The mainstay of chronic pain treatment remains pharmacotherapy, which is of low long-term effectiveness. Poor results of chronic pain medication (228–230) may be due to undiagnosed occult proximal neural injuries. Surgery is a straightforward approach when PNL is caused by external nerve compression. Unfortunately, in longstanding entrapment lesions surgical decompression may not bring about a complete relief because of possible intraneural fibrosis and consequent permanent neurological deficits. Invasive approaches may even worsen the clinical picture if tissue hypersensitivity remains unresolved, which may occur in the cases of multifocal nerve damage. For example, when TOS and CTS coexist, release of the latter alone may not abolish the sensitization effects of TOS and could result in painful scars (Supplementary Figure 4S). [This may explain why outcomes of surgical treatment of double crush syndrome are poorer than those of isolated distal nerve compression (231, 232).] Thus, conservative treatment may be the major or the only option that can be offered to many chronic pain patients.
How could the concept of cPNL help to improve non-invasive techniques of chronic pain treatment? The varying effectiveness of non-invasive modalities (233–235) may be due to not knowing the underlying pain mechanisms. The cPNL hypothesis enables explaining why some standard approaches work similarly [see, e.g., the review by Ferro Moura Franco et al. (236)] in so many different chronic pain conditions. Determining the exact location of proximal neural lesion may help to individualize treatment.
Certain effectiveness of anti-inflammatory medication in chronic pain (237) may be in part due to abatement of local tissue inflammation and neuritis at the site of cPNL. Most conservative treatments also involve motion therapy, which is naturally associated with reduction of daily physical load. Therapeutic motion enhances tissue blood circulation and facilitates gliding of nerves, which may have positive effects on neuropathic pain (238). Mild exercises and load reduction may also relieve myofascial tension that causes nerve compression. The effectiveness of sympatholytic treatments in the sympathetically mediated chronic pain (239) can also be explained by the cPNL hypothesis. Sympatholytic approaches enhance intraneural and muscular circulation at critical sites because of vasodilatation, which can eventually result in myofascial release and improvement of neural function. The therapeutic effects of acupuncture (240) may be due to an analogous mechanism. Changing lifestyle and employing psychological modalities like cognitive behavioral therapy have the benefit of offloading nerves and encouraging patient's self-management.
Patient's active participation in treatment may influence outcomes. For example, lack of patient control during physiotherapy may result in neural overload and thus worsen proximal nerve damage. Similarly, overuse of chronic pain medication (241) may lead to loss of the protective benefits of pain, especially during sleep, and thus aggravate nerve lesion (Figure 2). Analgesia-facilitated deep sleep is associated not only with patient's comfort, but also with an increased risk of positional nerve injury, e.g., as in the case of the “Saturday night palsy” (242) or during general anesthetic surgeries (192).
Why does peripheral tissue surgery help?
Why then do local surgical approaches work in many cases of unusually painful isolated conditions? Surgery can bring about an improvement because of elimination of the sensitizing trigger by, e.g., freeing nerves from scars (243) or by insulating neuromas (160). However, most important may be postoperative rest, which can produce resolution of sensitization by offloading the involved proximal nerves. Furthermore, the postsurgical patients are likely to receive physiotherapy, which may have a positive effect on chronic pain due to myofascial release (244) and nerve mobilization (238). Such effects of postsurgical management may explain, e.g., why improvement after painful neuroma surgery is not clearly associated with employed surgical techniques (160, 161).
Testing the cPNL hypothesis
As noted in the introduction, a distinct feature of the proposed hypothesis is the presupposition of persistence of noxious input via peripheral proximal neural lesion. In other respects, this postulation does not challenge current theories of chronic pain. Neurophysiologically, the cPNL hypothesis may be compatible with most other explanations of persistent pain. Therefore, frameworks of designs of previous relevant chronic pain studies could be adapted to testing the current hypothesis.
Experimentally, the hypothesis could be tested by exploring DRG neuron damage in association with postural changes in non-neural injury-induced pain. For example, exploration of referred hypersensitivity in experimental knee osteoarthritis in animals (245) may reveal a possible etiological influence of posture-induced cPNL. (There may be a similar relationship between the negative postural impact of osteoarthritis (246–248) and referred hypersensitivity in human knee osteoarthritis (249).) Also of mention is an experimental model in which an extensive subcutaneous striping lesion of the rat hind paw resulted in Wallerian degeneration of myelinated fibers of the involved spinal nerve (46), which again may be due to cPNL induced by pain-related postural changes. The latter factor may also have influenced the results of investigations that are cited in support of autonomous central sensitization (4).
Unfortunately, there is no animal model to demonstrate transition of acute to chronic pain. Furthermore, experiments may not deal with chronic pain as such, because the available pain assays (250, 251) do not necessarily indicate presence of persistent pain. Therefore, definitive testing of the introduced hypothesis should primarily involve multiple clinical studies targeted at diagnosing or excluding cPNL in chronic pain patients. The above explanations of the clinical chronic pain-related conundrums could serve as a rough logical testing of the hypothesis and help to develop relevant research. Obviously, clinical disorders, in which the cPNL hypothesis could be tested, are not limited to those discussed here.
Conclusions
The current report offers a practical explanation of chronic pain. Differently from the previous concepts, this paper hypothesizes that there is a common neuropathic etiology of all types of general chronic pain, and that transition of acute to chronic pain may involve development (or aggravation) of peripheral compressive proximal neural lesion.
Described is a physioanatomical mechanism of persistent ectopic peripheral neural input that possibly maintains functional PNL in the form of DRGn hyperexcitability and its consequent hyperactivity (i.e., peripheral sensitization), which leads to hyperexcitability of central nociceptive pathway (i.e., central sensitization). Initial DRGn hyperexcitability, which can be induced by both peripheral non-neural and neural lesions, may produce an intermittent cPNL via reflexive myospasm-induced myofascial tension as well as via resultant muscle imbalance- and/or pain-provoked compensatory overuse. Consequently, DRGn hyperexcitability and cPNL may reciprocally maintain each other. Reciprocity may also exist between cPNL-caused motor fiber dysfunction and muscle weakness-related compensatory overuse. This vicious cycle seems to be catalyzed by sensitization (i.e., neuronal hyperexcitability) that increases nerve susceptibility to damage. Focal neuroinflammation induced by cPNL is a pathophysiological cause of DRGn hyperexcitability. Intra- and para-neural fibrosis can cause dynamic neural damage by restricting mobility of nerves. Intermittent nature of cPNL may be essential in chronic pain, because healed (fibrotic) lesions are physiologically silent to provide nociceptive input. Stimuli by distal lesions alone may be insufficient to maintain chronic pain and cannot be fueled by the vicious cycle. Pain in isolated conditions that are usually painless, as well as overly intense pain in inherently painful lesions, may be caused by mechanical hyperalgesia and allodynia due to compressive PNL-induced sensitization. Initially, cPNL may be asymptomatic until its aggravation or occurrence of additional focal or systemic nerve damage, i.e., development of a double crush-type injury. Not all patients may be equally susceptible to develop cPNL, because occurrence of cPNL may vary as vary patients' musculoskeletal fitness, exposure to overuse injury and past traumas, affliction by systemic diseases, and other factors. This article encourages diagnostic alertness to compressive PNL in chronic pain management and opens new perspectives for clinical and experimental research.
Data availability statement
The original contributions presented in the study are included in the article/Supplementary Material, further inquiries can be directed to the corresponding author.
Ethics Statement
Ethical review and approval was not required for this study in accordance with the local legislation and institutional requirements.
Author contributions
The author confirms being the sole contributor of this work and has approved it for publication.
Conflict of interest
The author declares that the research was conducted in the absence of any commercial or financial relationships that could be construed as a potential conflict of interest.
Publisher's note
All claims expressed in this article are solely those of the authors and do not necessarily represent those of their affiliated organizations, or those of the publisher, the editors and the reviewers. Any product that may be evaluated in this article, or claim that may be made by its manufacturer, is not guaranteed or endorsed by the publisher.
Supplementary material
The Supplementary Material for this article can be found online at: https://www.frontiersin.org/articles/10.3389/fpain.2023.1037376/full#supplementary-material.
