- 1School of Stomatology, Lanzhou University, Lanzhou, China
- 2Institute of Modern Physics, Chinese Academy of Sciences, Lanzhou, China
- 3Institute of Solid Mechanics, School of Civil Engineering and Mechanics, Lanzhou University, Lanzhou, China
Oral cancer is a very aggressive disease with high rates of recurrence and metastasis. This study aimed at addressing how efficiently tongue cancer is suppressed after carbon ion irradiation. Here, the close relationship between upregulated expression of focal adhesion kinase (FAK) and high metastatic status in tongue squamous cell carcinoma patients was validated using bioinformatics and immunohistochemical analyses. Our data indicated that FAK suppression significantly enhanced the killing effect induced by irradiation in the tongue cancer cell line CAL27, as evidenced by increased apoptotic induction and reduced colony formation. More importantly, in FAK-deficient cells, carbon ion irradiation was shown to remarkably inhibit migration and invasion by delaying wound healing and slowing down motility. Further studies revealed that irradiation exposure caused disorganization of the actin cytoskeleton and reduced cell adhesive energy in FAK-deficient cells. Moreover, carbon ion treatment, in combination with FAK silencing, markedly blocked the phosphorylation levels of FAK, and paxillin, which partly contributed to the reduced motility of tongue squamous cell carcinoma CAL27 cells. Collectively, these results suggest that the prominent obstructing role of carbon ion irradiation in the growth inhibition and metastatic behavior of tumors, including attenuation of cell adhesiveness, motility, and invasiveness, could be distinctly modulated by FAK-mediated downstream pathways.
Introduction
Oral squamous cell carcinoma (OSCC) is the most lethal head and neck squamous cell carcinoma, with an increasing incidence among younger subjects (1, 2). Over the past decades, the prognosis of OSCC patients has remained dismally poor despite remarkable improvements in surgery, chemotherapy, and radiotherapy (3–5). A retrospective review of clinical outcomes showed that the 5-year survival rate of patients with OSCC who underwent postoperative radiation therapy (PORT) using cobalt 60 photons was prominently lower than those who did not undergo PORT (6). Moreover, approximately one-third of patients with OSCC experience locoregional recurrence or distant metastases after multimodality management, including PORT (7). Hence, the development of more effective radiation treatment for OSCC therapy is imperative.
Growing evidence indicates that radiotherapy with heavy ions is advantageous in clinical trials compared to conventional irradiation with photons, such as γ-rays or X-rays, owing to the unique characteristics of improved dose deposition and higher relative biological effectiveness (8, 9). Carbon ion irradiation is more efficient in inducing cell killing than X-ray irradiation (10). Multiple lines of evidence from vitro and in vivo studies have shown that conventional radiotherapy can enhance the formation of metastasizing cells (11–13). In contrast, heavy ion irradiation has mostly been found to suppress the migratory and invasive potential of cancer cells (14–17). Our previous data also demonstrated that cell motility was more suppressed after carbon ion irradiation than after X-ray irradiation in glioma cells (18), lung cancer cells (19), and tongue squamous cell carcinoma (TSCC) (20).
Focal adhesion kinase (FAK) is frequently overexpressed in various tumors and is a crucial signaling component that is activated by numerous stimuli and functions as a biosensor or integrator for regulating cell motility, adhesion, and growth (21). FAK amplification in OSCC was reported to correlate with lymph node metastasis (22). Knockdown of FAK has been found to inhibit the survival, invasion, and metastasis of oral cancer (2, 23). Hence, the control of growth and metastatic processes will lead to promising therapies for the clinical treatment of OSCC by targeting FAK.
This study aimed to unravel the influence and possible mechanisms of carbon ion irradiation on metastatic potential in TSCC, one of the most common oral cancers (24). Moreover, we explored the contribution of FAK signaling as a modulator of behavior in cancer cells receiving carbon ion irradiation.
Materials and Methods
Clinical Data Collection and Processing
The genomic and clinical data of squamous cell carcinoma of the patients with oral cancer were extracted from head and neck squamous cell carcinoma data in The Cancer Genome Atlas (TCGA) database (http://xena.ucsc.edu/). The TCGA dataset included 124 primary tumors samples and 13 normal samples. To analyze the relationship between FAK and metastasis in oral TSCC, we extracted stage I (n = 9) and IV (n = 47) cancer samples from clinical data. The area of the receiver operating characteristic curve (AUC) represents the performance of each gene.
Cell Culture and Treatment
The human TSCC cell line (CAL27) was purchased from BeNa Culture Collection (BNCC, Beijing, China). Lentiviral particles designed to silence human FAK (5-GATAGTGGACAGTCACAAA-3) and control lentiviral vectors were produced by Shanghai GeneChem Co. Ltd., China.
Carbon ion irradiation was conducted at the Heavy Ion Research Facility, Lanzhou of the Institute of Modern Physics, Chinese Academy of Sciences, using an 80 MeV/u carbon ion beam, with an LET 50 Kev/μm (18, 25).
Colony Formation Assay
We measured the colony forming ability of the irradiated CAL27 cells with or without FAK modification. The fixed colonies with chilled methanol were stained with 0.4% crystal violet (Sigma-Aldrich). Colonies of >50 cells were used to analyze the cloning efficiency.
Apoptosis Analysis by Flow Cytometry
Apoptosis was detected in irradiated CAL27 cells with or without FAK downregulation using a commercial kit (BD Biosciences, San Jose, CA, USA) according to the manufacturer's protocol. The apoptotic population was measured using a Flowsight imaging flow cytometer (Amnis/Merck Millipore, Darmstadt, Germany).
Wound Healing Assay
We determined the migratory ability via wound assays using IBIDI culture-inserts (ibidi, Martinsired, Germany). The cells at the logarithmic growth stage were incubated at 37°C in a humidified atmosphere containing 5% CO2. When the cells were adherent to the wall in a single layer, a circular wound was scratched using a sterile 200 μL pipette tip. Cells migrated into the wounded area, and photographs were captured immediately (0 h) and at 6, 12, 24, and 48 h using an optical microscope. The wound area and migration velocity were analyzed using ImageJ software (National Institute of Health, USA).
