- 1Department of Nutritional Sciences, School of Public Health, University of Michigan, Ann Arbor, MI, United States
- 2Center for Research on Nutrition and Health, National Institute of Public Health, Cuernavaca, Mexico
- 3Department of Environmental Health Sciences, School of Public Health, University of Michigan, Ann Arbor, MI, United States
- 4Center for Human Growth and Development, University of Michigan, Ann Arbor, MI, United States
- 5Department of Biostatistics, School of Public Health, University of Michigan, Ann Arbor, MI, United States
Introduction: Cadmium is a pervasive toxic metal that remains a public health concern and exposure in early life has been associated with growth deficits in infancy and childhood. Growth during adolescence also may be sensitive to effects of cadmium exposure, given the changes in distribution of lean and adipose tissue that vary by sex during puberty. This study examines whether prenatal and concurrent cadmium exposures are associated with adiposity measures at ages 8–15 years in a well-characterized birth cohort.
Methods: The sample included 185 participants from the ELEMENT birth cohorts in Mexico City with complete data on urinary cadmium exposures, anthropometry and covariates [child age and sex, household socioeconomic status, and maternal smoking history and body mass index (BMI)]. Maternal third trimester and adolescent urines were analyzed for cadmium using an Inductively Coupled Plasma Mass Spectrometer. Trained personnel obtained anthropometry including height, weight, waist circumference and subscapular, suprailiac, and triceps skinfold thickness. BMI z-scores for age and sex were calculated using the World Health Organization's reference standard. Linear regression models were used to estimate the association of prenatal and concurrent urinary cadmium levels with adolescent anthropometry, adjusting for covariates.
Results: Among 87 males and 98 females, median age was 10 years (IQR 9 –11 years). Pregnant women and children had median urinary cadmium concentrations of 0.19 μg/L (IQR 0.12– 0.27 μg/L) and 0.14 μg/L (IQR 0.11– 0.18 μg/L), respectively. Regression models showed inverse relationships between prenatal cadmium exposure and adolescent adiposity. An IQR increase in prenatal cadmium was associated with percent decreases in BMI z-score (−27%, p = 0.01), waist circumference (−3%, p = 0.01), and subscapular (−11%, p = 0.01), suprailiac (−11%, p = 0.02), and triceps (−8%, p < 0.01) skinfold thickness. When stratified by sex, these relationships remained statistically significant in females but not males.
Conclusions: Prenatal cadmium exposure was negatively associated with measures of both abdominal and peripheral adiposity in girls, but not in boys. These results emphasize the sex-dependent effects of in utero cadmium exposure on adiposity in adolescence.
Introduction
Cadmium is a pervasive toxic metal with two main routes of human exposure: inhalation of cigarette smoke and ingestion of foods with high levels of cadmium, such as leafy vegetables, potatoes, grains, peanuts, soybeans, and sunflower seeds (1). Although largely sourced from healthy foods—vegetables and whole grains (2)—cadmium exposure is associated with several health outcomes even at low exposure levels (3). Prenatal exposure is a public health concern for children, where sensitivities to cadmium can be pronounced and have lasting health effects (4, 5).
Several prospective studies have related prenatal cadmium exposure to growth deficits in infancy and early childhood (6–10). Cross-sectional studies in adolescents have shown inverse relationships of cadmium exposure with abdominal and subcutaneous fat (11, 12) and obesity (13, 14). One report examining the effects of both prenatal and childhood cadmium exposure on growth in a rural Bangladesh population (10) found the strongest inverse relationship between concurrent exposure and height at 5 years of age. These findings suggest that physical growth during both prenatal and postnatal periods may be sensitive to effects of cadmium exposure, but more studies are needed that examine effects across multiple developmental stages.
It is not known whether effects of prenatal exposure on growth persist into adolescence or how concurrent adolescent cadmium exposure may impact this relationship. Focusing on adolescent adiposity outcomes is important given the dynamic physiologic changes occurring during the pubertal transition, including increases in weight and height velocity, accumulation and changes in lean and fat mass, and reproductive initiation and subsequent secretion of sex steroids (15, 16). Body composition changes—increase in total body mass and its distribution—are related to pubertal development and vary substantially by sex (17, 18). Cadmium exposure is associated with decreases in female and male sex hormones and a delay in pubertal development (19–22). Given the role of cadmium as an endocrine disrupting chemical, both in utero and peripubertal exposures may have significant effects on adiposity in adolescence (23, 24).
This study considers whether prenatal and concurrent cadmium exposures are associated with height, weight, BMI, and skinfold thickness at ages 8–15 years in a well-characterized birth cohort.
