- Laboratoire Psychologie de la Perception, Université Paris Descartes and CNRS, Paris, France
By means of a new visuo-motor synchronization paradigm we test the frequently made proposition that one’s feeling of having voluntarily made a decision to act is in fact postdictively established contingent on the outcome of his action rather than on its aim. Subjects had to (1) synchronize a key-press with the end of a random synchronization interval (SI) shorter or longer than their reaction time (RT) and (2) judge thereafter whether (Q1) SI had been long enough to allow synchronization, (Q2) their motor response had been “reactive” (i.e., close to their RT) or delayed, or (Q3) whether SI was short or long. SI was denoted by the filling-up time of an annular “gauge.” In principle, the “synchronization” key-press should be reactive for SI ≤ RT and delayed in proportion with SI for SI > RT. Instead, response time distributions were bimodal for the shortest (0 ms) and longest (500 ms) SIs and widely spread for intermediate SIs. To all three questions asked, subjects’ responses strongly correlated with SI itself (r = 0.62−0.76) and barely with their actual response times (r = 0.03−0.42). Hence subjects’ introspective judgments on their trial-by-trial potential capability to synchronize their motor response (Q1) and on their reactive vs. delayed response mode reflected the objective cause of their action rather than being “corrupted” by its outcome (namely their actual response time). That subjects could not reliably decide whether their motor response was reactive or delayed implies that they did not have retrospective access to (or did not remember) their motor decisions which amounts to say that they could not decide on the intentionality of their actions.
Introduction
There are claims in the field of voluntary action that the sense of agency and hence of volition relies on a post-hoc (i.e., non-causal) evaluation of performed actions rather than on predictive mental processes (see reviews by Wegner and Wheatley, 1999; Wegner, 2002; Haggard, 2005, 2008, 2009; Knoblich and Sebanz, 2005; Hallett, 2007). Accordingly and in contrast with the “priority principle”1 (namely that “thought” comes before action; Wegner, 2002) the stand of a postdictive chain of mental events posits that action is promoted before thought (i.e., decision/intention to act) which is but an a posteriori interpretation of that action hence “corrupted” by the outcome of that action. It is in that sense that we equate “predictive” with “causal” (thought predicts/causes the ensued action) and postdictive with non-causal (action causes thought).
Most of the empirical approaches to the predictive–postdictive debate capitalized on the comparison between the introspected timing of the decision/intention to act and the timing of that action’s precursory neural activity (Libet, 1993; Lau et al., 2007; Haggard, 2008, 2009). This literature consistently showed that the introspected decision timing trails (sometimes by seconds; Soon et al., 2008) the timing of premotor activity. Such paradigms raise concerns about the very definition of intention, volition, etc. (e.g., Nachev et al., 2008), about subject’s capability of estimating the moment of his intention to act and about the techniques used for such assessments (Pockett, 2002; Danquah et al., 2008).
Here we introduce a new method of testing the stand of a postdictive appraisal of one’s intentionality. To be specific, we equate here “intentionality” with subjects’ capacity of assessing the physical causes of their actions inasmuch as the notion of intentionality is meaningless in the absence of such assessment. The method capitalizes on subject’s retrospective judgments on the feasibility of a just executed synchronization motor task and on whether this task had been executed in a speeded/“reactive” or delayed/“intentional” mode. Such distinction is not more conceptually debatable (Pashler, 1998; Nachev et al., 2008; Haggard, 2009) than the notion of volition/free will itself, ultimately an introspective belief. Here, the understanding is that a speeded/“reactive” action is spontaneous or irrepressible, hence non-intentional, while delayed/“intentional” requires pondering of stimulus parameters (such as speed) and internal factors (such as one’s reaction time, RT) and involves top-down processes (Frank et al., 2009). A reactive action can be objectively operationalized as an action whose latency with respect to stimulation is in the range of subject’s simple RT and is based on bottom-up processes. This does not mean however that subjects necessarily use such a criterion for segregating their spontaneous vs. delayed responses. In fact, the present data show that they do not.
Subjects were requested to synchronize a key-press with the termination of a synchronization interval (SI; randomly shorter or longer than their RT) and thereafter to judge (in separate sessions) whether that interval had been long enough to allow synchronization (Q1), whether their motor response had been reactive or delayed (Q2), or whether SI had been long or short (Q3).