References
1. Barke A, Korwisi B, Jakob R, Konstanjsek N, Rief W, Treede R-D. Classification of chronic pain for the international classification of diseases (ICD-11): results of the 2017 international world health organization field testing. Pain. (2022) 163:e310–318. doi: 10.1097/j.pain.000000000000228733863861
2. Woolf CJ. Central sensitization: implications for the diagnosis and treatment of pain. Pain. (2011) 152:S2–15. doi: 10.1016/j.pain.2010.09.03020961685
3. den Boer C, Dries L, Terluin B, van der Wouden JC, Blankenstein AH, van Wilgen CP, et al. Central sensitization in chronic pain and medically unexplained symptom research: a systematic review of definitions, operationalizations and measurement instruments. J Psychosom Res. (2019) 117:32–40. doi: 10.1016/j.jpsychores.2018.12.01030665594
4. Harte SE, Harris RE, Clauw DJ. The neurobiology of central sensitization. J Appl Biobehav Res. (2018) 23:e12137. doi: 10.1111/jabr.12137
5. Ji R-R, Nackley A, Huh Y, Terrando N, Maixner W. Neuroinflammation and central sensitization in chronic and widespread pain. Anesthesiology. (2018) 129:343–66. doi: 10.1097/ALN.000000000000213029462012
6. Vergne-Salle P, Bertin P. Chronic pain and neuroinflammation. Joint Bone Spine. (2021) 88:105222. doi: 10.1016/j.jbspin.2021.10522234022418
7. Ji R-R, Xu Z-Z, Gao Y-J. Emerging targets in neuroinflammation-driven chronic pain. Nat Rev Drug Discov. (2014) 13:533–48. doi: 10.1038/nrd433424948120
8. Apkarian AV, Baliki MN, Geha PY. Towards a theory of chronic pain. Prog Neurobiol. (2009) 87:81–97. doi: 10.1016/j.pneurobio.2008.09.01818952143
9. Chapman CR, Vierck CJ. The transition of acute postoperative pain to chronic pain: an integrative overview of research on mechanisms. J Pain. (2017) 18:359.e1–359.e38. doi: 10.1016/j.jpain.2016.11.00427908839
10. Price TJ, Ray PR. Recent advances toward understanding the mysteries of the acute to chronic pain transition. Curr Opin Physiol. (2019) 11:42–50. doi: 10.1016/j.cophys.2019.05.01532322780
11. Voscopoulos C, Lema M. When does acute pain become chronic? Br J Anaesth. (2010) 105(Suppl 1):i69–85. doi: 10.1093/bja/aeq32321148657
12. Puntillo F, Giglio M, Paladini A, Perchiazzi G, Viswanath O, Urits I, et al. Pathophysiology of musculoskeletal pain: a narrative review. Ther Adv Musculoskelet Dis. (2021) 13:1759720X21995067. doi: 10.1177/1759720X2199506733737965
13. Glare P, Aubrey KR, Myles PS. Transition from acute to chronic pain after surgery. Lancet. (2019) 393:1537–46. doi: 10.1016/S0140-6736(19)30352-630983589
14. McCarberg B, Peppin J. Pain pathways and nervous system plasticity: learning and memory in pain. Pain Med Malden Mass. (2019) 20:2421–37. doi: 10.1093/pm/pnz017
15. Damo E, Simonetti M. Axon guidance molecules and pain. Cells. (2022) 11:3143. doi: 10.3390/cells1119314336231105
16. Sandkühler J. Models and mechanisms of hyperalgesia and allodynia. Physiol Rev. (2009) 89:707–58. doi: 10.1152/physrev.00025.2008
17. Baloh RW. Why does pain persist in so many cases? In: Baloh RW, editor. Sciatica and chronic pain. Cham: Springer International Publishing (2019). p. 71–88. doi: 10.1007/978-3-319-93904-9_7
18. Yang S, Chang MC. Chronic pain: structural and functional changes in brain structures and associated negative affective states. Int J Mol Sci. (2019) 20:E3130. doi: 10.3390/ijms20133130
19. Yarns BC, Cassidy JT, Jimenez AM. At the intersection of anger, chronic pain, and the brain: a mini-review. Neurosci Biobehav Rev. (2022) 135:104558. doi: 10.1016/j.neubiorev.2022.10455835122780
20. Nijs J, Lahousse A, Kapreli E, Bilika P, Saraçoğlu İ, Malfliet A, et al. Nociplastic pain criteria or recognition of central sensitization? Pain phenotyping in the past, present and future. J Clin Med. (2021) 10:3203. doi: 10.3390/jcm1015320334361986
21. Baron R, Hans G, Dickenson AH. Peripheral input and its importance for central sensitization. Ann Neurol. (2013) 74:630–6. doi: 10.1002/ana.2401724018757
22. Devor M. Ectopic discharge in A-beta afferents as a source of neuropathic pain. Exp Brain Res. (2009) 196:115–28. doi: 10.1007/s00221-009-1724-619242687
23. Meacham K, Shepherd A, Mohapatra DP, Haroutounian S. Neuropathic pain: central vs. Peripheral mechanisms. Curr Pain Headache Rep. (2017) 21:28. doi: 10.1007/s11916-017-0629-528432601
24. Brazenor GA, Malham GM, Teddy PJ. Can central sensitization after injury persist as an autonomous pain generator? A comprehensive search for evidence. Pain Med Malden Mass. (2022) 23:1283–98. doi: 10.1093/pm/pnab319
25. Latremoliere A, Woolf CJ. Central sensitization: a generator of pain hypersensitivity by central neural plasticity. J Pain. (2009) 10:895–926. doi: 10.1016/j.jpain.2009.06.01219712899
26. Campbell JN, Meyer RA. Mechanisms of neuropathic pain. Neuron. (2006) 52:77–92. doi: 10.1016/j.neuron.2006.09.02117015228
27. Osborne NR, Anastakis DJ, Davis KD. Peripheral nerve injuries, pain, and neuroplasticity. J Hand Ther. (2018) 31:184–94. doi: 10.1016/j.jht.2018.01.01129706196
28. Cohen SP, Mao J. Neuropathic pain: mechanisms and their clinical implications. Br Med J. (2014) 348:f7656. doi: 10.1136/bmj.f7656
29. Finnerup NB, Kuner R, Jensen TS. Neuropathic pain: from mechanisms to treatment. Physiol Rev. (2021) 101:259–301. doi: 10.1152/physrev.00045.201932584191
30. Dellon L, Andonian E, Rosson GD. Lower extremity complex regional pain syndrome: long-term outcome after surgical treatment of peripheral pain generators. J Foot Ankle Surg. (2010) 49:33–6. doi: 10.1053/j.jfas.2009.08.00320123284
31. Dellon AL, Andonian E, Rosson GD. CRPS Of the upper or lower extremity: surgical treatment outcomes. J Brachial Plex Peripher Nerve Inj. (2009) 4:1. doi: 10.1186/1749-7221-4-119232118
32. Oaklander AL, Fields HL. Is reflex sympathetic dystrophy/complex regional pain syndrome type I a small-fiber neuropathy? Ann Neurol. (2009) 65:629–38. doi: 10.1002/ana.2169219557864
33. Borchers AT, Gershwin ME. The clinical relevance of complex regional pain syndrome type I: the emperor’s new clothes. Autoimmun Rev. (2017) 16:22–33. doi: 10.1016/j.autrev.2016.09.02427666818
34. Grayston R, Czanner G, Elhadd K, Goebel A, Frank B, Üçeyler N, et al. A systematic review and meta-analysis of the prevalence of small fiber pathology in fibromyalgia: implications for a new paradigm in fibromyalgia etiopathogenesis. Semin Arthritis Rheum. (2019) 48:933–40. doi: 10.1016/j.semarthrit.2018.08.00330314675
35. Littlejohn G. Neurogenic neuroinflammation in fibromyalgia and complex regional pain syndrome. Nat Rev Rheumatol. (2015) 11:639–48. doi: 10.1038/nrrheum.2015.10026241184
36. Martínez-Lavín M. Fibromyalgia and small fiber neuropathy: the plot thickens!. Clin Rheumatol. (2018) 37:3167–71. doi: 10.1007/s10067-018-4300-2
37. Ghasemi M, Rajabally YA. Small fiber neuropathy in unexpected clinical settings: a review. Muscle Nerve. (2020) 62:167–75. doi: 10.1002/mus.2680831943276
38. Chan ACY, Wilder-Smith EP. Small fiber neuropathy: getting bigger!. Muscle Nerve. (2016) 53:671–82. doi: 10.1002/mus.2508226872938
39. Sopacua M, Hoeijmakers JGJ, Merkies ISJ, Lauria G, Waxman SG, Faber CG. Small-fiber neuropathy: expanding the clinical pain universe. J Peripher Nerv Syst. (2019) 24:19–33. doi: 10.1111/jns.1229830569495
40. Sène D. Small fiber neuropathy: diagnosis, causes, and treatment. Joint Bone Spine. (2018) 85:553–9. doi: 10.1016/j.jbspin.2017.11.002