Methods
Study Population
The study population and methods for measuring cadmium exposure and anthropometric outcomes have been described in detail elsewhere (25, 26). Briefly, our study population consists of participants from the Early Life Exposure in Mexico to Environmental Toxicants (ELEMENT) project, wherein three sequentially enrolled cohorts of pregnant women were recruited between 1997 and 2004 from maternity hospitals in Mexico City serving low- to moderate-income populations. During their third trimester—mean gestation week 34 (standard deviation (SD) 2.3, range 25.1–40.4)—pregnant women provided a urine sample, anthropometry, and completed interview-based questionnaires. Between 2008 and 2011, a subset of child participants, who were now 8–15 years of age, were re-contacted based on availability of stored cord blood and maternal urine. At the time of re-recruitment, urine samples, anthropometry, and interview-based questionnaires were collected for children and adolescents (hereafter referred to only as “adolescents”).
The ethics and research committees of the Mexico National Institute of Public Health and the University of Michigan approved all research protocols, and all participants provided informed consent prior to enrollment.
Cadmium Exposures
Urinary cadmium (UCd) was measured in 214 maternal third trimester (fasting spot) and 250 adolescent (arbitrary spot) urines. Urine was collected in sterile cups and samples were aliquot an hour after collection, frozen at −20 degrees Fahrenheit, and then moved to −70 degree Fahrenheit freezer for storage.
UCd was determined as previously described (27, 28). Briefly, 0.5 mL of sample was digested with an equal volume of concentrated nitric acid overnight in a fume hood. Milli-Q water was then added to the digest to bring the acid content to 2% (28). The digest was analyzed for Cd using an Inductively Coupled Plasma Mass Spectrometer (ICPMS; Varian 820MS). Potential elemental interferences were minimized via the ICPMS' collision reaction interface. The analytical accuracy was determined using a urinary reference material obtained from the Institut National de Santé Publique du Québec (QMEQAS10U-04) that has certified values for Cd. Every batch of 20 samples included a reference material and in total 25 measures were taken. The mean (SD) concentration of Cd measured in the reference material was 1.7 (0.1) μg/L compared to the actual certified value of 2.1 μg/L thus resulting in an accuracy of 82%. The analytical precision of urinary Cd was determined by creating a pool of urine that was run in each batch so that inter-day variability could be assessed. Every batch of 20 samples included a pooled sample and in total 25 measures were taken. The mean (SD) concentration of Cd measured in the pool was 0.13 (0.02) μg/L, providing a coefficient of variation (or precision) of 16%. In addition, each batch run contained procedural blanks that yielded a value of 0.01 (0.01) μg/L. The analytical detection limit was set as three times the standard deviation of the mean blank value, and was set at 0.04 μg/L; 190 (99%) of maternal and 215 (95%) of adolescent samples were greater than or equal to the limit of detection (LOD). For participants with UCd concentrations below the LOD, we retained UCd values between zero and the LOD.
UCd was corrected for specific gravity (SG) using the following equation: Pc = P[(SGp-1)/(SGi-1)] where Pc is SG-corrected UCd (μg/L), P is the measured UCd, SGp is the population-specific median urinary specific gravity (maternal = 1.013, adolescent = 1.018), and SGi is the individual's urinary specific gravity. Specific gravity (SG) was measured using a handheld digital refractometer (Atago Co., Ltd., Tokyo, Japan).
Anthropometry
Trained study personnel used standard protocols to measure adolescents' anthropometry including waist circumference (cm), subscapular, suprailiac, and triceps skinfold thickness (mm), height (cm), and weight (kg). Subcutaneous subscapular and suprailiac skinfolds provide measures of truncal fat distribution and triceps skinfolds, obtained in the upper arm, reflect peripheral fat distribution. Skinfolds were measured with a calibrated caliper (Lange, Beta Technology) to the nearest 0.1 mm. Height and weight were obtained using professional scales (PAME, Puebla, Puebla) to the nearest 0.1 cm and 0.1 kg, respectively. Body mass index (BMI), defined as weight (kg) divided by height (m) squared , was calculated. Adolescent BMI z-scores were defined as BMI for age and sex, using the World Health Organization's reference standard (29).
Covariates
Covariates included child age and sex and maternal BMI. Household socioeconomic (SES) level was measured with a 13-item score used in international settings to classify household assets (30). We coded maternal smoking history as never- or ever-smoker. Among ever-smokers, two percent reported smoking during pregnancy. Among mothers who reported a positive history of smoking, 70 percent also reported someone else smoking inside the home, including spouse, father, mother, and others. Thus, adolescents in our sample with a maternal history of smoking were potentially exposed from smokers in the home including mothers who often relapse by their child's first birthday (31, 32).
Lead Exposure
Maternal bone (patella) lead levels were assessed at 1 month postpartum using in vivo K-X-ray fluorescence to describe cumulative in utero lead exposure (26, 33). Bone lead measures were available for a subset of the sample (n = 164). The distributions of cadmium exposure and anthropometric outcomes did not differ between those with and without lead measurements.