A valid answer to Q1 requires subjects to compare their estimation of the current SI with their introspected RT, iRT (SI <> iRT). On the assumption that iRT is a constant (say the introspected mean RT acquired through life experience or within the very first trials of a RT experiment), subjects’ responses should correlate with SI irrespectively of their fluctuating synchronization response time (RsT), i.e., the outcome of their action. However, the postdictive stand requires that their response to Q1 should be based on their trial-by-trial RT appraisal, a capacity known to exist (James, 1890; Gorea et al., 2010). To answer Q2 subjects should compare their current RsT and iRT (RsT <> iRT) so that their responses should correlate with their fluctuating RsT but not (or less) with SI. Q2 is all the more meaningful given that for the same SI subjects showed a bimodal RsT distribution with means separated by more than 100 ms (see Results). To answer Q3 subjects only need to appraise the mean SI and the current SI (SI <> )2. Hence their responses should correlate again with SI. Basing their responses to Qs 1 and 3 on SI would substantiate the view that subjects have an objective appraisal of the external world (i.e., SI). In contrast, the stand of a postdictive/subjective appraisal of the world and hence of our intention to act upon it (i.e., react or delay our motor responses) predicts that subjects’ responses to Qs 1–3 will correlate with their RsT and/or with the RsT–SI difference more than with SI. For this to be the case, subjects must be able to appraise (consciously or not) and use their RsT on a trial-by-trial base. The present results show that subjects’ responses to the three questions asked mostly correlate with SI. We take this as evidence against the postdictive hypothesis. In the case of Q2, subjects’ reliance on SI shows that they prefer to rely on the external world even though such reliance is inappropriate (an “over-objectivization”). This suggests that subjects do not have conscious access to or, alternatively, do not remember what their decision (to react or delay their response) had been.
Materials and Methods
We describe below four distinct experiments two of which are preliminary. The purpose of preliminary experiments was to assess subjects’ simple RT in a standard RT paradigm using the stimuli of the two main experiments. These RTs were subsequently compared with subjects points of subjective synchronization (PSS) derived as described below. Main Experiment 1 was run with a large but sparse sample of SI that in conjunction with subjects’ answers to Q1 were used to choose a narrower but denser range of SI to be run in the main Experiment 2. In the latter subjects answered each of the three questions mentioned in the Introduction.
Stimuli
The stimulus was a 1° thick virtual annulus (the “gauge”) with a 3° external edge radius (Figure 1A) that started to fill up (randomly at one out of eight locations marked by a radial bar) either up white (100 cd/m2) or in black (0.05 cd/m2) on a 50 cd/m2 gray background. Polarity was swapped on each trial to prevent adaptation effects. The filling gage was displayed on a 19” E96f+SB ViewSonic screen (1024 × 768 pixels, 100 Hz refresh rate) 40 cm away from observers’ eyes. The filling-up was always counterclockwise (Figure 1B) and occurred randomly within one out of seven (0, 100, 200, 250, 300, 400, 500 ms; Preliminary 2 and Experiment 1), or out of 21 SIs (10 ms apart within a range of 200 ms more or less centered about each observer’s RT; Experiment 2; see Procedure). Stimulus presentation and response recording were controlled using the Psychtoolbox (Brainard, 1997; Pelli, 1997) under Matlab.
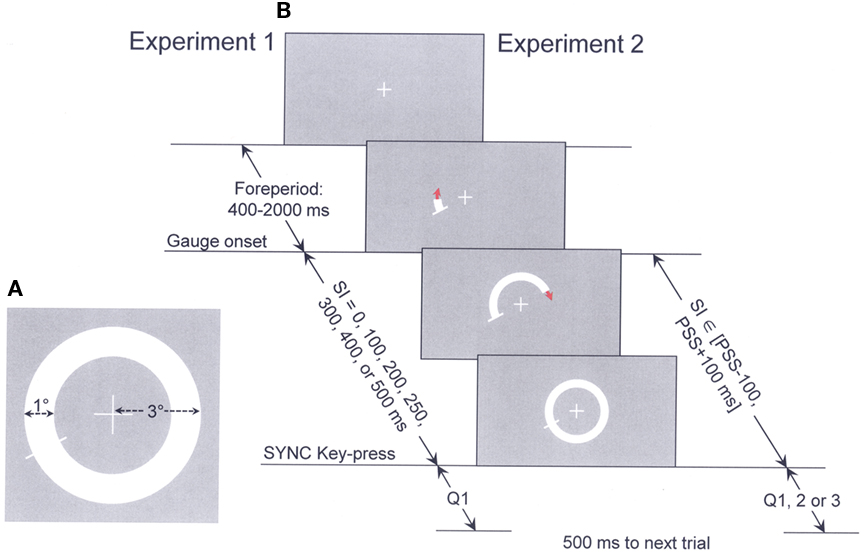
Figure 1. Spatial (A) and temporal (B) display of the stimulus. Note the different ranges of the SI used and the different questions asked in Experiments 1 and 2.
Procedure
Preliminary 1: Participants were asked to react (press a key) as soon as possible to the appearance of the annular gage that was filled up from the start (SI = 0). Each subject performed 100 trials in one single session. Preliminary 2: As above but this time with seven SIs (hence speeds) between 0 and 500 ms (see Stimuli) randomized across trials. There were 80 trials per SI and per subject distributed in two sessions.
Comparing the mean RTs obtained in Preliminary 1 and 2 was meant to test the SI effect per se. As no such effect was found, mean RTs were subsequently compared with subjects’ PSS derived from their binary classification responses in Experiments 1 and 2. In both these experiments participants were asked to synchronize their key-presses with the moment the filling of the gage was completed. In Experiment 1 this range was the same as in Preliminary 2. After each motor synchronization response participants provided a binary response to Q1:
Q1: Has the current SI been long enough for you to synchronize with?
The mean of the cumulative Gaussian fit to the frequency of “Yes” responses as a function of SI is referred to as participant’s PSS derived relatively to the SI, PSSSI. For the reasons given below, this PSSSI was used to determine for each subject a narrower and denser SI range to be run in Experiment 2. In this second experiment, subjects responded to the following two additional questions:
Q2: Have you reacted or delayed your response to achieve synchronization?