41. Strand N, Wie C, Peck J, Maita M, Singh N, Dumbroff J, et al. Small fiber neuropathy. Curr Pain Headache Rep. (2022) 26:429–38. doi: 10.1007/s11916-022-01044-835384587
42. Schmid AB, Hailey L, Tampin B. Entrapment neuropathies: challenging common beliefs with novel evidence. J Orthop Sports Phys Ther. (2018) 48:58–62. doi: 10.2519/jospt.2018.060329385943
43. Schmid AB, Bland JDP, Bhat MA, Bennett DLH. The relationship of nerve fibre pathology to sensory function in entrapment neuropathy. Brain J Neurol. (2014) 137:3186–99. doi: 10.1093/brain/awu288
44. Orita S, Ishikawa T, Miyagi M, Ochiai N, Inoue G, Eguchi Y, et al. Pain-related sensory innervation in monoiodoacetate-induced osteoarthritis in rat knees that gradually develops neuronal injury in addition to inflammatory pain. BMC Musculoskelet Disord. (2011) 12:134. doi: 10.1186/1471-2474-12-13421679434
45. Ferreira-Gomes J, Adães S, Sousa RM, Mendonça M, Castro-Lopes JM. Dose-dependent expression of neuronal injury markers during experimental osteoarthritis induced by monoiodoacetate in the rat. Mol Pain. (2012) 8:50. doi: 10.1186/1744-8069-8-5022769424
46. Kajita Y, Suetomi K, Okada T, Ikeuchi M, Arai Y-CP, Sato K, et al. Behavioral and neuropathological changes in animal models of chronic painful scar. J Orthop Sci. (2013) 18:1005–11. doi: 10.1007/s00776-013-0453-723963587
47. Rempel D, Dahlin L, Lundborg G. Pathophysiology of nerve compression syndromes: response of peripheral nerves to loading. J Bone Joint Surg Am. (1999) 81:1600–10. doi: 10.2106/00004623-199911000-0001310565653
48. Bove GM, Delany SP, Hobson L, Cruz GE, Harris MY, Amin M, et al. Manual therapy prevents onset of nociceptor activity, sensorimotor dysfunction, and neural fibrosis induced by a volitional repetitive task. Pain. (2019) 160:632–44. doi: 10.1097/j.pain.000000000000144330461558
49. Dilley A, Harris M, Barbe MF, Bove GM. Aberrant neuronal activity in a model of work-related upper limb pain and dysfunction. J Pain. (2022): 23:852–63. doi: 10.1016/j.jpain.2021.12.00434958943
50. Jakobsson U. The epidemiology of chronic pain in a general population: results of a survey in southern Sweden. Scand J Rheumatol. (2010) 39:421–9. doi: 10.3109/0300974100368561620476853
51. Bekkering GE, Bala MM, Reid K, Kellen E, Harker J, Riemsma R, et al. Epidemiology of chronic pain and its treatment in The Netherlands. Neth J Med. (2011) 69:141–53.21444943
52. Català E, Reig E, Artés M, Aliaga L, López JS, Segú JL. Prevalence of pain in the spanish population telephone survey in 5000 homes. Eur J Pain. (2002) 6:133–40. doi: 10.1053/eujp.2001.0310
53. Torrance N, Smith BH, Bennett MI, Lee AJ. The epidemiology of chronic pain of predominantly neuropathic origin. Results from a general population survey. J Pain. (2006) 7:281–9. doi: 10.1016/j.jpain.2005.11.00816618472
54. Yongjun Z, Tingjie Z, Xiaoqiu Y, Zhiying F, Feng Q, Guangke X, et al. A survey of chronic pain in China. Libyan J Med. (2020) 15:1730550. doi: 10.1080/19932820.2020.173055032066339
55. Yoshii Y, Ochiai N. Physiological response of peripheral nerve to repetitive compression: a rabbit experimental model. J Musculoskelet Res. (2021) 24:2150008. doi: 10.1142/S0218957721500081
56. Quintner J, Elvey R. The neurogenic hypothesis of RSI. [working paper]. Canberra, Australia: The Australian National University (1991).
57. Cohen ML, Arroyo JF, Champion GD, Browne CD. In search of the pathogenesis of refractory cervicobrachial pain syndrome. A deconstruction of the RSI phenomenon. Med J Aust. (1992) 156:432–6. doi: 10.5694/j.1326-5377.1992.tb139853.x1545753
58. van Wilgen CP, Keizer D. The sensitization model to explain how chronic pain exists without tissue damage. Pain Manag Nurs. (2012) 13:60–5. doi: 10.1016/j.pmn.2010.03.00122341140
59. Matsuda M, Huh Y, Ji R-R. Roles of inflammation, neurogenic inflammation, and neuroinflammation in pain. J Anesth. (2019) 33:131–9. doi: 10.1007/s00540-018-2579-430448975
60. Ji R-R, Berta T, Nedergaard M. Glia and pain: is chronic pain a gliopathy? Pain. (2013) 154(Suppl 1):S10–28. doi: 10.1016/j.pain.2013.06.02223792284
61. Noguchi K. Central sensitization following nerve injury: molecular mechanisms. In: Cervero F, Jensen T, editors. Handbook of clinical neurology. London: Elsevier (2006). p. 277–91. doi: 10.1016/S0072-9752(06)80024-2
62. Costigan M, Scholz J, Woolf CJ. Neuropathic pain: a maladaptive response of the nervous system to damage. Annu Rev Neurosci. (2009) 32:1–32. doi: 10.1146/annurev.neuro.051508.13553119400724
63. Gottrup H, Kristensen AD, Bach FW, Jensen TS. Aftersensations in experimental and clinical hypersensitivity. Pain. (2003) 103:57–64. doi: 10.1016/s0304-3959(02)00415-312749959
64. Jensen TS, Finnerup NB. Allodynia and hyperalgesia in neuropathic pain: clinical manifestations and mechanisms. Lancet Neurol. (2014) 13:924–35. doi: 10.1016/S1474-4422(14)70102-425142459
65. Bennett GJ, Xie Y-K. A peripheral mononeuropathy in rat that produces disorders of pain sensation like those seen in man. Pain. (1988) 33:87–107. doi: 10.1016/0304-3959(88)90209-62837713
66. Satkeviciute I, Dilley A. Neuritis and vinblastine-induced axonal transport disruption lead to signs of altered dorsal horn excitability. Mol Pain. (2018) 14:1744806918799581. doi: 10.1177/174480691879958130130994
67. Torebjörk HE, Lundberg LE, LaMotte RH. Central changes in processing of mechanoreceptive input in capsaicin-induced secondary hyperalgesia in humans. J Physiol. (1992) 448:765–80. doi: 10.1113/jphysiol.1992.sp019069
68. Woolf CJ, Ma Q. Nociceptors–noxious stimulus detectors. Neuron. (2007) 55:353–64. doi: 10.1016/j.neuron.2007.07.01617678850
69. Arendt-Nielsen L, Andersen OK. Capsaicin in human experimental pain models of skin, muscle and visceral sensitization. In: Malmberg AB, Bley KR, editors. Turning up the heat on pain: TRPV1 receptors in pain and inflammation. Progress in Inflammation Research. Basel: Birkhäuser-Verlag (2005). p. 117–44. doi: 10.1007/3-7643-7379-2_7
70. Brennan TJ. Pathophysiology of postoperative pain. Pain. (2011) 152:S33–40. doi: 10.1016/j.pain.2010.11.00521232860
71. Fernández-de-las-Peñas C, de la Llave-Rincón AI, Fernández-Carnero J, Cuadrado ML, Arendt-Nielsen L, Pareja JA. Bilateral widespread mechanical pain sensitivity in carpal tunnel syndrome: evidence of central processing in unilateral neuropathy. Brain J Neurol. (2009) 132:1472–9. doi: 10.1093/brain/awp050
72. Fernández-de-Las-Peñas C, Ortega-Santiago R, Ambite-Quesada S, Jiménez-Garcí A R, Arroyo-Morales M, Cleland JA. Specific mechanical pain hypersensitivity over peripheral nerve trunks in women with either unilateral epicondylalgia or carpal tunnel syndrome. J Orthop Sports Phys Ther. (2010) 40:751–60. doi: 10.2519/jospt.2010.3331
73. Bove G. Epi-perineurial anatomy, innervation, and axonal nociceptive mechanisms. J Bodyw Mov Ther. (2008) 12:185–90. doi: 10.1016/j.jbmt.2008.03.00419083672
74. Teixeira MJ, Almeida DB, Yeng LT. Concept of acute neuropathic pain. The role of nervi nervorum in the distinction between acute nociceptive and neuropathic pain. Rev Dor. (2016) 17:5–10. doi: 10.5935/1806-0013.20160038
75. Amiri M, Alavinia M, Singh M, Kumbhare D. Pressure pain threshold in patients with chronic pain: a systematic review and meta-analysis. Am J Phys Med Rehabil. (2021) 100:656–74. doi: 10.1097/PHM.000000000000160333002911
76. de-la-Llave-Rincón AI, Puentedura EJ, Fernández-de-las-Peñas C. New advances in the mechanisms and etiology of carpal tunnel syndrome. Discov Med. (2012) 13:343–8.