Dietary Intake
Diets of pregnant women were assessed during the third trimester using an interview-administered semi-quantitative food frequency questionnaire (FFQ) designed to allow recall of dietary intake over the previous month (34). The questionnaire was translated and validated for use in the Mexican Spanish-speaking adult women of reproductive age; the list of about 100 foods was built from items that proved most representative of local consumption under the 1983 Dietary Survey of the Mexican National Institute of Nutrition (35, 36).
The energy content of each item in the FFQ (for a standard portion size: one natural unit, cup, slice, piece, etc.) was obtained from food composition tables supplied by two sources (Instituto Nacional de Salud Pública 2002): (1) the United States Department of Agriculture (USDA) and (2) the Mexican National Institute of Nutrition and Medical Sciences Salvador Zubirán (37). Frequency values, ranging from never to six or more times a day, were multiplied by the energy amount in each food item and then summed over all food sources to estimate the energy equivalent amount per day.
Statistical Analysis
All statistical analyses were conducted using SAS version 9.2 (SAS Institute, Cary, NC, USA). The analytic dataset was limited to 185 adolescents, based on complete information of both prenatal and concurrent cadmium exposure measure and other covariates, including adolescent age and sex, household socioeconomic level, and maternal smoking history. No statistically significant difference was detected in mean cadmium exposures or anthropometry between the analyses of data with complete cases vs. data with all available cases.
Univariate statistics characterized distributions of cadmium exposure and anthropometric outcome variables. Variables with non-normal distributions were ln-transformed. Both prenatal and concurrent cadmium distributions were non-normal right-skewed; ln-transformed cadmium concentrations provided bell-shaped distributions and were used in analyses. Anthropometric outcomes that were ln-transformed include height, weight, waist circumference (WC) and subscapular, suprailiac, and triceps skinfold thicknesses (SFT); BMI z-score was not ln-transformed. Through the residual analysis, the appropriateness of such transformation was confirmed by the quantile-quantile normal plot. Wilcoxon signed-rank sum test determined the difference in median values by sex.
Bivariate correlation coefficients were used to identify covariates marginally associated with exposure and outcome. Potential covariates included birth cohort, adolescent age and sex, SES level, and maternal BMI and smoking history. Maternal bone lead and dietary intake were included in linear regression models as confounders.
Multiple linear regression was used to quantify the relationship of prenatal or concurrent cadmium exposure with anthropometric outcomes, unadjusted and adjusting for covariates. Each outcome was assessed individually. These relationships were quantified within the entire analytic sample and for prenatal and concurrent cadmium exposure, in separate analyses stratified by sex. We determined correlation between maternal-child cadmium measures (Figure 1) and estimated multiple linear regression of both prenatal and concurrent exposure on anthropometric outcomes. Results were back-transformed for interpretability and are reported as percent change in anthropometric outcome per inter-quartile range (IQR) increase in cadmium exposure. An interaction between maternal smoking history and cadmium exposure was not significant in linear regression models.
Confounding by maternal lead levels and dietary intake were assessed on the association of prenatal cadmium with anthropometry. Maternal lead exposure was correlated with prenatal cadmium exposure (r = 0.17, p = 0.028) and previous studies in this cohort demonstrate relationships of prenatal lead with childhood growth (26, 33, 38). Maternal fruit and vegetable intake was positively associated with urinary cadmium in pregnant women in our population, as previously published (25) and literature suggests that maternal diet is related to anthropometry in childhood (39).
Robust multiple linear regression was used to obtain stable results in the presence of substantial outliers in the UCd data. We used the classical multiple M-estimation procedure that combines high breakdown value estimation (Least Trimmed Squares) and conventional Huber's M estimation (40) to produce robust estimation and inference for association. Robust multiple linear regression models for prenatal cadmium associations with adiposity resulted in significant inverse relationships similar to multiple linear regression results reported.
Results
The study population comprised 87 males and 98 females (median age 10 years, SD 1.5) residing in Mexico City. Data for the median and variation of cadmium exposures and anthropometry can be found in Table 1, overall and stratified by sex. Pregnant women and adolescents had median urinary cadmium concentrations of 0.19 μg/L (IQR 0.12–0.27 μg/L) and 0.14 μg/L (IQR 0.11–0.18 μg/L), respectively. Differences by sex were detected in skinfold thicknesses, with females having higher values reflecting expected shifts in fat distribution observed during peripuberty.
The results shown in Table 2 are obtained from separate models estimating the association of prenatal or concurrent cadmium exposure with adolescent anthropometry. Adjusted linear regression models showed negative relationships between prenatal cadmium exposure and adolescent anthropometry: height (p = 0.36), weight (p = 0.01), BMI z-scores (p = 0.01), waist circumference (p = 0.01), and subscapular (p = 0.01), suprailiac (p = 0.02), and triceps (p < 0.01) skinfold thickness (Table 2). A prenatal Cd exposure increase from the 25th to the 75th percentile (0.12–0.27 μg/L) was associated with a percent decrease in waist circumference (−3%) and subscapular, suprailiac, and triceps skinfold thickness (−11, −11, −8%, respectively); indicating higher prenatal cadmium exposure was associated with a decrease in both indicators of subcutaneous as well as abdominal fat. An IQR increase in prenatal Cd exposure was associated with a 27% decrease in BMI z-score, equivalent to a change of −0.27 in BMI z-score. Prenatal cadmium was negatively associated with weight. When stratified by sex, these relationships remained significant in girls but not boys (Table 3).
