- 1Non-Profit Research, Antwerp, Belgium
- 2Medstar Health, Brooke Grove Rehabilitation Village, Sandy Spring, MD, United States
- 3Infectious Disease, Adventist Healthcare, White Oak Medical Center, Silver Spring, MD, United States
- 4Departments of Family and Community Medicine and Public Health Sciences, Penn State College of Medicine, Pennsylvania, PA, United States
- 5Department of Emergency Medicine, Augusta University, Augusta, GA, United States
- 6Department of Paediatric Surgery, Park Medical Research and Welfare Society, Kolkata, West Bengal, India
- 7Department of Surgery, Aga Khan University, Karachi, Pakistan
- 8Otolaryngology Unit, Treviso Hospital, Treviso, Italy
- 9Department of Pharmacy, University of Patras, Patras, Greece
- 10Department of Oral Biology, Augusta University, Augusta, GA, United States
- 11Department of Oral Health and Diagnostic Sciences, Augusta University, Augusta, GA, United States
With unrelenting SARS-CoV-2 variants, additional COVID-19 mitigation strategies are needed. Oral and nasal saline irrigation (SI) is a traditional approach for respiratory infections/diseases. As a multidisciplinary network with expertise/experience with saline, we conducted a narrative review to examine mechanisms of action and clinical outcomes associated with nasal SI, gargling, spray, or nebulization in COVID-19. SI was found to reduce SARS-CoV-2 nasopharyngeal loads and hasten viral clearance. Other mechanisms may involve inhibition of viral replication, bioaerosol reduction, improved mucociliary clearance, modulation of ENaC, and neutrophil responses. Prophylaxis was documented adjunctive to personal protective equipment. COVID-19 patients experienced significant symptom relief, while overall data suggest lower hospitalization risk. We found no harm and hence recommend SI use, as safe, inexpensive, and easy-to-use hygiene measure, complementary to hand washing or mask-wearing. In view of mainly small studies, large well-controlled or surveillance studies can help to further validate the outcomes and to implement its use.
Keypoints
• Oronasal saline irrigation is an inexpensive intervention to prevent and relieve common colds and upper respiratory infections.
• COVID-19 patients may also benefit, as saline irrigation of the nose and throat was found to reduce nasopharyngeal viral load, fasten viral clearance, and relieve symptoms of COVID-19.
• Overall, the small studies point to a potential benefit of saline irrigation on transmission, hospitalization, oxygen need, ICU admission, and/or mortality; confirmation in large trials is warranted.
• Mechanisms of saline include, apart from the rinse effect that can limit micro-aspiration of virus and secretions from the nasopharynx to deeper airways and lungs, also direct effects of iso- or mild hypertonic saline on SARS-CoV-2 replication (impairment of growth and fusion in vitro), mucosal hydration, mucociliary clearance, ENaC channel activity, and obstructive mucus/NET formation, all mechanisms that can help to reduce and prevent the development of more invasive severe disease.
• Oral and nasal saline rinsing can be considered for prevention and as an early intervention in SARS-CoV-2 infection, adjunctive to current standard protection including mask wear, distancing, and hand hygiene.
• Saline nebulization can be considered in patients who cannot do saline irrigation, or with lower respiratory tract signs or symptoms: The released bioaerosol does not contain live virus.
1. Introduction
In the ongoing COVID-19 pandemic, measures to limit the spread of SARS-CoV-2 have been a continuous and evolving challenge (1). Continuous mutations of its spike (S) protein circumvent effective vaccine and monoclonal antibody development (2, 3). New antiviral treatments are expensive and subject to side effects (4). Saline irrigation (SI) of the nose and throat is an inexpensive hygienic option that is proven effective for reducing the burden of other viral, bacterial, and acute or chronic respiratory illnesses (5–8) and has been empirically suggested as option for SARS-CoV-2 mitigation (9–12). Today, SI is also removed from the general WHO myth buster page (13, 14). While consensus guidance on its use has recently been proposed in China by a Chinese Rhinopathy Research Cooperation Group (15), published evidence for the utility of SI as adjunctive hygiene in COVID-19 has yet to be reviewed. We conducted a narrative review of this topic and present the clinical evidence and currently understood mechanisms for SI's efficacy in COVID-19 and propose recommendations for use and further evaluation.
2. Methods
Mixed methods were used for this descriptive review, generated with systematic search strategies, as described in the Supplementary Appendix Part A. The objectives of the reviewing process were to review the evidence for saline in COVID-19 by (1) evaluating clinical outcomes obtained with saline as (oro)nasal irrigation fluid or gargling in COVID-19 or SARS-CoV-2 infection and (2) exploring clinically relevant mechanisms of how these outcomes may be achieved—this with the objective to consolidate benefits and risks, as well as formulate recommendations. Nebulization was added as the search term to allow assessment of the harm–benefit balance of this procedure, often discouraged in COVID-19 (16).
The search strategies for the clinical data and mechanisms of action are reported in Supplementary Appendix Part A.1. p. 02–05, (Supplementary Table S1) and Supplementary Appendix Part A.2. p. 10–11 (Supplementary Table S2), respectively. With the literature on saline being abundant, a stricter search strategy was conducted for clinical reports of relevance on PubMed from 1 January 2022 to 27 October 2022 (Figure 1). Initial screening of clinicaltrials.gov and WHO trial databases had revealed that saline was not the subject of a large public or developmental program, while it was often also serving as a reference or placebo, which explains the substantial heterogeneity of study designs. Therefore, studies were eligible, irrespective of whether saline (irrigation, mouth rinse, gargling, spray/drop, or nebulization) was the subject or the reference/placebo of the study. In view of the exploratory nature of this review, additional searches on the Internet and PubMed were performed, with the use of additional search terms, described in Supplementary Appendix, p. 03, such as a clinical trial or clinical study, nasal sprays or drops, or using the protocol numbers from trial databases, as to retrieve eventual results. The material was progressively compiled. Details on reasons for rejection of sources are listed in Supplementary Appendix, p. 03–06 and p. 10, respectively. Most studies were small. If a bias was identified, this was noted in the tables and/or discussed in the text. In total, 33 clinical studies or reports were eligible and tabulated, which are stratified (Supplementary Appendix, p. 06, 07) by the effects of saline on viral load and/or clearance (Supplementary Table S3, p. 11) and/or effects on clinical outcomes (Supplementary Table S4, p. 17). Rates of hospitalization and risks (intensive care uptake, pneumonia severity scores, ventilation, and mortality) were compiled separately in Supplementary Table S5, p. 30. Grading, pooling, or meta-analysis of outcomes was not performed due to often small-sized (local) studies, substantial heterogeneity in study design, disease severity and outcome parameters, and/or poor or limited data reporting.
For the mechanisms of action of saline of relevance to COVID-19, initial search strategies (Supplementary Appendix, p. 10) addressed the effect(s) of saline on SARS-CoV-2 viral load (aspiration), replication, exhaled bioaerosol, ENaC channel activity, hydration and mucociliary clearance, neutrophil response, and hypochlorous (HOCl) formation. Yet, mechanisms were also examined from a broader translational view by the Network. As the COVID-19 literature is extensive, sources were limited to one (if consistent) or the most representative articles or reviews (if multiple sources or reviews). The mechanisms addressed and found in support of SI are summarized in Figure 2.
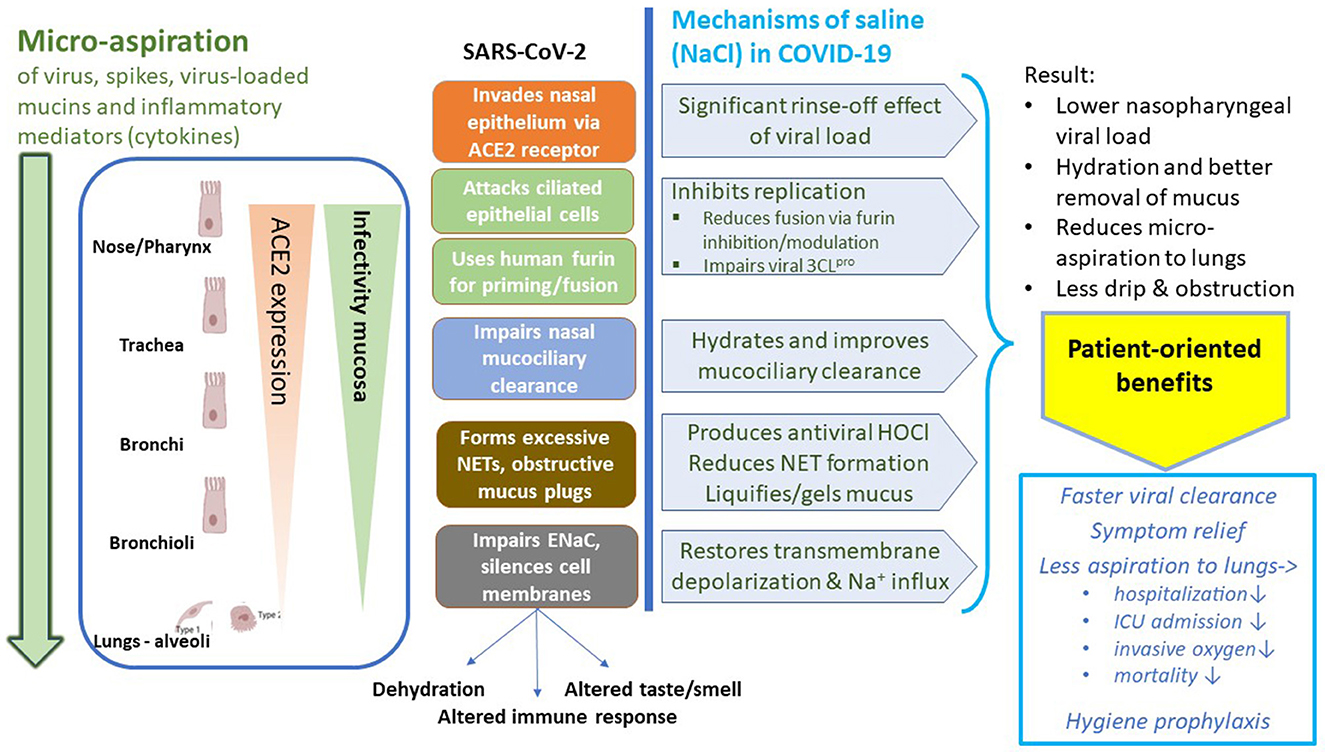
Figure 2. Mechanisms of action of saline relevant to COVID-19. ACE2, angiotensin-converting enzyme 2; NET, neutrophil extracellular trap; ENaC, epithelial sodium channel; HOCl, hypochlorous acid formed through neutrophil myeloperoxidase activity in the presence of NaCl.