77. Mehta V, Snidvongs S, Ghai B, Langford R, Wodehouse T. Characterization of peripheral and central sensitization after dorsal root ganglion intervention in patients with unilateral lumbosacral radicular pain: a prospective pilot study. Br J Anaesth. (2017) 118:924–31. doi: 10.1093/bja/aex08928575334
78. Waxman SG, Zamponi GW. Regulating excitability of peripheral afferents: emerging ion channel targets. Nat Neurosci. (2014) 17:153–63. doi: 10.1038/nn.360224473263
79. Wei S-Q, Tao Z-Y, Xue Y, Cao D-Y. Peripheral sensitization. In: Turker H, Garcia Benavides L, Ramos Gallardo G, Méndez Del Villar M, editors. Peripheral nerve disorders and treatment. London: IntechOpen (2020). p. 131–56. doi: 10.5772/intechopen.90319
80. Issa CJ, Svientek SR, Dehdashtian A, Cederna PS, Kemp SWP. Pathophysiological and neuroplastic changes in postamputation and neuropathic pain: review of the literature. Plast Reconstr Surg Glob Open. (2022) 10:e4549. doi: 10.1097/GOX.000000000000454936187278
81. Bove GM, Dilley A. The conundrum of sensitization when recording from nociceptors. J Neurosci Methods. (2010) 188:213–8. doi: 10.1016/j.jneumeth.2010.02.010
82. Satkeviciute I, Goodwin G, Bove GM, Dilley A. Time course of ongoing activity during neuritis and following axonal transport disruption. J Neurophysiol. (2018) 119:1993–2000. doi: 10.1152/jn.00882.201729465329
83. Nascimento AI. Sensory neurons have an axon initial segment that initiates spontaneous activity in neuropathic pain [thesis]. Porto, Portugal: University of Porto (2022). Available at: https://repositorio-aberto.up.pt/bitstream/10216/141057/2/556882.pdf
84. Kocot-Kępska M, Zajączkowska R, Mika J, Wordliczek J, Dobrogowski J, Przeklasa-Muszyńska A. Peripheral mechanisms of neuropathic pain-the role of neuronal and non-neuronal interactions and their implications for topical treatment of neuropathic pain. Pharm Basel. (2021) 14:77. doi: 10.3390/ph14020077
85. Gold MS. Peripheral pain mechanisms and nociceptor sensitization. In: Ballantyne JC, Fishman SM, Rathmell JP, editors. Bonica’s management of pain. Philadelphia: Lippincott Williams & Wilkins (LWW) (2018). p. 24–37.
86. Ma C, LaMotte RH. Multiple sites for generation of ectopic spontaneous activity in neurons of the chronically compressed dorsal root ganglion. J Neurosci Off J Soc Neurosci. (2007) 27:14059–68. doi: 10.1523/JNEUROSCI.3699-07.2007
87. Hart AM, Terenghi G, Wiberg M. Neuronal death after peripheral nerve injury and experimental strategies for neuroprotection. Neurol Res. (2008) 30:999–1011. doi: 10.1179/174313208X36247919079974
88. Terenghi G, Hart A, Wiberg M. The nerve injury and the dying neurons: diagnosis and prevention. J Hand Surg Eur Vol. (2011) 36:730–4. doi: 10.1177/175319341142220222058229
89. McKay Hart A, Brannstrom T, Wiberg M, Terenghi G. Primary sensory neurons and satellite cells after peripheral axotomy in the adult rat: timecourse of cell death and elimination. Exp Brain Res. (2002) 142:308–18. doi: 10.1007/s00221-001-0929-011819038
90. Willis WD. Dorsal root potentials and dorsal root reflexes: a double-edged sword. Exp Brain Res. (1999) 124:395–421. doi: 10.1007/s00221005063710090653
91. Esposito MF, Malayil R, Hanes M, Deer T. Unique characteristics of the dorsal root ganglion as a target for neuromodulation. Pain Med. (2019) 20:S23–30. doi: 10.1093/pm/pnz01231152179
92. Krames ES. The role of the dorsal root ganglion in the development of neuropathic pain. Pain Med. (2014) 15:1669–85. doi: 10.1111/pme.1241324641192
93. Chung JM, Chung K. Importance of hyperexcitability of DRG neurons in neuropathic pain. Pain Pract Off J World Inst Pain. (2002) 2:87–97. doi: 10.1046/j.1533-2500.2002.02011.x
94. Devor M. Rethinking the causes of pain in herpes zoster and postherpetic neuralgia: the ectopic pacemaker hypothesis. Pain Rep. (2018) 3:e702. doi: 10.1097/PR9.000000000000070230706041
95. Messlinger K, Balcziak LK, Russo AF. Cross-talk signaling in the trigeminal ganglion: role of neuropeptides and other mediators. J Neural Transm Vienna Austria. (2020) 127:431–44. doi: 10.1007/s00702-020-02161-7
96. Wall PD, Gutnick M. Ongoing activity in peripheral nerves: the physiology and pharmacology of impulses originating from a neuroma. Exp Neurol. (1974) 43:580–93. doi: 10.1016/0014-4886(74)90197-64827166
97. Scadding JW. Development of ongoing activity, mechanosensitivity, and Adrenaline sensitivity in severed peripheral nerve axons. Exp Neurol. (1981) 73:345–64. doi: 10.1016/0014-4886(81)90271-57262242
98. Wu G, Ringkamp M, Murinson BB, Pogatzki EM, Hartke TV, Weerahandi HM, et al. Degeneration of myelinated efferent fibers induces spontaneous activity in uninjured C-fiber afferents. J Neurosci. (2002) 22:7746–53. doi: 10.1523/JNEUROSCI.22-17-07746.200212196598
99. Michaelis M, Blenk KH, Jänig W, Vogel C. Development of spontaneous activity and mechanosensitivity in axotomized afferent nerve fibers during the first hours after nerve transection in rats. J Neurophysiol. (1995) 74:1020–7. doi: 10.1152/jn.1995.74.3.10207500128
100. Nascimento AI, Da Silva TF, Fernandes EC, Luz LL, Mar FM, Safronov BV, et al. Sensory neurons have an axon initial segment that initiates spontaneous activity in neuropathic pain. Brain J Neurol. (2022) 145:1632–40. doi: 10.1093/brain/awac078
101. Estera LA, Walsh SP, Headen JA, Williamson RE, Kalinski AL. Neuroinflammation: breaking barriers and bridging gaps. Neurosci Res. (2021) S0168-0102(21)00222–4. doi: 10.1016/j.neures.2021.11.00134748905
102. Noh M, Mikler B, Joy T, Smith PA. Time course of inflammation in dorsal root ganglia correlates with differential reversibility of mechanical allodynia. Neuroscience. (2020) 428:199–216. doi: 10.1016/j.neuroscience.2019.12.04031918012
103. Dilley A, Bove GM. Resolution of inflammation-induced axonal mechanical sensitivity and conduction slowing in C-fiber nociceptors. J Pain. (2008) 9:185–92. doi: 10.1016/j.jpain.2007.10.01218309534
104. Eliav E, Benoliel R, Tal M. Inflammation with no axonal damage of the rat saphenous nerve trunk induces ectopic discharge and mechanosensitivity in myelinated axons. Neurosci Lett. (2001) 311:49–52. doi: 10.1016/s0304-3940(01)02143-711585565
105. Bove GM, Ransil BJ, Lin H-C, Leem J-G. Inflammation induces ectopic mechanical sensitivity in axons of nociceptors innervating deep tissues. J Neurophysiol. (2003) 90:1949–55. doi: 10.1152/jn.00175.200312724363
106. Dilley A, Lynn B, Pang SJ. Pressure and stretch mechanosensitivity of peripheral nerve fibres following local inflammation of the nerve trunk. Pain. (2005) 117:462–72. doi: 10.1016/j.pain.2005.08.01816154692
107. Goodwin G. The role of axonal transport disruption in the development of axonal mechanical sensitivity in intact C-fibre neurons [PhD thesis]. University of Brighton (2018). Available at: https://cris.brighton.ac.uk/ws/portalfiles/portal/5937865/GG_final_thesis.pdf
108. Dilley A, Bove GM. Disruption of axoplasmic transport induces mechanical sensitivity in intact rat C-fibre nociceptor axons. J Physiol. (2008) 586:593–604. doi: 10.1113/jphysiol.2007.14410518006580
109. Dubový P. Wallerian degeneration and peripheral nerve conditions for both axonal regeneration and neuropathic pain induction. Ann Anat. (2011) 193:267–75. doi: 10.1016/j.aanat.2011.02.011
110. Xanthos DN, Sandkühler J. Neurogenic neuroinflammation: inflammatory CNS reactions in response to neuronal activity. Nat Rev Neurosci. (2014) 15:43–53. doi: 10.1038/nrn361724281245
111. Kang J, Cho SS, Kim HY, Lee BH, Cho HJ, Gwak YS. Regional hyperexcitability and chronic neuropathic pain following spinal cord injury. Cell Mol Neurobiol. (2020) 40:861–78. doi: 10.1007/s10571-020-00785-731955281
112. Koch SC, Acton D, Goulding M. Spinal circuits for touch, pain, and itch. Annu Rev Physiol. (2018) 80:189–217. doi: 10.1146/annurev-physiol-022516-03430328961064
113. Zhang T, Zhang M, Cui S, Liang W, Jia Z, Guo F, et al. The core of maintaining neuropathic pain: crosstalk between glial cells and neurons (neural cell crosstalk at spinal cord). Brain Behav. (2023):e2868. doi: 10.1002/brb3.286836602945
114. Stroncek JD, Reichert WM. Overview of wound healing in different tissue types. In: Reichert WM, editors. Indwelling neural implants: strategies for contending with the in vivo environment. Frontiers in Neuroengineering. Boca Raton, FL: CRC Press/Taylor & Francis (2008). p. 3–38.