Q3: Has the current SI been short or long?3
To any of the questions above subjects responded by pressing the left (“Yes: long enough,” “reacted,” “short”) or right arrow key on the keyboard. Observers were specifically and repeatedly instructed not to base their response on the delay between the gauge filling up completion and their RsT.
Clearly, very short (SI ≪ RT) and very long (SI ≫ RT) SIs would (and did) entail 100% reliable responses to Q1 as such intervals will most obviously be beyond and within subject’s synchronization capacities independently of his RsT, or even in the absence of any motor response. This would also be the case for Qs 2, 3 (see Introduction and below). Instead, an SI range narrowly limited about the reaction/proaction transition point as given by PSSSI (and, as it will be shown, close to participant’s RT), will entail much less reliable answers to any of the three questions asked. This opens the possibility that participants will appeal to their introspectively estimated RsT as an alternative source of information (even though less reliable than a “direct” SI estimation; see Gorea et al., 2010). An additional advantage of using a relatively narrow SI range about each participant’s PSSSI is that it dilutes the correlation between SI and RsT, a necessary condition for disentangling (at least partly) participant’s reliance on one or the other. Reliance on RsT should be even more pronounced given that subjects can compare the moment of the completion of the gage (a visual signal) and their own RsT (motor and proprioceptive signals). Under such conditions, observers are capable of judging their synchronization response (i.e., whether it had been early or delayed) with an accuracy of less than ±50 ms, more than twice better than their perceptual estimation of the SI they have to synchronize with (Gorea et al., 2010).
Given the considerations above, Experiment 2 was a re-run of Experiment 1 but with a 200 ms SI range centered on each participant’s PSSSI. Each of the three questions was asked and answered in blocked sessions. There were 40 trials per SI and per session (i.e., 40 × 7 = 280 trials/session) repeated four times (i.e., a total of 160 trials per SI, participant and question asked). At least 5 s breaks were imposed after every 20 trials.
Subjects
They were five master students (four male) with normal or corrected to normal vision aged in-between 23–25 years. Only one of them had had extensive training with the specific paradigm and was acquainted with the purpose of the experiment.
Results
The median and mean RTs assessed with the filled gage (227.4 ± 15.9 ms and 230.6 ± 13.1 ms; Preliminary 1) and with the randomized filling-up durations (230.4 ± 8 ms and 242.6 ± 12.1; Preliminary 2) were not statistically different.
Figure 2A presents mean RsTs for each participant (different symbols; keep in mind that RT and RsT refer to response time measured in speeded and in a synchronization response mode, respectively) as a function of SI in Experiment 1. This representation is not quite legitimate as at least three of the seven RsT means per subject were derived from bimodal RsT distributions (as assessed by Akaike Information Criterion, AIC; Akaike, 1974), with all subjects showing bimodal RsT distributions for SI = 0 and for SI = 500 ms. These bimodal RsT distributions for the two extreme SIs are exemplified in Figures 2a1,a2 for a typical subject, SL). The average difference between the means of the two distributions is 129 ms for SI = 0 and of 109 ms for SI = 500 ms. Averaging RsTs over such bimodal distributions accounts for subjects’ too early synchronization responses (i.e., RsTs below the main dashed diagonal) for the longest two SIs (400 and 500 ms; for such SIs, subjects show perfect synchronization under blocked SI conditions; Gorea et al., 2010). While the source of this bimodality is of critical interest in understanding the synchronization process (an issue addressed in an ongoing study), it is of little relevance for the present topical issue. It is worth noting, however, that the means of the fastest RsT distributions averaged over the five subjects for SI = 0 (249 ± 29.8 ms) are close to and not significantly different from their mean RTs assessed in the two preliminary (RT) experiments (230.6 ± 13 and 242.6 ± 12.1 ms). This suggests that in mixed SI blocks including SIs too short to synchronize with, subjects’ behavior alternates in about equal proportions between reactive and delayed response modes.
