- 1Anhui Center for Disease Control and Prevention, Public Health Research Institute of Anhui Province, Hefei, China
- 2Department of Occupational and Environmental Health, School of Public Health, Capital Medical University, Beijing, China
- 3Beijing Key Laboratory of Environmental Toxicology, School of Public Health, Capital Medical University, Beijing, China
- 4Chinese Center for Disease Control and Prevention, National Institute for Occupational Health and Poison Control, Beijing, China
- 5National Center for Chronic and Non-communicable Disease Control and Prevention, Chinese Center for Disease Control and Prevention, Beijing, China
Background: Children are vulnerable to the respiratory effects of air pollution, and their lung function has been associated with long-term exposure to low air pollution level in developed countries. However, the impact of contemporary air pollution level in developing countries as a result of recent efforts to improve air quality on children's lung function is less understood.
Methods: We obtained a cross-sectional sample of 617 schoolchildren living in three differently polluted areas in Anhui province, China. 2-year average concentrations of air pollutants at the year of spirometry and the previous year (2017–2018) obtained from district-level air monitoring stations were used to characterize long-term exposure. Forced vital capacity (FVC), forced expiratory volume in 1 second (FEV1), and forced expiratory flow between 25 and 75% of FVC (FEF25−75) were determined under strict quality control. Multivariable regression was employed to evaluate the associations between air pollution level and lung function parameters, overall and by demographic characteristics, lifestyle, and vitamin D that was determined by liquid chromatography tandem mass spectrometry.
Results: Mean concentration of fine particulate matter was 44.7 μg/m3, which is slightly above the interim target 1 standard of the World Health Organization. After adjusting for confounders, FVC, FEV1, and FEF25−75 showed inverse trends with increasing air pollution levels, with children in high exposure group exhibiting 87.9 [95% confidence interval (CI): 9.5, 166.4] mL decrement in FEV1 and 195.3 (95% CI: 30.5, 360.1) mL/s decrement in FEF25−75 compared with those in low exposure group. Additionally, the above negative associations were more pronounced among those who were younger, girls, not exposed to secondhand smoke, non-overweight, physically inactive, or vitamin D deficient.
Conclusions: Our study suggests that long-term exposure to relatively high air pollution was associated with impaired lung function in children. More stringent pollution control measures and intervention strategies accounting for effect modification are needed for vulnerable populations in China and other developing countries.
Introduction
Air pollution is ubiquitous and poses a significant threat to global health. Ambient pollutants get into the body mainly through breathing, with the potential of irritating airways and penetrating deeply into the lung, making respiratory system the primary target of adverse effects. According to the Global Burden of Diseases Study 2019, air pollution ranked second among risk factors contributing to chronic respiratory diseases (1). As children have a higher ventilation rate and usually spend more time outdoors than adults, they are more prone to air pollutants-induced respiratory problems. Lung function is an objective measure of respiratory health and reduced lung function during childhood has clinical relevance with lung growth and later respiratory morbidity and mortality (2). Thus, it is vital to study the impact of air pollution on the lung function of children.
Compared with short-term exposure, studying long-term exposure to air pollution has more implications because of greater effect and less reversibility of lung function. Both cross-sectional and longitudinal studies have shown that long-term exposure to air pollution may affect lung function among children. For example, a multicenter study taking advantage of data from several European birth cohorts found that the annual average concentrations of fine particulate matter (PM2.5) and nitrogen dioxide (NO2) at the current address were negatively associated with forced vital capacity (FVC) and forced expiratory volume in 1 second (FEV1) (3). The Dutch population-based Prevention and Incidence of Asthma and Mite Allergy cohort study demonstrated that exposure to all pollutants during the preschool period was associated with reduced growth of FEV1 (4). However, most of the previous studies were conducted in developed countries with typically low pollution levels (e.g., PM2.5 below 20 μg/m3). Indeed, more than 90% of global children were exposed to levels of air pollution above the World Health Organization (WHO) air quality guideline (5). China is the world's largest developing country where the air pollution level is much higher than that in developed countries. A few multicity studies carried out between 2009 and 2012 have linked long-term exposure to high level of air pollution to the impaired lung function of Chinese children (6, 7). To tackle this severe environmental issue and protect public health, the central government of China released a 5-year action plan in 2013, aiming at improving air quality and reducing heavy pollution days. With the implementation of supportive clean air actions, the population-weighted mean PM2.5 concentration was estimated to decrease from 61.8 μg/m3 in 2013 to 42.0 μg/m3 in 2017 (8). Despite the remarkable reduction, few studies have examined the association between long-term air pollution exposure and children's lung function in the context of moderate air pollution. Additionally, understanding factors that modify the respiratory effects of air pollution is crucial for screening high-risk subpopulations and designing targeted invention measures. Available investigations mainly focused on sex and asthma status (9), although studies simultaneously exploring the modification effects of demographic characteristics, lifestyle, and micronutrients are needed.
To address these knowledge gaps, we examined the associations of long-term exposure to air pollution with a range of lung function parameters and if there is an exposure-response relationship in 617 schoolchildren who resided in three differently polluted areas in a less developed province, China, between 2017 and 2018. Furthermore, whether the above associations varied by age, sex, secondhand smoke exposure, body mass index (BMI), physical exercise, and serum vitamin D were also tested.
Methods
Study population
Three districts were, respectively chosen from three cities located in Anhui province, central China, based on historical air monitoring quality data and hereafter referred to as low, moderate, and high polluted areas. A multistage cluster sampling strategy was used to recruit schoolchildren from these polluted areas. In the first stage, one primary school and one secondary school were randomly selected within each district. In the second stage, one class was randomly selected from grades 1–6 of primary school and grades 7–8 of secondary school. In the final stage, 26 children were randomly selected from each selected class. Children were eligible if they were aged 6–15 years, had lived in the current address for at least 2 years, and showed no respiratory symptoms in the previous 14 days before spirometry. A total of 620 schoolchildren participated in this study between October 2017 to May 2018. Among these, three children had incomplete data on the questionnaire, resulting in 617 children being included in the final analysis. With this sample size, we have 80% power to detect a Cohen's f of 0.126 considering a type I error of 5%.
Data collection
According to the air quality data from environmental monitoring stations near to school, annual average concentrations of particulate matter with aerodynamic meter ≤10 μm (PM10), PM2.5, NO2, sulfur dioxide (SO2), ozone (O3), and carbon monoxide (CO) during 2017–2018 were calculated to characterize the long-term exposure levels of schoolchildren. In addition, 2-day (current day and 1 day before spirometry, lag01) moving average of PM2.5 concentration was calculated as a surrogate of short-term exposure.
Through face-to-face interviews, a standardized questionnaire was administered to all participants by experienced investigators to collect data on sociodemographic characteristics, lifestyle factors, self-reported chronic diseases, and medication history. Physical measurements including height and weight were also performed with participants in light clothing without shoes. Besides, 5 mL of venous blood and 50 mL of urine were obtained from each participant after an overnight fast of 8 h.
Secondhand smoke exposure was defined as exposure to passive tobacco smoking at least once per week. Children who got <180 min per week of moderate and/or vigorous activities were considered physically inactive. Vegetable and fruit intake was categorized as insufficient if less than four servings were consumed per day, otherwise as sufficient. Parental education was divided into two classes, high school or below and college or above. BMI was calculated by dividing weight (kg) by squares of height (m). Age- and sex-specific BMI z-score was further calculated based on WHO growth reference, and a BMI z-score equal to or above the corresponding 85th percentile was deemed as overweight/obesity (10).