115. Fehlberg CR, Lee JK. Fibrosis in the central nervous system: from the meninges to the vasculature. Cell Tissue Res. (2022) 387:351–60. doi: 10.1007/s00441-021-03491-y34189605
116. Ghosh N, Kalbermatten D, Madduri S, Guzman R. Fibrosis and regulation of nerve regeneration in the peripheral and central nervous systems. CNS Neurol Disord Drug Targets. (2020) 19:560–71. doi: 10.2174/187152731966620072622255832713338
117. Lee SK, Wolfe SW. Peripheral nerve injury and repair. J Am Acad Orthop Surg. (2000) 8:243–52. doi: 10.5435/00124635-200007000-0000510951113
118. Watson CPN, Deck JH, Morshead C, Van der Kooy D, Evans RJ. Post-herpetic neuralgia: further post-mortem studies of cases with and without pain. Pain. (1991) 44:105–17. doi: 10.1016/0304-3959(91)90124-G1711192
119. Li Q, Chen J, Chen Y, Cong X, Chen Z. Chronic sciatic nerve compression induces fibrosis in dorsal root ganglia. Mol Med Rep. (2016) 13:2393–400. doi: 10.3892/mmr.2016.481026820076
120. Li Q, Liang J, Wang D, Shang Z. Fibrotic changes in rat dorsal root ganglion following chronic sciatic nerve compression. Chin J Tissue Eng Res. (2020) 24:4686–91. doi: 10.3969/j.issn.2095-4344.2741
121. Greening J, Dilley A. Peripheral mechanisms of chronic upper limb pain. In: Fernandez de las Penas C, Cleland JA, Huijbregts PA, editors. Neck and arm pain syndromes: Evidence-informed screening, diagnosis and management. Edinburgh: Churchill Livingstone (2011). p. 476–95. doi: 10.1016/B978-0-7020-3528-9.00037-6
122. Bernardino S, Hal M, Rs M, Co H, Fhm S, Bml A, et al. Pain threshold by pressure algometry in the carpal tunnel syndrome. Med Res Arch. (2019) 7. doi: 10.18103/mra.v7i11.2009
123. Ma W, Bisby MA. Differential expression of galanin immunoreactivities in the primary sensory neurons following partial and complete sciatic nerve injuries. Neuroscience. (1997) 79:1183–95. doi: 10.1016/s0306-4522(97)00088-29219977
124. Ma W, Bisby MA. Increase of calcitonin gene-related peptide immunoreactivity in the axonal fibers of the gracile nuclei of adult and aged rats after complete and partial sciatic nerve injuries. Exp Neurol. (1998) 152:137–49. doi: 10.1006/exnr.1998.68279682021
125. Hu P, Bembrick AL, Keay KA, McLachlan EM. Immune cell involvement in dorsal root ganglia and spinal cord after chronic constriction or transection of the rat sciatic nerve. Brain Behav Immun. (2007) 21:599–616. doi: 10.1016/j.bbi.2006.10.01317187959
126. Millesi H, Hausner T, Schmidhammer R, Trattnig S, Tschabitscher M. Anatomical structures to provide passive motility of peripheral nerve trunks and fascicles. Acta Neurochir Suppl. (2007) 100:133–5. doi: 10.1007/978-3-211-72958-8_2817985562
127. Jones MR, Urits I, Ehrhardt KP, Cefalu JN, Kendrick JB, Park DJ, et al. A comprehensive review of trigeminal neuralgia. Curr Pain Headache Rep. (2019) 23:74. doi: 10.1007/s11916-019-0810-031388843
128. Cohen BH, Gaspar MP, Daniels AH, Akelman E, Kane PM. Multifocal neuropathy: expanding the scope of double crush syndrome. J Hand Surg. (2016) 41:1171–5. doi: 10.1016/j.jhsa.2016.09.009
129. Upton AR, McComas AJ. The double crush in nerve entrapment syndromes. Lancet Lond Engl. (1973) 2:359–62. doi: 10.1016/s0140-6736(73)93196-6
130. Nemoto K. Experimental study on the vulnerability of the peripheral nerve. Nippon Seikeigeka Gakkai Zasshi. (1983) 57:1773–86.6676393
131. Nemoto K, Matsumoto N, Tazaki K, Horiuchi Y, Uchinishi K, Mori Y. An experimental study on the “double crush” hypothesis. J Hand Surg. (1987) 12:552–9. doi: 10.1016/s0363-5023(87)80207-1
132. Dellon AL, Mackinnon SE. Chronic nerve compression model for the double crush hypothesis. Ann Plast Surg. (1991) 26:259–64. doi: 10.1097/00000637-199103000-000082029136
133. Novak CB, Collins ED, Mackinnon SE. Outcome following conservative management of thoracic outlet syndrome. J Hand Surg. (1995) 20:542–8. doi: 10.1016/S0363-5023(05)80264-3
134. Armstrong BD, Hu Z, Abad C, Yamamoto M, Rodriguez WI, Cheng J, et al. Induction of neuropeptide gene expression and blockade of retrograde transport in facial motor neurons following local peripheral nerve inflammation in severe combined immunodeficiency and BALB/C mice. Neuroscience. (2004) 129:93–9. doi: 10.1016/j.neuroscience.2004.06.08515489032
135. Schmid AB, Fundaun J, Tampin B. Entrapment neuropathies: a contemporary approach to pathophysiology, clinical assessment, and management. Pain Rep. (2020) 5:e829. doi: 10.1097/PR9.000000000000082932766466
136. Hoeijmakers JGJ, Merkies ISJ, Gerrits MM, Waxman SG, Faber CG. Genetic aspects of sodium channelopathy in small fiber neuropathy. Clin Genet. (2012) 82:351–8. doi: 10.1111/j.1399-0004.2012.01937.x22803682
137. Waxman SG. Chasing men on fire: the story of the search for a pain gene. Cambridge, MA, USA: MIT Press (2018).
138. Ygge J. Neuronal loss in lumbar dorsal root ganglia after proximal compared to distal sciatic nerve resection: a quantitative study in the rat. Brain Res. (1989) 478:193–5. doi: 10.1016/0006-8993(89)91497-22924117
139. Fernández-de-Las-Peñas C, Madeleine P, Martínez-Perez A, Arendt-Nielsen L, Jiménez-García R, Pareja JA. Pressure pain sensitivity topographical maps reveal bilateral hyperalgesia of the hands in patients with unilateral carpal tunnel syndrome. Arthritis Care Res. (2010) 62:1055–64. doi: 10.1002/acr.20189
140. de la Llave-Rincón AI, Fernández-de-las-Peñas C, Laguarta-Val S, Alonso-Blanco C, Martínez-Perez A, Arendt-Nielsen L, et al. Increased pain sensitivity is not associated with electrodiagnostic findings in women with carpal tunnel syndrome. Clin J Pain. (2011) 27:747–54. doi: 10.1097/AJP.0b013e31821c29d3
141. Schmid AB, Soon BTC, Wasner G, Coppieters MW. Can widespread hypersensitivity in carpal tunnel syndrome be substantiated if neck and arm pain are absent? Eur J Pain. (2012) 16:217–28. doi: 10.1016/j.ejpain.2011.06.00322323374
142. Chien A, Eliav E, Sterling M. Whiplash (grade II) and cervical radiculopathy share a similar sensory presentation: an investigation using quantitative sensory testing. Clin J Pain. (2008) 24:595–603. doi: 10.1097/AJP.0b013e31816ed4fc18716498
143. Jensen OK, Nielsen CV, Stengaard-Pedersen K. Diffuse central sensitization in low back patients. Medicine (Baltimore). (2020) 99:e22198. doi: 10.1097/MD.000000000002219832957350
144. Chien A, Eliav E, Sterling M. Hypoaesthesia occurs with sensory hypersensitivity in chronic whiplash–further evidence of a neuropathic condition. Man Ther. (2009) 14:138–46. doi: 10.1016/j.math.2007.12.00418294899
145. Caro-Morán E, Díaz-Rodríguez L, Cantarero-Villanueva I, Galiano-Castillo N, Arroyo-Morales M, Fernández-Lao C. Nerve pressure pain hypersensitivity and upper limb mechanosensitivity in breast cancer survivors: a case-control study. Pain Med. (2014) 15:1715–23. doi: 10.1111/pme.12567
146. Rojas VEA, Pluma AF, Pecos-Martín D, Achalandabaso-Ochoa A, Fernández-Matías R, Martinez-Merinero P, et al. Relationship between neuromuscular mechanosensitivity and chronic neck pain in guitarists: a cross-sectional study. Int J Environ Res Public Health. (2021) 18:2673. doi: 10.3390/ijerph1805267333800943
147. Moloney N, Hall T, Doody C. Sensory hyperalgesia is characteristic of nonspecific arm pain: a comparison with cervical radiculopathy and pain-free controls. Clin J Pain. (2013) 29:948–56. doi: 10.1097/AJP.0b013e31827c7ae823370077
148. Gude W, Morelli V. Ganglion cysts of the wrist: pathophysiology, clinical picture, and management. Curr Rev Musculoskelet Med. (2008) 1:205–11. doi: 10.1007/s12178-008-9033-419468907
149. Vandecasteele L, Degreef I. Pain in dupuytren’s disease. Acta Orthop Belg. (2020) 86:555–62.33581042
150. Bijlard E, Uiterwaal L, Kouwenberg CAE, Mureau MAM, Hovius SER, Huygen FJPM. A systematic review on the prevalence, etiology, and pathophysiology of intrinsic pain in dermal scar tissue. Pain Physician. (2017) 20:1–13. doi: 10.36076/ppj.2017.2.1328158149
151. Salvo E, Campana WM, Scheff NN, Nguyen TH, Jeong S-H, Wall I, et al. Peripheral nerve injury and sensitization underlie pain associated with oral cancer perineural invasion. Pain. (2020) 161:2592–602. doi: 10.1097/j.pain.000000000000198632658150
152. Oliveira KMC, Pindur L, Han Z, Bhavsar MB, Barker JH, Leppik L. Time course of traumatic neuroma development. PLoS One. (2018) 13:e0200548. doi: 10.1371/journal.pone.020054830011306
153. Poppler LH, Mackinnon SE. The role of the peripheral nerve surgeon in the treatment of pain. Neurotherapeutics. (2019) 16:9–25. doi: 10.1007/s13311-018-00695-z30542905
154. Lu C, Sun X, Wang C, Wang Y, Peng J. Mechanisms and treatment of painful neuromas. Rev Neurosci. (2018) 29:557–66. doi: 10.1515/revneuro-2017-007729306933
155. Arnold DMJ, Wilkens SC, Coert JH, Chen NC, Ducic I, Eberlin KR. Diagnostic criteria for symptomatic neuroma. Ann Plast Surg. (2019) 82:420–7. doi: 10.1097/SAP.000000000000179630855369
156. Howe JF, Loeser JD, Calvin WH. Mechanosensitivity of dorsal root ganglia and chronically injured axons: a physiological basis for the radicular pain of nerve root compression. Pain. (1977) 3:25–41. doi: 10.1016/0304-3959(77)90033-1195255
157. Dilley A, Richards N, Pulman KG, Bove GM. Disruption of fast axonal transport in the rat induces behavioral changes consistent with neuropathic pain. J Pain. (2013) 14:1437–49. doi: 10.1016/j.jpain.2013.07.00524035352
158. Richards N, Dilley A. Contribution of hyperpolarization-activated channels to heat hypersensitivity and ongoing activity in the neuritis model. Neuroscience. (2015) 284:87–98. doi: 10.1016/j.neuroscience.2014.08.05825290015
159. Ellis RF. Neurodynamic evaluation of the sciatic nerve during neural mobilisation: ultrasound imaging assessment of sciatic nerve movement and the clinical implications for treatment [PhD thesis]. Auckland University of Technology (2011).