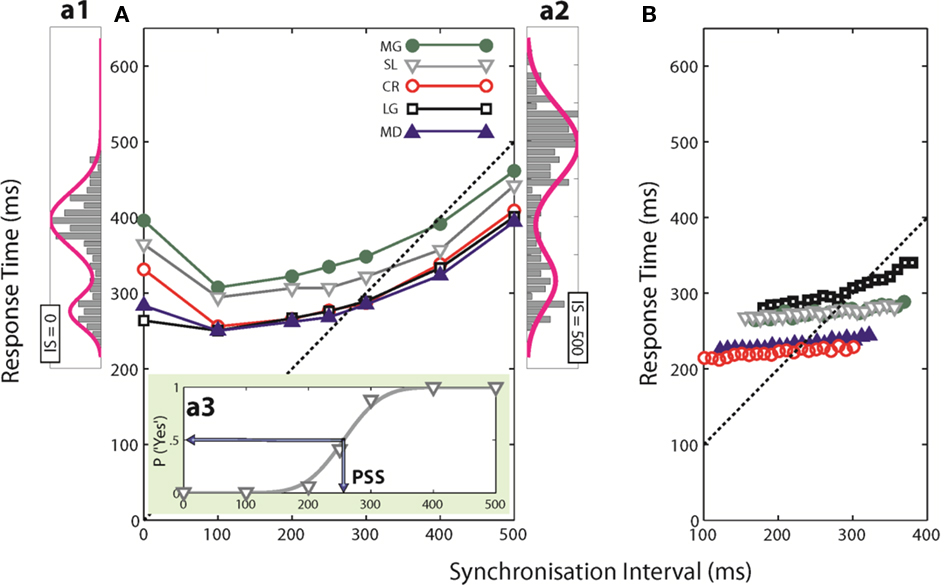
Figure 2. Synchronization response times (RsT) as a function of SI in Experiments 1 (A) and 2 (B) of all five participants (different symbols) together with representative bimodal RsT distributions of participant SL for SI = 0 (a1) and SI = 500 ms (a2) as well as a representative cumulative Gaussian fitted to this subject’s “Yes” responses to Q1 as a function of SI (a3). The dashed main diagonals in (A) and (B) show perfectly synchronized RsTs. The double arrowed right-angle lines in a3 point to this subject’s RsT at 50% “Yes” responses (i.e., the mean of the fitted Gaussian), i.e., his point of subjective synchronization referred to SI (PSSSI).
Figure 2a3 displays the cumulative Gaussian (μ, σ) fitted to subject’s SL “Yes” responses to Q1 in Experiment 1 (Neider–Mead simplex algorithm). The means of such functions fitted to each participant’s “Yes” responses are their PSSSI and have been used as midpoints of the 200 ms SI range tested in Experiment 2. A first notable observation from Figure 2 is that the PSSSI averaged over the five participants in Experiment 1 (246.4 ± 34.2 ms) are similar to these participants’ mean RTs assessed in the preliminary experiments and to their mean RsTs derived from their fastest RsT distributions for SI = 0 (249.0 ± 29.8 ms). This suggests that subjects have an accurate implicit knowledge of their mean RT and use it when answering Q1.
The restricted SI range about each participant’s PSSSI used in Experiment 2 (in-between 117–183 to 317–383 ms) ensured that they operated in an uncertainty react/delay range and that their RsTs were minimally correlated with SI (so as to maximize the disentanglement between subjects’ putative reliance, when answering any of the three questions, on SI from their reliance on RsT and, as a consequence, on the SI-RsT difference). That this was achieved is demonstrated by the shallow slopes [0.06–0.3; r ∈ (0.11–0.48)] of the mean RsT vs. SI functions displayed for each participant in Figure 2B (same symbols as in Figure 2A). It remains however that for this restricted 200 ms SI range, the RsT distributions covered an average range of 214 ± 27 ms across the five subjects, much smaller than the RT range obtained in the preliminary RT experiments over an equivalent SI range (126 ± 9 ms). This indicates that subjects tempted to synchronize their key-press with the completion of the SI rather than reacting to the onset of the gage. On a number of occasions, subjects may have nonetheless responded to the onset of the gage (i.e., reacted) rather than to its completion.
Figure 3 shows the percentages of “Yes” responses of the same representative participant as in Figures 2a1–a3 for each of the three questions asked (circles, squares and triangles for Q1, Q2, and Q3) together with the corresponding cumulative Gaussian fits as a function of SI (3a), RsT (3b), and SI-RsT (3c). Whatever the question asked, the steepest sigmoids (smallest σ) are obtained when the “Yes” responses are related to SI (σSI = 36.9 ± 1.7 ms), slightly shallower when related to SI-RsT (σSI-RsT = 43.1 ± 3.0 ms) and flat for all practical reasons when related to RsT (σRsT = 235.8 ± 102.2 ms). These observations are true for all participants (with two marginal exceptions out of 15 comparisons for Q1, subjects MD and CR) as shown in Table 1. While the possibility that subjects may have partially based their judgments on the SI-RsT difference cannot be entirely excluded, the present results show that this difference was not their main source of information.