Measurement of lung function
Lung function tests were performed by two trained technicians following the guidelines of the American Thoracic Society and European Respiratory Society (11). The child, standing and wearing a nose clip, was asked to blow at least three times into MasterScreen Pneumo spirometer (CareFusion, Germany) with a disposable mouthpiece. Acceptable reproducibility was obtained if the differences between the two highest FVC values and FEV1 values were both ≤150 mL (or within 5%). For children whose lung function results did not meet this standard, a maximum of eight blows were required. An expert panel conducted quality control on all reports of lung function tests, and calibration was done daily using a 3L syringe. FVC, FEV1, and forced expiratory flow between 25 and 75% of FVC (FEF25−75) were derived from the best curve. FEV1/FVC is the ratio of FEV1 to FVC. Moreover, the predicted values of FVC, FEV1, and FEF25−75 were calculated based on the 2012 Global Lung Function Initiative reference equation taking age, sex, and height into account (12). Poor lung function was defined as observed FVC <85% predicted value, FEV1 <85% predicted value, FEF25−75 <75% predicted value, and FEV1/FVC<85% according to relevant cutoff values applicable to children (13, 14).
Serum vitamin D detection
The serum was separated by centrifugation and stored at −80°C. Serum level of 25(OH)D (sum of 25(OH)D2 and D3) was detected by liquid chromatography tandem mass spectrometry (AB6500, U.S.). The reference standard was purchased from Sigma-Aldrich (U.S.) and other analytical grade chemical reagents were commercially available from Merck (Germany). In brief, 150 μL of serum was pipetted into a microtube and mixed with 15 μL internal standard (1,000 ng/mL). Then 150 μL aqueous zinc sulfate and 300 μL acetonitrile were added to precipitate protein, and the tube was vortexed for 30 s and left for 15 min at room temperature. After adding 750 μL hexane, the mixture was vortexed for 30 s and centrifugated at 13,000 rpm for 5 min. Following drying under nitrogen gas of 0.5 mL supernatant, the residue was reconstituted using 200 μL mobile phase for further analysis. All samples were performed in duplicate, and the coefficients of variation for intra- and inter-assay were <10%.
Statistical analysis
Differences between exposure groups were tested using analysis of variance for continuous variables and chi-square test for categorical variables. Multivariable linear regression models were used to examine the associations between long-term air pollution exposure levels and lung function parameters. The model was adjusted for age (continuous), sex (boys/girls), height (continuous), weight (continuous), secondhand smoke exposure (yes/no), physical activity (active/inactive), vegetable and fruit intake (sufficient/insufficient), and parental education (high school or below/college or above). Similar to previous studies that accounted for the confounding effect of short-term exposure (15, 16), PM2.5 concentration on lag01 days of lung function measurement was also included as a covariate. When testing the linear trends of lung function parameters with increasing air pollution levels, the exposure group was modeled as an ordinal variable. For associations with the prevalence of poor lung function, multivariable logistic regression models were employed with the same covariates.
Effect modification of the above associations was further evaluated through subgroup analyses stratified by age, sex, secondhand smoke exposure, BMI, physical activity, and serum vitamin D. To this end, age was dichotomized as 11 years or younger and 12 or older, and vitamin D was also dichotomized with its median (i.e., 16.6 ng/mL) as a cutoff value. For a more straightforward comparison between subgroups, marginally adjusted means or probability was computed for all combinations of exposure group and stratified variable, which are weighted averages reflecting confounder distribution in the total population (17). To respect 0 and 1 bounds, the predicted probability and its confidence interval (CI) were first generated on the logit scale and then transformed back to the probability scale. The interaction was formally tested by introducing a product term between exposure group and modifier, and a Wald test evaluating the joint significance of all associated cross-product terms was used to assess statistical significance. Furthermore, the joint impacts of air pollution and secondhand smoke on lung function were investigated by classifying the participants into six groups according to air pollution levels and secondhand smoke exposure status, with schoolchildren in the low exposure group and not exposed to secondhand smoke as reference.
Several sensitivity analyses were conducted as follows: (a) using percent predicted values instead of observed values of lung function parameters as dependent variables; (b) excluding children exposed to secondhand smoke; (c) excluding children with a self-reported diagnosis of asthma; (d) additionally adjusted for short-term NO2 or O3.
All analyses were done with R (version 3.6.0) and a P-value < 0.05 was considered statistically significant. Mean differences and odds ratio (OR) and their 95% CI were reported for results of linear and logistic regression models, respectively.
Results
Supplementary Figure S1 displays the geographic locations of six selected schools in Anhui province, China. The 2-year mean concentrations of PM10, PM2.5, NO2, SO2, O3, and CO were 72.4 μg/m3 [standard deviation (SD) = 42.7], 44.7 μg/m3 (SD = 33.3), 31.2 μg/m3 (SD = 19.0), 12.5 μg/m3 (SD = 5.6), 94.1 μg/m3 (SD = 45.9), and 0.8 mg/m3 (SD = 0.3), respectively (Table 1). While the concentrations of PM10, PM2.5, and O3 increased from low exposure group to high exposure group, the concentration of NO2 was highest in moderate group. Considering that NO2 is an indicator of vehicle exhaust, this discrepancy may be explained by more urbanization occurring in the district where the moderate group was recruited.
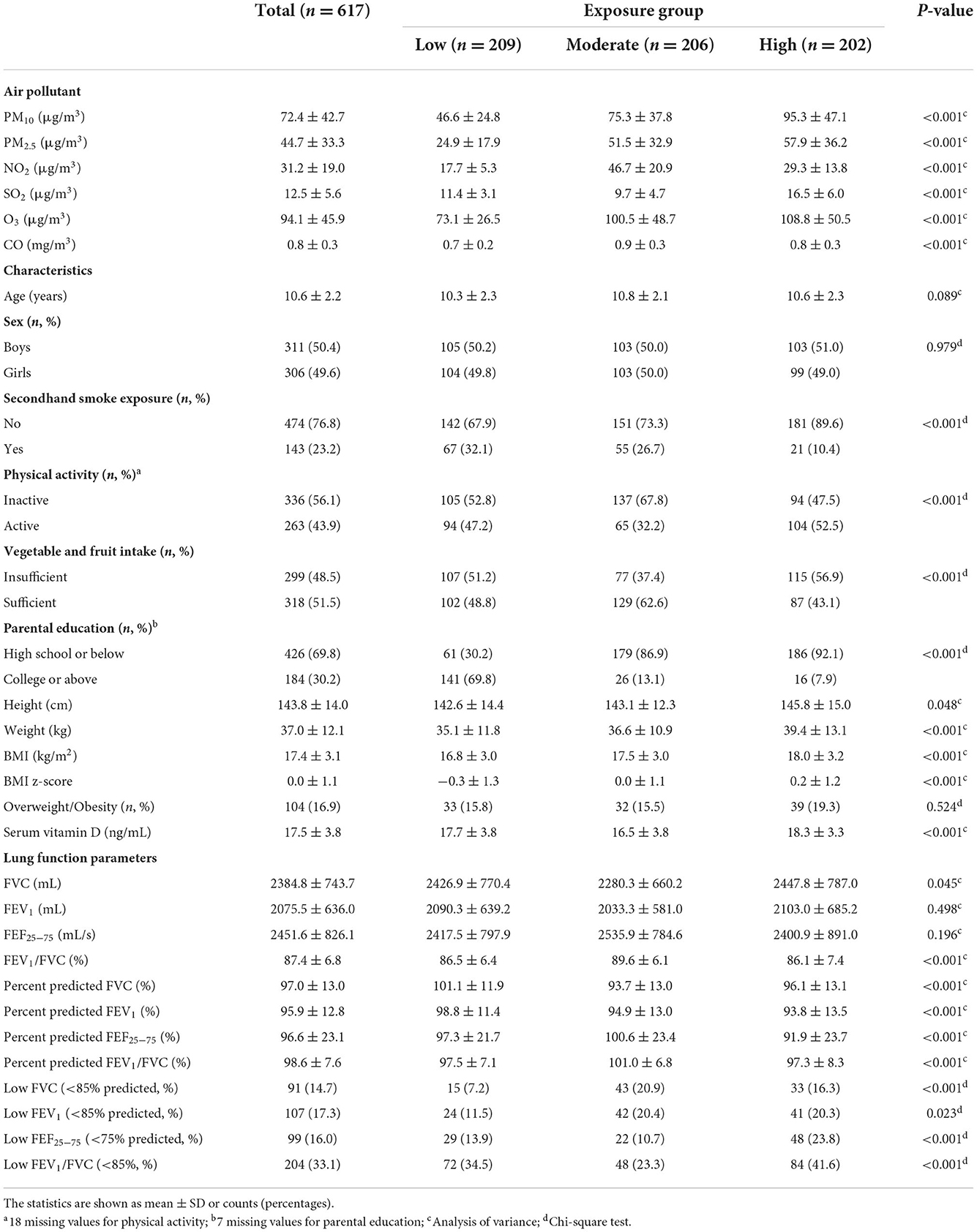
Table 1. Air pollutant concentrations, general characteristics, and lung function parameters among schoolchildren, overall and by exposure group.