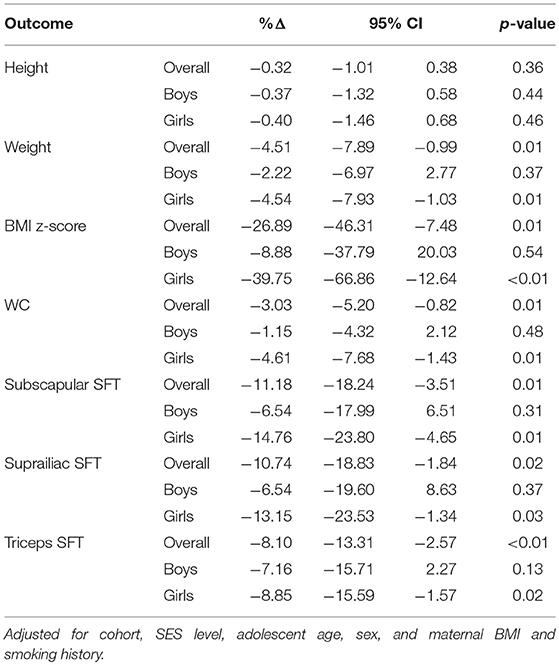
Table 3. Percent change in anthropometric outcomes per IQR increase in prenatal UCd stratified by sex.
Adjusted linear regression models suggested negative relationships between concurrent cadmium exposure and adolescent anthropometry, although none were statistically significant (Table 2).
Supplemental Table 1 shows results obtained from additional sets of covariates included in the linear regression models. No correlation between cadmium exposure measures was found (r = −0.02, p = 0.75), which eliminates the potential collinearity issue when both are included in one model. Inclusion of both exposures in a single model to account for possible shared variance strengthened effect estimates by <10 percent and did not alter significance. Models with maternal lead and dietary intake did not substantially change effect estimates and confidence intervals overlap. Such a comparison of models exhibits the sensitivity or robustness of a chosen model on examining the relationship of prenatal UCd with anthropometric outcomes in adolescence.
Discussion
We considered the independent associations of cadmium exposure in two time periods with adolescent anthropometry and found that prenatal but not concurrent cadmium exposure was significantly inversely associated with BMI z-score and measures of fat distribution, e.g., waist circumference and skinfolds. After stratifying by sex, we showed that these relationships were significant in females but not males. This is the first study to test whether the effects of prenatal cadmium exposure persist into adolescence, accounting for concurrent exposure.
Other published results are consistent with an inverse relationship between prenatal cadmium exposure and anthropometric outcomes in younger children (6–9, 41). A prospective study among Flemish children found inverse associations between prenatal cadmium exposure and measures of abdominal (WC) and subcutaneous fat (sum of subscapular, suprailiac, triceps, and biceps skinfold thickness) in females but not males 7–9 years of age. Our study similarly found statistically significant associations in females. However, our study differs from this and other reports by following children into adolescence and accounting for concurrent cadmium exposure.
Our study also has the advantage of using maternal urinary instead of cord blood cadmium to assess prenatal exposure. While blood and urinary cadmium are correlated, they may measure different aspects of exposure, dose and body burden. Blood cadmium is a measure of much more recent exposure (3–4 months) than that of urinary cadmium (10–30 years) (3). Additionally, placental transport of cadmium is limited (8, 42, 43), so offspring cord blood cadmium characterizes only direct recent exposure to the fetus, whereas maternal urinary cadmium may be more representative of maternal health throughout pregnancy (7, 8, 44). Evidence from animal studies suggest that fetuses exposed indirectly to Cd via maternal injection are more at risk than those directly exposed, possibly due to placental accumulation of Cd that results in trophoblastic damage (45), leading to decrease in utero-placental blood flow, and then a decrease in nutrient and oxygen transport (24). Use of maternal urinary cadmium to assess prenatal exposure could also be more indicative of effects on fetal epigenetic programming. A recent study found differences in epigenetic DNA methylation among infants and children at 4.5 years according to maternal cadmium exposure (46), with changes in methylation sites related to lower birth weight.