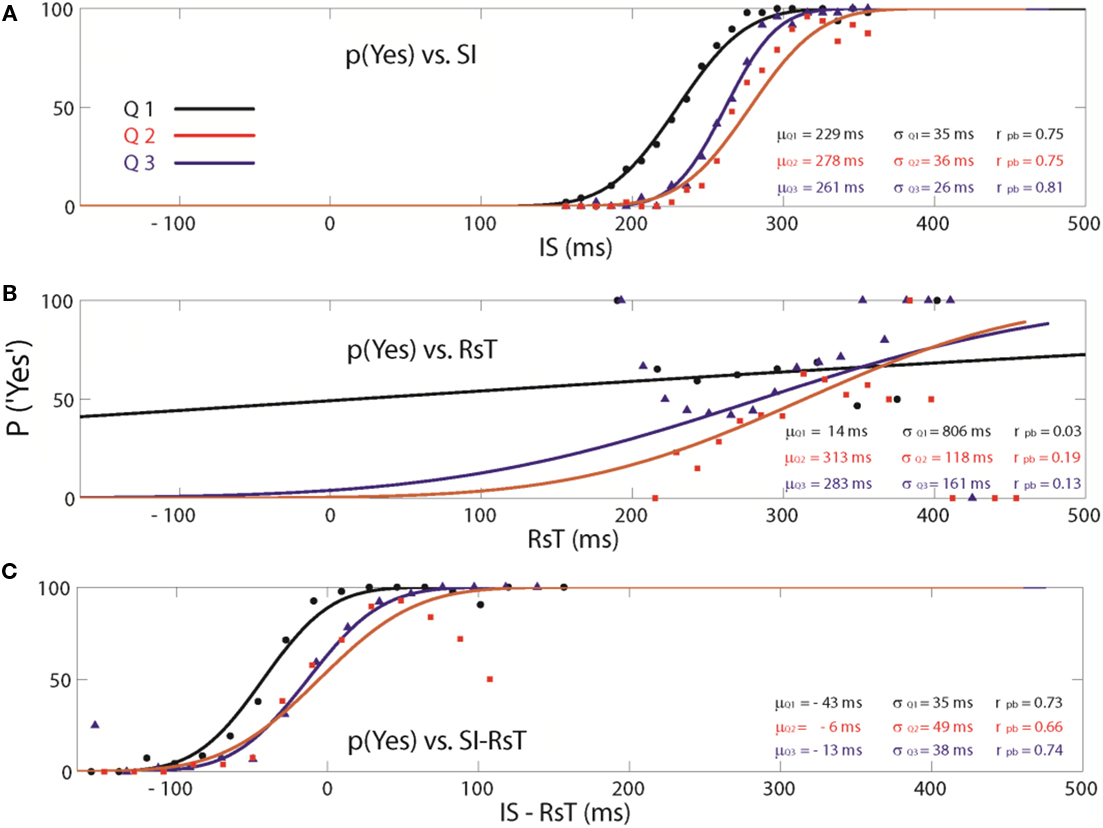
Figure 3. Percentage of “Yes” responses to Qs1-3 (circles, squares, and triangles, respectively) of a representative subject (SL) as a function of SI (A), RsT (B), and SI-RsT (C) together with the best cumulative Gaussian fits (sigmoids of corresponding colors). Insets show the respective means and SDs of the fits.
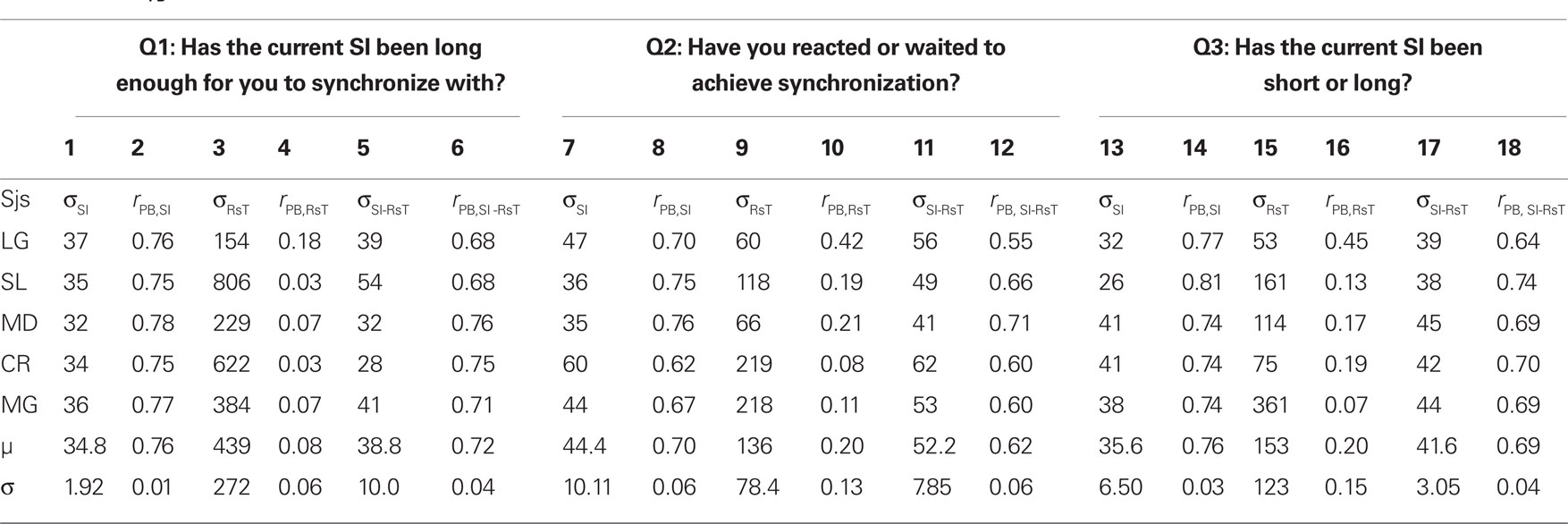
Table 1. Standard deviations of the cumulative Gaussians fitted to subjects’ “Yes” responses to each of the three questions asked in Experiment 2 as a function of the synchronization interval (σSI), response time (σRsT), and SI-RsT (σSI-RsT; odd cols) together with the corresponding point-biserial correlations (rPB; even cols).