The findings of the review process were compiled according to (A) the major mechanism documented clinically for saline irrigation (viral load reduction) and its relevance to transmission, (B) additional mechanisms that can be effectuated by saline mucosal irrigation, and that may be protective in the initial stage of COVID-19 (sodium chloride (NaCl) homeostasis and innate immune response), and (C) clinical outcomes of studies in COVID-19. Clinical benefits, risks, lessons learned, recommendations, and conclusion were formulated and reflect the final consensus in January 2023, after evaluation and discussion of the benefit–harm balance, based on the consolidated findings from the tabulated data.
3. Results
3.1. Viral load reduction and transmission
3.1.1. SARS-CoV-2 pathophysiology: rationale for viral load reduction
Similarly as for common colds (8), the main rationale proposed for using saline irrigation is to rinse the nasal and throat mucosa, removing SARS-CoV-2 and limiting the viral load from these tissues.
Nasal multiciliated cells are primary targets for the entry of SARS-CoV-2 (17), which after replication are released at their apical side (18), allowing rapid reinfection and progressive epithelial damage, with a spread from the naso- and oropharynx to the deeper throat and airways. The infection initially starts almost exclusively in the ciliated epithelia of the nose (19), while micro-aspiration of virus, sputum, mucins, and inflammatory secretions from the oral and nasal cavities is increasingly recognized as the main mechanism for infecting deeper airways and lungs (Figure 2) (20–22). This pathway in SARS-CoV-2 pathophysiology is supported by the findings of (upregulated) expression patterns of SARS-CoV-2 entry genes (such as ACE2 and TMPRRS2) and broad nasal, buccal, and blood gene expression analysis, the absence of alveolar macrophages and alveolar invasion at onset of the disease, the difficulty to infect human lung tissues with SARS-CoV-2 in vitro (in contrast to nasal and in vitro host cells), and the progressively observed immune gene upregulation (20–25). In addition, mucin secretions are abnormal and become upregulated, as well as nasal inflammatory cytokines (26, 27). Mucins often present as viscous plugs obstructing the trachea and bronchi (see also Section 3.2.2.). The highest viral loads are detected in sputum, followed by nasopharynx, while these are usually lower in saliva (28–30). Moreover, development of viral SARS-CoV-2 RNA shedding and bronchopneumonia was found to be dose-dependent in response to the dose of virus intranasally delivered, supporting the concept that rinsing off virus in an early stage may reduce disease development and severity (31). To further illustrate the importance of the removal of aerosolized secretions, it is important to note that elements of the virus itself, such as the released S proteins, can generate inflammation and tissue damage in the picomolar range, as well as bind multiple innate immune receptors, contributing to the disturbed local immune response (32, 33)]. An in vitro study showed that endothelial cells are normally not invaded by SARS-CoV-2; following viral replication, however, epithelial released cytokines and components induced during the infection affected the neighboring endothelial cells, resulting in damage of the epithelial/endothelial barrier function and causing the viral dissemination (34). Observations that the cytokine levels in sputum, and not serum, are predictive for lung damage and prognosis of severe COVID-19 (35), and that mucus surprisingly releases finer aerosol particles the more its viscoelasticity decreases (36) (dry plugs common in COVID-19), support further the concept of micro-aspiration of virus and infected bioaerosol to the deeper airways and lungs.
Micro-aspiration of virus and secretions from the oronasal cavity not only allows progressive viral invasion of deeper airways and lungs causing COVID-19 pneumonia but also may contribute to severe co-infected pneumonia: Viral, bacterial, and fungal secondary infections are common in SARS-CoV-2 patients, and viruses, bacteria, and fungi were frequently found in the plugs removed from the trachea and bronchi during bronchial/tracheal lavage (37–40). Studies on microbiota identified depletion of beneficial commensals and an upsurge of opportunistic pathogens, also in the oral and nasopharyngeal cavity (41).
Higher SARS-CoV-2 viral loads and longer virus shedding have been associated with disease severity and disease progression, as well as with transmission (42–44). Hence, their reduction may help to avert the infection, reduce micro-aspiration of the virus to the lower respiratory tract, and prevent the progression to a more severe disease state. Earlier studies in other respiratory conditions have shown that nasal SI helps to remove pathogens, allergens, debris, and secretions from the nasal cavity and reduce sinonasal and respiratory infections (6–8, 10, 45), while respecting the normal sinonasal commensal microbiome (46). SI is, therefore, a reasonable choice to reduce viral loads and micro-aspiration during COVID-19 and potentially help prevent the development of pneumonia and acute respiratory distress syndrome (ARDS).
As both the nasal and oropharyngeal cavity may become infected, and so may lead to both micro-aspiration of the virus to the lungs and exhaled infectious bioaerosol, and so to airborne transmission, (also via saliva) (47), the effects of nasal irrigation, gargling and nebulization of saline on viral loads, bioaerosol formation, transmission, and prophylaxis are considered in this section.
3.1.2. Rinse effect reducing viral loads
Fifteen studies were identified, reporting on the effect of saline gargles (n = 4) and SI (n = 9) or nasal spray (n = 2) on viral load or clearance to PCR-negative status (Supplementary Table S3, p. 11). Symptomatic and asymptomatic patients, different saline concentrations (0.9–5%) and schedules, routes (nasal, gargling, and combined), and methods of SI (neti pot, spray or pressurized bottle, or lavage system) were evaluated (48–62).
The studies and their outcomes are schematically represented by salivary, nasopharyngeal, and mid-turbinate viral load testing, and their randomized (RCT) or non-randomized controlled trial (non-RCT) designs are shown in Figure 3. Overall, studies in COVID-19 patients report that single-day and repeated SI reduces nasopharyngeal viral loads.
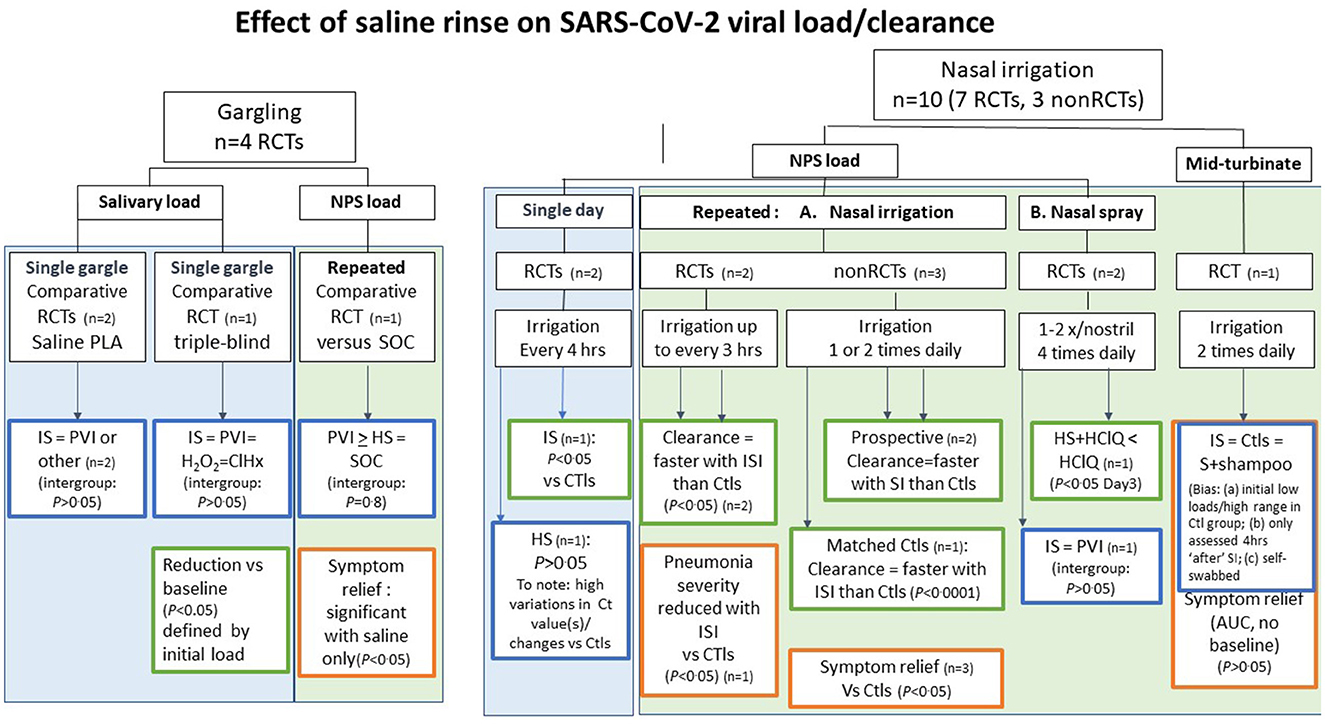
Figure 3. Clinical studies or reports of saline rinse or SI on SARS-CoV-2 viral load and clearance, stratified by method (gargling or nasal irrigation/spray) and the type of viral load (salivary, nasopharyngeal swabbed (NPS), or mid-turbinate): schematic overview of benefit–risk balance assessment. RTC, randomized clinical trial; non-RCT, non-randomized clinical trial; n, number of studies; CTls, controls; IS, isotonic saline (ISI, isotonic saline irrigation); HT, hypertonic saline; HClQ, hydroxychloroquine; PVI, polyvidone iodine; H2O2, hydrogen peroxide; ClHx, chlorhexidine; AUC, area under the curve of cumulative total symptom scores. Significant difference vs. controls or comparator: P < 0.05; non-significant P > 0.05.
3.1.2.1. Single gargle studies (n = 3)
Two studies evaluating the effect of a single gargle (after 5 or 30 min) of an antiseptic mouth rinse vs. a short 30-s 10–20 mL saline gargle (control) did not find significant intergroup differences in mean salivary viral load reductions between saline and the antiseptic mouth rinses (49, 50). In another study, the median salivary viral load wa s reduced by 89% at 15 min after gargling 20 mL of saline for 60 s (90% after 45 min) vs. baseline. The median changes were similar for the three antiseptic comparators varying between 61–89% and 70–97%, respectively, while mean reductions (25–75%) highly fluctuated in values (P > 0.05 upon intergroup comparison) (48). There was, however, a significant correlation between baseline viral load and reduction at 15 min (P = 0.0073) and persistence at 45 min (P = 0.0087) for all mouth rinses tested. In cases where the viral load was <104/mL copies, a 100% reduction was obtained (48).
3.1.2.2. Single-day SI studies
Mean nasopharyngeal viral load in hospitalized COVID-19 pneumonia patients was reduced by 17.3% (median 23.6%) with hypertonic saline after three nasal SIs performed within 6 h while not among the small control group (not reaching significance) (52), while a larger randomized controlled study with 0.9% SI, 10 mL in each nostril every 4 h for 16 h, found a significant reduction in nasopharyngeal viral load at 24 h vs. the control group (P = 0.005) (51).
3.1.2.3. Repeated SI studies
After 3–10 days of oronasal SI, fast(er) viral clearance was observed compared with controls in most studies (51, 54–59). Start of SI immediately after testing cleared the virus in 91% of patients on Day 10 vs. 28% in matched controls not receiving SI (P < 0.0001) (54). Even a delayed start of SI (7 days after testing) also favored its use in a prospective non-RCT (55).