The general characteristics and lung function levels of schoolchildren are shown in Table 1. Although there were no significant differences across exposure groups in terms of age and sex, lower proportion of secondhand smoke exposure and parental education level, and higher BMI were observed for children with higher exposure levels. The averages of lung function parameters were 2384.8 mL (SD = 743.7) for FVC, 2075.5 mL (SD = 636.0) for FEV1, 2451.6 mL/s (SD = 826.1) for FEF25−75, and 87.4% (SD = 6.8) for FEV1/FVC. The prevalence of poor lung function for FVC, FEV1, FEF25−75, and FEV1/FVC was 14.7, 17.3, 16.0, and 33.1%, respectively.
The associations between air pollution exposure and lung function parameters are presented in Table 2. After adjusting for covariates, FVC, FEV1, and FEF25−75 showed inverse trends with increasing air pollution levels (ptrend = 0.030, 0.004, 0.012, respectively). Specifically, children in the high exposure group exhibited 73.3 (95% CI: −14.6, 161.2) mL decrement in FVC, 87.9 (95% CI: 9.5, 166.4) mL decrement in FEV1, and 195.3 (95% CI: 30.5, 360.1) mL/s decrement in FEF25−75 compared with those in the low exposure group. FEV1/FVC, reflecting the association patterns with FEV1 and FVC, was not significantly different between groups. As for associations with the prevalence of poor lung function, the results were generally insignificant regardless of the increased odds ratio in the high exposure group.
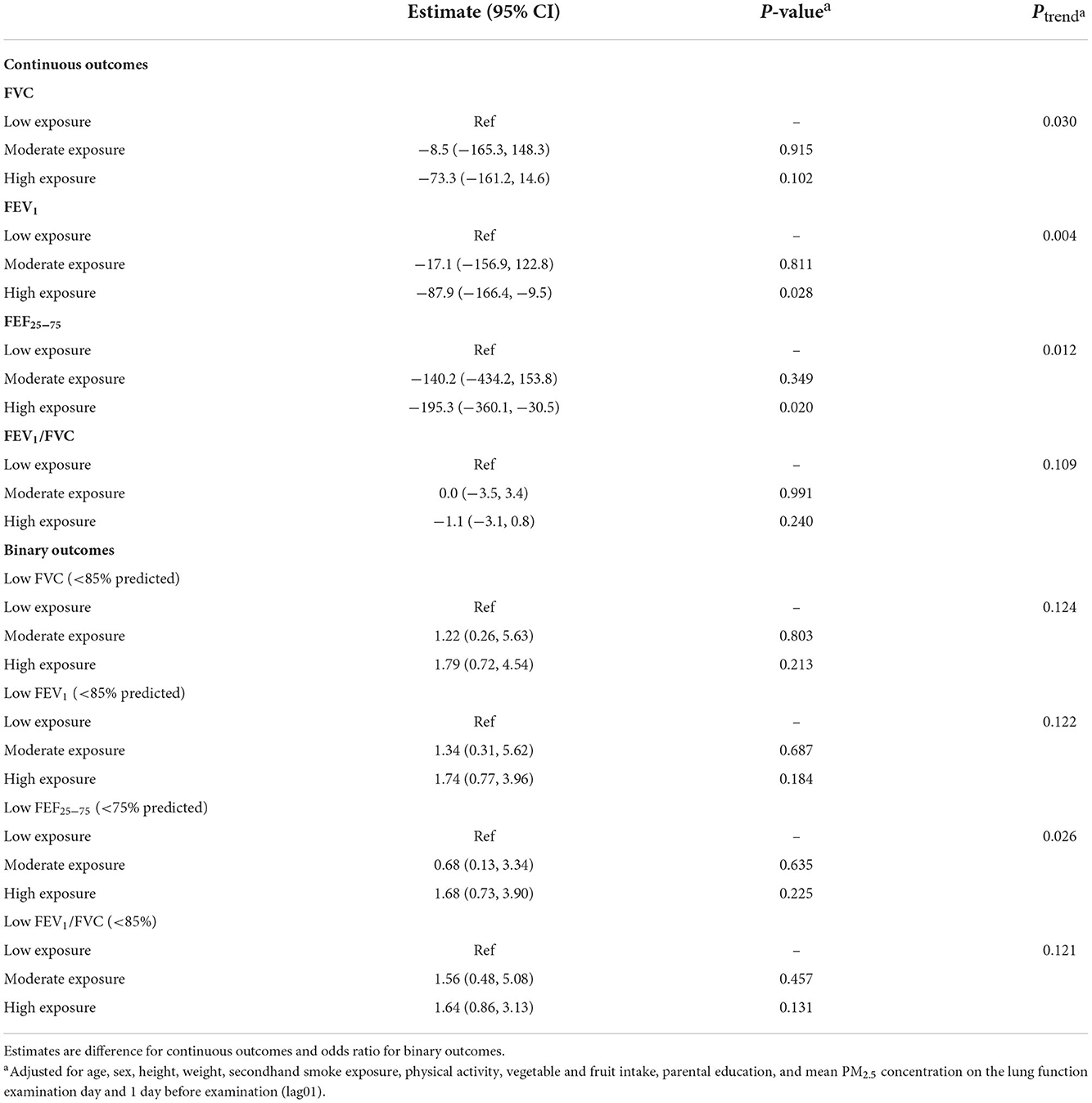
Table 2. Multivariable adjusteda associations between air pollution levels and lung function parameters among all schoolchildren.
Significant interactions of age and sex with air pollution were observed in relation to FEF25−75 and FEV1 (pinteraction = 0.023 and 0.041, respectively). In addition, although no significant modification effects were found for secondhand smoke exposure, BMI, physical activity, and serum vitamin D levels, we did observe significant associations among children with normal weight, non-regular exercise, lower vitamin D, or without exposure to secondhand smoke but not their counterparts (Figure 1). The differences and 95% CI comparing high vs. low exposure group were −107.9 (95% CI: −195.6, −20.3) mL for FEV1 and −311.1 (95% CI: −496.6, −125.5) mL/s for FEF25−75 among children aged 11 years or younger, −98.6 (95% CI: −191.7, −5.4) mL for FEV1 and −264.9 (95% CI: −461.1, −68.8) mL/s for FEF25−75 among girls, −189.7 (95% CI: −366.9, −12.4) mL/s for FEF25−75 among children not exposed to secondhand smoke, −227.3 (95% CI: −397.5, −57.2) mL/s for FEF25−75 among children with normal weight, −100.6 (95% CI: −196.5, −4.8) mL for FEV1 and −256.7 (95% CI: −457.9, −55.6) mL/s for FEF25−75 among physically inactive children, and −274.1 (95% CI: −487.7, −60.5) mL/s for FEF25−75 among children with lower vitamin D levels. Besides, stronger associations with the prevalence of poor lung function for FEF25−75 and/or FEV1/FVC were found in girls and children with normal weight or lower vitamin D (Figure 2).