Transwell Assay
Cell invasion was assessed using BD Matrigel invasion chambers (BD Biosciences). Following FAK silencing and/or irradiation, CAL27 cells were seeded into the upper culture compartments supplemented with serum-free medium. The lower culture compartments were filled with DMEM containing 10% fetal bovine serum. Cells that invaded through the pores were fixed and stained with crystal violet after 24 h incubation.
AFM for Imaging and Mechanical Measurements
Single-cell topographical and mechanical characteristics of living CAL27 cells were measured by a AFM nano-indentation method (JPK Instruments AG, Germany), as described previously (26, 27). Adhesion energy in the cytoplasmic regions was obtained using the JPK data processing software (Version spm-4.2.50, JPK, Germany) (28, 29).
Immunofluorescence/Immunohistochemical Staining Assay
For F-actin staining, the fixed cells were stained with fluorescein isothiocyanate-phalloidin (Sigma-Aldrich, St. Louis, MO). The slides were mounted in VECTASHIELD with DAPI (Vector Laboratories, Burlingame, CA) and were viewed using a confocal microscope. The cytoskeleton obtained by immunofluorescence staining can be extracted using ImageJ software (NIH, USA), and the cytoskeleton structure was lined and analyzed. The cytoskeleton was marked with different colors according to the complexity of its connections, and the complexity of the cytoskeleton can be determined according to the distribution of colors.
Tissue microarray chips containing 20 tissues of TSCC were obtained from Shanghai Biochip Co., Ltd (HOraC060PG01 and Horac080PG01, and the ID of ethics approval was T20-0361). FAK expression was detected in cancer and juxtacancerous tissue (JCT) using immunohistochemical staining.
Western Blot Analysis
Protein samples were extracted from cells in RIPA buffer (Solarbio, Beijing, China) and analyzed in cells 24 h after irradiation with or without FAK knockdown. Total protein samples were blotted with the following antibodies: anti-FAK, anti-phospho-FAK (Y397), anti-phospho-paxillin (Y118), and anti-β-actin (GeneTex, Irvine, CA).
Statistical Analysis
Three independent experiments were carried out to obtain the quantitative data. Statistical analysis was performed using SPSS 16.0 (SPSS Inc., Chicago, IL, USA). Comparisons between two groups were performed with one-way analysis of variance (ANOVA) followed by LSD post hoc test. A P-value of <0.05 was considered statistically significant.
Result
Screening and Validation of TSCC Biomarkers Based on Bioinformatics Analysis
Based on previous literature, 12 transcriptomic biomarkers that are OSCC diagnostic biomarkers were screened in our study (30). As displayed in Figure 1A, the diagnosis and classification of primary (N0) and metastatic (≥N1) tumors using receiver operating characteristic curves were tested. The results showed that MMP-1, DUSP1, and ITGA3 were downregulated in OSCC, with AUC values of 0.536, 0.527, and 0.599, respectively. Conversely, SATA1, CXCL8, ITGA4, EGFR, and PTK2/FAK were upregulated in OSCC. More importantly, EGFR and PTK2/FAK had the highest AUC value (0.715) among all genes, indicating that EGFR and PTK2/FAK could be suitable biomarker candidates associated with cancer progression.
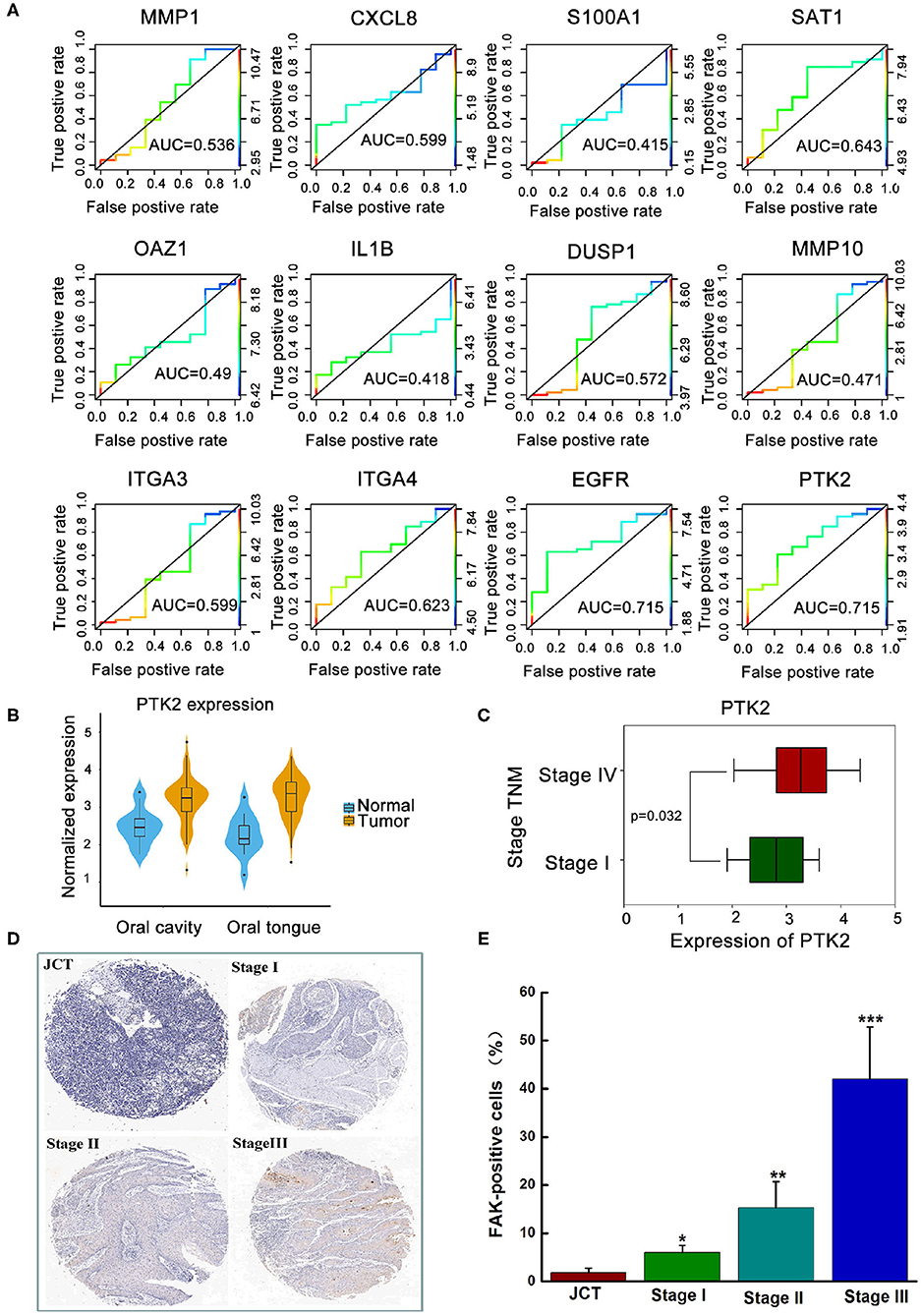
Figure 1. The clinical analysis between the expression of FAK and oral cancer progression. (A) Exploration of key genes involved in primary (N0) and metastatic (≥N1) tumors via the ROC curve test. (B) Higher expression of PTK2/FAK in the oral cavity or oral tongue cancer. (C) Difference between PTK2/FAK expression in stages I and IV tongue squamous cell carcinoma. (D) Detection of the expression of FAK in the tissue chip. (E) Quantitative analysis of FAK positive cell proportion. *P < 0.05, **P < 0.01, and ***P < 0.001 vs. the juxtacancerous tissue (JCT) group.