In three RCTs, it was shown that SI or nasal spray added to standard of care (SOC) also cleared viral loads faster in more severe (56) or hospitalized cases (58, 59). In a dose-finding study done in COVID-19 outpatients with pneumonia without ARDS, regular SI (20 mL every 3 h) led to more patients becoming PCR-negative on Day 5 than controls (68 vs. 25%; P < 0.05) (56). As further discussed under Section 3.3.3, this was associated with improvement in SARS-COV-2 pneumonia. Nasal SI resulted in significant earlier clearance in hospitalized patients for Omicron infection (P < 0.05) (59). Hypertonic nasal spray use was found to reduce significantly the nasopharyngeal viral load during the initial treatment days when used add-on to hydroxychloroquine vs. hydroxychloroquine alone (P = 0.019) (58).
3.1.2.4. Salivary and mid-turbinate loads
Salivary or mid-turbinate viral load reductions, obtained with saline nasal spray or SI, were not significantly different between saline and antiseptic comparators (mouth rinse or nasal spray containing polyvidone iodine, chlorhexidine, or detergent) (48–50, 53, 60, 61). Short (15 s) hypertonic saline gargling three times daily did not render mild patients getting faster negative in the PCR test (sampled by nasal and oropharyngeal swabbing) than their controls (median 9 days), despite significant symptom recovery with saline only (Day 4, P < 0.04) (53). These data suggest that oronasal SI or the combination of nasal rinse and gargling may achieve the highest effect.
3.1.3. Viral replication inhibition in vitro
Saline (0.9–1.3% = 150–200 mM) has been reported to reduce viral replication by 50–98% in vitro, thereby reintroducing voltage-gated depolarization in the infected host cells (63). These concentrations also reduce the activity of furin, a human protease, hijacked by SARS-CoV-2 for cleaving the unique furin cleavage site in its spike, thereby priming the spikes for cell fusion (64), and involved in its virulence (65). Furin is highly expressed in salivary glands and present in saliva, and it has been proposed to induce virus pre-activation, which could favor prompt spread through saliva droplets of more infectious virions than those through sneezing and coughing (66, 67). Saline at these salt concentrations also impairs 3CLpro (Mpro), a SARS-CoV-2 protease regulating replication (68). These modest effects may be relevant to combining nasal SI with protease inhibitors to reduce relapse with latter antivirals (62, 69).
3.1.4. Effects on exhaled viral load/bioaerosol
As reviewed (70), NaCl has unique ionic properties leading to droplet/vesicle aggregation, optimal viscosity, and properties of mucus and reduced bioaerosol. Faster hygroscopic growth of iso-/hypertonic bioaerosol and mucus droplets due to saline leads to earlier deposition in nasopharynx/airways, while these particles will be removed continuously from the airways via the mucociliary clearance and be swallowed, and so limits penetration of pathogens in the deeper airway and lungs (71–73). In line with these unique ionic properties of saline, nebulizing saline was found to reduce exhaled droplet numbers in bioaerosol superspreaders (74), while in hospitalized COVID-19 patients, the continuously sampled bioaerosol during 0.9% saline nebulization was found to be devoid of SARS-CoV-2 RNA or live virus: In contrast, nebulization of viral culture, equivalent in titer to the sampled nasopharyngeal load of these patients, resulted in positive bioaerosol samples (75). So, there is no evidence of dissemination by the aerosolizing/nebulizing procedure. It is interesting to note that in a study of 12 hospitalized qPCR-positive patients, nasopharyngeal viral loads (sampled following a 20-mL saline gargle to generate baseline values before H2O2 assessment) were low, with only five in 12 showing loads expected to contain viable virus, while intriguingly upon culturing, only one viable out of the five (76). The relevance of these observations deserves further study, whether due to the sampling method, or saline rinse effects [such as diluting and/or aggregating virus, reducing furin-mediated priming, and/or deactivating virus via salivary myeloperoxidase (see Section 3.2.3)]. Facial mask impregnation with salt/saline has been found to lead to better filtration or deactivation of virus (70, 77).
3.1.5. Reduced transmission and prophylaxis
Overall, publications on the prophylactic use of SI in COVID-19 are limited so far (n = 5). One study relates to SARS-CoV transmission (78), and three sources were related to SASR-CoV-2 transmission, during the initial (79), Alpha-Delta (80), and omicron wave (59), while one more experience was solely reported in the press (therefore not tabulated) (81). One randomized study used local saline brands containing minimal amounts of chlorine (nose rinse spray and mouthwash) in healthcare workers (HCWs) during the first COVID-19 wave (82) (For details, see Supplementary Table S4, p. 17).
An earlier randomized trial assessing SI for common colds reported a 35% reduction in household transmission of respiratory viruses, including human coronavirus, compared with controls (8). In line with this controlled study, a prospective study of SI in COVID-19 patients found limited household contacts testing positive after SI (12.7%) (80); expected rates for within-household transmission average 18.8–27.0% (range 4.6–90%; for the original variant) (83, 84) and 15–35% according to a large UK study (Alpha variants are 50% more infectious than wild-type while 35% less infectious than Delta) (85)–(relevant to this study, run 24 September−21 December 2020).
Successful prophylaxis has been reported in HCWs using SI adjunctive to personal protective equipment (PPE), during initial (79, 82), Delta (81), and Omicron waves (59). This beneficial effect of nasal rinsing was already observed during the first SARS-CoV wave (78). A significant reduction was found in an initial randomized prophylactic study with electrolyzed SI (nose rinses and mouthwashes), resulting in 12% HCWs becoming infected with saline prophylaxis and 12% without (P < 0.05) (82). An Indian press report reported similar protection rates during the Delta wave (no study details available): 1% of HCWs using daily SI became PCR-positive compared with 10% in the wards not using SI (81). A COVID-19 designated hospital in China recently reported zero Omicron infections among HCWs in a large COVID-19 designated hospital in Shenzhen (1,930 COVID-19 patients admitted during Omicron peak), all obliged to use daily SI add-on to strict PPE wear (59).
Indirect support for SI and nebulization in reducing transmission also comes from an ecological regression analysis, finding that highly populated coastal areas with high concentrations of atmospheric salt recorded significantly fewer COVID-19 infections and deaths per capita compared to inland regions by 25–30% (P < 0.05) (86).
3.2. Mucosal NaCl homeostasis and innate immune response
3.2.1. Modulation of ion channels and homeostasis in the mucosa
A commonly overlooked effect of SARS-CoV-2 infection is the impairment of ENaC (sodium channel) activity by SARS-CoV-2 and its S and E proteins, likely due to the virus competing for and so hijacking furin, a ubiquitous human protease activating ENaC (63, 87). They silence the cell membranes, eliminating voltage-gated transmembrane potentials and depolarization, so impairing Na+-influx and fluid reabsorption (63, 87). This leads to a complex dysregulation of many processes requiring salt gradients or ion transport, some of which are discussed below, others are beyond the scope of this article: For instance, ENaC is the driving regulator of airway surface liquid and mucosal hydration, ciliary motion, mucus transport, and reabsorption of airway and alveolar secretions; beyond the respiratory tract, ENaC also (co)regulates taste buds, ACE2, systemic hemodynamic, and immune responses (88–90). As saline re-introduces depolarizations and so restores Na+-influx in infected cells (63), SI may help to modulate or limit ENaC-associated dysfunctional processes in the respiratory mucosa.
3.2.2. Improved mucociliary clearance, mucus, and cough clearance
SARS-CoV-2 infection has been shown to impair MMC in SARS-CoV-infection (70, 91), which often causes nasal obstruction and dry cough (92). Established actions of iso/hypertonic SI in other respiratory diseases include hydration of the periciliary layer and improving MMC and cough clearance (70). These properties may contribute to faster SARS-CoV-2 clearance (see Section 3.1.2.). Saline profoundly changes the viscosity/elasticity of mucus and adhesion/cohesion of mucins, both hydrating and gelling/ thickening these toward easier transportable mucus (93–95). Moreover, the mucus production, stimulated by interferon-aryl hydrocarbon receptor signaling in SARS-CoV-2 infection, would trigger the hypoxia of COVID-19 (96). As mucus plugs (often combined with neutrophil extracellular traps (NETs) in COVID-19, see Section 3.2.3.) create local hypoxic environments that can contribute to enhanced secretion of destructive neutrophil granule contents (97), mucus removal by early SI may help to reduce and prevent nasal and airway obstruction and its deleterious consequences. Postnasal drip, nasal obstruction, cough, and sneeze are relieved by SI in mild-to-moderate COVID-19 (see Section 3.3.1.).
3.2.3. Dampening neutrophil elastase activity
One of the altered immune responses in COVID-19 involves excessive neutrophil activity and NET formation, which is already observed (also nasally) early in the disease (98) and has been implicated in the development of acute lung injury, the induction of the SARS-CoV-2-induced cytokine storm, ARDS, and immuno-thrombosis (99, 100). NETs are composed of decondensed chromatin fibers coated with antimicrobial proteins, such as myeloperoxidase (MPO) and neutrophil elastase, aimed at capturing and neutralizing pathogens to prevent microbial dissemination (99). However, SARS-CoV-2 develops more invasive cell infection and higher pro-inflammatory responses in the presence of neutrophils in vitro (101), while the complex actions of MPO and elastase are thought to contribute to the inflammatory damage in COVID-19 (70, 99, 100, 102). SI may help to clear the adhesive mucus/NETs through the simple action of rinsing the mucosal surfaces, as evidenced by the therapeutic plug removal with saline bronchial lavage or washing in COVID-19 patients (37, 103, 104). Other relevant mechanisms that have been observed with hypertonic SI (>200 mM NaCl; 150 mM = isotonic) in respiratory disease include lowered NET formation and decreases in neutrophil elastase activity, pro-inflammatory cytokines, and reactive oxygen species (ROS) (NaCl ion-specific effects) (105–107).
3.2.4. Local virucidal hypochlorous acid production
Moreover, NaCl has been shown to inhibit the replication of enveloped and non-enveloped DNA and RNA virus in vitro, via the production of virucidal hypochlorous acid (HOCl) by neutrophil phagosomes—considered to be an innate antiviral immune response and involving myeloperoxidase, thereby also providing a mechanism for oxidative NET-mediated microbial killing (70, 108–110). HOCl also controls neutrophil elastase activity and NET release (109, 111), so representing additional mechanisms by which saline may act to limit the illness progression. The role of this innate immune process in the inactivation of the virus [a study finding low infectivity of saline gargles from COVID-19 patients (76)] deserves further study, as myeloperoxidase activity is markedly increased in saliva and lungs of SARS-CoV-2 infected patients (112).