At a first look, the accuracy of participants’ judgments appears to depend on the question asked (σQ1 = 170.9 ± 134.1; σQ2 = 68.3 ± 23.8; σQ3 = 76.7 ± 38.1 ms) but this is mainly due to the very inaccurate responses to Q1 when related to RsT (σRsT,Q1 = 439 ± 121.8 ms). A two-way ANOVA (factors: question-type and relevant index, i.e., SI, RsT, and SI-RsT) confirms these observations, namely a -significant index effect4 [F(2,8) = 13.8, p = 0.021] but no question-type effect (F = 5.3, p = 0.076). A partial comparison reveals a significantly steeper SI-related than (SI-RsT)-related slope [F(1,4) = 3.44, p = 0.02]. Table 1 also displays the point-biserial correlations (rPB; Lev, 1949) between the binary (Yes/No) and the continuous (SI, RsT, and SI-RsT) variables. As it should, the steepness of the fitted functions and rPB show a high negative correlation (r = −0.62, p ≪ 0.001). In short, the data clearly show that whatever the question asked participants base their responses on the actual duration of SI with practically no “postdictive” interference of their motor synchronization response.
Additional support for this conclusion transpires from a closer look at subjects’ PSSs and RTs. As already noted, RTs are practically identical when directly measured in the preliminary RT experiments with the gage instantaneously filled (SI = 0) or filling-up over 0 to 500 ms intervals or when derived from the fastest RsT distribution to the instantaneously filled up gage in the synchronization Experiment 1 (black circle, square, and triangle in column “RT” of Figure 4). To answer the introspective Qs 1 and 2, subjects must, in principle, refer their responses to their introspected RT. The fact that the PSSSI (column “PSS_SI” in Figure 4) derived from their answers to these questions (Q1, solid red and open blue circles for Experiments 1 and 2, respectively; Q2, open blue square, Experiment 2) are about equal to their RTs, while the corresponding PSSRsT (column “PSS_RsT”; same colors and symbols) differ markedly from the RTs as well as across experiments (circle vs. square) and subjects (error bars) support the notion that subjects’ introspection was based on the SIs rather than on their own RsT. As the mean of the SIs used in Experiment 2 was by construction equal to the PSSSI derived in Experiment 1 (red solid circle), the PSSs derived from subjects’ responses to Q3 (that bore on the SI itself) should coincide with the mean SI. This is the case for the PSSSI but not for the PSSRsT implying once again that subjects based their responses to Q3, as they should have, on the estimated SI and not on their own RsT. Hence, subjects base their judgments on the objective duration of the interval they have to synchronize with whether in response to an introspective (Q1, Q2) or “objective” (Q3) question. Finally, the possibility that subjects calibrated their responses (whatever the question asked) relatively to their average RsT is not supported by the data as these averages (“AV_RsT” column in Figure 4) are significantly above subjects’ RT and PSSSI.
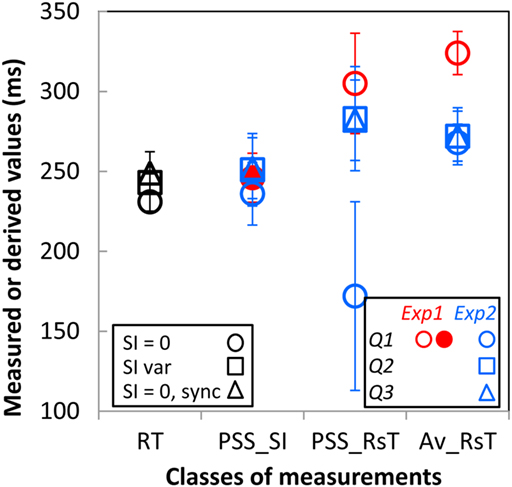
Figure 4. Reaction times (RT; black symbols), points of subjective synchronization derived from the cumulative Gaussian fits of the “Yes” responses as a function of the SI (PSS_SI) and as a function of subjects’ synchronization response time (PSS_RsT) and the means of these RsT (Av_RsT) as measured or derived from the different experiments. RTs measured in response to the instantaneously filled (SI = 0) gage and to a gage filling over 0 to 500 ms intervals are shown as the black circle and square, respectively. RT derived from the fastest RsT distribution to the instantaneously filled gage in the synchronization Experiment 1 is shown a the black triangle. PSS and Av_RsT derived from responses to questions 1, 2, and 3 are shown as circles, squares, and triangles with their colors referring to the Experiment where they have been assessed (red, Experiment 1; blue, Experiment 2). The solid red circle is the PSSSI from Experiment 1 used as the midpoint of the SI range tested in Experiment 2. Each symbol is the average over the five participants with the vertical bars showing 1SE.
Discussion
The present experiments demonstrate that despite observers -having explicit feedback on their motor synchronization response with the completion of a random time interval, their introspective judgment of their synchronization capacity relative to the length of this interval (Q1) or of them having been in a reactive or delayed motor response mode (Q2) are poorely, if at all, related to the timing of their actual synchronization response but strongly related to the physical duration of this interval. In fact, the accuracy of such introspective judgments is no different from that assessed when subjects are asked to judge the “absolute” duration of these same intervals (Q3). The statistical analysis shows that the accuracy of subjects’ judgments is higher when these judgments are referred to the time interval itself rather than to the difference between this interval and the timing of their synchronization response. The data also show that subjects’ PSS are not significantly different from their mean RT and less variable (over experiments and subjects) when derived from subjects’ answers to any of the three questions asked as a function of the actual duration of the SI than as a function of their synchronization RsT.