Considering the different locations of the primary oral tumor, Figure 1B shows a higher PTK2/FAK expression in oral cavity or oral tongue cancer. Boxplot analysis of stage I and IV samples indicated that PTK2/FAK was more prominently upregulated in stage IV than in stage I samples in the TSCC patients (P = 0.032, Figure 1C). Moreover, there was increased expression of FAK protein in the late stage of the tumor compared to the adjacent normal tissue in the TSCC patients using the tissue chip technique (Figures 1D,E). Taken together, these findings imply that PTK2/FAK is highly associated with the metastasis progression of TSCC patients.
Target Effects of FAK Signaling in TSCC Cells Exposed to Carbon Ion Irradiation
To test the contribution of FAK signaling in tumor inhibition during carbon ion radiation therapy, we knocked down FAK expression in CAL27 cells using lentivirus carrying FAK shRNA. Reduced expressions of FAK, FAK-pY397, and paxillin-pY118 were observed in the irradiated cells, FAK shRNA-infected cells, and cells treated with combined treatment compared to control shRNA-infected cells (Figure 2A). Moreover, a diminished trend was more prominent in the FAK shRNA combined with irradiation treatment group. Additionally, the proportion of apoptotic cells was up to 7.86, 13.51, and 20.13% in the FAK shRNA, irradiation, and FAK shRNA combined with irradiation groups, respectively, compared to the control shRNA group (1.11%) (Figure 2B and Supplementary Figure 3).
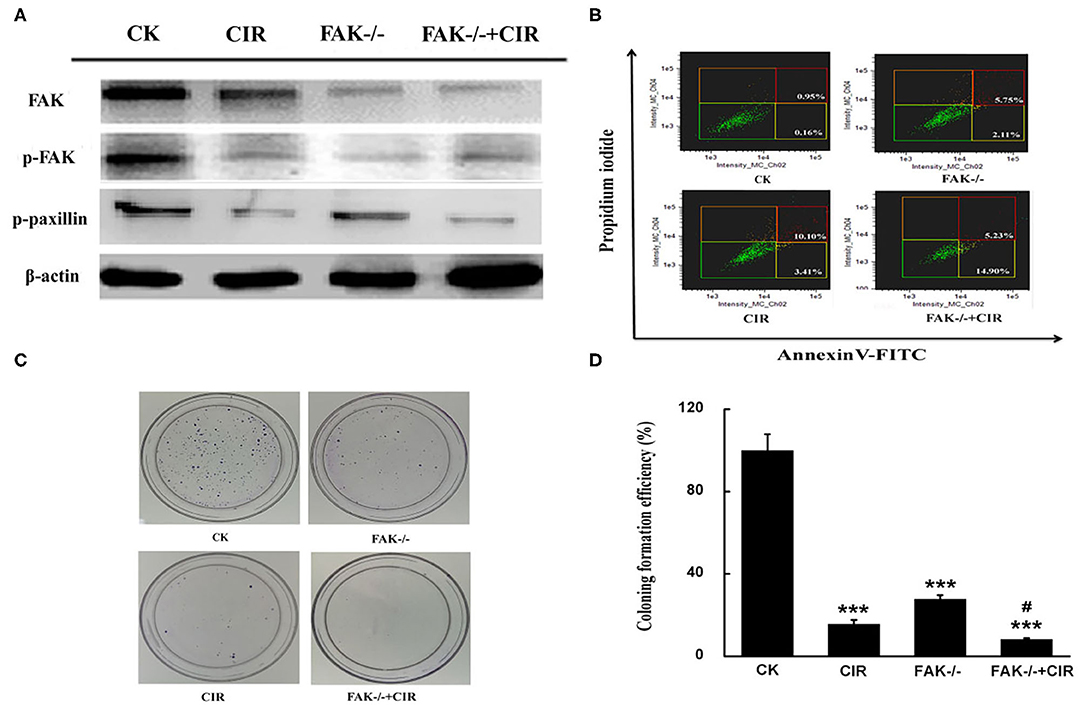
Figure 2. FAK depression is effective at inhibiting the growth of CAL27 cells. (A) Detection of FAK, FAK phosphorylation at Y397, and paxillin phosphorylation at Y118 expression. (B) Determination of the proportion of apoptotic cells by flow cytometry. (C,D) Representative images of the colony formation and quantitative evaluation of cell proliferation ability using clonogenic survival assays. The means ± SEM (N = 3) were calculated for each value. ***P < 0.001 vs. the control group. #P < 0.05 vs. the irradiation group.