3.3. Clinical benefits of SI in COVID-19
Twenty-one eligible clinical (mainly small) studies were identified through PubMed and broad Internet searches (some reviewed for viral load under Section 3.1.2) (53–62, 80, 92, 113–124). They are listed in Supplementary Table S4, p. 17–29. As illustrated in Figure 4, these studies used ample-volume (oro)nasal SI (n = 8), repetitive saline sprays or drops (sometimes as placebo) (n = 8), gargling (n = 1) or nebulization (n = 3), spanning various study designs, disease severity, irrigants or nasal spray/drop comparators, and outcomes. As many studies were small and study samples were not powered to detect differences in outcomes on COVID-19-related risks, such as pneumonia, hospitalization, ICU admission, invasive oxygen need/ventilation, and mortality, the latter results are consolidated in Supplementary Table S5, p. 30.
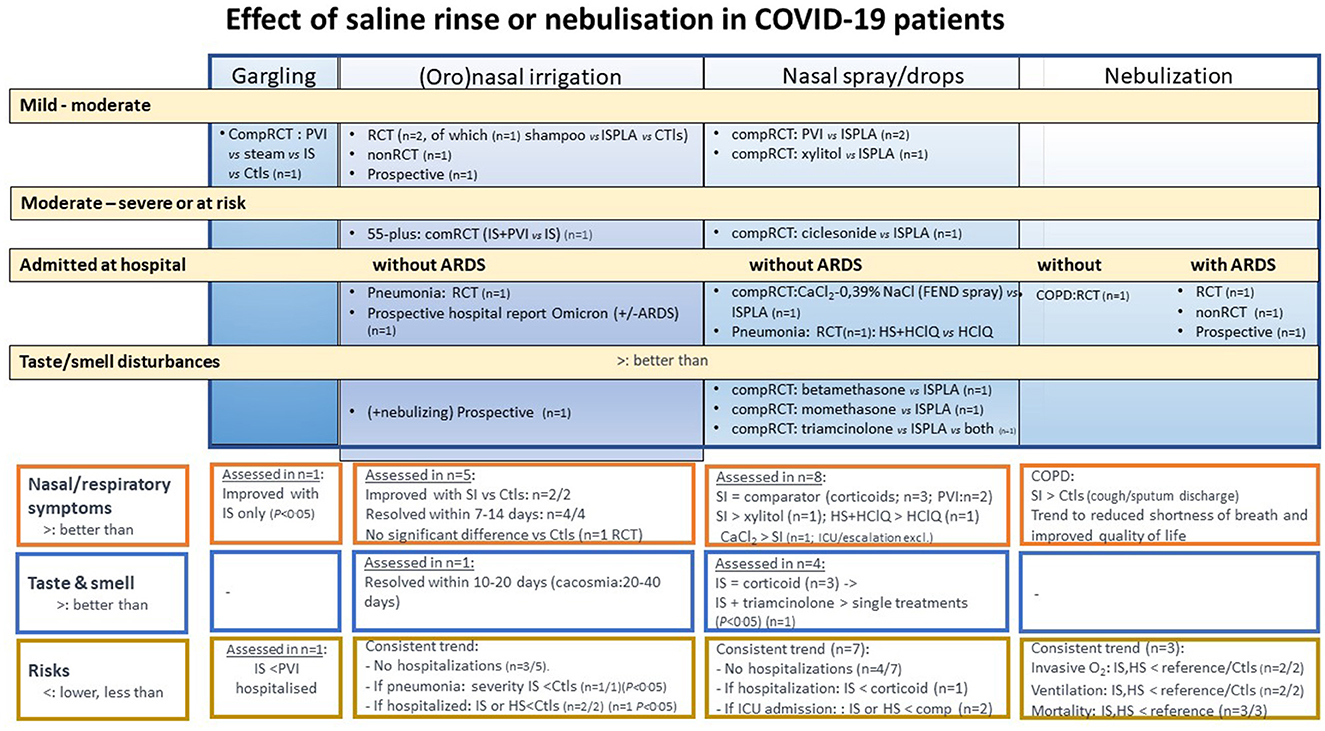
Figure 4. Effect of saline rinse or nebulization in COVID-19 patients, stratified by disease severity and type of rinse/nebulization: schematic overview of benefit–risk balance assessment. See also Figure 3; comp, comparative; O2, oxygen; IS, isotonic saline; ISPLA, isotonic saline as placebo; HS, hypertonic saline; ARDS, acute respiratory distress syndrome; COPD, chronic obstructive pulmonary disease. Meaning of symbols: (A) respiratory symptoms (orange framed outcome) and (B) taste/smell disturbances (blue framed outcomes): “=”, effect comparable between treatment groups; “>”, generally better outcome with saline than with Ctls or comparator; “<”, comparator doing better than saline placebo; (C) risks: <, rate or severity outcome lower with saline than Ctls, comparator or reference population.
3.3.1. Respiratory symptom reduction
In a non-randomized case-controlled study, newly diagnosed COVID-19 patients, starting immediately isotonic SI once daily after PCR testing, experienced significantly more nasal symptom relief than controls (p < 0.05) (54). In addition, other studies comparing to controls have reported significant symptom recovery, reduction of postnasal drip, nasal obstruction, cough, or sneeze (p < 0.05) (53, 113, 114, 116, 121).
In a prospective study, PCR-positive patients older than 55 years (mean age 65 years), mostly with high BMI, were assigned to two different pressurized SI techniques (80). COVID-19 symptoms (loss of smell or taste, fatigue, fever >100.4°F, chills, muscle aches, runny nose, new onset (or worsening of chronic) cough, shortness of breath, nausea or vomiting, headache, abdominal pain, and diarrhea) were resolved after 14 days of SI in 63 of 71 participants (one hospitalization, see Section 3.3.3); symptom resolution was more likely for those reporting twice daily SI than once (P = 0.0032) (80).
Studies with nasal spray or drops comparing symptom relief with saline (as placebo) and various treatments (polyvidone iodine, calcium chloride, and ciclesonide) have not reported the superiority of SI, though they have shown symptomatic improvement consistent with the comparators (see Supplementary Table S4, p. 20_22) (53, 60, 80, 92, 115).
3.3.2. Smell and taste recovery
Symptoms of smell or taste dysfunction in COVID-19 patients may take 10–40 days to resolve with SI (54, 61, 80, 113, 116–120). For specific studies in COVID-19 patients with smell and taste disturbances, see Supplementary Table S4, p. 23 (studies in long-COVID-19 patients were excluded). Overall SI and nasal steroids were found to perform equally well (no consistent statistically significant differences) (118–120). Yet, a significantly faster resolution was obtained with the combination of SI and triamcinolone, a finding that needs confirmation in larger clinical studies, while at best also evaluating the impact of frequency of SI (120).
3.3.3. Hospitalization and intensive care risks
3.3.3.1. SI or spray use
Although not set as primary outcomes, except for two studies (80, 113), many studies using SI or saline spray reported on the rate of hospitalizations, some ICU outcomes, and mortality. Overall, the studies were too small to detect significant differences. For tabulation of these outcomes, see Supplementary Table S5, p. 30.
If hospitalizations were observed, rates were generally lower with the saline formulations than with controls or comparators. Relevant to patients aged 55 or more years, Baxter et al., assessing hospitalization as a primary parameter, recorded one hospitalization [1/78 (1.3%)] (no deaths) with isotonic SI in this age group with high BMI: These rates were 9.5% (plus 1.5% deaths) in a nested control population over 50 years, over the same period (80). Another study in COVID-19 patients during the first wave found unexplained high hospitalization rates in both groups, yet lower with SI users, but not significantly reduced vs. controls (P = 0.66), while there was no high adherence to the rinse procedure (113).
In line with faster clearance of viral loads and so reduced risk of micro-aspiration from nose to lungs (see Section 3.1.1.), patients with COVID-19 pneumonia (without ARDS) experienced a significant reduction in computed tomogram-evaluated pneumonia severity score after 5 days of isotonic SI compared with controls (P < 0.05); also the percent of patients worsening (8.8%) was lower when compared with controls (36.4%; P = 0.028) (56). In line with these results, another randomized study of isotonic SI, initiated in hospitalized COVID-19 patients and leading to faster viral clearance Day 2 (P < 0.02), suggests that rapid initiation of SI following a positive PCR test may help to lower subsequent need for oxygen administration, ICU admission, and mortality, although the differences did not reach statistical significance between the small patient groups (n = 25 per group), and a potential bias may have incurred by the average older age of the control group (51).
3.3.3.2. Nebulization
Two prospective and one randomized hospital studies used nebulized saline adjunctive to SOC (122–124). In patients with ARDS, saline nebulization (+ibuprofenate) (123) was associated with improved respiratory rate (123), low need for invasive oxygenation or intubation (122, 123), and low(er) mortality (compared to controls) (123, 124). These findings are in line with observations in patients with bronchiolitis and other respiratory diseases, in whom iso- or hypertonic saline has been shown to improve oxygen saturation (125, 126).
In a four-arm study in ventilated patients with ARDS comparing two solutions vs. saline 0.9% and controls (twice daily), methicillin-resistant Staphylococcus aureus incidence significantly decreased with the nebulizing treatments vs. controls (12% for saline vs. 34.6% among controls; P < 0.05); mortality was lowest among the patients receiving nebulized saline (40.5 vs. 59.6% among controls; not significant) (124).
In line with the findings on bioaerosol, following saline nebulization in hospitalized COVID-19 patients (75) (reported in Section 3.1.4), nebulization was reported not to lead to transmission or spread of the virus among HCWs (122).
In conclusion, studies in COVID-19 patients have consistently reported safe use and outcomes favoring SI use in COVID-19, whether it was used as sole intervention initiated immediately after positive PCR testing in mild-to-moderate COVID-19, or as part of the patient treatment protocol adjunctive to SOC in an ambulatory setting or upon hospitalization. The results require confirmation in large, randomized studies.
3.4. Patient satisfaction and risk
This review found that SI is accepted and well-tolerated in all studies reported. Nasal burning or discomfort was reported consistent with other reports and clinical practice. These can often be ameliorated by reducing salinity and technique adjustment. Epistaxis is rare and was not reported in reviewed studies, except in older individuals (80). Nasal SI requires the use of clean water, as rare infection, and death with N. fowleri has been reported (127).
Concerns for SI affecting the blood pressure adversely have not proven to be a problem, as has been evidenced in patients with chronic lung disease, also at hypertonic concentration (128, 129). Patients should be advised not to swallow saline drip or gargles but to spit these out safely in a sink or receptacle.
Daily use of repeated SI may show lower compliance in vulnerable patients, such as those admitted in care homes with COVID-19. This challenge can be overcome by motivating home residents and staff to apply SI and survey the significant reduction in respiratory complications (130). SI materials (Neti pot and squeeze bottle) should be cleaned daily. Routine precautions for limiting the COVID-19 spread remain essential.