The only prior longitudinal study assessing cadmium exposure in multiple time periods did not find an independent association of prenatal cadmium exposure with growth of children in rural Bangladesh. After adjusting for prenatal and concurrent cadmium exposure and covariates (including exposure to lead), only concurrent childhood exposure was inversely associated with height and weight at 5 years (10). The inconsistency with our findings could be due to lower exposure levels in our sample; mean maternal urinary cadmium was 0.35 μg/L in our population compared with 0.65 μg/L in mothers of children in rural Bangladesh. Effects on growth at low levels of cadmium exposure previously have been documented in the literature with stronger associations observed for exposures below the median compared with those above the median (10, 12). Gardner et al. (10) did not assess measures of adiposity, e.g., BMI, WC and skinfold thicknesses among Bangladeshi children. Finally, these null findings were in children at 5 years of age whereas we examined the relationship of in utero cadmium exposure to anthropometry in adolescence, when measures of adiposity can change rapidly among boys and girls during puberty. We found a suggestive inverse relationship between concurrent cadmium exposure and adiposity. Previous cross-sectional studies in adolescents also have demonstrated negative relationships between concurrent cadmium exposure and weight, height, BMI, and WC (11, 12).
Cadmium may disrupt fat distribution through its endocrine properties, which may underlie the sex-specific results. Evidence in humans and animal studies suggests that cadmium disrupts steroidogenesis, particularly in the placenta, and acts as an endocrine-disrupting chemical, capable of the stimulation or inhibition of steroid hormonal activity (endogenous estradiol) (23, 24, 47). In laboratory studies, cadmium was observed to interact with both estrogen and androgen receptors and to activate the estrogen receptor alpha (48–50). The receptor-mediated mechanism of action may explain cadmium's pronounced effects at low-levels of exposure (12). In view of the stimulation of lipid mobilization and lipolysis known to be induced by sex hormones (51), this xenoestrogenic activity might contribute to the negative association with body fat observed for girls. In contrast, cadmium exposure in adolescent boys has been associated with lower circulating levels of estradiol and testosterone in the blood (22). Finally, in experimental animals, high doses of cadmium have been shown to lower plasma concentrations of insulin-like growth factor 1, a hormone critical for childhood growth, and disrupt pituitary levels (52–54).
Our study had the advantage of measuring confounding from lead and diet. When adjusting for cumulative in utero lead exposure, as measured by maternal patella bone lead, prenatal cadmium was still significantly inversely associated with adolescent weight, BMI z-score, WC, and SFT. Similarly, when Tian et al. adjusted for prenatal blood lead, prenatal cadmium remained significantly inversely associated with infant length and weight and child height at 4.5 years (47). Inclusion of maternal fruit and vegetable intake strengthened effects estimates, suggesting our first model may have underestimated the total effect of prenatal cadmium on adiposity. These results indicate that prenatal cadmium was independently associated with adolescent adiposity.
This study examined the relationship of cadmium exposure during two sensitive developmental periods with adolescent adiposity. Findings must be interpreted in light of several limitations. Use of a single urine sample to quantify UCd does not account for within-person variation and could attenuate associations with anthropometric outcomes (55). However, adjustment for urinary specific gravity can account for urine dilution. Our sample size was limited (n = 185) to participants with both measures of exposure, and stratification by sex limited our ability to consider interactions among covariates. Nevertheless, estimates of main effects reported here did not differ from models in which at least one time point for cadmium exposure was available (n = 192 prenatal and n = 223 concurrent) (results not shown). Finally, while we were able to account for maternal smoking history prior to pregnancy, more precise measures such as prenatal cotinine level and smoking habits of children may have provided more insight into relationships between different sources of cadmium exposure and growth outcomes.
Conclusions
Prenatal cadmium exposure was negatively associated with measures of abdominal and peripheral adiposity in peripubertal girls, but not in boys. Prenatal cadmium remained an independent predictor of adiposity after adjusting for concurrent cadmium exposure and maternal lead exposure and dietary intake. These results emphasize the sex-dependent adiposity effects of cadmium exposure in utero that persist into adolescence.
Author Contributions
MM and KP designed research. MM conducted research. MM and PS analyzed data. MM wrote the paper. AM-G and MT-R performed fieldwork. AC managed FFQ data. AJ provided global context. JC examined exposure data. MM and KP had primary responsibility for final content. All authors read and approved the final manuscript.
Conflict of Interest Statement
The authors declare that the research was conducted in the absence of any commercial or financial relationships that could be construed as a potential conflict of interest.
Acknowledgments
This study was supported by NIEHS 1R01ES021446-01, P20 ES018171-01/RD834800, P01 ES02284401/RD 83543601, and the National Institute of Public Health/Ministry of Health of Mexico. We also acknowledge the American British Cowdray Hospital for providing the facilities used for this research.
Supplementary Material
The Supplementary Material for this article can be found online at: https://www.frontiersin.org/articles/10.3389/fpubh.2019.00061/full#supplementary-material
Abbreviations
SD, Standard deviation; IQR, Interquartile range; UCd, Urinary cadmium; BMI, Body mass index; WC, Waist circumference, SFT, Skinfold thickness.