To validate the long-term effect of cell growth, colony formation was evaluated in CAL27 cells exposed to carbon ions in the presence or absence of FAK. Compared to the irradiation alone treatment, the relative number of colonies formed by CAL27 cells after treatment with irradiation plus FAK knockdown was reduced by 47.2% (Figures 2C,D).
Alterations in Cellular Motility Abilities Induced by FAK Silencing and Carbon Ion Irradiation
The motility abilities of CAL27 cells following FAK deletion before carbon ion irradiation were evaluated via wound healing and Transwell invasion assays. The moving paths of circular wound recovery showed a decreased migration velocity of collective cells within 48 h in every treatment group compared to that in the shRNA-control group (Figure 3A). Moreover, the quantitative results of cells taken at 24 h after scratching indicated a markedly significant decline in the wound area by 44.6, 52.4, and 77.2% in irradiated, FAK-/-, and FAK-/- irradiated cells compared to that in control cells (Figure 3D). Further data revealed that a reduction in wound healing ability was significantly found in the FAK silencing and irradiation groups compared to the irradiation group (P < 0.01).
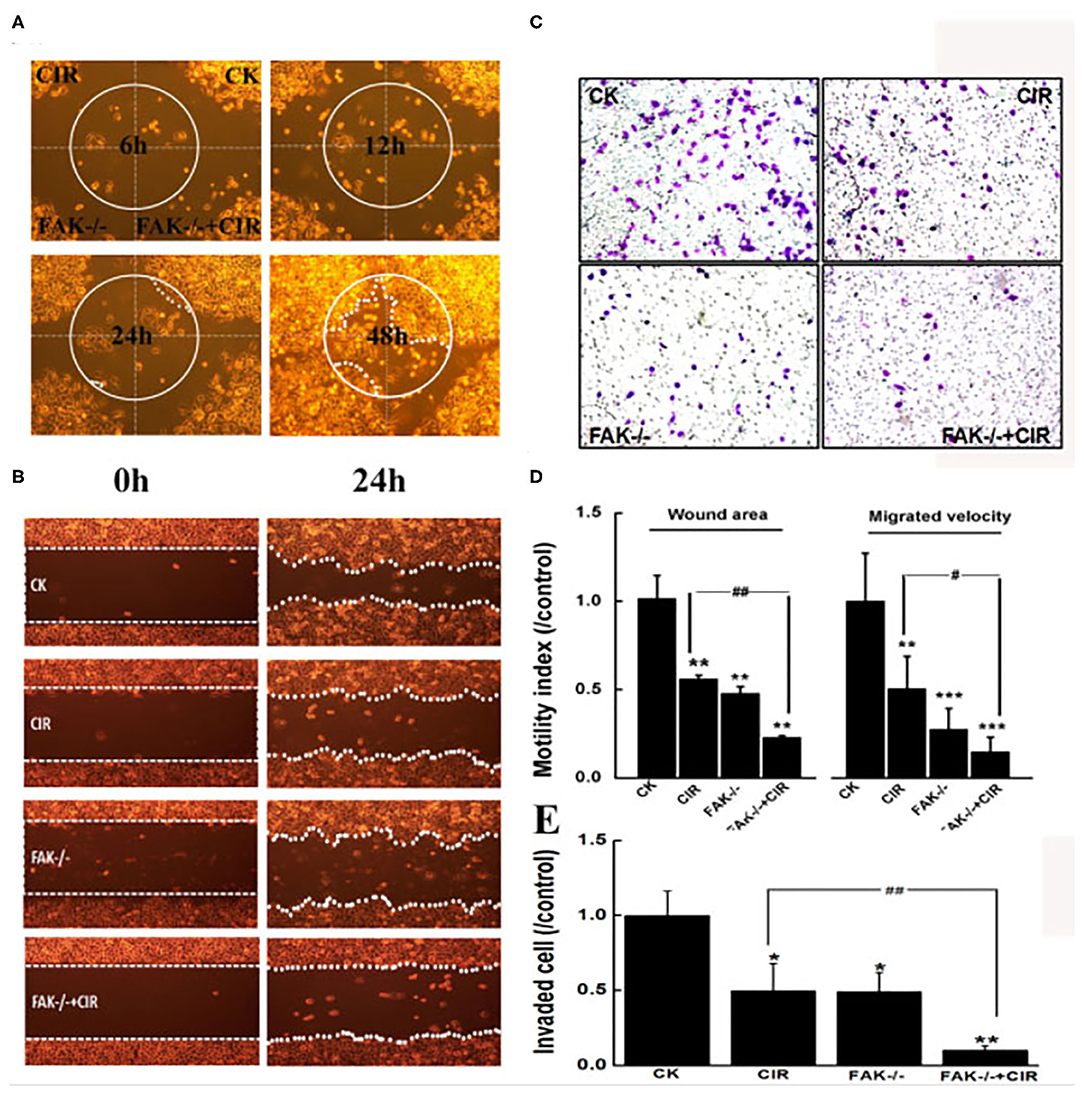
Figure 3. Inhibition of FAK reduces migration and invasion in CAL27 cells after carbon ion irradiation. (A) Monitoring of moving paths of the circular wound of cells up to 48 h. (B) Typical images of cells taken at 0 h and 24 h after scratching. (C) Representative images of cells invaded through the matrigel-coated membrane. (D) Quantitative analyses of cell migration signatures. (E) Quantitative evaluation of cell invasion ability. *P < 0.05, **P < 0.01, and ***P < 0.001 compared to the control group. #P < 0.05 and ##P < 0.01 vs. the irradiation group.
The Transwell invasion assay showed that the invasiveness capacity of CAL27 cells in irradiated cells (P < 0.05), FAK shRNA-infected cells (P < 0.05), and cells treated with FAK shRNA combined with irradiation (P < 0.01) was remarkably lower than that of control cells (Figure 3C). The number of invaded cells subjected to irradiation alone was 4.83-fold higher than that of cells treated with the combination of FAK shRNA infection and carbon ion irradiation (Figure 3E).