4. Discussion and lessons learned
While it is acknowledged that a short 30-s contact of saline with the SARS-CoV-2 virus does not exhibit direct virucidal activity (131), this narrative review on SI revealed that nasal SI performed repeatedly for 1 day may lead to a significant reduction in nasopharyngeal virus load, while a repeated application for 7–10 days leads to faster viral clearance. Overall, patients with positive swab tests experienced substantial viral load reduction, became PCR-negative faster, and had milder symptoms than untreated patients. Hence, repeated application of SI can be recommended for fastening viral clearance, symptom relief, and prophylaxis against respiratory infection, as has been increasingly proposed in (self-care) treatment or hygiene prevention guides for COVID-19 (9–13, 15, 59, 70, 79, 132–134).
Albeit found to significantly relieve symptoms in the study (53), and in line with pathophysiology (infection starting in the ciliated nasopharynx), short daily hypertonic saline gargling in COVID-19 patients did not fasten the SAR-CoV-2 nasopharyngeal clearance. In view of the low rate of live virus in gargle samples so far assessed in one study (76), larger-scale, well-validated studies are desirable to appreciate its potential role in reducing salivary loads and transmissibility in patients with COVID-19.
In the studies reviewed, both isotonic and hypertonic SI (1.5–3%) were used and found to be safe and effective for nose and throat irrigation in COVID-19, when administered with mildly pressurized flasks or devices, using protocols similar to those established in other respiratory diseases (5–13). Regular daily application of SI volumes, twice daily up to every 3 h rather than once daily, may enhance outcomes, as was observed in COVID-19 with comorbidity (80) or pneumonia (56).
Our evaluation of the mechanisms of saline and clinical studies suggests that saline may act more than placebo, similarly as previously proposed in other respiratory conditions (135, 136). This action may deserve attention in studies of nasal steroids, using saline drops or spray as comparator: A recent Cochrane analysis on the prevention of olfactory dysfunction was unable to conclude their efficacy (137). Whether combinations of saline with anti-inflammatory agents or corticosteroids provide extra-benefits over saline alone needs further study.
4.1. Additives
Overall, our data did not indicate a rationale for (antiseptic) additives to oral or nasal saline for daily oronasal hygiene (no significant intergroup differences) (46, 48–50, 53, 60, 61, 80). Possibly, the effect of additives may diminish fast due to salivary clearance (138). Many substances have been proposed for the development of nasal sprays or mouth rinses in COVID-19 (139). Bias through direct interaction with the PCR test by the additive should be excluded (see Supplementary Appendix A, p. 05, point 4). This is not a problem with saline which, in contrast, is used as a common PCR test sampling fluid.
4.2. Controversies and risks
Contrary to concerns that SI may cause harm through postnasal drip or contamination from nose to throat, respiratory tract, or olfactory bulb through viral movement or micro-aspiration (140–143), this review does not find evidence of such a risk. Moreover, this review did not find evidence for an increased hazard to contaminate or transmit SARS-CoV-2 through the use of nebulized saline for COVID-19, in the context of healthcare or household settings. The findings that viable virus is absent in (exhaled) bioaerosol during saline nebulization (75), and that transmission was not a problem during the clinical experiences with inhaled saline (121–124), support the guidance of authorities that encourage nebulization to be used or continued during COVID-19 (16). They also make nebulization of saline an acceptable alternative in (older) patients unable to perform SI. In Germany, pneumologists have acknowledged the effects of SI on bioaerosol since the start of the pandemic (132) and have encouraged patients to nebulize saline solution against COVID-19 (144). So, the use of SI or nebulization in the hospital or at home should not be discouraged, and this synthesis may help to inform authorities to adapt and/or adopt this hygienic measure.
Although data on SI for COVID-19 are far from complete, the results of this review show that there is solid translational evidence that for COVID-19, SI is unlikely to harm and likely to help. It is, therefore, reasonable, indeed ethically necessary, for authors of published and online news or recommendations on saline to amend erroneous claims suggesting harm for its use in COVID-19, for example (13, 140–143). The WHO has already taken this step by deleting SI from the Mythbusters webpage (14).
4.3. Limitations and developments needed
Most studies were small and of substantial heterogeneity, so bias cannot be excluded. However, given the overall robust in vitro and in vivo effects of nasal and oral SI, and the emerging evidence from observational and randomized studies (irrespective of saline being the object or reference/placebo), both health authority support and institutional funding are needed to design and perform large, randomized trials to formally test SI when implemented as an adjunctive preventive and treatment modality for COVID-19.
Yet, as SI use appears to be safe and is already practiced for common colds and viral upper respiratory infections (5–8), current evidence suggests that SI can be recommended as a public health measure along with other routine hygiene measures, albeit with strict attention to clean water and washing of SI materials. This is corroborated by experience with SI in COVID-19 in a hospital in China (59) and by a recent consensus of Chinese experts in otorhinolaryngology (15).
4.4. Practical recommendations
As an efficacious measure particularly if early started in COVID-19, SI can be effectively implemented at every age in those with positive SARS-CoV-2 test. We recommend its regular use as needed, up to 2–3 days after resolving symptoms, with a minimum of 10 days of repetitive saline nasal irrigation and gargling and repeated up to every 3 h in case of moderate COVID-19/pneumonia. Ideally, nasal irrigation and gargling are combined, as both nose and throat can be affected, and are started as soon as common symptoms develop and/or COVID-19 positivity is diagnosed. Larger well-standardized, randomized research trials may help to determine optimal volume, pressure, and salinity. Based on our analysis and the broader Chinese hands-on experience (15), any concentration between 0.9 and 3.0% appears to be suitable, while large, repeated volumes are preferred. For prophylaxis, several protocols have been suggested for use as part of the post-exposure or preventive protocol (15, 59, 70, 79), which can be all-inclusive (washing hands/face and saline eye rinse, nasal irrigation, and gargling), thereby aiming at covering all sites of viral entry or attachment (79). For video instructions how to make or apply SI at no cost, three links to video instructions are found under Supplementary Table S4.
5. Conclusion
Overall, substantial evidence was found in vitro and in vivo in support of SI use in COVID-19, also emerging from observational and randomized studies irrespectively of saline being the main intervention or reference/placebo in the study. As an international network of investigators with substantial clinical and research experience in saline use for several respiratory diseases and SARS-CoV-2 infections, we, therefore, propose to include rinsing the nose and throat with saline as an early intervention to prevent, and relieve symptoms of, SARS-CoV-2 infection: It is easy-to-use, inexpensive, safe, and feasible at every age. Considering the current high SARS-CoV-2 mutation rate and COVID-19 waves less responsive to population-based immunization, the recommendation to use nasal and oral saline irrigation is adjunctive to current biologicals and therapeutics, as well as to protective interventions, such as mask wear, social distancing, and hand hygiene. We also advocate for health authorities to dedicate further research funding to further clarify the preventive and therapeutic role of saline irrigation for SARS-CoV-2 infections at a global level.
Author contributions
All authors of this Pro Bono Initiative, started by informal contacts per e-mail and Zoom, had pharmacological and/or clinical experience with saline irrigation in COVID-19 or related respiratory conditions. All the authors contributed by their knowledge, specific (pre)clinical research, or experiences (see reference list). Manuscript development occurred through the submission of work or description and discussion of mechanisms by e-mail and by Zoom meetings inviting all network members and coordinated by SH, SP, and DR. For completeness of the review, continuous searches for additional data were performed and new data were circulated by SH, while interpretations and problem-solving, development of lessons learned, and recommendations occurred through e-mail and discussion at the regular Zoom meetings. Integration of changes in the manuscript and revisions thereof were coordinated by SH, while regular feedback by e-mail to the Network and recirculation of the manuscript. All authors contributed to the development of consolidated knowledge, conception, and/or interpretation and helped in critical revision and resolving questions at each circulation until final consensus for submission. All authors listed have made a substantial, direct, and intellectual contribution to the work and approved it for publication.
Conflict of interest
The authors declare that the research was conducted in the absence of any commercial or financial relationships that could be construed as a potential conflict of interest.
Publisher's note
All claims expressed in this article are solely those of the authors and do not necessarily represent those of their affiliated organizations, or those of the publisher, the editors and the reviewers. Any product that may be evaluated in this article, or claim that may be made by its manufacturer, is not guaranteed or endorsed by the publisher.
Supplementary material
The Supplementary Material for this article can be found online at: https://www.frontiersin.org/articles/10.3389/fpubh.2023.1161881/full#supplementary-material
References
1. Sachs JD, Karim SSA, Aknin L, Allen J, Brosbøl K, Colombo F, et al. The Lancet Commission on lessons for the future from the COVID-19 pandemic. Lancet. (2022) 400:1224–80. doi: 10.1016/S0140-6736(22)01585-9
2. Hewins B, Rahman M, Bermejo-Martin JF, Kelvin AA, Richardson CD, Rubino S, et al. Alpha, Beta, Delta, Omicron, and SARS-CoV-2 breakthrough cases: defining immunological mechanisms for vaccine waning and vaccine-variant mismatch. Review article. Front Virol. (2022) 2:849936. doi: 10.3389/fviro.2022.849936
3. Uraki R, Ito M, Furusawa Y, Yamayoshi S, Iwatsuki-Horimoto K, Adachi E, et al. Humoral immune evasion of the omicron subvariants BQ11 and XBB. Lancet Infect Dis. (2023) 23:30–2. doi: 10.1016/S1473-3099(22)00816-7
4. DTB team. Drug review. Two new oral antivirals for covid-19: ▾ molnupiravir and ▾ nirmatrelvir plus ritonavir. New Drug Ther Bull. (2022) 60:73−7. doi: 10.1136/dtb.2022.000008
5. International Pharmaceutical Federation (FIP). Cold, Flu Sinusitis. Managing Symptoms Supporting Self-care. A Handbook for Pharmacists. (2021). Available online at: https://www.fip.org/file/5089 (sccessed November 5, 2022).
6. Tano L, Tano K. A daily nasal spray with saline prevents symptoms of rhinits. Acta Otolaryngol. (2004) 124:1–4 doi: 10.1080/00016480410017657
7. Ao H, Wang Q, Jiang B, He P. Efficacy and mechanism of nasal irrigation with a hand pump against influenza and non-influenza viral upper respiratory tract infection. J Infect Dis Immun. (2011) 3:96-105.