While it has been shown that judgements of a stimulus having entailed an action (such as the location of a saccade target and of the corresponding saccade landing) are not biased by putative motor errors (Collins et al., 2009; Munuera et al., 2009; Ostendorf et al., 2010), the present study is the first to show that introspection on one’s actions in relation to the events having triggered them is based on these events rather than on the outcome of the actions they have entailed. Notwithstanding, such introspection appears to be calibrated with respect to subjects’ introspected mean reaction time. The observation that responses to Q2, that bears on subjects’ past decision to react or to delay action, do not correlate with their actual RsT implies that, at least in the present experimental conditions, subjects cannot access or, alternatively, do not remember what this decision had been. This observation raises serious doubts about the experimental paradigm used in many studies where subjects were asked to specify when their decision to act had been taken (e.g., Libet, 1993; see Pockett, 2002; Danquah et al., 2008).
Reactive and delayed movements are known to reflect (e.g., Frith et al., 1991; Jahanshahi et al., 1995; Jenkins et al., 2000; Brass and Haggard, 2007; Haggard, 2008, 2009) and actually be triggered by the activity of distinct neural networks hypothesized to subtend reflexive and deliberate actions, respectively (Desmurget et al., 2009). While the distinction between such responsive modes is fuzzy (Nachev et al., 2008; Ridderinkhof et al., 2011), it is widely accepted that they involve bottom-up vs. top-down processes (e.g., Frank et al., 2009; Ridderinkhof et al., 2011). Hence their segregation according to the response latencies remains an acceptable operationalization.
The anatomo-physiological distinction between the two response modes does not appear to be sustained by subjects’ presently assessed introspective judgments of their actions having been reactive or delayed (Q2). This is so even though, in contrast with previous laboratory manipulations of volition (of the kind “Have free will now!” – see Haggard, 2009), subjects’ switch from a reactive to a delayed response mode was entirely under their own control. The conundrum appears to be solved, though not conceptually, by Desmurget et al.’s (2009) astonishing findings that stimulation of distinct cortices may trigger the “intention and desire” to move a limb or the lips together with the feeling of having done so in the absence of any corresponding electromyographic activity (inferior parietal regions) and reciprocally, an overt movement without the “feeling” of having executed it (premotor area). In as much as the “consciousnesses” of acting and the action itself appear to be dissociated (or at least dissociable), it is not more remarkable to admit that reactive and delayed actions may not be consciously dissociable either. Such dissociations are actually amply documented in amputees and anosognostic patients (for the first type of dissociation) and in patients with utilization behavior or with delusions of control (for the second type of dissociation; Frith et al., 2000). Temptingly, one would take such intention/action dissociations as evidence in favor of the notion that free will is but an illusion (Wegner, 2002). The present results provide paradoxical denotations in this respect. On the one hand, subjects’ incapacity of retrospectively classifying their reactive vs. delayed motor response (Q2) based on the actual latency of this response comforts the view of an illusory free will. On the other hand, the fact that subjects judge their capacity of synchronizing a motor response with a random time interval based on their estimation of this interval rather than on their introspected response time (Q1) implies that their judgment is causal (or predictive) in nature (i.e., not based on the outcome of their action or postdictive), a prerequisite of the free will stand. Hence, not astonishingly, the present experiments and results reveal an intrinsic duality of the free will concept debated since the antiquity (e.g., Bobzien, 1998; O’Keefe, 2005).
Conflict of Interest Statement
The authors declare that the research was conducted in the absence of any commercial or financial relationships that could be construed as a potential conflict of interest.
Acknowledgments
Thanks to Patrick Cavanagh, Pascal Mamassian, and Florian Waszak for helpful discussions. This work was supported by grant ANR-06-NEURO-042-01 from AgenceNationale pour la Recherche.
Footnotes
- ^To the priority principle Wegner adds the “consistency” (that thought be compatible with the action it triggers) and exclusivity (that thought be the only cause of action) principles.
- ^Estimating the mean of a uniform distribution has been shown to be achieved over the first 10–20 trials and to yield discrimination accuracies in a 2AFC task equivalent to those obtained in the presence of the standard (Morgan et al., 2000; Brown and Steyvers, 2005; Gorea et al., 2010).
- ^Note that none of the three Qs bears on participant’s estimate of his key-press being early or delayed with respect to SI.
- ^All effects are corrected for sphericity – Greenhouse–Geisser.
References
Akaike, H. (1974). A new look at the statistical model identification. IEEE Trans. Automat. Contr. 19, 716–723.
Brass, M., and Haggard, P. (2007). To do or not to do: the neural signature of self control. J. Neurosci. 27, 9141–9145.
Brown, S., and Steyvers, M. (2005). The dynamics of experimentally induced criterion shifts. J. Exp. Psychol. Learn. Mem. Cogn. 31, 587–599.