160. Poppler LH, Parikh RP, Bichanich MJ, Rebehn K, Bettlach CR, Mackinnon SE, et al. Surgical interventions for the treatment of painful neuroma: a comparative meta-analysis. Pain. (2018) 159:214–23. doi: 10.1097/j.pain.000000000000110129189515
161. Decrouy-Duruz V, Christen T, Raffoul W. Evaluation of surgical treatment for neuropathic pain from neuroma in patients with injured peripheral nerves. J Neurosurg. (2018) 128:1235–40. doi: 10.3171/2017.1.JNS16177828621630
162. Thomas DC, Mallareddy SD, Okeson JP, Thankachan J, Pitchumani PK, Pichammal RC. Trigeminal traumatic neuroma: a comprehensive review of the literature based on a rare case. Curr Pain Headache Rep. (2022) 26:219–33. doi: 10.1007/s11916-022-01018-w35119601
163. Lee EJ, Calcaterra TC, Zuckerbraun L. Traumatic neuromas of the head and neck. Ear Nose Throat J. (1998) 77:670–4; 676. doi: 10.1177/0145561398077008169745184
164. Guerrieri P, Oliveira A, Arosio F, Murgia MS, Viganò L, Casu C. Vascular-nerve complications following oral surgery: hematoma and neuroma: a literature review. Int J Appl Dent Sci. (2021) 7:104–7. doi: 10.22271/oral.2021.v7.i1b.1124
165. Kretschmer T, Happel LT, England JD, Nguyen DH, Tiel RL, Beuerman RW, et al. Accumulation of PN1 and PN3 sodium channels in painful human neuroma-evidence from immunocytochemistry. Acta Neurochir (Wien). (2002) 144:803–10. doi: 10.1007/s00701-002-0970-112181690
166. England JD, Happel LT, Kline DG, Gamboni F, Thouron CL, Liu ZP, et al. Sodium channel accumulation in humans with painful neuromas. Neurology. (1996) 47:272–6. doi: 10.1212/wnl.47.1.2728710095
167. Black JA, Nikolajsen L, Kroner K, Jensen TS, Waxman SG. Multiple sodium channel isoforms and mitogen-activated protein kinases are present in painful human neuromas. Ann Neurol. (2008) 64:644–53. doi: 10.1002/ana.2152719107992
168. Bear D, Rummler L, Chao T, Mozaffar T, Gupta R. Localized ion channel migration after neural injury induces pain. In: Transactions of the orthopaedic research society 31. USA: Chicago (2006). p. 1868.
169. Cancio JM, Eskridge S, Shannon K, Orr A, Mazzone B, Farrokhi S. Development of overuse musculoskeletal conditions after combat-related upper limb amputation: a retrospective cohort study. J Hand Ther. (2021) S0894-1130(21)00075–2. doi: 10.1016/j.jht.2021.05.003
170. Ostlie K, Franklin RJ, Skjeldal OH, Skrondal A, Magnus P. Musculoskeletal pain and overuse syndromes in adult acquired major upper-limb amputees. Arch Phys Med Rehabil. (2011) 92:1967–1973.e1. doi: 10.1016/j.apmr.2011.06.02622133243
171. Burger H, Vidmar G. A survey of overuse problems in patients with acquired or congenital upper limb deficiency. Prosthet Orthot Int. (2016) 40:497–502. doi: 10.1177/030936461558465826023075
172. Ehde DM, Jensen MP, Engel JM, Turner JA, Hoffman AJ, Cardenas DD. Chronic pain secondary to disability: a review. Clin J Pain. (2003) 19:3–17. doi: 10.1097/00002508-200301000-0000212514452
173. de la Vega R, Groenewald C, Bromberg MH, Beals-Erickson SE, Palermo TM. Chronic pain prevalence and associated factors in adolescents with and without physical disabilities. Dev Med Child Neurol. (2018) 60:596–601. doi: 10.1111/dmcn.1370529468673
174. Creed F. A review of the incidence and risk factors for fibromyalgia and chronic widespread pain in population-based studies. Pain. (2020) 161:1169–76. doi: 10.1097/j.pain.000000000000181932040078
175. Levine NA, Rigby BR. Thoracic outlet syndrome: biomechanical and exercise considerations. Healthcare. (2018) 6:68. doi: 10.3390/healthcare602006829921751
176. Radhakrishnan K, Litchy WJ, O’Fallon WM, Kurland LT. Epidemiology of cervical radiculopathy: a population-based study from Rochester, Minnesota, 1976 through 1990. Brain. (1994) 117:325–35. doi: 10.1093/brain/117.2.3258186959
177. Fayaz A, Croft P, Langford RM, Donaldson LJ, Jones GT. Prevalence of chronic pain in the UK: a systematic review and meta-analysis of population studies. BMJ Open. (2016) 6:e010364. doi: 10.1136/bmjopen-2015-01036427324708
178. Domenichiello AF, Ramsden CE. The silent epidemic of chronic pain in older adults. Prog Neuropsychopharmacol Biol Psychiatry. (2019) 93:284–90. doi: 10.1016/j.pnpbp.2019.04.00631004724
179. Steingrímsdóttir ÓA, Landmark T, Macfarlane GJ, Nielsen CS. Defining chronic pain in epidemiological studies: a systematic review and meta-analysis. Pain. (2017) 158:2092–107. doi: 10.1097/j.pain.0000000000001009
180. Wolf JM, Cannada L, Van Heest AE, O’Connor MI, Ladd AL. Male and female differences in musculoskeletal disease. J Am Acad Orthop Surg. (2015) 23:339–47. doi: 10.5435/JAAOS-D-14-0002026001426
181. Ortner CM, Granot M, Richebé P, Cardoso M, Bollag L, Landau R. Preoperative scar hyperalgesia is associated with post-operative pain in women undergoing a repeat caesarean delivery. Eur J Pain. (2013) 17:111–23. doi: 10.1002/j.1532-2149.2012.00171.x22689634
182. Hanley MA, Jensen MP, Smith DG, Ehde DM, Edwards WT, Robinson LR. Preamputation pain and acute pain predict chronic pain after lower extremity amputation. J Pain. (2007) 8:102–9. doi: 10.1016/j.jpain.2006.06.00416949876
183. Langford DJ, Schmidt B, Levine JD, Abrams G, Elboim C, Esserman L, et al. Preoperative breast pain predicts persistent breast pain and disability after breast cancer surgery. J Pain Symptom Manage. (2015) 49:981–94. doi: 10.1016/j.jpainsymman.2014.11.29225527442
184. Monib S, Abdelaziz MI. Epidemiology and predictive factors for persistent breast pain following breast-conserving surgery. Cureus. (2021) 13:e14063. doi: 10.7759/cureus.1406333898146
185. Limakatso K, Bedwell GJ, Madden VJ, Parker R. The prevalence and risk factors for phantom limb pain in people with amputations: a systematic review and meta-analysis. PloS One. (2020) 15:e0240431. doi: 10.1371/journal.pone.024043133052924
186. Maccarrone J, Stripling A, Iannucci J, Nierenberg B. Exposure to trauma, PTSD and persistent pain in older adults: a systematic review. Aggress Violent Behav. (2021) 57:101488. doi: 10.1016/j.avb.2020.101488
187. Crombie IK, Davies HTO, Macrae WA. Cut and thrust: antecedent surgery and trauma among patients attending a chronic pain clinic. Pain. (1998) 76:167–71. doi: 10.1016/S0304-3959(98)00038-49696470
188. Castillo RC, MacKenzie EJ, Wegener ST, Bosse MJ. Prevalence of chronic pain seven years following limb threatening lower extremity trauma. Pain. (2006) 124:321–9. doi: 10.1016/j.pain.2006.04.02016781066
189. Kolstadbraaten KM, Spreng UJ, Wisloeff-Aase K, Gaarder C, Naess PA, Raeder J. Incidence of chronic pain 6 years after major trauma. Acta Anaesthesiol Scand. (2019) 63:1074–8. doi: 10.1111/aas.1338031012096