Modulation of Cytoskeletal Rearrangement and Biomechanical Properties via FAK Downregulation
As shown in Figure 4A and immunofluorescence staining in Supplementary Figure 2, an unordered actin filament arrangement accompanied by decreased intensity of actin fibers was observed in CAL27 cells with different treatments. In particular, actin staining exhibited an obvious ring-like distribution around the membrane protrusion structures of cells in the combination treatment group with FAK inhibition and irradiation. Moreover, in contrast to the control cells, FAK inhibition remarkably disrupted the formation of lamellipodia, filopodia, and membrane protrusions in the irradiated cells. Moreover, the abundance of the extracted cytoskeleton decreased in all treatment groups compared to that in the control group, but this trend was not significant.
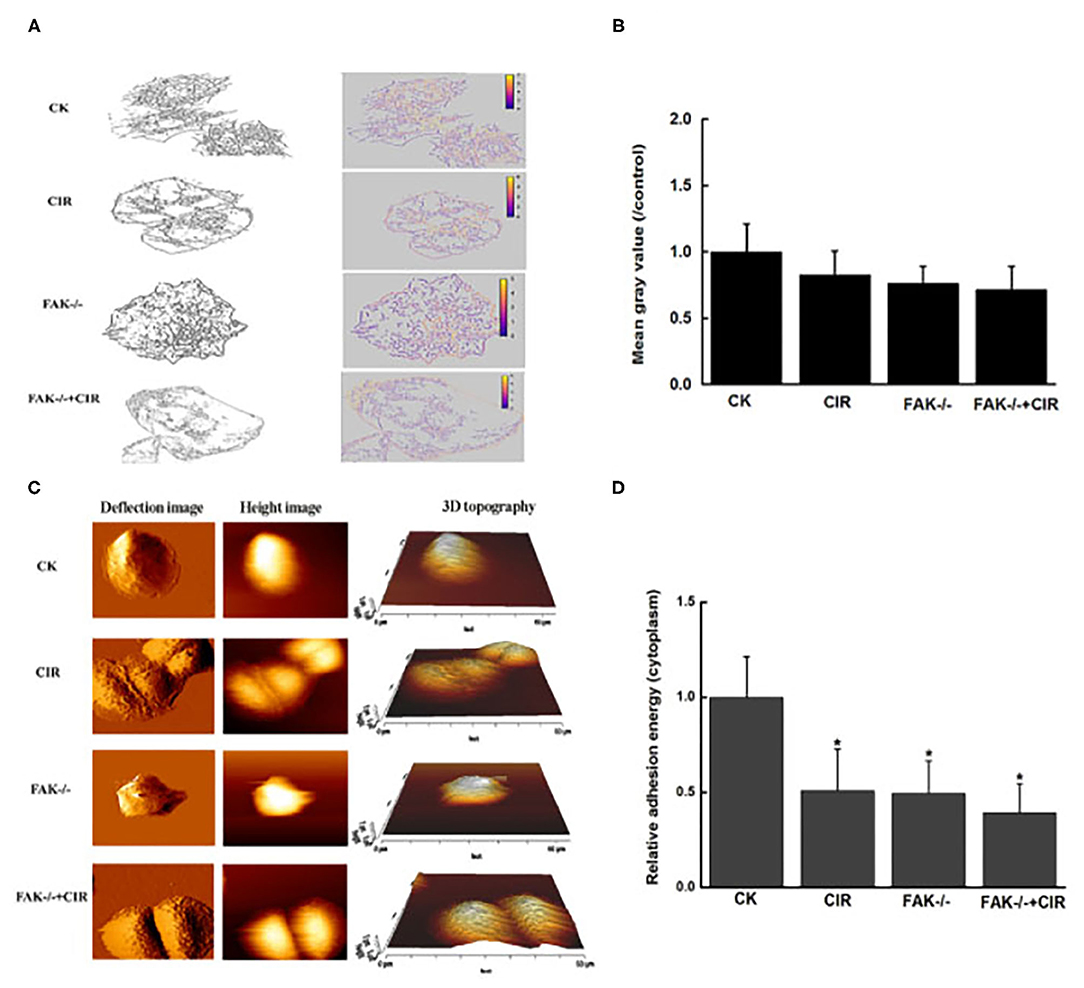
Figure 4. Modulation of cytoskeletal structure and cellular adhesion after treatment with carbon ion irradiation in the presence or absence of FAK activity. (A) Characteristic extraction of cytoskeletal structure. (B) Measurement of the relative mean gray value of cells in images. (C) Representative examples of vertical deflection, height, and 3D images in a single cell. (D) Quantitative analysis of adhesion energy in the cytoplasmatic region. The mean ± SEM (N = 3) were calculated for each value. *P < 0.05 vs. the control group.
The topography and deflection images are displayed in Figure 4C. Compared to the control group, there was a relatively rough plasma membrane in the combined treatment group. At this point, the variations in cytoplasmic adhesion energy from force-displacement curves acquired by the AFM indentations were decreased in the irradiated cells, FAK shRNA-infected cells, and cells treated with FAK shRNA combined with irradiation compared to the control cells (Figure 4D).
Discussion
More aggression is found in TSCC than in other forms of OSCC because of its propensity for rapid local invasion and spread (31). As shown in Supplementary Figure 1, from the TCGA database, FAK/PTK2 was identified as one of the valuable diagnostic biomarkers in OSCC. Our data from nine patients with stage I TSCC (N0) and 47 patients with stage IV TSCC (≥N1) using public databases showed that genomic alteration of FAK closely modulated the malignant progression of TSSC, including histological differentiation, TNM stage, and lymph node metastasis (Figure 1). FAK is a critical non-receptor tyrosine kinase involved in many aspects of the metastatic process, including adhesion, migration, and invasion (32). Therefore, FAK could be a more suitable candidate for predicting the metastatic status of TSSCs than other existing biomarkers. Here, we propose that targeting FAK may present a feasible approach for improving the efficacy of radiotherapy for TSCC.