References
1. U.S. Agency for Toxic Substances and Disease Registry. Toxicological Profile for Cadmium. Atlanta, GA: U.S. Agency for Toxic Substances and Disease Registry (2012).
2. Egan SK, Bolger PM, Carrington CD. Update of US FDA's total diet study food list and diets. J Expo Sci Env Epidemiol. (2007) 17:573–82. doi: 10.1038/sj.jes.7500554
3. Järup L, Åkesson A. Current status of cadmium as an environmental health problem. Toxicol Appl Pharmacol. (2009) 238:201–8. doi: 10.1016/j.taap.2009.04.020
4. Barker DJ. The developmental origins of adult disease. J Am Coll Nutr. (2004) 23(Suppl. 6):588S−95S. doi: 10.1080/07315724.2004.10719428
5. Barouki R, Gluckman PD, Grandjean P, Hanson M, Heindel JJ. Developmental origins of non-communicable disease: implications for research and public health. Environ Health. (2012) 11:42. doi: 10.1186/1476-069X-11-42
6. Delvaux I, Van Cauwenberghe J, Den Hond E, Schoeters G, Govarts E, Nelen V, et al. Prenatal exposure to environmental contaminants and body composition at age 7-9 years. Environ Res. (2014) 132:24–32. doi: 10.1016/j.envres.2014.03.019
7. Tian LL, Zhao YC, Wang XC, Gu JL, Sun ZJ, Zhang YL, et al. Effects of gestational cadmium exposure on pregnancy outcome and development in the offspring at age 4.5 years. Biol Trace Elem Res. (2009) 132:51–9. doi: 10.1007/s12011-009-8391-0
8. Lin CM, Doyle P, Wang D, Hwang YH, Chen PC. Does prenatal cadmium exposure affect fetal and child growth? Occup Env Med. (2011) 68:641–6. doi: 10.1136/oem.2010.059758
9. Cheng L, Zhang B, Zheng T, Hu J, Zhou A, Bassig B, et al. Critical windows of prenatal exposure to cadmium and size at birth. Int J Environ Res Public Health. (2017) 14:E58. doi: 10.3390/ijerph14010058
10. Gardner RM, Kippler M, Tofail F, Bottai M, Hamadani J, Grandér M, et al. Environmental exposure to metals and children's growth to age 5 years: a prospective cohort study. Am J Epidemiol. (2013) 177:1356–67. doi: 10.1093/aje/kws437
11. Padilla MA, Elobeid M, Ruden DM, Allison DB. An examination of the association of selected toxic metals with total and central obesity indices: NHANES 99-02. Int J Environ Res Public Health. (2010) 7:3332–47. doi: 10.3390/ijerph7093332
12. Dhooge W, Den Hond E, Koppen G, Bruckers L, Nelen V, Van De Mieroop E, et al. Internal exposure to pollutants and body size in flemish adolescents and adults: associations and dose-response relationships. Environ Int. (2010) 36:330–7. doi: 10.1016/j.envint.2010.01.005
13. Shao W, Liu Q, He X, Liu H, Gu A, Jiang Z. Association between level of urinary trace heavy metals and obesity among children aged 6–19 years: NHANES 1999–2011. Environ Sci Pollut Res. (2017) 24:11573–81. doi: 10.1007/s11356-017-8803-1
14. Riederer AM, Belova A, George BJ, Anastas PT. Urinary cadmium in the 1999–2008 U.S. National Health and Nutrition Examination Survey (NHANES). Environ Sci Technol. (2013) 47:1137–47. doi: 10.1021/es303556n
15. Kelsey MM, Zeitler PS. Insulin resistance of puberty. Curr Diab Rep. (2016) 16:64. doi: 10.1007/s11892-016-0751-5
16. Alberga AS, Sigal RJ, Goldfield G, Prud Homme D, Kenny GP. Overweight and obese teenagers: why is adolescence a critical period? Pediatr Obes. (2012) 7:261–73. doi: 10.1111/j.2047-6310.2011.00046.x
17. Rodríguez G, Moreno LA, Blay MG, Blay VA, Garagorri JM, Sarría A, et al. Body composition in adolescents: measurements and metabolic aspects. Int J Obes Relat Metab Disord. (2004) 28(Suppl. 3):S54–8. doi: 10.1038/sj.ijo.0802805
18. Tanner JM, Whitehouse RH. Revised standards for triceps and subscapular skinfolds in British children. Arch Dis Child. (1975) 50:142–5. doi: 10.1136/adc.50.2.142
19. Gollenberg AL, Hediger ML, Lee PA, Himes JH, Louis GMB. Association between lead and cadmium and reproductive hormones in peripubertal U.S. girls. Env Heal Perspect. (2010) 118:1782–7. doi: 10.1289/ehp.1001943
20. Interdonato M, Pizzino G, Bitto A, Galfo F, Irrera N, Mecchio A, et al. Cadmium delays puberty onset and testis growth in adolescents. Clin Endocrinol. (2015) 83:357–62. doi: 10.1111/cen.12704
21. Samuel JB, Stanley JA, Princess RA, Shanthi P, Sebastian MS. Gestational cadmium exposure-induced ovotoxicity delays puberty through oxidative stress and impaired steroid hormone levels. J Med Toxicol. (2011) 7:195–204. doi: 10.1007/s13181-011-0143-9
22. Dhooge W, den Hond E, Koppen G, Bruckers L, Nelen V, van de Mieroop E, et al. Internal exposure to pollutants and sex hormone levels in flemish male adolescents in a cross-sectional study: associations and dose-response relationships. J Expo Sci Env Epidemiol. (2009) 21:106–13. doi: 10.1038/jes.2009.63
23. Henson MC, Chedrese PJ. Endocrine disruption by cadmium, a common environmental toxicant with paradoxical effects on reproduction. Exp Biol Med. (2004) 229:383–92. doi: 10.1177/153537020422900506