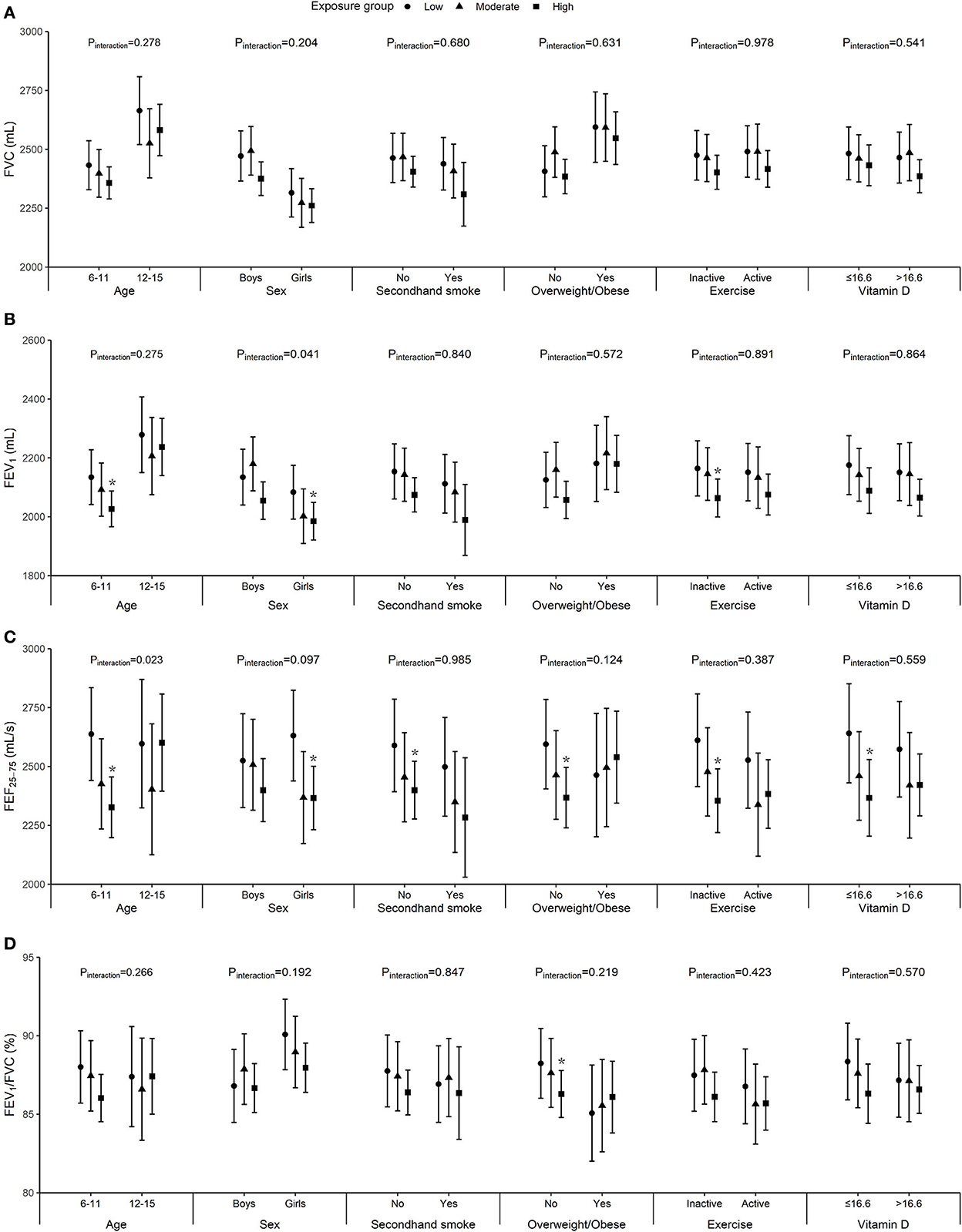
Figure 1. Marginally adjusted means of FVC (A), FEV1 (B), FEF25−75 (C), and FEV1/FVC (D) by age, sex, secondhand smoke exposure, body mass index (BMI), physical exercise, and vitamin D (the median value was 16.6 ng/mL). Models were adjusted for age, height, weight, vegetable and fruit intake, parental education, and mean PM2.5 concentration on the lung function examination day and 1 day before examination (lag01), and sex, secondhand smoke exposure, and physical exercise when appropriate. Error bars represent 95% CI and asterisk indicates p < 0.05.
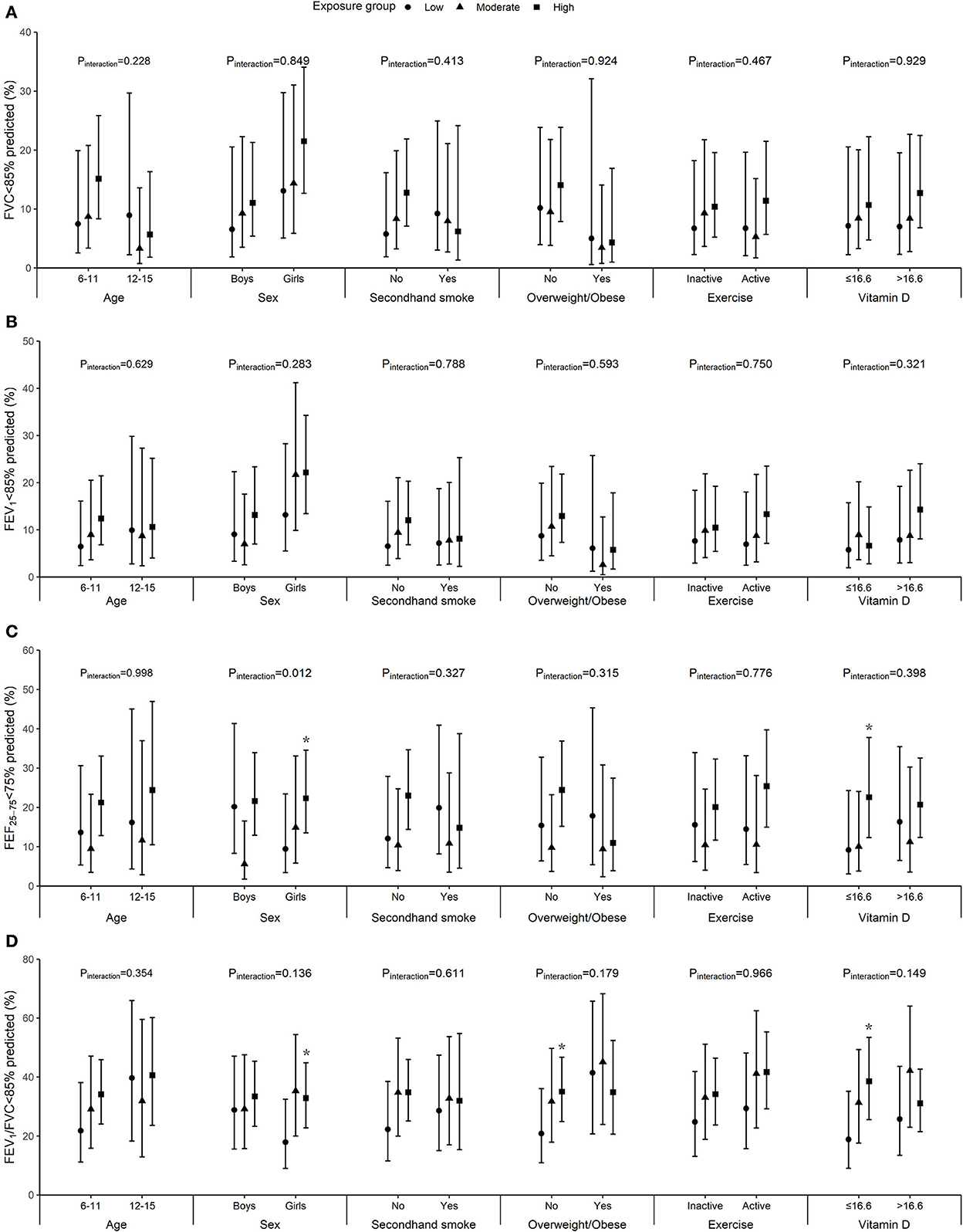
Figure 2. Marginally adjusted probability of low FVC (A), FEV1 (B), FEF25−75 (C), and FEV1/FVC (D) by age, sex, secondhand smoke exposure, body mass index (BMI), physical exercise, and vitamin D (the median value was 16.6 ng/mL). Models were adjusted for age, height, weight, vegetable and fruit intake, parental education, and mean PM2.5 concentration on the lung function examination day and 1 day before examination (lag01), and sex, secondhand smoke exposure, and physical exercise when appropriate. Error bars represent 95% CI and asterisk indicates p < 0.05.