8. Ramalingam S, Graham C, Dove J, Morrice L, Sheikh A. Hypertonic saline nasal irrigation and gargling should be considered as a treatment option for COVID-19. J Glob Health. (2020) 10:010332. doi: 10.7189/jogh.10.010332
9. Panta P, Chatti K, Andhavarapu A. Do saline water gargling and nasal irrigation confer protection against COVID-19? Explore. (2021) 17:127–9. doi: 10.1016/j.explore.2020.09.010
10. Borah A, Goswami A. Nasal irrigation in Covid-19 pandemic: is it justified? IOSR JDMS. (2020) 19:19–21. doi: 10.9790/0853-1906071921
11. Khan FR. Simple public health interventions such as use of hypertonic saline oral and nasal rinse could be beneficial in COVID-19 patients. J Pak Dent Assoc. (2020) 29(suppl.):S4-S5. doi: 10.25301/JPDA.29S.S4
12. Farrell NF, Klatt-Cromwell C, Schneider JS. Benefits and safety of nasal saline irrigations in a pandemic-washing COVID-19 away. JAMA Otolaryngol Head Neck Sur. (2020) 146:787–8. doi: 10.1001/jamaoto.2020.1622
13. Panta P, Baruah B, Patil S. Hypertonic saline use and traditional nasal irrigation: a possible preventative measure against COVID-19. J Contemp Dent Pract. (2022) 23:379-82. doi: 10.5005/jp-journals-10024-3278
14. World Health Organization. Coronavirus Disease (COVID-19) Advice for the Public: Mythbuster. (2022). Available online at: https://www.who.int/emergencies/diseases/novel-coronavirus-2019/advice-for-public/myth-busters (accessed September 29, 2022).
15. Yu S, Yang Y, Xu Y, Meng J, Zuo K, Wang H, et al. Chinese Rhinopathy Research Cooperation Group. Expert consensus on nasal saline irrigation for the prevention of SARS-CoV-2 infection. Chin J Ophthalmol Otorhinolaryngol. (2022) 22:329–34.
16. Cazzola M, Ora J, Bianco A, Rogliani P, Matera MG. Guidance on nebulization during the current COVID-19 pandemic. Respir Med. (2021) 176:106236. doi: 10.1016/j.rmed.2020.106236
17. Ahn JH, Kim J, Hong SP, Choi SY, Yang MJ, Ju YS, et al. Nasal ciliated cells are primary targets for SARS-CoV-2 replication in early stage of COVID-19. J Clin Investigation. (2021) 131:1–4. doi: 10.1172/JCI148517
18. Pizzorno A, Padey B, Julien T, Trouillet-Assant S, Traversier A, Errazuriz-Cerda E, et al. Characterization and treatment of SARS-CoV-2 in nasal and bronchial human airway epithelia. Cell Rep Med. (2020) 1:100059. doi: 10.1016/j.xcrm.2020.100059
19. Sungnak W, Huang N, Bécavin C, Berg M, Queen R, Litvinukova M, et al. SARS-CoV-2 entry factors are highly expressed in nasal epithelial cells together with innate immune genes. Nature Med. (2020) 26:681-7. doi: 10.1038/s41591-020-0868-6
20. Hou Y, Zhao J, Martin W, Kallianpur A, Chung MK, Jehi L, et al. New insights into genetic susceptibility of COVID-19: an ACE2 and TMPRSS2 polymorphism analysis. BMC Med. (2020) 18:216. doi: 10.1186/s12916-020-01673-z
21. Bridges JP, Vladar EK, Huang H, Mason RJ. Respiratory epithelial cell responses to SARS-CoV-2 in COVID-19. Thorax. (2022) 77:203-9. doi: 10.1136/thoraxjnl-2021-217561
22. Chatterjee US. Multisystem inflammatory syndrome in children: how to live with COVID-19? J Indian Assoc Pediatr Surg. (2022) 27:659-60. doi: 10.4103/jiaps.jiaps_18_22
23. Cheemarla NR, Watkins TA, Mihaylova VT, Wang B, Zhao D, Wang G, et al. Dynamic innate immune response determines susceptibility to SARS-CoV-2 infection and early replication kinetics. J Exp Med. (2021) 218:e20210583. doi: 10.1084/jem.20210583
24. Liu Y, Qu HQ, Qu J, Tian L, Hakonarson H. Expression pattern of the SARS-CoV-2 entry genes ACE2 and TMPRSS2 in the respiratory tract. Viruses. (2020) 12:1174. doi: 10.3390/v12101174
25. Gómez-Carballa A, Rivero-Calle I, Pardo-Seco J, Gómez-Rial J, Rivero-Velasco C, Rodríguez-Núñez N, et al. A multi-tissue study of immune gene expression profiling highlights the key role of the nasal epithelium in COVID-19 severity. Environ Res. (2022) 210:112890. doi: 10.1016/j.envres.2022.112890
26. Kato T, Asakura T, Edwards CE, Dang H, Mikami Y, Okuda K, et al. Prevalence and mechanisms of mucus accumulation in COVID-19 lung disease. Am J Respir Crit Care Med. (2022) 206:1336–52. doi: 10.1164/rccm.202111-2606OC
27. Monel B, Planas D, Grzelak L, Smith N, Robillard N, Staropoli I, et al. Release of infectious virus and cytokines in nasopharyngeal swabs from individuals infected with non-alpha or alpha SARS-CoV-2 variants: an observational retrospective study. EBioMedicine. (2021) 73:103637. doi: 10.1016/j.ebiom.2021.103637
28. Liu R, Yi S, Zhang J, Lv Z, Zhu C, Zhang Y. Viral load dynamics in sputum and nasopharyngeal swab in patients with COVID-19. J Dent Res. (2020) 99:1239-44. doi: 10.1177/0022034520946251
29. Huang Y, Chen S, Yang Z, Guan W, Liu D, Lin Z, et al. SARS-CoV-2 viral load in clinical samples from critically ill patients. Am J Respir Crit Care Med. (2020) 201:1435-38. doi: 10.1164/rccm.202003-0572LE
30. Zou L, Ruan F, Huang M, Liang L, Huang H, Hong Z, et al. SARS-CoV-2 viral load in upper respiratory specimens of infected patients. N Engl J Med. (2020) 382:1177-79. doi: 10.1056/NEJMc2001737
31. Ryan KA, Bewley KR, Fotheringham SA, Slack GS, Brown P, Hall Y, et al. Dose-dependent response to infection with SARS-CoV-2 in the ferret model and evidence of protective immunity. Nat Commun. (2021) 12:81. doi: 10.1038/s41467-020-20439-y
32. Kraus A, Huertas M, Ellis L, Boudinot P, Levraud JP, Salinas I. Intranasal delivery of SARS-CoV-2 spike protein is sufficient to cause olfactory damage, inflammation and olfactory dysfunction in zebrafish. Brain Behav Immun. (2022) 102:341–59. doi: 10.1016/j.bbi.2022.03.006
33. Gao C, Zeng J, Jia N, Stavenhagen K, Matsumoto Y, Zhang H, et al. SARS-CoV-2 spike protein interacts with multiple innate immune receptors. bioRxiv. (2020). doi: 10.1101/2020.07.29.227462
34. Deinhardt-Emmer S, Böttcher S, Häring C, Giebeler L, Henke A, Zell R, et al. SARS-CoV-2 causes severe epithelial inflammation and barrier dysfunction. J Virol. (2021) 95:e00110–21. doi: 10.1128/JVI.00110-21
35. Wang C, Kang K, Lan X, Fei D, Wang Q, Li X, et al. Cytokine levels in sputum, not serum, may be more helpful for indicating the damage in the lung and the prognosis of severe COVID-19 - A case series. J Infect. (2021) 83:e6–9. doi: 10.1016/j.jinf.2021.08.026
36. Hasan A, Lange CF, King ML. Effect of artificial mucus properties on the characteristics of airborne bioaerosol droplets generated during simulated coughing. J Nonnewt Fluid Mech. (2021) 165:1431–41. doi: 10.1016/j.jnnfm.2010.07.005
37. Baron A, Hachem M, Tran Van Nhieu J, Botterel F, Fourati S, Carteaux G, et al. Bronchoalveolar lavage in patients with COVID-19 with invasive mechanical ventilation for acute respiratory distress syndrome. Ann Am Thorac Soc. (2021) 18:723-6. doi: 10.1513/AnnalsATS.202007-868RL
38. Torrego A, Pajares V, Fernández-Arias C, Vera P, Mancebo J. Bronchoscopy in patients with COVID-19 with invasive mechanical ventilation: a single-center experience. Am J Respir Crit Care Med. (2020) 202:284-7. doi: 10.1164/rccm.202004-0945LE
39. Suranadi IW, Sucandra IMAK, Fatmawati NND, Wisnawa ADF. A retrospective analysis of the bacterial infections, antibiotic use, and mortality predictors of COVID-19 patients. Int J Gen Med. (2022) 15:3591-603. doi: 10.2147/IJGM.S351180
40. Waring S, Gamtkitsulashvili G, Kumar S, Narayan Y, D'Souza A, Jiwani S, et al. Impact of empirical antibiotic use in patients with COVID-19 on morbidity and mortality during the first and second UK SARS-CoV-2 waves. Thorax. (2021) 76(Suppl. 2):A36-7. doi: 10.1136/thorax-2021-BTSabstracts.59
41. De R, Dutta S. Role of the microbiome in the pathogenesis of COVID-19. Review. Front Cell Infect Microbiol. (2022) 31:736397. doi: 10.3389/fcimb.2022.736397
42. Liu Y, Yan LM, Wan L, Xiang TX, Le A, Liu JM, et al. Viral dynamics in mild and severe cases of COVID-19. Lancet Infect Dis. (2020) 20:656-7. doi: 10.1016/S1473-3099(20)30232-2
43. Fajnzylber J, Regan J, Coxen K, Corry H, Wong C, Rosenthal A, et al. SARS-CoV-2 viral load is associated with increased disease severity and mortality. Nat Commun. (2020) 11:5493. doi: 10.1038/s41467-020-19057-5
44. Kawasuji H, Takegoshi Y, Kaneda M, Ueno A, Miyajima Y, Kawago K, et al. Transmissibility of COVID-19 depends on the viral load around onset in adult and symptomatic patients. PLoS ONE. (2020) 15:e0243597. doi: 10.1371/journal.pone.0243597