24. Takiguchi M, Yoshihara S. New aspects of cadmium as endocrine disruptor. Environ Sci. (2006) 13:107–16.
25. Moynihan M, Peterson KE, Cantoral A, Song PXK, Jones A, Solano-González M, et al. Dietary predictors of urinary cadmium among pregnant women and children. Sci Total Environ. (2017) 575:1255–62. doi: 10.1016/j.scitotenv.2016.09.204
26. Afeiche M, Peterson K, Sánchez B, Cantonwine D, Lamadrid-Figueroa H, Schnaas L, et al. Prenatal lead exposure and weight of 0-to 5-year-old children in Mexico city. Env Heal Perspect. (2011) 119:1436–41. doi: 10.1289/ehp.1003184
27. Basu N, Abare M, Buchanan S, Cryderman D, Nam D-H, Sirkin S, et al. A combined ecological and epidemiologic investigation of metal exposures amongst indigenous peoples near the marlin mine in western Guatemala. Sci Total Environ. (2010) 409:70–7. doi: 10.1016/j.scitotenv.2010.09.041
28. Srigboh RK, Basu N, Stephens J, Asampong E, Perkins M, Neitzel RL, et al. Multiple elemental exposures amongst workers at the agbogbloshie electronic waste (E-Waste) site in ghana. Chemosphere. (2016) 164:68–74. doi: 10.1016/j.chemosphere.2016.08.089
30. Asociación Mexicana de Agencias de Investigación y Opinión Publica. Niveles Socio Economicos. (2009).
31. Homish GG, Leonard KE. Spousal influence on smoking behaviors in a US community sample of newly married couples. Soc Sci Med. (2005) 61:2557–67. doi: 10.1016/j.socscimed.2005.05.005
32. Merzel C, English K, Moon-Howard J. Identifying women at-risk for smoking resumption after pregnancy. Matern Child Heal J. (2010) 14:600–11. doi: 10.1007/s10995-009-0502-1
33. Afeiche M, Peterson KE, Sánchez BN, Schnaas L, Cantonwine D, Ettinger AS, et al. Windows of lead exposure sensitivity, attained height, and body mass index at 48 months. J Pediatr. (2012) 160:1044–9. doi: 10.1016/j.jpeds.2011.12.022
35. Hernández M, Aguirre J, Serrano L. Alimentación de obreros y sus familias, México. División de Nutritición de Comunidad INelNSZ. National Institute of Medical Science and Nutrition Salvador Zubiran: México (1983).
36. Willett WC, Sampson L, Stampfer MJ, Rosner B, Bain C, Witschi J, et al. Reproducibility and validity of a semiquantitative food frequency questionnaire. Am J Epidemiol. (1985) 122:51–65. doi: 10.1093/oxfordjournals.aje.a114086
37. Bourges H. Tablas de Composición de Alimentos “50 Aniversario Instituto Nacional de Nutrición.” National Institute of Medical Science and Nutrition Salvador Zubiran (1996).