The combined impacts of air pollution and secondhand smoke are shown in Figure 3. Compared with children in the low exposure group and not exposed to secondhand smoke, those exposed to both high level air pollution and secondhand smoke had the worst lung function, with 154.4 (95% CI: −1.1, 309.8) mL decrement in FVC, 164.6 (95% CI: 25.9, 303.3) mL decrement in FEV1, and 305.9 (95% CI: 14.3, 597.5) mL/s decrement in FEF25−75. Similar analyses related to the prevalence of poor lung function yielded no significant results (data not shown).
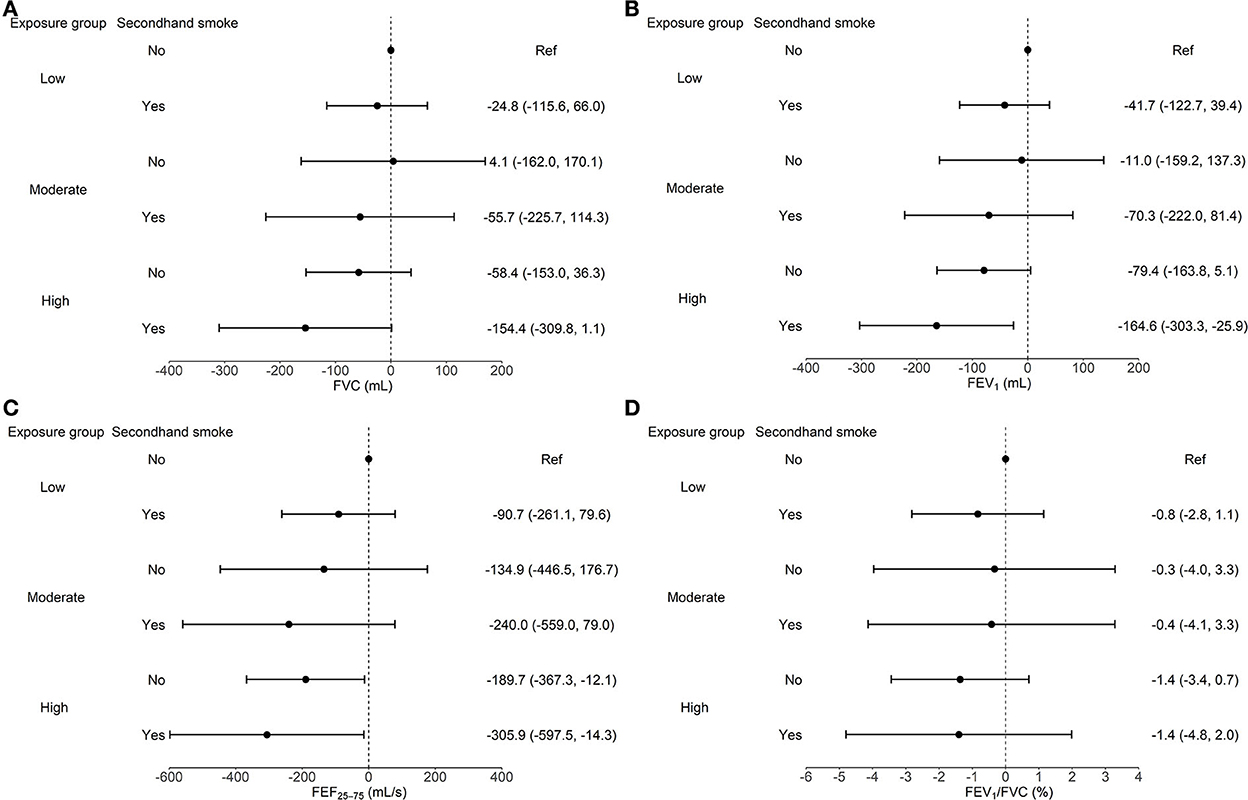
Figure 3. Joint analysis of air pollution and secondhand smoke exposure on FVC (A), FEV1 (B), FEF25−75 (C), and FEV1/FVC (D) among all schoolchildren. Models were adjusted for age, sex, height, weight, physical activity, vegetable and fruit intake, parental education, and mean PM2.5 concentration on the lung function examination day and 1 day before examination (lag01). Error bars represent 95% CI.
In the sensitivity analyses, the inverse trends persisted with regard to associations with percent predicted FEV1 and FEF25−75 (Supplementary Table S1). Regression results restricting to children not exposed to secondhand smoke or without self-reported asthma and additionally adjusting for short-term NO2 or O3 did not differ substantially from those based on the whole population (Supplementary Table S2).
Discussion
To our knowledge, this work represents one of the few studies that investigated the impact of long-term air pollution at contemporary exposure levels in developing countries on the lung function of children in school. Our results showed reduced FEV1 and FEF25−75 with increasing levels of air pollution. The negative associations were more pronounced among children who were younger, girls, not exposed to secondhand smoke, non-overweight, physically inactive, or vitamin D deficient. Moreover, air pollution and secondhand smoke exhibited joint effects on lung function decline.
Among lung function parameters, the largest change associated with air pollution was found for FEF25−75. The same pattern has been observed in regions with low (U.S., Netherlands, Norway, and Italy) and moderate (Hong Kong, China) air pollution level (18–22). Since FEF25−75 is more reflective of small airway dysfunction, our finding suggested that air pollution might exert more influence on small airways than on large airways. This is plausible because higher doses of air pollutants are supposed to deposit in the small airways (23). Although we could not disentangle the individual contribution of each pollutant, PM2.5 and O3 seemed to be responsible for FEF25−75 reduction considering their concentration contrasts between cities and the potential of entering into small airways (18, 24). Smaller particles can more easily penetrate into the lower respiratory tract, and a number of studies support the relation between long-term PM2.5 exposure and lower FEF25−75 (18, 24, 25). Due to relatively low water solubility, O3 can also reach and subsequently be retained in small airways, in spite of the epidemiological evidence regarding its effect on FEF25−75 are somewhat inconsistent (26, 27). In addition to airway flow measure, parameters indicative of mechanical ventilatory function were also involved here and the negative association was found with FEV1 but not FVC. This is in line with many studies reporting a larger association magnitude for FEV1 than for FVC (18–22), which indicates a greater impact on airway obstruction than lung size. The observed changes in lung function, although small from the individual perspective, may lead to a shift in population distribution. We therefore further determined the prevalence of lung function parameters below clinically relevant thresholds and found null associations. In contrast, prior studies based on data from Chinese children reported significant effects of chronic air pollution on the prevalence of poor lung function (6, 28). We acknowledged this study might be underpowered to detect such associations. Differences in age range, air pollution level, and formula used to calculate percent predicted values might also help explain the inconsistent results across studies.