45. Rabago D, Zgierska A. Saline nasal irrigation for upper respiratory conditions. Am Fam Phys. (2009) 80:1117–9.
46. Liu CM, Kohanski MA, Mendiola M, Soldanova K, Dwan MG, Lester R, et al. Impact of saline irrigation and topical corticosteroids on the postsurgical sinonasal microbiota. Int Forum Allergy Rhinol. (2015) 5:185-90. doi: 10.1002/alr.21467
47. Huang N, Pérez P, Kato T, Mikami Y, Okuda K, Gilmore RC, et al. SARS-CoV-2 infection of the oral cavity and saliva. Nat Med. (2021) 27:892-903. doi: 10.1038/s41591-021-01296-8
48. Chaudhary P, Melkonyan A, Meethil A, Saraswat S, Hall DL, Cottle J, et al. Estimating salivary carriage of severe acute respiratory syndrome coronavirus 2 in nonsymptomatic people and efficacy of mouthrinse in reducing viral load: a randomized controlled trial. J Am Dent Assoc. (2021) 152:903-8. doi: 10.1016/j.adaj.2021.05.021
49. Natto ZS, Bakhrebah MA, Afeef M, Al-Harbi S, Nassar MS, Alhetheel AF, et al. The short-term effect of different chlorhexidine forms versus povidone iodine mouth rinse in minimizing the oral SARS-CoV-2 viral load: An open label randomized controlled clinical trial study. Medicine. (2022) 101:e28925. doi: 10.1097/MD.0000000000028925
50. Sevinç Gül SN, Dilsiz A, Saglik I, Aydin NN. Effect of oral antiseptics on the viral load of SARS-CoV-2: a randomized controlled trial. Dent Med Probl. (2022) 59:357–63. doi: 10.17219/dmp/150831
51. Pantazopoulos I, Chalkias A, Mavrovounis G, Dimeas I, Sinis S, Miziou A, et al. Nasopharyngeal wash with normal saline decreases SARS-CoV-2 viral load: a randomized pilot-controlled trial. Can Respir J. (2022) 2022:8794127. doi: 10.1155/2022/8794127
52. Vantarakis A, Velissaris D, Kotsalou C, Oikonomou E, Paraskevas T, Poulas K. First report of reduced severe acute respiratory syndrome coronavirus 2 viral load after nasopharyngeal wash with hypertonic water. Qeios. (2020). doi: 10.32388/FIU5K3
53. Chalageri VH, Bhushan S, Saraswathi S, Ranganath TS, Rani VD, Majgi SM, et al. Impact of steam inhalation, saline gargling, and povidone-iodine gargling on clinical outcome of COVID-19 patients in Bengaluru, Karnataka: a randomized control trial. Indian J Community Med. (2022) 47:207–12. doi: 10.4103/ijcm.ijcm_804_21
54. Spinato G, Fabbris C, Costantini G, Conte F, Scotton PG, Cinetto F, et al. The effect of isotonic saline nasal lavages in improving symptoms in SARS-CoV-2 infection: A Case-Control Study. Front Neurol. (2021) 12:794471. doi: 10.3389/fneur.2021.794471
55. Ciprandi G, La Mantia I, Brunese FP, Varricchio A, Varricchio A. Hypertonic saline with xylitol and hyaluronate may shorten the viral shedding duration in asymptomatic COVID-19 positive subjects: a pilot study. J Biol Regul Homeost Agents. (2021) 35:1151-4. doi: 10.23812/21-138-L
56. Chatterjee U, Chakraborty A, Naskar S, Saha B, Bandyapadhyay B, Shee S. Efficacy of normal saline nasal spray and gargle on SARS-CoV-2 for prevention of COVID-19 pneumonia. Res Square. (2021). PPR277214. doi: 10.21203/rs.3.rs-153598/v2
57. Varricchio A, La Mantia I, Brunese FP, Varricchio A, Ciprandi G. Viral shedding in symptomatic patients with mild COVID-19: an experience with nebulized nasal treatment. J Biol Regul Homeost Agents. (2021) 35:1155-7. doi: 10.23812/21-137-L
58. Yilmaz YZ, Yilmaz BB, Ozdemir YE, Kocazeybek BS, Karaali R, Çakan D, et al. Effects of hypertonic alkaline nasal irrigation on COVID-19. Laryngoscope Investig Otolaryngol. (2021) 6:1240–7. doi: 10.1002/lio2.686
59. Cao J, Wen M, Shi Y, Huang T, Yi Y, Su Y, et al. How should designated COVID-19 hospitals in megacities implement a precise management strategy in response to Omicron? Biosci Trends. (2022) 16:242–4. doi: 10.5582/bst.2022.01261
60. Zarabanda D, Vukkadala N, Phillips KM, Qian ZJ, Mfuh KO, Hatter MJ, et al. The effect of povidone-iodine nasal spray on nasopharyngeal SARS-CoV-2 viral load: a randomized control trial. Laryngoscope. (2021) 132:2089–95. doi: 10.1002/lary.29935
61. Esther CR Jr, Kimura KS, Mikami Y, Edwards CE, Das SR, Freeman MH, et al. Pharmacokinetic-based failure of a detergent virucidal for severe acute respiratory syndrome-coronavirus-2 (SARS-CoV-2) nasal infections: a preclinical study and randomized controlled trial. Int Forum Allergy Rhinol. (2022) 12:1137–47. doi: 10.1002/alr.22975
62. Zou R, Peng L, Shu D, Zhao L, Lan J, Tan G, et al. Antiviral efficacy and safety of molnupiravir against Omicron variant infection: a randomized controlled clinical trial. Front Pharmacol. (2022) 13:939573. doi: 10.3389/fphar.2022.939573
63. Machado RRG, Glaser T, Araucho DB, Petiz LL, Oliveira DBL, Durigon GS, et al. Inhibition of severe acute respiratory syndrome Coronavirus 2 replication by hypertonic saline solution in lung and kidney epithelial cells. ACS Pharmacol Transl Sci. (2021) 4-5:1527. doi: 10.1021/acsptsci.1c00080
64. Li C, Li AW. Hypertonic saline and aprotinin inhibit furin and nasal protease to reduce SARS-CoV-2 specific furin site cleavage activity. J Explor Res Pharmacol. (2022) 7:208–14. doi: 10.14218/JERP.2021.00058
65. Johnson BA, Xie X, Bailey AL, Kalveram B, Lokugamage KG, Muruato A, et al. Loss of furin cleavage site attenuates SARS-CoV-2 pathogenesis. Nature. (2021) 591:293–9. doi: 10.1038/s41586-021-03237-4
66. Zupin L, Pascolo L, Crovella S. Is FURIN gene expression in salivary glands related to SARS-CoV-2 infectivity through saliva? J Clin Pathol. (2021) 74:209–11. doi: 10.1136/jclinpath-2020-206788
67. Sakaguchi W, Kubota N, Shimizu T, Saruta J, Fuchida S, Kawata A, et al. Existence of SARS-CoV-2 entry molecules in the oral cavity. Int J Mol Sci. (2020) 21:6000. doi: 10.3390/ijms21176000
68. Legare S, Heide F, Bailey-Elkin BA, Stetefeld J. Improved SARS-CoV-2 main protease high-throughput screening assay using a 5-carboxyfluorescein substrate. J Biol Chem. (2022) 298:101739. doi: 10.1016/j.jbc.2022.101739
69. Wang L, Volkow ND, Davis PB, Berger NA, Kaelber DC, Xu R. COVID-19 rebound after Paxlovid treatment during Omicron BA.5 vs BA.2.12.1 subvariant predominance period. medRxiv [preprint]. (2022) doi: 10.1101/2022.08.04.22278450
70. Huijghebaert S, Hoste L, Vanham G. Essentials in saline pharmacology for nasal or respiratory hygiene in times of COVID-19. Eur J Clin Pharmacol. (2021) 77:1275–93. doi: 10.1007/s00228-021-03102-3
71. Haddrell AE, Davies JF, Miles RE, Reid JP, Dailey LA, Murnane D. Dynamics of aerosol size during inhalation: hygroscopic growth of commercial nebulizer formulations. Int J Pharm. (2014) 463:50–61. doi: 10.1016/j.ijpharm.2013.12.048
72. Heyder J. Deposition of inhaled particles in the human respiratory tract and consequences for regional targeting in respiratory drug delivery. Proc Am Thorac Soc. (2004) 1:315–20. doi: 10.1513/pats.200409-046TA
73. Kuek LE, Lee RJ. First contact: the role of respiratory cilia in host-pathogen interactions in the airways. Am J Physiol Lung Cell Mol Physiol. (2020) 319:L603–19. doi: 10.1152/ajplung.00283.2020
74. Fiegel J, Clarke R, Edwards DA. Airborne infectious disease and the suppression of pulmonary bioaerosols. Drug Discov Today. (2006) 11:51–7. doi: 10.1016/S1359-6446(05)03687-1
75. Thibon C, Vecellio L, Dubus JC, Kabamba B, Reychler G. Nebulization and COVID-19: Is the risk of spread actual? Respir Med. (2022) 197:106854. doi: 10.1016/j.rmed.2022.106854
76. Gottsauner MJ, Michaelides I, Schmidt B, Scholz KJ, Buchalla W, Widbiller M, et al. A prospective clinical pilot study on the effects of a hydrogen peroxide mouth rinse on the intraoral viral load of SARS-CoV-2. Clin Oral Invest. (2020) 24:3707–13. doi: 10.1007/s00784-020-03549-1
77. Quan FS, Rubino I, Lee SH, Koch B, Choi HJ. Universal and reusable virus deactivation system for respiratory protection. Sci Rep. (2017) 7:39956. doi: 10.1038/srep39956
78. Liu W, Tang F, Fang LQ, De Vlas SJ, Ma HJ, Zhou JP, et al. Risk factors for SARS infection among hospital healthcare in Beijing: a case control study. Trop Med Int Health. (2009) 14:52–9. doi: 10.1111/j.1365-3156.2009.02255.x
79. Parviz S, Duncan L, Rabago D. Soap and water to hands and face-eye rinse, nasal irrigation and gargling with saline for COVID-19 with anecdotal evidence. Rhinology. (2021) 4:185-93. doi: 10.4193/RHINOL/21.040
80. Baxter AL, Schwartz KR, Johnson RW, Kuchinski AM, Swartout KM, Srinivasa Rao ASR, et al. Rapid initiation of nasal saline irrigation to reduce severity in high-risk COVID+ outpatients. Ear Nose Throat J. (2022) 1455613221123737. doi: 10.1177/01455613221123737
81. Singh V. (Investigator) Reported by Shaerma A. How the Practice of Jal Neti Helped Staff at a Jaipur Hospital Beat Covid. Daiji World News (2021). Available online at: https://www.daijiworld.com/news/newsDisplay?newsID=812519 (accessed January 10, 2023).