In previous studies, carbon ion irradiation as a promising therapy has been proven to efficiently induce cell death in X-ray-resistant OSCC by modulating diverse signaling molecules, such as AKT and SPHK1 (33, 34). Analogously, FAK has been reported to exert an anti-apoptotic action against ionizing radiation in HL-60 cells by inhibiting the mitochondrial apoptosis pathway (35). Our data showed that carbon ion irradiation remarkably repressed the expression of FAK and phosphorylation of FAK on Tyr397 and paxillin on Tyr118 in CAL27 cells. Furthermore, when cells were exposed to the combination of FAK downregulation by shRNA and irradiation, the clonogenic formation assay revealed a strong inhibitory effect on cell survival, along with a higher proportion of apoptotic cells (Figure 2). In agreement with previous findings, glioblastoma (36), colon cancer (37), and head and neck cancer cells (38) showed enhanced cell-killing effects in response to ionizing radiation by FAK deletion.
The activation of the FAK-paxillin signaling pathway has been considered a crucial index for tumor metastasis (39). In the current study, carbon ions distinctly limited the area and speed of wound healing cell migration as well as the number of invading cells through the invasion chamber (Figure 3). It is worth noting that, after FAK knockdown, the migratory and invasive abilities of CAL27 cells were more prominently inhibited when compared to cells treated with radiation alone. Here, the precise role of the combination of FAK knockdown and carbon ion irradiation was confirmed to suppress the high-motility capability of the TSCC cell line CAL27. Moreover, our findings suggest that FAK activity helps to elucidate the molecular mechanisms underlying motility reduction caused by carbon ion beams in TSCC. In this regard, previous findings have also demonstrated that heavy ion radiotherapy is more effective than conventional photon beam therapy in preventing metastasis in preclinical studies (40).
To provide further evidence for FAK knockdown-mediated cell motility repression, alterations in cytoskeletal structure and cell adhesion were determined in irradiated CAL27 cells. Cell movement relies on changes in the dynamics of actin filaments (41). Figure 4 shows that a certain decrease in the mean actin density was observed in each treatment group. However, as expected, as shown in Supplementary Figure 2, obvious disorganization of actin distribution and reduction of cell protrusion occurred in the combined FAK shRNA plus irradiation group, indicating that there is a lack of cell polarization and can limit cell motility potential. Furthermore, compared to control cells, FAK silencing resulted in reduced adhesion energy to the substrates in the irradiated cells, FAK shRNA-infected cells, or cells treated with FAK shRNA combined with irradiation, which was consistent with changes in cell motility and phosphorylation of adhesion proteins.
This study demonstrated that carbon ion irradiation and FAK inhibition, especially their combined application, can effectively inhibit OSCC, providing a basis for further in vivo experiments and clinical trials.
In addition, the detection of cytoskeletal structure and cell adhesion illustrated the relationship between their variation and cell motor ability. In future studies, we will further study the effects of heavy ions and FAK on cell properties, such as shape, elasticity, rigidity, and viscosity, as well as the relationship between cell properties and their migration and invasion ability, to find a more appropriate way to inhibit the metastasis of OSCC.
Conclusion
Mechanistically, we have provided evidence that carbon ion irradiation significantly blocked the FAK-related signal pathway, which partially explains the anti-tumor mechanisms of carbon ions. Furthermore, combining FAK downregulation with carbon ion irradiation could synergistically offer comparable therapeutic benefits for TSCC patients regarding the inhibition of metastatic potential.
Data Availability Statement
The raw data supporting the conclusions of this article will be made available by the authors, without undue reservation.
Ethics Statement
The studies involving human participants were reviewed and approved by Tissue microarray chip containing oral squamous cell carcinoma were obtained from Shanghai Biochip Co. The ID of ethics approval is T20-0361, submitted in this system. The patients/participants provided their written informed consent to participate in this study.
Author Contributions
QS: designed the experiment, executed the research and wrote the original draft. QY, ZB, RF, and XH: executed the research and interpreted the data. BL and JW: discussed reviewed the manuscript. XA and YL: acquired the data, analyzed the data and interpreted the data, and reviewed the manuscript. All authors contributed to the article and approved the submitted version.
Funding
This study was supported by grants from Talent Innovation and Entrepreneurship Project of Lanzhou City (2018-RC-36), Clinical Research Center of Shanxi Province for Dental and Maxillofacial Diseases, College of Stomatology, Xi'an Jiaotong University (2019YHJB10), and Natural Science Foundation of Gansu Province (20JR5RA264).
Conflict of Interest
The authors declare that the research was conducted in the absence of any commercial or financial relationships that could be construed as a potential conflict of interest.
Supplementary Material
The Supplementary Material for this article can be found online at: https://www.frontiersin.org/articles/10.3389/fpubh.2021.631118/full#supplementary-material
Abbreviations
AFM, Atomic Force Microscope; CK, control check; CIR, carbon ion radiotherapy; FAK, focal adhesion kinase; JCT, juxtacancerous tissue; OSCC, oral squamous cell carcinoma; PORT, postoperative radiation therapy; PTK2, protein tyrosine kinase 2; TSCC, tongue squamous cell carcinoma.