Previous studies have established the exposure-response relationship between long-term air pollution exposure and respiratory disease mortality (29, 30). As lung function is a marker of early lung injury, it is interesting to explore the corresponding relationship using lung function as outcome. Nevertheless, the existing evidence is limited, especially for children, and mainly derived from statistical models. A large longitudinal study in China revealed preliminary results that the decreases of lung function appeared to be faster up to PM2.5 concentration range of 20–25 μg/m3 and slower thereafter (28). In the current study, we provided direct evidence that there is a linear exposure-response relationship between long-term air pollution and lung function impairment when PM2.5 is in the range of 25–60 μg/m3, which is valuable for assessing the health risk of children in developing countries. Likewise, Tsui et al. (27) found that lifetime exposure to intermediate levels of PM10 (25–85 μg/m3) was linearly associated with lower lung function in non-asthmatic children from Taiwan, China. In addition, the fact that lung function started to decrease in the moderate exposure group whose exposure level was slightly above interim target 1 (IT-1) values recommended by WHO highlights the necessity of mandating IT-1 standard in countries with moderate and high air pollution to protect vulnerable population.
It is known that the behaviors and lung development of younger children are different from those of older children, leading us to hypothesize that air pollution might display distinct impacts on children of various ages. However, this topic is understudied before possibly due to the narrow age range of the study population. Two cross-sectional studies in Rome and U.S. showed stronger associations of NO2 and blood manganese with lung function in older children (21, 31). They speculated that longer cumulative exposure to low-dose air pollution might account for this observation. Unlike these studies, younger children seemed to be more vulnerable in this study, suggesting that the role of cumulative exposure in affecting lung function is less obvious in relatively high pollution settings. Indeed, older children generally have higher antioxidant capacity than younger children, enabling them better protection against air pollution-related oxidative stress. A Japanese study found a positive correlation between the serum level of antioxidant capacity biomarker and age in 77 children (32). Besides, the adaptive responses may be more evident among older children as they are exposed to air pollution for a longer period. Relative to age, researches on sex differences in lung function associated with air pollution are more abundant, but the results are rather mixed. While some studies demonstrated a larger reduction of lung function among girls as seen in this study (19–21), others found opposite patterns or no significant results (22, 33–35). The age more or less close to puberty of our subjects lend us credence that the observed finding may be partially attributed to estrogen, since estrogen level correlates with symptom severity of asthma (36).
Exposure to secondhand smoke and overweight/obesity are common among children, both of which appear to affect lung function. Here, the negative associations were stronger in children not exposed to secondhand smoke. To support this finding, many previous cohorts including Framingham Heart, Normative aging, and UK Biobank studies reported higher effects on never/former smokers for associations between PM2.5 and lung function parameters (15, 37, 38). This is not surprising because smoking may have already damaged lung function through inflammation, thus masking the additional effect of air pollution to some extent. However, this does not necessarily imply that avoidance of exposure to secondhand smoke is meaningless for children. Notably, the mean levels of FVC, FEV1, and FEF25−75 were all lower in children exposed to secondhand smoke, and the largest reduction of lung function parameters was found in children who lived in the most polluted area and were exposed to secondhand smoke. As a result, campaigns protecting children from secondhand smoke should be advocated as ever. On the other hand, we found children with normal weight might be more sensitive to the respiratory effect of air pollution, which could also be explained by the pre-existing inflammation induced by excess fat. Contradicting result was reported by the Seven Northeastern Chinese Cities study (6). In that study, the associations between prevalence of lung function impairment and air pollutants were strongest in obese children, followed by overweight children, and weakest in normal-weight children. The modification of age on the relationship of BMI with lung function is likely to shed some light on the discrepancy. In younger children, lung function tends to be higher with increasing BMI (39). But after puberty, their relationship is characterized by an inverted U shape, with lower lung function at both ends of BMI (40). Given our population composition (about 75% are from primary school), the detrimental effect of overweight/obesity on lung function is not expected. This postulation is further evidenced by the stratified result that the modification of overweight/obesity on the association between particulate matter and lung function impairment was more evident in older children than in younger children (6).
As aforementioned, children take physical activity more regularly than adults and higher physical activity has a favorable effect on lung function. Meanwhile, more air pollutants are inhaled by children during exercise. It remains equivocal on whether physical activity promotes or attenuates the adverse health effects of air pollution, which is also a major concern for many parents and policy makers. In the present study, we found physically inactive children might be more susceptible than active children. Similarly, a Chinese nationwide study found the impact of PM10 on FVC was stronger in inactive children (7). Both results add evidence for guiding the behavior of children during pollution days. Although biological mechanisms underlying the modification effect of physical activity are not fully understood, anti-inflammation should be one of the possible pathways. Olivo et al. (41) found that mice undergoing 10-week exercise training (5 days/week, 1 hour/day) exhibited lower proinflammatory cytokines in the lung in response to diesel exhaust particle exposure.
Micronutrients are indispensable for the maintenance of normal tissue function and their deficiencies can cause deficits in lung function (42). Previous studies have explored the role of micronutrients, especially those with antioxidant properties, in modifying the association between air pollution and lung function. For instance, Romieu et al. (43) observed a significantly negative association of O3 at 1 day before spirometry with FEV1 and FEF25−75 in children with asthma receiving a placebo but not those receiving vitamin supplement (vitamin C and E). Although vitamin D is well known for its implication in calcium homeostasis, there is growing evidence relating vitamin D to lung function and asthma (44, 45). In Normative aging study, smokers with vitamin D deficiency were found to have lower lung function and more rapid lung function decline (46). To our knowledge, this is the first study demonstrating that a lower level of vitamin D might aggravate the reduction of lung function among healthy children exposed to long-term air pollution. Animal study manifested mice exposed to vitamin D deficiency had increased airway smooth muscle mass, which is a feature of airway remodeling of asthma and chronic obstructive pulmonary disease (47). Given the cross-sectional nature of our study, however, whether vitamin D supplements can alleviate the harmful effects of air pollution on the respiratory system needs further investigations using a well-designed randomized controlled trial.
This study has several limitations. First, air pollution data of fixed monitoring stations were used as a proxy of children's exposure level as detailed residential address was not mandatory in the questionnaire, raising concerns of exposure misclassification. However, such measurement errors should not be substantial in terms of characterizing long-term exposure and non-differential misclassification usually biases estimates toward no association. Second, although we did find an increased prevalence of poor lung function in the high exposure group as well as stronger associations in certain subgroups, most of these results did not reach statistical significance possibly owing to sample size. Third, only including vitamin D is not adequate to provide a full picture of nutritional intervention in alleviating the adverse health effects of air pollution. Fourth, parental education was severely imbalanced across exposure groups. Covariate adjustment might not fully remove residual confounding. Finally, cotinine was not determined to measure the actual exposure level of secondhand smoke more accurately, nor moderate and vigorous physical activities were differentiated from each other.
In conclusion, our findings indicate that long-term air pollution at levels of exposure currently experienced by many people in developing countries is associated with lower levels of large and small airway function in Chinese children, with a stronger impact on the latter. Reducing secondhand smoke exposure, encouraging physical activity, and supplementing vitamin D are important public health priorities to mitigate the burden of respiratory disease among children in China and other developing countries with comparable air pollution levels. Future studies measuring individual exposure and analyzing the data with more advanced methods (e.g., exposome-wide association) are warranted to provide more insight into the impact of long-term air pollution on lung growth of children and the potential effect of air pollution regulation policies (48, 49).
Data availability statement
The raw data supporting the conclusions of this article will be made available by the authors, without undue reservation.
Ethics statement
The studies involving human participants were reviewed and approved by Research Ethics Committee of the Anhui Center for Disease Control and Prevention and the National Institute for Occupational Health and Poison Control, Chinese Center for Disease Control and Prevention. Written informed consent to participate in this study was provided by the participants' legal guardian/next of kin.
Author contributions
WL, HD, and JT contributed to conceptualization and critical revision of the manuscript. JT collected and interpreted the data. JL conducted data analysis and drafted the manuscript. All authors contributed to the article and approved the submitted version.