Collins, T., Rolfs, M., Deubel, H., and Cavanagh, P. (2009). Post-saccadic location judgments reveal remapping of saccade targets to non-foveal locations. J. Vis. 9, 1–9.
Danquah, A. N., Farrell, M. J., and O’Boyle, D. J. (2008). Biases in the subjective timing of perceptual events: Libet et al. (1983) revisited. Conscious. Cogn. 17, 616–627.
Desmurget, M., Reilly, K. T., Richard, N., Szathmari, A., Mottolese, C., and Sirigu, A. (2009). Movement intention after parietal cortex stimulation in humans. Science 324, 811–813.
Frank, M. J., Cohen, M. X., and Sanfey, A. G. (2009). Multiple systems in decision making: a neurocomputational perspective. Curr. Dir. Psychol. Sci. 18, 73–77.
Frith, C. D., Blakemore, S. J., and Wolpert, D. M. (2000). Abnormalities in the awareness and control of action. Philos. Trans. R Soc. Lond B Biol. Sci. 355, 1771–1788.
Frith, C. D., Friston, K., Liddle, P. F., and Frackowiak, R. S. J. (1991). Willed action and the prefrontal cortex in man: a study with PET. Proc. R Soc. Lond B 244, 241–246.
Gorea, A., Mamassian, P., and Cardoso-Leite, P. (2010). Introspective duration estimation of reactive and proactive motor responses. Acta Psychol. 134, 142–153.
Hallett, M. (2007). Volitional control of movement: the physiology of free will. Clin. Neurophysiol. 118, 1179–1192.
Jahanshahi, M., Jenkins, I. H., Brown, R. G., Marsden, C. D., Passingham, R. E., and Brooks, D. J. (1995). Self-initiated versus externally triggered movements. An investigation using measurement of regional cerebral blood flow with PET and movement-related potentials in normal and Parkinson’s disease subjects. Brain 118, 913–933.
Jenkins, I. H., Jahanshahi, M., Jueptner, M., Passingham, R. E., and Brooks, D. J. (2000). Self-initiated versus externally triggered movements. II. The effect of movement predictability on regional cerebral blood flow. Brain 123, 1216–1228.
Lau, H. C., Rogers, R. D., and Passingham, R. E. (2007). Manipulating the -experienced onset of intention after action execution. J. Cogn. Neurosci. 19, 1–10.
Libet, B. (1993). Neurophysiology of Consciousness: Selected Papers and New Essays by Benjamin Libet. Boston, MA: Birkhauser.
Morgan, M. J., Watamaniuk, S. N. J., and McKee, S. P. (2000). The use of an implicit standard for measuring discrimination thresholds. Vision Res. 40, 2341–2349.
Munuera, J., Morel, P., Duhamel, J. R., and Deneve, S. (2009). Optimal sensorimotor control in eye movement sequences. J. Neurosci. 29, 3026–3035.
Nachev, P., Husain, M., and Kennard, C. (2008). Volition and eye movements. Prog. Brain Res. 171, 391–398.
Ostendorf, F., Liebermann, D., and Ploner, C. J. (2010). Human thalamus contributes to perceptual stability across eye movements. Proc. Natl. Acad. Sci. U.S.A. 107, 1229–1234.
Pelli, D. G. (1997). The VideoToolbox software for visual psychophysics: transforming numbers into movies. Spat. Vis. 10, 437–442.
Pockett, S. (2002). On subjective back-referral and how long it takes to become conscious of a stimulus: a reinterpretation of Libet’s data. Conscious. Cogn. 11, 144–161.
Ridderinkhof, K. R., Forstmann, U. B., Scott, A. W., Burle, B., and van den Wildenberg, W. P. M. (2011). Neurocognitive mechanisms of action control: resisting the call of the Sirens. Wiley Interdiscip. Rev. Cogn. Sci. 2, 174–192.
Soon, C. S., Brass, M., Heinze, H.-J., and Haynes, J.-D. (2008). Unconscious determinants of free decisions in the human brain. Nat. Neurosci. 11, 543–545.
Keywords: introspection, motor synchronization, reaction time, delayed action, postdiction
Citation: Gorea A and Rider D (2011) Introspecting on the timing of one’s actions in a visuo-motor synchronization task. Front. Psychology 2:106. doi: 10.3389/fpsyg.2011.00106
Received: 28 March 2011;
Accepted: 10 May 2011;
Published online: 27 May 2011.
Edited by:
Morten Overgaard, Aarhus University, Aarhus University Hospital, DenmarkReviewed by:
Sebastien Marti, Institut National de la Santé et de la Recherche Médicale, FranceMyrto Loanna Mylopoulos, City University of New York, USA
Copyright: © 2011 Gorea and Rider. This is an open-access article subject to a non-exclusive license between the authors and Frontiers Media SA, which permits use, distribution and reproduction in other forums, provided the original authors and source are credited and other Frontiers conditions are complied with.
*Correspondence: Andrei Gorea, Laboratoire Psychologie de la Perception, Université Paris Descartes and CNRS, Biomédicale des Saints Pères, 45 rue des Saints Pères, 75006 Paris, France. e-mail: andrei.gorea@parisdescartes.fr