38. Gonzalez-Cossio T, Peterson KE, Sanin LH, Fishbein E, Palazuelos E, Aro A, et al. Decrease in birth weight in relation to maternal bone-lead burden. Pediatrics. (1997) 100:856–62. doi: 10.1542/peds.100.5.856
39. Vidakovic AJ, Gishti O, Voortman T, Felix JF, Williams MA, Hofman A, et al. Maternal plasma PUFA concentrations during pregnancy and childhood adiposity: the generation R study. Am J Clin Nutr. (2016) 103:1017–25. doi: 10.3945/ajcn.115.112847
40. Yohai VJ. High breakdown point and high efficiency robust estimates for regression. Ann Stat. (1987) 15:642–56. doi: 10.1214/aos/1176350366
41. Fábelová L, Vandentorren S, Vuillermoz C, Garnier R, Lioret S, Botton J. Hair concentration of trace elements and growth in homeless children aged. Environ Int. (2017) 114:318–25. doi: 10.1016/j.envint.2017.10.012
42. Osman K, Akesson A, Berglund M, Bremme K, Schütz A, Ask K, et al. Toxic and essential elements in placentas of Swedish women. Clin Biochem. (2000) 33:131–8. doi: 10.1016/S0009-9120(00)00052-7
43. Zhou C, Zhang R, Cai X, Xiao R, Yu H. Trace elements profiles of maternal blood, umbilical cord blood, and placenta in Beijing, China. J Matern Neonatal Med. (2017) 21:1–7. doi: 10.1080/14767058.2017.1416602
44. Vacchi-Suzzi C, Kruse D, Harrington J, Levine K, Meliker JR. Is urinary cadmium a biomarker of long-term exposure in humans? a review. Curr Environ Health Rep. (2016) 3:450–8. doi: 10.1007/s40572-016-0107-y
45. Erboga M, Kanter M. Effect of cadmium on trophoblast cell proliferation and apoptosis in different gestation periods of rat placenta. Biol Trace Elem Res. (2016) 169:285–93. doi: 10.1007/s12011-015-0439-8
46. Kippler M, Engström K, Jurkovic Mlakar S, Bottai M, Ahmed S, Hossain MB, et al. Sex-specific effects of early life cadmium exposure on DNA methylation and implications for birth weight. Epigenetics. (2013) 8:494–503. doi: 10.4161/epi.24401
47. Tian H, Chen S, Leng Y, Li T, Li Z, Chen H, et al. Exposure to cadmium during gestation and lactation affects development and function of leydig cells in male offspring. Environ Toxicol. (2017) 33:351–60. doi: 10.1002/tox.22522
48. Martin MB, Voeller HJ, Gelmann EP, Lu J, Stoica EG, Hebert EJ, et al. Role of cadmium in the regulation of AR gene expression and activity. Endocrinology. (2002) 143:263–75. doi: 10.1210/endo.143.1.8581
49. Stoica A, Katzenellenbogen BS, Martin MB. Activation of estrogen receptor-α by the heavy metal cadmium. Mol Endocrinol. (2000) 14:545–53. doi: 10.1210/mend.14.4.0441
50. Parodi D, Greenfield M, Evans C, Chichura A, Alpaugh A, Williams J, et al. Alteration of mammary gland development and gene expression by in utero exposure to cadmium. Int J Mol Sci. (2017) 18:1939. doi: 10.3390/ijms18091939
51. Hackney AC, Muoio D, Meyer WR. The effect of sex steroid hormones on substrate oxidation during prolonged submaximal exercise in women. Jpn J Physiol. (2000) 50:489–94. doi: 10.2170/jjphysiol.50.489
52. Lafuente A, Cano P, Esquifino AI. Are cadmium effects on plasma gonadotropins, prolactin, ACTH, GH and TSH levels, dose-dependent? Biometals. (2003) 16:243–50. doi: 10.1023/A:1020658128413
53. Turgut S, Kaptanoglu B, Turgut G, Emmungil G, Genc O. Effects of cadmium and zinc on plasma levels of growth hormone, insulin-like growth factor I, and insulin-like growth factor-binding protein 3. Biol Trace Elem Res. (2005) 108:197–204. doi: 10.1385/BTER:108:1-3:197
54. Caride A, Fernandez-Perez B, Cabaleiro T, Tarasco M, Esquifino AI, Lafuente A. Cadmium chronotoxicity at pituitary level: effects on plasma ACTH, GH, and TSH daily pattern. J Physiol Biochem. (2010) 66:213–20. doi: 10.1007/s13105-010-0027-5
Keywords: adiposity, adolescence, cadmium, prenatal, sex-dependent
Citation: Moynihan M, Telléz-Rojo MM, Colacino J, Jones A, Song PXK, Cantoral A, Mercado-García A and Peterson KE (2019) Prenatal Cadmium Exposure Is Negatively Associated With Adiposity in Girls Not Boys During Adolescence. Front. Public Health 7:61. doi: 10.3389/fpubh.2019.00061
Received: 30 October 2018; Accepted: 27 February 2019;
Published: 12 April 2019.
Edited by:
Mohiuddin Md. Taimur Khan, Washington State University, United StatesReviewed by:
Sofia Kottou, National and Kapodistrian University of Athens, GreeceMarie-Cecile Genevieve Chalbot, College of New Rochelle, United States
Copyright © 2019 Moynihan, Telléz-Rojo, Colacino, Jones, Song, Cantoral, Mercado-García and Peterson. This is an open-access article distributed under the terms of the Creative Commons Attribution License (CC BY). The use, distribution or reproduction in other forums is permitted, provided the original author(s) and the copyright owner(s) are credited and that the original publication in this journal is cited, in accordance with accepted academic practice. No use, distribution or reproduction is permitted which does not comply with these terms.
*Correspondence: Karen E. Peterson, a2FyZW5lcEB1bWljaC5lZHU=