Funding
This study was supported by grants of the National Natural Science Foundation of China (NSFC 82103797) and the National Key R&D Program of China (2017YFC0211604).
Conflict of interest
The authors declare that the research was conducted in the absence of any commercial or financial relationships that could be construed as a potential conflict of interest.
Publisher's note
All claims expressed in this article are solely those of the authors and do not necessarily represent those of their affiliated organizations, or those of the publisher, the editors and the reviewers. Any product that may be evaluated in this article, or claim that may be made by its manufacturer, is not guaranteed or endorsed by the publisher.
Supplementary material
The Supplementary Material for this article can be found online at: https://www.frontiersin.org/articles/10.3389/fpubh.2022.988242/full#supplementary-material
References
1. GBD Risk Factors Collaborators. Global burden of 87 risk factors in 204 countries and territories, 1990–2019: a systematic analysis for the global burden of disease study 2019. Lancet. (2020) 396:1223–49. doi: 10.1016/S0140-6736(20)30752-2
2. Bui DS, Burgess JA, Lowe AJ, Perret JL, Lodge CJ, Bui M, et al. Childhood lung function predicts adult chronic obstructive pulmonary disease and asthma-chronic obstructive pulmonary disease overlap syndrome. Am J Respir Crit Care Med. (2017) 196:39–46. doi: 10.1164/rccm.201606-1272OC
3. Gehring U, Gruzieva O, Agius RM, Beelen R, Custovic A, Cyrys J, et al. Air pollution exposure and lung function in children: the ESCAPE project. Environ Health Perspect. (2013) 121:1357–64. doi: 10.1289/ehp.1306770
4. Milanzi EB, Koppelman GH, Smit HA, Wijga AH, Oldenwening M, Vonk JM, et al. Air pollution exposure and lung function until age 16 years: the PIAMA birth cohort study. Eur Respir J. (2018) 52:1800218. doi: 10.1183/13993003.00218-2018
5. Available online at: https://www.who.int/news/item/29-10-2018-more-than-90-of-the-worlds-children-breathe-toxic-air-every-day (accessed January 22, 2022).
6. Xing X, Hu L, Guo Y, Bloom MS Li S, Chen G, et al. Interactions between ambient air pollution and obesity on lung function in children: the seven Northeastern Chinese cities (SNEC) Study. Sci Total Environ. (2020) 699:134397. doi: 10.1016/j.scitotenv.2019.134397
7. Wang HJ Li Q, Guo Y, Song JY, Wang Z, Ma J. Geographic variation in Chinese children' forced vital capacity and its association with long-term exposure to local PM10: a national cross-sectional study. Environ Sci Pollut Res Int. (2017) 24:22442–9. doi: 10.1007/s11356-017-9812-9
8. Zhang Q, Zheng Y, Tong D, Shao M, Wang S, Zhang Y, et al. Drivers of improved PM2.5 air quality in China from 2013 to 2017. Proc Natl Acad Sci USA. (2019) 116:24463–9. doi: 10.1073/pnas.1907956116
9. Schultz ES, Litonjua AA, Melen E. Effects of long-term exposure to traffic-related air pollution on lung function in children. Curr Allergy Asthma Rep. (2017) 17:41. doi: 10.1007/s11882-017-0709-y
10. de Onis M, Onyango AW, Borghi E, Siyam A, Nishida C, Siekmann J. Development of a WHO growth reference for school-aged children and adolescents. Bull World Health Organ. (2007) 85:660–7. doi: 10.2471/BLT.07.043497
11. Miller MR, Hankinson J, Brusasco V, Burgos F, Casaburi R, Coates A, et al. Standardisation of spirometry. Eur Respir J. (2005) 26:319–38. doi: 10.1183/09031936.05.00034805
12. Quanjer PH, Stanojevic S, Cole TJ, Baur X, Hall GL, Culver BH, et al. Multi-ethnic reference values for spirometry for the 3-95-yr age range: the global lung function 2012 equations. Eur Respir J. (2012) 40:1324–43. doi: 10.1183/09031936.00080312
13. Moshammer H, Hoek G, Luttmann-Gibson H, Neuberger MA, Antova T, Gehring U, et al. Parental smoking and lung function in children: an international study. Am J Respir Crit Care Med. (2006) 173:1255–63. doi: 10.1164/rccm.200510-1552OC
14. Johnson JD, Theurer WM, A. stepwise approach to the interpretation of pulmonary function tests. Am Fam Physician. (2014) 89:359–66. doi: 10.1016/j.aprim.2013.09.001
15. Lepeule J, Litonjua AA, Coull B, Koutrakis P, Sparrow D, Vokonas PS, et al. Long-term effects of traffic particles on lung function decline in the elderly. Am J Respir Crit Care Med. (2014) 190:542–8. doi: 10.1164/rccm.201402-0350OC
16. Jacquemin B, Lepeule J, Boudier A, Arnould C, Benmerad M, Chappaz C, et al. Impact of geocoding methods on associations between long-term exposure to urban air pollution and lung function. Environ Health Perspect. (2013) 121:1054–60. doi: 10.1289/ehp.1206016
17. Muller CJ, MacLehose RF. Estimating predicted probabilities from logistic regression: different methods correspond to different target populations. Int J Epidemiol. (2014) 43:962–70. doi: 10.1093/ije/dyu029
18. Neophytou AM, White MJ, Oh SS, Thakur N, Galanter JM, Nishimura KK, et al. Air pollution and lung function in minority youth with asthma in the GALA II (Genes-environments and admixture in Latino Americans) and SAGE II (Study of African Americans, asthma, genes, and environments) studies. Am J Respir Crit Care Med. (2016) 193:1271–80. doi: 10.1164/rccm.201508-1706OC
19. Gehring U, Beelen R, Eeftens M, Hoek G, de Hoogh K, de Jongste JC, et al. Particulate matter composition and respiratory health: the PIAMA Birth Cohort study. Epidemiology. (2015) 26:300–9. doi: 10.1097/EDE.0000000000000264
20. Oftedal B, Brunekreef B, Nystad W, Madsen C, Walker SE, Nafstad P. Residential outdoor air pollution and lung function in schoolchildren. Epidemiology. (2008) 19:129–37. doi: 10.1097/EDE.0b013e31815c0827
21. Rosenlund M, Forastiere F, Porta D, De Sario M, Badaloni C, Perucci CA. Traffic-related air pollution in relation to respiratory symptoms, allergic sensitisation, and lung function in schoolchildren. Thorax. (2009) 64:573–80. doi: 10.1136/thx.2007.094953
22. Gao Y, Chan EY Li LP, He QQ, Wong TW. Chronic effects of ambient air pollution on lung function among Chinese children. Arch Dis Child. (2013) 98:128–35. doi: 10.1136/archdischild-2011-301541
23. Gotschi T, Heinrich J, Sunyer J, Kunzli N. Long-term effects of ambient air pollution on lung function: a review. Epidemiology. (2008) 19:690–701. doi: 10.1097/EDE.0b013e318181650f
24. Gauderman WJ, Urman R, Avol E, Berhane K, McConnell R, Rappaport E, et al. Association of improved air quality with lung development in children. N Engl J Med. (2015) 372:905–13. doi: 10.1056/NEJMoa1414123
25. Sarigiannis DA, Karakitsios SP, Zikopoulos D, Nikolaki S, Kermenidou M. Lung cancer risk from PAHs emitted from biomass combustion. Environ Res. (2015) 137:147–56. doi: 10.1016/j.envres.2014.12.009
26. Usemann J, Decrue F, Korten I, Proietti E, Gorlanova O, Vienneau D, et al. Exposure to moderate air pollution and associations with lung function at school-age: A birth cohort study. Environ Int. (2019) 126:682–9. doi: 10.1016/j.envint.2018.12.019