190. Radresa O, Chauny J-M, Lavigne G, Piette E, Paquet J, Daoust R. Current views on acute to chronic pain transition in post-traumatic patients: risk factors and potential for pre-emptive treatments. J Trauma Acute Care Surg. (2014) 76:1142–50. doi: 10.1097/TA.000000000000018824662883
191. Schug SA, Bruce J. Risk stratification for the development of chronic postsurgical pain. Pain Rep. (2017) 2:e627. doi: 10.1097/PR9.000000000000062729392241
192. Winfree CJ, Kline DG. Intraoperative positioning nerve injuries. Surg Neurol. (2005) 63:5–18. doi: 10.1016/j.surneu.2004.03.02415639509
193. Maekawa M, Yoshizawa E, Hayata G, Ohashi S. Physical and psychological effects of postural educational intervention for students experienced school refusal. Curr Psychol. (2021). doi: 10.1007/s12144-021-01677-9
194. Hunt C, Moman R, Peterson A, Wilson R, Covington S, Mustafa R, et al. Prevalence of chronic pain after spinal cord injury: a systematic review and meta-analysis. Reg Anesth Pain Med. (2021) 46:328–36. doi: 10.1136/rapm-2020-10196033408161
195. Nagoshi N, Kaneko S, Fujiyoshi K, Takemitsu M, Yagi M, Iizuka S, et al. Characteristics of neuropathic pain and its relationship with quality of life in 72 patients with spinal cord injury. Spinal Cord. (2016) 54:656–61. doi: 10.1038/sc.2015.21026620877
196. Turner JA, Cardenas DD, Warms CA, McClellan CB. Chronic pain associated with spinal cord injuries: a community survey. Arch Phys Med Rehabil. (2001) 82:501–9. doi: 10.1053/apmr.2001.2185511295011
197. Warner FM, Cragg JJ, Jutzeler CR, Finnerup NB, Werhagen L, Weidner N, et al. Progression of neuropathic pain after acute spinal cord injury: a meta-analysis and framework for clinical trials. J Neurotrauma. (2019) 36:1461–8. doi: 10.1089/neu.2018.596030417730
198. Modirian E, Pirouzi P, Soroush M, Karbalaei-Esmaeili S, Shojaei H, Zamani H. Chronic pain after spinal cord injury: results of a long-term study. Pain Med. (2010) 11:1037–43. doi: 10.1111/j.1526-4637.2010.00865.x20492574
199. Siddall PJ, McClelland JM, Rutkowski SB, Cousins MJ. A longitudinal study of the prevalence and characteristics of pain in the first 5 years following spinal cord injury. Pain. (2003) 103:249–57. doi: 10.1016/S0304-3959(02)00452-912791431
200. Rath GP, editor Handbook of trigeminal neuralgia. Singapore: Springer Singapore (2019). doi: 10.1007/978-981-13-2333-1
201. Chung MK, Wang S, Yang J, Alshanqiti I, Wei F, Ro JY. Neural pathways of craniofacial muscle pain: implications for novel treatments. J Dent Res. (2020) 99:1004–12. doi: 10.1177/002203452091938432374638
202. Sessle BJ. The neural basis of temporomandibular joint and masticatory muscle pain. J Orofac Pain. (1999) 13:238–45.10823036
203. Marbach JJ. Medically unexplained chronic orofacial pain. Temporomandibular pain and dysfunction syndrome, orofacial phantom pain, burning mouth syndrome, and trigeminal neuralgia. Med Clin North Am. (1999) 83:691–710, vi–vii. doi: 10.1016/s0025-7125(05)70130-910386121
204. Setty S, David J. Classification and epidemiology of orofacial pain. In: Vadivelu N, Vadivelu A, Kaye AD, editors. Orofacial pain. Cham: Springer International Publishing (2014). p. 15–24. doi: 10.1007/978-3-319-01875-1_3
205. Logan DE, Donado C, Kaczynski K, Lebel A, Schechter N. From one pain to many: the emergence of overlapping pains in children and adolescents. Clin J Pain. (2021) 37:404–12. doi: 10.1097/AJP.000000000000093933859112
206. Maixner W, Fillingim RB, Williams DA, Smith SB, Slade GD. Overlapping chronic pain conditions: implications for diagnosis and classification. J Pain. (2016) 17:T93–107. doi: 10.1016/j.jpain.2016.06.00227586833
207. Blake P, Burstein R. Emerging evidence of occipital nerve compression in unremitting head and neck pain. J Headache Pain. (2019) 20:76. doi: 10.1186/s10194-019-1023-y31266456
208. Herd CP, Tomlinson CL, Rick C, Scotton WJ, Edwards J, Ives N, et al. Botulinum toxins for the prevention of migraine in adults. Cochrane Database Syst Rev. (2018) 6:CD011616. doi: 10.1002/14651858.CD011616.pub229939406
209. Littlejohn GO, Guymer E. Chronic pain syndromes: overlapping phenotypes with common mechanisms. F1000Res. (2019) 8:255. doi: 10.12688/f1000research.16814.1
210. Aman MM, Mahmoud A, Waghray-Penmetcha T. Neuropathic pain: complex regional pain syndrome (CRPS). In: Shimoji K, Nader A, Hamann W, editors. Chronic pain management in general and hospital practice. Singapore: Springer Singapore (2021). p. 225–47. doi: 10.1007/978-981-15-2933-7_13
211. Seror P. Neuralgic amyotrophy. An update. Joint Bone Spine. (2017) 84:153–8. doi: 10.1016/j.jbspin.2016.03.00527263426
212. Brantigan CO, Roos DB. Diagnosing thoracic outlet syndrome. Hand Clin. (2004) 20:27–36. doi: 10.1016/s0749-0712(03)00080-515005381
213. Doneddu PE, Coraci D, De Franco P, Paolasso I, Caliandro P, Padua L. Thoracic outlet syndrome: wide literature for few cases. Status of the art. Neurol Sci. (2017) 38:383–8. doi: 10.1007/s10072-016-2794-427987052
214. Dorn P, Zehnder A, Kocher GJ. Das thoracic-outlet-syndrom: selten, vielmals verpasst oder zu häufig diagnostiziert? [thoracic outlet syndrome: rare, often missed or over-diagnosed?]. Praxis. (2021) 110:391–6. doi: 10.1024/1661-8157/a00366034019442
215. McGillicuddy JE. Cervical radiculopathy, entrapment neuropathy, and thoracic outlet syndrome: how to differentiate? Invited submission from the joint section meeting on disorders of the spine and peripheral nerves, march 2004. J Neurosurg Spine. (2004) 1:179–87. doi: 10.3171/spi.2004.1.2.017915347004
216. Hoffman GJ, Ha J, Alexander NB, Langa KM, Tinetti M, Min LC. Underreporting of fall injuries of older adults: implications for wellness visit fall risk screening. J Am Geriatr Soc. (2018) 66:1195–200. doi: 10.1111/jgs.1536029665016
217. Dellon AL. “Think nerve” in upper extremity reconstruction. Clin Plast Surg. (1989) 16:617–27. doi: 10.1016/S0094-1298(20)31327-42673640
218. Hendler N. Ethical issues in the medical assessment and subsequent treatment of chronic pain. In: Schatman ME, editors. Ethical issues in chronic pain management. Boca Raton: CRC Press (2007). p. 259–76.
219. Hendler NH, Kozikowski JG. Overlooked physical diagnoses in chronic pain patients involved in litigation. Psychosomatics. (1993) 34:494–501. doi: 10.1016/S0033-3182(93)71823-X8284339
220. Hendler N. Why chronic pain patients are misdiagnosed 40 to 80% of the time? J Recent Adv Pain. (2016) 2:94–8. doi: 10.5005/jp-journals-10046-0051
221. Kleinbrink EL, Nikitin AG, Keller O. Misdiagnosis of chronic pain: a bioethical problem. Mich Acad. (2007) 37:47–54.