References
1. Hsing EW, Shiah SG, Peng HY, Chen YW, Chuu CP, Hsiao JR, et al. TNF-alpha-induced miR-450a mediates TMEM182 expression to promote oral squamous cell carcinoma motility. PLoS ONE. (2019) 14:e0213463. doi: 10.1371/journal.pone.0213463
2. Xi WH, Yang LY, Cao ZY, Qian Y. Tivantinib (ARQ-197) exhibits anti-tumor activity with down-regulation of FAK in oral squamous cell carcinoma. Biochem Biophys Res Commun. (2015) 457:723–9. doi: 10.1016/j.bbrc.2015.01.062
3. Almangush A, Heikkinen I, Makitie AA, Coletta RD, Laara E, Leivo I, et al. Prognostic biomarkers for oral tongue squamous cell carcinoma: a systematic review and meta-analysis. Br J Cancer. (2017) 117:856–66. doi: 10.1038/bjc.2017.244
4. van Dijk BA, Brands MT, Geurts SM, Merkx MA, Roodenburg JL. Trends in oral cavity cancer incidence, mortality, survival and treatment in the Netherlands. Int J Cancer. (2016) 139:574–83. doi: 10.1002/ijc.30107
5. Yen CJ, Tsou HH, Hsieh CY, Chu CY, Chiu CF, Chen CC, et al. Sequential therapy of neoadjuvant biochemotherapy with cetuximab, paclitaxel, and cisplatin followed by cetuximab-based concurrent bioradiotherapy in high-risk locally advanced oral squamous cell carcinoma: Final analysis of a phase 2 clinical trial. Head Neck. (2019) 41:1703–12. doi: 10.1002/hed.25640
6. Garzino-Demo P, Dell'Acqua A, Dalmasso P, Fasolis M, La Terra Maggiore GM, Ramieri G, et al. Clinicopathological parameters and outcome of 245 patients operated for oral squamous cell carcinoma. J Craniomaxillofac Surg. (2006) 34:344–50. doi: 10.1016/j.jcms.2006.04.004
7. Hosni A, Huang SH, Chiu K, Xu W, Su J, Bayley A, et al. Predictors of early recurrence prior to planned postoperative radiation therapy for oral cavity squamous cell carcinoma and outcomes following salvage intensified radiation therapy. Int J Radiat Oncol Biol Phys. (2019) 103:363–73. doi: 10.1016/j.ijrobp.2018.09.013
8. Jin X, Li F, Liu B, Zheng X, Li H, Ye F, et al. Different mitochondrial fragmentation after irradiation with X-rays and carbon ions in HeLa cells and its influence on cellular apoptosis. Biochem Biophys Res Commun. (2018) 500:958–65. doi: 10.1016/j.bbrc.2018.04.214
9. Wang Y, Guan H, Xie DF, Xie Y, Liu XD, Wang Q, et al. Proteomic analysis implicates dominant alterations of RNA metabolism and the proteasome pathway in the cellular response to carbon-ion irradiation. PLoS ONE. (2016) 11:e0163896. Epub 2016/10/07. doi: 10.1371/journal.pone.0163896
10. Sato K, Azuma R, Imai T, Shimokawa T. Enhancement of mTOR signaling contributes to acquired X-ray and C-ion resistance in mouse squamous carcinoma cell line. Cancer Sci. (2017) 108:2004–10. doi: 10.1111/cas.13323
11. Blyth BJ, Cole AJ, MacManus MP, Martin OA. Radiation therapy-induced metastasis: radiobiology and clinical implications. Clin Exp Metastas. (2018) 35:223–36. doi: 10.1007/s10585-017-9867-5
12. Moncharmont C, Levy A, Guy JB, Falk AT, Guilbert M, Trone JC, et al. Radiation-enhanced cell migration/invasion process: a review. Crit Rev Oncol Hematol. (2014) 92:133–42. doi: 10.1016/j.critrevonc.2014.05.006
13. Vilalta M, Rafat M, Graves EE. Effects of radiation on metastasis and tumor cell migration. Cell Mol Life Sci. (2016) 73:2999–3007. doi: 10.1007/s00018-016-2210-5
14. Konings K, Vandevoorde C, Belmans N, Vermeesen R, Baselet B, Walleghem MV, et al. The combination of particle irradiation with the hedgehog inhibitor GANT61 differently modulates the radiosensitivity and migration of cancer cells compared to X-ray irradiation. Front Oncol. (2019) 9:391. doi: 10.3389/fonc.2019.00391
15. Moncharmont C, Guy JB, Wozny AS, Gilormini M, Battiston-Montagne P, Ardail D, et al. Carbon ion irradiation withstands cancer stem cells' migration/invasion process in Head and Neck Squamous Cell Carcinoma (HNSCC). Oncotarget. (2016) 7:47738–49. doi: 10.18632/oncotarget.10281
16. Stahler C, Roth J, Cordes N, Taucher-Scholz G, Mueller-Klieser W. Impact of carbon ion irradiation on epidermal growth factor receptor signaling and glioma cell migration in comparison to conventional photon irradiation. Int J Radiat Biol. (2013) 89:454–61. doi: 10.3109/09553002.2013.766769
17. Wozny AS, Vares G, Alphonse G, Lauret A, Monini C, Magne N, et al. ROS production and distribution: a new paradigm to explain the differential effects of X-ray and carbon ion irradiation on cancer stem cell migration and invasion. Cancers. (2019) 11:4. doi: 10.3390/cancers11040468
18. Liu Y, Liu Y, Sun C, Gan L, Zhang L, Mao A, et al. Carbon ion radiation inhibits glioma and endothelial cell migration induced by secreted VEGF. PLoS ONE. (2014) 9:e98448. doi: 10.1371/journal.pone.0098448
19. Liu Y, Liu Y, Zhang H, Sun C, Zhao Q, Di C, et al. Effects of carbon-ion beam irradiation on the angiogenic response in lung adenocarcinoma A549 cells. Cell Biol Int. (2014) 38:1304–10. doi: 10.1002/cbin.10327
20. Zheng Q, Liu Y, Zhou HJ, Du YT, Zhang BP, Zhang J, et al. X-ray radiation promotes the metastatic potential of tongue squamous cell carcinoma cells via modulation of biomechanical and cytoskeletal properties. Hum Exp Toxicol. (2015) 34:894–903. doi: 10.1177/0960327114561664
21. Mitra SK, Hanson DA, Schlaepfer DD. Focal adhesion kinase: in command and control of cell motility. Nat Rev Mol Cell Biol. (2005) 6:56–68. doi: 10.1038/nrm1549
22. de Vicente JC, Rosado P, Lequerica-Fernandez P, Allonca E, Villallain L, Hernandez-Vallejo G. Focal adhesion kinase overexpression: correlation with lymph node metastasis and shorter survival in oral squamous cell carcinoma. Head Neck. (2013) 35:826–30. doi: 10.1002/hed.23038