27. Tsui HC, Chen CH, Wu YH, Chiang HC, Chen BY, Guo YL. Lifetime exposure to particulate air pollutants is negatively associated with lung function in non-asthmatic children. Environ Pollut. (2018) 236:953–61. doi: 10.1016/j.envpol.2017.10.092
28. Guo C, Hoek G, Chang LY, Bo Y, Lin C, Huang B, et al. Long-term exposure to ambient fine particulate matter (PM25) and lung function in children, adolescents, and young adults: a longitudinal cohort study. Environ Health Perspect. (2019) 127:127008. doi: 10.1289/EHP5220
29. Fischer PH, Marra M, Ameling CB, Hoek G, Beelen R, de Hoogh K, et al. Air pollution and mortality in seven million adults: the dutch environmental longitudinal study (DUELS). Environ Health Perspect. (2015) 123:697–704. doi: 10.1289/ehp.1408254
30. Strak M, Weinmayr G, Rodopoulou S, Chen J, de Hoogh K, Andersen ZJ, et al. Long term exposure to low level air pollution and mortality in eight European cohorts within the ELAPSE project: pooled analysis. BMJ. (2021) 374:n1904. doi: 10.1136/bmj.n1904
31. Madrigal JM, Persky V, Pappalardo A, Argos M. Association of heavy metals with measures of pulmonary function in children and youth: results from the National Health and Nutrition Examination Survey (NHANES). Environ Int. (2018) 121:871–8. doi: 10.1016/j.envint.2018.09.045
32. Morimoto M, Hashimoto T, Tsuda Y, Kitaoka T, Kyotani S. Evaluation of oxidative stress and antioxidant capacity in healthy children. J Chin Med Assoc. (2019) 82:651–4. doi: 10.1097/JCMA.0000000000000045
33. Lee YL, Wang WH, Lu CW, Lin YH, Hwang BF. Effects of ambient air pollution on pulmonary function among schoolchildren. Int J Hyg Environ Health. (2011) 214:369–75. doi: 10.1016/j.ijheh.2011.05.004
34. Svendsen ER, Gonzales M, Mukerjee S, Smith L, Ross M, Walsh D, et al. GIS-modeled indicators of traffic-related air pollutants and adverse pulmonary health among children in El Paso, Texas. Am J Epidemiol. (2012) 176(Suppl 7):S131–41. doi: 10.1093/aje/kws274
35. Rice MB, Rifas-Shiman SL, Litonjua AA, Oken E, Gillman MW, Kloog I, et al. Lifetime exposure to ambient pollution and lung function in children. Am J Respir Crit Care Med. (2016) 193:881–8. doi: 10.1164/rccm.201506-1058OC
36. Keselman A, Heller N. Estrogen signaling modulates allergic inflammation and contributes to sex differences in asthma. Front Immunol. (2015) 6:568. doi: 10.3389/fimmu.2015.00568
37. Rice MB, Ljungman PL, Wilker EH, Dorans KS, Gold DR, Schwartz J, et al. Long-term exposure to traffic emissions and fine particulate matter and lung function decline in the Framingham heart study. Am J Respir Crit Care Med. (2015) 191:656–64. doi: 10.1164/rccm.201410-1875OC
38. Doiron D, de Hoogh K, Probst-Hensch N, Fortier I, Cai Y, De Matteis S, et al. Air pollution, lung function and COPD: results from the population-based UK Biobank study. Eur Respir J. (2019) 54:2018. doi: 10.1183/13993003.02140-2018
39. Kochli S, Endes K, Bartenstein T, Usemann J, Schmidt-Trucksass A, Frey U, et al. Lung function, obesity and physical fitness in young children: the EXAMIN YOUTH study. Respir Med. (2019) 159:105813. doi: 10.1016/j.rmed.2019.105813
40. Byberg KK, Mikalsen IB Eide GE, Forman MR, Juliusson PB, Oymar K. The associations between weight-related anthropometrics during childhood and lung function in late childhood: a retrospective cohort study. BMC Pulm Med. (2018) 18:10. doi: 10.1186/s12890-017-0567-3
41. Olivo CR, Castro TBP, Riane A, Regonha T, Rivero D, Vieira RP, et al. The effects of exercise training on the lungs and cardiovascular function of animals exposed to diesel exhaust particles and gases. Environ Res. (2022) 203:111768. doi: 10.1016/j.envres.2021.111768
42. McKeever TM, Lewis SA, Smit HA, Burney P, Cassano PA, Britton J, et al. multivariate analysis of serum nutrient levels and lung function. Respir Res. (2008) 9:67. doi: 10.1186/1465-9921-9-67
43. Romieu I, Sienra-Monge JJ, Ramirez-Aguilar M, Tellez-Rojo MM, Moreno-Macias H, Reyes-Ruiz NI, et al. Antioxidant supplementation and lung functions among children with asthma exposed to high levels of air pollutants. Am J Respir Crit Care Med. (2002) 166:703–9. doi: 10.1164/rccm.2112074
44. Zosky GR, Berry LJ, Elliot JG, James AL, Gorman S, Hart PH. Vitamin D deficiency causes deficits in lung function and alters lung structure. Am J Respir Crit Care Med. (2011) 183:1336–43. doi: 10.1164/rccm.201010-1596OC
45. Andujar-Espinosa R, Salinero-Gonzalez L, Illan-Gomez F, Castilla-Martinez M, Hu-Yang C, Ruiz-Lopez FJ. Effect of vitamin D supplementation on asthma control in patients with vitamin D deficiency: the ACVID randomised clinical trial. Thorax. (2021) 76:126–33. doi: 10.1136/thoraxjnl-2019-213936
46. Lange NE, Sparrow D, Vokonas P, Litonjua AA. Vitamin D deficiency, smoking, and lung function in the normative aging study. Am J Respir Crit Care Med. (2012) 186:616–21. doi: 10.1164/rccm.201110-1868OC
47. Foong RE, Bosco A, Jones AC, Gout A, Gorman S, Hart PH, et al. The effects of in utero vitamin D deficiency on airway smooth muscle mass and lung function. Am J Respir Cell Mol Biol. (2015) 53:664–75. doi: 10.1165/rcmb.2014-0356OC
48. Patel CJ, Bhattacharya J, Butte AJ. An Environment-Wide Association Study (EWAS) on type 2 diabetes mellitus. PLoS ONE. (2010) 5:e10746. doi: 10.1371/journal.pone.0010746
Keywords: air pollution, long-term, lung function, children, effect modification
Citation: Teng J, Li J, Yang T, Cui J, Xia X, Chen G, Zheng S, Bao J, Wang T, Shen M, Zhang X, Meng C, Wang Z, Wu T, Xu Y, Wang Y, Ding G, Duan H and Li W (2022) Long-term exposure to air pollution and lung function among children in China: Association and effect modification. Front. Public Health 10:988242. doi: 10.3389/fpubh.2022.988242
Received: 07 July 2022; Accepted: 18 November 2022;
Published: 14 December 2022.
Edited by:
Tao Liu, Jinan University, ChinaReviewed by:
Bülent Taner Karadaǧ, Marmara University, TurkeyDenis Sarigiannis, Aristotle University of Thessaloniki, Greece
Rong Zhang, Hebei Medical University, China
Copyright © 2022 Teng, Li, Yang, Cui, Xia, Chen, Zheng, Bao, Wang, Shen, Zhang, Meng, Wang, Wu, Xu, Wang, Ding, Duan and Li. This is an open-access article distributed under the terms of the Creative Commons Attribution License (CC BY). The use, distribution or reproduction in other forums is permitted, provided the original author(s) and the copyright owner(s) are credited and that the original publication in this journal is cited, in accordance with accepted academic practice. No use, distribution or reproduction is permitted which does not comply with these terms.
*Correspondence: Weidong Li, bHdkQGFoY2RjLmNvbS5jbg==
†These authors have contributed equally to this work and share first authorship