222. Shapiro AP, Teasell RW. Misdiagnosis of chronic pain as hysteria and malingering. Curr Rev Pain. (1998) 2:19–28. doi: 10.1007/s11916-998-0059-5
223. Walker T, Cuff A, Salt E, Lynch G, Littlewood C. Examination of the neck when a patient complains of shoulder pain: a global survey of current practice (2019). Musculoskeletal Care. (2020) 18:256–64. doi: 10.1002/msc.145832162792
224. Pang HY, Farrer C, Wu W, Gakhal NK. Quality of rheumatology care for patients with fibromyalgia and chronic pain syndromes. BMJ Open Qual. (2021) 10:e001061. doi: 10.1136/bmjoq-2020-00106133766832
225. Militello LG, Anders S, Downs SM, Diiulio J, Danielson EC, Hurley RW, et al. Understanding how primary care clinicians make sense of chronic pain. Cogn Technol Work. (2018) 20:575–84. doi: 10.1007/s10111-018-0491-130842708
226. Nawabi D, Jayakumar P, Carlstedt T. Peripheral nerve surgery. Ann R Coll Surg Engl. (2006) 88:327–8. doi: 10.1308/003588406X106351c
227. Mackinnon SE, Yee A. Nerve surgery. Stuttgart: Georg Thieme Verlag (2015). doi: 10.1055/b-003-106416
228. Finnerup NB, Attal N, Haroutounian S, McNicol E, Baron R, Dworkin RH, et al. Pharmacotherapy for neuropathic pain in adults: a systematic review and meta-analysis. Lancet Neurol. (2015) 14:162–73. doi: 10.1016/S1474-4422(14)70251-025575710
229. Serrano Afonso A, Carnaval T, Videla Cés S. Combination therapy for neuropathic pain: a review of recent evidence. J Clin Med. (2021) 10:3533. doi: 10.3390/jcm1016353334441829
230. McDonagh MS, Selph SS, Buckley DI, Holmes RS, Mauer K, Ramirez S, et al. Nonopioid pharmacologic treatments for chronic pain. Rockville (MD): Agency for Healthcare Research and Quality (US) (2020).
231. Wessel LE, Fufa DT, Canham RB, La Bore A, Boyer MI, Calfee RP. Outcomes following peripheral nerve decompression with and without associated double crush syndrome: a case control study. Plast Reconstr Surg. (2017) 139:119–27. doi: 10.1097/PRS.000000000000286327627055
232. Stoy C, Beredjiklian P, Kreitz T, Tulipan J. Postoperative functional analysis of double crush versus single peripheral nerve decompression: a retrospective study. Hand N Y N. (2022): Online ahead of print. doi: 10.1177/15589447211038681
233. Skelly AC, Chou R, Dettori JR, Turner JA, Friedly JL, Rundell SD, et al. Noninvasive nonpharmacological treatment for chronic pain: a systematic review update. Rockville (MD): Agency for Healthcare Research and Quality (AHRQ) (2020). doi: 10.23970/AHRQEPCCER227
234. Masquelier E, D’haeyere J. Physical activity in the treatment of fibromyalgia. Joint Bone Spine. (2021) 88:105202. doi: 10.1016/j.jbspin.2021.10520233962033
235. Jesson T, Runge N, Schmid AB. Physiotherapy for people with painful peripheral neuropathies: a narrative review of its efficacy and safety. Pain Rep. (2020) 5:e1–834. doi: 10.1097/PR9.0000000000000834
236. Ferro Moura Franco K, Lenoir D, Dos Santos Franco YR, Jandre Reis FJ, Nunes Cabral CM, Meeus M. Prescription of exercises for the treatment of chronic pain along the continuum of nociplastic pain: a systematic review with meta-analysis. Eur J Pain. (2021) 25:51–70. doi: 10.1002/ejp.166632976664
237. Ho KY, Gwee KA, Cheng YK, Yoon KH, Hee HT, Omar AR. Nonsteroidal anti-inflammatory drugs in chronic pain: implications of new data for clinical practice. J Pain Res. (2018) 11:1937–48. doi: 10.2147/JPR.S16818830288088
238. Basson A, Olivier B, Ellis R, Coppieters M, Stewart A, Mudzi W. The effectiveness of neural mobilization for neuromusculoskeletal conditions: a systematic review and meta-analysis. J Orthop Sports Phys Ther. (2017) 47:593–615. doi: 10.2519/jospt.2017.711728704626
239. Strong JA, Zhang J-M, Schaible H-G. The sympathetic nervous system and pain. In: Wood JN, editors. The Oxford handbook of the neurobiology of pain. New York: Oxford University Press (2020). p. 155–78. doi: 10.1093/oxfordhb/9780190860509.013.6
240. Vickers AJ, Vertosick EA, Lewith G, MacPherson H, Foster NE, Sherman KJ, et al. Acupuncture for chronic pain: update of an individual patient data meta-analysis. J Pain. (2018) 19:455–74. doi: 10.1016/j.jpain.2017.11.00529198932
241. Hsu ES. Medication overuse in chronic pain. Curr Pain Headache Rep. (2017) 21:2. doi: 10.1007/s11916-017-0606-z28097532
242. Doughty CT, Bowley MP. Entrapment neuropathies of the upper extremity. Med Clin North Am. (2019) 103:357–70. doi: 10.1016/j.mcna.2018.10.01230704687
243. Laing TA, Sierakowski A, Elliot D. Management of painful scar-tethered cutaneous nerves of the upper limb. Hand Surg Rehabil. (2017) 36:208–14. doi: 10.1016/j.hansur.2017.03.00128465200
244. Tozzi P. Selected fascial aspects of osteopathic practice. J Bodyw Mov Ther. (2012) 16:503–19. doi: 10.1016/j.jbmt.2012.02.00323036882
245. Chao D, Tran H, Hogan QH, Pan B. Analgesic dorsal root ganglion field stimulation blocks both afferent and efferent spontaneous activity in sensory neurons of rats with monosodium iodoacetate-induced osteoarthritis. Osteoarthritis Cartilage. (2022) 30:1468–81. S1063-4584(22)00826–3. doi: 10.1016/j.joca.2022.08.00836030058
246. Tateuchi H, Akiyama H, Goto K, So K, Kuroda Y, Ichihashi N. Gait- and posture-related factors associated with changes in hip pain and physical function in patients with secondary hip osteoarthritis: a prospective cohort study. Arch Phys Med Rehabil. (2019) 100:2053–62. doi: 10.1016/j.apmr.2019.04.00631054296
247. Nagymate G, Pethes A, Szabo G, Bejek Z, Paroczai R, Kiss RM. The impact of the severity of knee osteoarthritis on the postural stability. Mater Today Proc. (2016) 3:1069–73. doi: 10.1016/j.matpr.2016.03.050
248. Truszczyńska A, Rąpała K, Gmitrzykowska E, Trzaskoma Z, Drzał-Grabiec J. Postural stability disorders in patients with osteoarthritis of the hip. Acta Bioeng Biomech. (2014) 16:45–50.
249. Moss P, Knight E, Wright A. Subjects with knee osteoarthritis exhibit widespread hyperalgesia to pressure and cold. PloS One. (2016) 11:e0147526. doi: 10.1371/journal.pone.014752626809009
250. Sadler KE, Mogil JS, Stucky CL. Innovations and advances in modelling and measuring pain in animals. Nat Rev Neurosci. (2022) 23:70–85. doi: 10.1038/s41583-021-00536-734837072
Keywords: chronic pain, nociceptive sensitization, nerve compression syndromes, nervous system trauma, neuroma, neuropathic pain, nociception, pain management and treatment
Citation: Macionis V (2023) Chronic pain and local pain in usually painless conditions including neuroma may be due to compressive proximal neural lesion. Front. Pain Res. 4:1037376. doi: 10.3389/fpain.2023.1037376
Received: 5 September 2022; Accepted: 12 January 2023;
Published: 20 February 2023.
Edited by:
Marco Pagliusi Jr., University of São Paulo, BrazilReviewed by:
Scott Holmes, Boston Children's Hospital and Harvard Medical School, United StatesPhilip Robert Effraim, Yale University, United States
Olga Kopach, University College London, United Kingdom
© 2023 Macionis. This is an open-access article distributed under the terms of the Creative Commons Attribution License (CC BY). The use, distribution or reproduction in other forums is permitted, provided the original author(s) and the copyright owner(s) are credited and that the original publication in this journal is cited, in accordance with accepted academic practice. No use, distribution or reproduction is permitted which does not comply with these terms.
*Correspondence: Valdas Macionis dmFsZGFzLm1hY2lvbmlzLm1kQGdtYWlsLmNvbQ==
†ORCID Valdas Macionis orcid.org/0000-0002-7517-895X
Specialty Section: This article was submitted to Pain Mechanisms, a section of the journal Frontiers in Pain Research