23. Xiao W, Jiang M, Li H, Li C, Su R, Huang K. Knockdown of FAK inhibits the invasion and metastasis of Tca8113 cells in vitro. Mol Med Rep. (2013) 8:703–7. doi: 10.3892/mmr.2013.1555
24. Camisasca DR, Honorato J, Bernardo V, da Silva LE, da Fonseca EC, de Faria PAS, et al. Expression of Bcl-2 family proteins and associated clinicopathologic factors predict survival outcome in patients with oral squamous cell carcinoma. Oral Oncology. (2009) 45:225–33. doi: 10.1016/j.oraloncology.2008.05.021
25. Fushimi K, Uzawa K, Ishigami T, Yamamoto N, Kawata T, Shibahara T, et al. Susceptible genes and molecular pathways related to heavy ion irradiation in oral squamous cell carcinoma cells. Radiother Oncol. (2008) 89:237–44. doi: 10.1016/j.radonc.2008.04.015
26. Dufrene YF, Ando T, Garcia R, Alsteens D, Martinez-Martin D, Engel A, et al. Imaging modes of atomic force microscopy for application in molecular and cell biology. Nat Nanotechnol. (2017) 12:295–307. doi: 10.1038/nnano.2017.45
27. Li QS, Lee GY, Ong CN, Lim CT. AFM indentation study of breast cancer cells. Biochem Biophys Res Commun. (2008) 374:609–13. doi: 10.1016/j.bbrc.2008.07.078
28. Trache A, Xie L, Huang H, Glinsky VV, Meininger GA. Applications of atomic force microscopy for adhesion force measurements in mechanotransduction. Methods Mol Biol. (2018) 1814:515–28. doi: 10.1007/978-1-4939-8591-3_30
29. Zhang BP, Li L, Li ZQ, Liu Y, Zhang H, Wang JZ. Carbon ion-irradiated hepatoma cells exhibit coupling interplay between apoptotic signaling and morphological and mechanical remodeling. Sci Rep-Uk. (2016) 6:35131. doi: 10.1038/srep35131
30. Yakob M, Fuentes L, Wang MB, Abemayor E, Wong DT. Salivary biomarkers for detection of oral squamous cell carcinoma - current state and recent advances. Curr Oral Health Rep. (2014) 1:133–41. doi: 10.1007/s40496-014-0014-y
31. Liu X, Wang A, Lo Muzio L, Kolokythas A, Sheng S, Rubini C, et al. Deregulation of manganese superoxide dismutase (SOD2) expression and lymph node metastasis in tongue squamous cell carcinoma. BMC Cancer. (2010) 10:365. doi: 10.1186/1471-2407-10-365
32. Sparano A, Quesnelle KM, Kumar MS, Wang Y, Sylvester AJ, Feldman M, et al. Genome-wide profiling of oral squamous cell carcinoma by array-based comparative genomic hybridization. Laryngoscope. (2006) 116:735–41. doi: 10.1097/01.mlg.0000205141.54471.7f
33. Higo M, Uzawa K, Kawata T, Kato Y, Kouzu Y, Yamamoto N, et al. Enhancement of SPHK1 in vitro by carbon ion irradiation in oral squamous cell carcinoma. Int J Radiat Oncol Biol Phys. (2006) 65:867–75. doi: 10.1016/j.ijrobp.2006.02.048
34. Takahashi A, Ma H, Nakagawa A, Yoshida Y, Kanai T, Ohno T, et al. Carbon-ion beams efficiently induce cell killing in X-ray resistant human squamous tongue cancer cells. Int J Med. (2014) 3:133–42. doi: 10.4236/ijmpcero.2014.33019
35. Storch K, Sagerer A, Cordes N. Cytotoxic and radiosensitizing effects of FAK targeting in human glioblastoma cells in vitro. Oncol Rep. (2015) 33:2009–16. doi: 10.3892/or.2015.3753
36. Luo CW, Wu CC, Ch'ang HJ. Radiation sensitization of tumor cells induced by shear stress: the roles of integrins and FAK. Biochim Biophys Acta. (2014) 1843:2129–37. doi: 10.1016/j.bbamcr.2014.06.007
37. Skinner HD, Giri U, Yang L, Woo SH, Story MD, Pickering CR, et al. Proteomic profiling identifies PTK2/FAK as a driver of radioresistance in hpv-negative head and neck cancer. Clin Cancer Res. (2016) 22:4643–50. doi: 10.1158/1078-0432.CCR-15-2785
38. Kasahara T, Koguchi E, Funakoshi M, Aizu-Yokota E, Sonoda Y. Antiapoptotic action of focal adhesion kinase (FAK) against ionizing radiation. Antioxid Redox Signal. (2002) 4:491–9. doi: 10.1089/15230860260196290
39. Chen C, Wang Y, Chen S, Ruan X, Liao H, Zhang Y, et al. Genistein inhibits migration and invasion of cervical cancer HeLa cells by regulating FAK-paxillin and MAPK signaling pathways. Taiwan J Obstet Gynecol. (2020) 59:403–8. doi: 10.1016/j.tjog.2020.03.012
40. Ogata T, Teshima T, Kagawa K, Hishikawa Y, Takahashi Y, Kawaguchi A, et al. Particle irradiation suppresses metastatic potential of cancer cells. Cancer Res. (2005) 65:113–20. Available online at: https://cancerres.aacrjournals.org/content/65/1/113
Keywords: carbon ion irradiation, FAK, metastatic potential, tongue squamous cell carcinoma, cell growth
Citation: Si Q, Ye Q, Bing Z, Fan R, Hu X, Liu B, Wang J, Liu Y and An X (2021) Carbon Ion Irradiation Enhances the Anti-tumor Efficiency in Tongue Squamous Cell Carcinoma via Modulating the FAK Signaling. Front. Public Health 9:631118. doi: 10.3389/fpubh.2021.631118
Received: 19 November 2020; Accepted: 05 January 2021;
Published: 03 February 2021.
Edited by:
Lu Cai, University of Louisville, United StatesReviewed by:
Xin Duan, Cincinnati Children's Hospital Medical Center, United StatesQiang Liu, Chinese Academy of Medical Sciences and Peking Union Medical College, China
Copyright © 2021 Si, Ye, Bing, Fan, Hu, Liu, Wang, Liu and An. This is an open-access article distributed under the terms of the Creative Commons Attribution License (CC BY). The use, distribution or reproduction in other forums is permitted, provided the original author(s) and the copyright owner(s) are credited and that the original publication in this journal is cited, in accordance with accepted academic practice. No use, distribution or reproduction is permitted which does not comply with these terms.
*Correspondence: Yang Liu, bGl1eUBpbXBjYXMuYWMuY24=; Xiaoli An, YW54bEBsenUuZWR1LmNu