82. Gutiérrez-García R, De la Cerda-Ángeles JC, Cabrera-Licona A, Delgado-Enciso I, Mervitch-Sigal N, Paz-Michel BA. Nasopharyngeal and oropharyngeal rinses with neutral electrolyzed water prevents COVID-19 in front-line health professionals: a randomized, open-label, controlled trial in a general hospital in Mexico City. Biomed Rep. (2022) 16:11. doi: 10.3892/br.2021.1494
83. Madewell ZJ, Yang Y, Longini IM Jr, Halloran ME, Dean NE. Household transmission of SARS-CoV-2: a systematic review and meta-analysis. JAMA Netw Open. (2020) 3:e2031756. doi: 10.1001/jamanetworkopen.2020.31756
84. Lei H, Xu X, Xiao S, Wu X, Shu Y. Household transmission of COVID-19-a systematic review and meta-analysis. J Infect. (2020) 81:979–97. doi: 10.1016/j.jinf.2020.08.033
85. House T, Riley H, Pellis L, Pouwels KB, Bacon S, Eidukas A, et al. Inferring risks of coronavirus transmission from community household data. Stat Methods Med Res. (2022) 31:1738–56. doi: 10.1177/09622802211055853
86. Field RD, Moelis N, Salzman J, Bax A, Ausiello D, Woodward SM, et al. Inhaled water and salt suppress respiratory droplet generation and COVID-19 iIncidence and death on US coastlines. Mol Front J. (2021) 5:1-13. doi: 10.1142/S2529732521400058
87. Grant SN, Lester HA. Regulation of epithelial sodium channel activity by SARS-CoV-1 and SARS-CoV-2 proteins. Biophys J. (2021) 120:2805-13. doi: 10.1016/j.bpj.2021.06.005
88. Gentzsch M, Rossier BC. A Pathophysiological model for COVID-19: critical importance of transepithelial sodium transport upon airway infection. Function. (2020) 1:zqaa024. doi: 10.1093/function/zqaa024
89. Zhang WC, Du LJ, Zheng XJ, Chen XQ, Shi C, Chen BY, et al. Elevated sodium chloride drives type I interferon signalling in macrophages and increases antiviral resistance. J Biol Chem. (2018) 293:1030–9. doi: 10.1074/jbc.M117.805093
90. Müller S, Quast T, Schröder A, Hucke S, Klotz L, Jantsch J, et al. Salt-dependent chemotaxis of macrophages. PLoS ONE. (2013) 8:e73439. doi: 10.1371/annotation/1907a0e9-290a-4221-a3fa-9147cb48a6f3
91. Koparal M, Kurt E, Altuntas EE, Dogan F. Assessment of mucociliary clearance as an indicator of nasal function in patients with COVID-19: a cross-sectional study. Eur Arch Otorhinolaryngol. (2021) 278:1863-8. doi: 10.1007/s00405-020-06457-y
92. George CE, Scheuch G, Seifart U, Inbaraj LR, Chandrasingh S, Nair IK, et al. COVID-19 symptoms are reduced by targeted hydration of the nose, larynx and trachea. Sci Rep. (2022) 12:4599. doi: 10.1038/s41598-022-08609-y
93. King M, Dasgupta B, Tomkiewicz RP, Brown NE. Rheology of cystic fibrosis sputum after in vitro treatment with hypertonic saline alone and in combination with recombinant human deoxyribonuclease I. Am J Respir Crit Care Med. (1997) 156:173-7. doi: 10.1164/ajrccm.156.1.9512074
94. Kim CH, Hyun Song M, Eun Ahn Y, Lee JG, Yoon JH. Effect of hypo-, iso- and hypertonic saline irrigation on secretory mucins and morphology of cultured human nasal epithelial cells. Acta Otolaryngol. (2005) 125:1296-300. doi: 10.1080/00016480510012381
95. Charriot J, Volpato M, Petit A, Vachier I, Bourdin A. Methods of sputum and mucus assessment for muco-obstructive lung diseases in 2022: time to “unplug” from our daily routine! Cells. (2022) 11:812. doi: 10.3390/cells11050812
96. Liu Y, Lv J, Liu J, Li M, Xie J, Lv Q, et al. Mucus production stimulated by IFN-AhR signaling triggers hypoxia of COVID-19. Cell Res. (2020) 30:1078–87. doi: 10.1038/s41422-020-00435-z
97. Lodge KM, Cowburn AS, Li W, Condliffe AM. The impact of hypoxia on neutrophil degranulation and consequences for the host. Int J Mol Sci. (2020) 21:1183. doi: 10.3390/ijms21041183
98. Akgun E, Tuzuner MB, Sahin B, Kilercik M, Kulah C, Cakiroglu HN, et al. Proteins associated with neutrophil degranulation are upregulated in nasopharyngeal swabs from SARS-CoV-2 patients. PLoS ONE. (2020) 15:e0240012. doi: 10.1371/journal.pone.0240012
99. Ackermann M, Anders HJ, Bilyy R, Bowlin GL, Daniel C, De Lorenzo R, et al. Patients with COVID-19: in the dark-NETs of neutrophils. Cell Death Differ. (2021) 28:3125–3139. doi: 10.1038/s41418-021-00805-z
100. Al-Kuraishy HM, Al-Gareeb AI, Al-Hussaniy HA, Al-Harcan NAH, Alexiou A, Batiha GE. Neutrophil extracellular traps (NETs) and Covid-19: a new frontiers for therapeutic modality. Int Immunopharmacol. (2022) 104:108516. doi: 10.1016/j.intimp.2021.108516
101. Calvert BA, Quiroz EJ, Lorenzana Z, Doan N, Kim S, Senger CN, et al. Neutrophilic inflammation promotes SARS-CoV-2 infectivity and augments the inflammatory responses in airway epithelial cells. Front Immunol. (2023) 14:1112870:0–16. doi: 10.3389/fimmu.2023.1112870
102. Guéant JL, Guéant-Rodriguez RM, Fromonot J, Oussalah A, Louis H, Chery C, et al. Elastase and exacerbation of neutrophil innate immunity are involved in multi-visceral manifestations of COVID-19. Allergy. (2021) 76:1846–58. doi: 10.1111/all.14746
103. Arenas-De Larriva M, Martín-DeLeon R, Urrutia Royo B, Fernández-Navamuel I, Gimenez Velando A, Nuñez García L, et al. The role of bronchoscopy in patients with SARS-CoV-2 pneumonia. ERJ Open Res. (2021) 7:00165-2021. doi: 10.1183/23120541.00165-2021
104. Wauters E, Van Mol P, Garg AD, Jansen S, Van Herck Y, Vanderbeke L, et al. Discriminating mild from critical COVID-19 by innate and adaptive immune single-cell profiling of bronchoalveolar lavages. Cell Res. (2021) 31:272–90. doi: 10.1038/s41422-020-00455-9
105. Nadesalingam A, Chen JHK, Farahvash A, Khan MA. Hypertonic saline suppresses NADPH oxidase-dependent neutrophil extracellular trap formation and promotes apoptosis. Front Immunol. (2018) 9:359. doi: 10.3389/fimmu.2018.00359
106. Theobaldo MC, Llimona F, Petroni RC, Rios EC, Velasco IT, Soriano FG. Hypertonic saline solution drives neutrophil from bystander organ to infectious site in polymicrobial sepsis: a cecal ligation and puncture model. PLoS ONE. (2013) 8:e74369. doi: 10.1371/journal.pone.0074369
107. Hatanaka E, Shimomi FM, Curi R, Campa A. Sodium chloride inhibits cytokine production by lipopolysaccharide-stimulated human neutrophils and mononuclear cells. Shock. (2007) 27:32–5. doi: 10.1097/01.shk.0000238061.69579.a5
108. Ramalingam S, Cai B, Wong J, Twomey M, Chen R, Fu RM, et al. Antiviral innate immune response in non-myeloid cells is augmented by chloride ions via an increase in intracellular hypochlorous acid levels. Sci Rep. (2018) 8:13630. doi: 10.1038/s41598-018-31936-y
109. Ulfig A, Leichert LI. The effects of neutrophil-generated hypochlorous acid and other hypohalous acids on host and pathogens. Cell Mol Life Sci. (2021) 78:385–414. doi: 10.1007/s00018-020-03591-y
110. Parker H, Winterbourn CC. Reactive oxidants and myeloperoxidase and their involvement in neutrophil extracellular traps. Front Immunol. (2013) 3:424. doi: 10.3389/fimmu.2012.00424
111. Palmer LJ, Cooper PR, Ling MR, Wright HJ, Huissoon A, Chapple IL. Hypochlorous acid regulates neutrophil extracellular trap release in humans. Clin Exp Immunol. (2012) 167:261–8. doi: 10.1111/j.1365-2249.2011.04518.x
112. Saheb Sharif-Askari N, Saheb Sharif-Askari F, Mdkhana B, Hussain Alsayed HA, Alsafar H, Alrais ZF, et al. Upregulation of oxidative stress gene markers during SARS-COV-2 viral infection. Free Radic Biol Med. (2021) 172:688–98. doi: 10.1016/j.freeradbiomed.2021.06.018
113. Jadhav RB, Patil SS, Deolekar P, Yadav P. A comparative study to evaluate the use of saline nasal lavage and gargling in patients with COVID-19 infection. Int J Pharm Res. (2022) 14:12–7. doi: 10.31838/ijpr/2022.14.01.003
114. Kimura KS, Freeman MH, Wessinger BC, Gupta V, Sheng Q, Huang LC, et al. Interim analysis of an open-label randomized controlled trial evaluating nasal irrigations in non-hospitalized patients with Coronavirus Disease 2019. Int Forum Allergy Rhinol. (2020) 10:1325-28. doi: 10.1002/alr.22703
115. Ezer N, Belga S, Daneman N, Chan A, Smith BM, Daniels SA, et al. Inhaled and intranasal ciclesonide for the treatment of COVID-19 in adult outpatients: CONTAIN phase II randomised controlled trial. BMJ. (2021) 375:e068060. doi: 10.1136/bmj-2021-068060
116. Soler E, de Mendoza A, Cuello VI, Silva-Vetri MG, Núñez ZH, Ortega RG, et al. Intranasal xylitol for the treatment of COVID-19 in the outpatient setting: a pilot study. Cureus. (2022) 14:e27182. doi: 10.7759/cureus.27182
117. Varricchio A, La Mantia I, Brunese FP, Ciprandi G. Smell recovery in patients with COVID-19: an experience with nebulized nasal treatment. J Biol Reg Hom Agents. (2021) 35:683-6. doi: 10.23812/21-28-L
118. Kasiri H, Rouhani N, Salehifar E, Ghazaeian M, Fallah S. Mometasone furoate nasal spray in the treatment of patients with COVID-19 olfactory dysfunction: a randomized, double blind clinical trial. Int Immunopharmacol. (2021) 98:107871. doi: 10.1016/j.intimp.2021.107871
119. Rashid RA, Zgair A, Al-Ani RM. Effect of nasal corticosteroid in the treatment of anosmia due to COVID-19: a randomised double-blind placebo-controlled study. Am J Otolaryngol. (2021) 42:103033. doi: 10.1016/j.amjoto.2021.103033
120. Yildiz E, Koca Yildiz S, Kuzu S, Günebakan Ç, Bucak A, Kahveci OK. Comparison of the healing effect of nasal saline irrigation with triamcinolone acetonide versus nasal saline irrigation alone in COVID-19 related olfactory dysfunction: a randomized controlled study. Indian J Otolaryngol Head Neck Surg. (2022) 74(Suppl. 2):3022-7. doi: 10.1007/s12070-021-02749-9
121. Titova ON, Kuzubova NA, Skliarova DB, Aleksandrov AL, Egorova NV. The effectiveness of combination of 7% hypertonic saline and 0.1% natrii hyaluronan in patients with chronic obstructive pulmonary disease who have suffered a new coronavirus infection. Med Council. (2021) 16:85–91. (In Russ.). doi: 10.21518/2079-701X-2021-16-85-91
122. Voshaar T,. COVID-19 Therapie aus Sicht eines Aerosol-Experten. PARIde - Artzeportal (2020). Available online at: https://www.pari.com/de/aerzteportal/news/covid-19-therapie-aus-sicht-eines-aerosol-experten (accessed January 10, 2020).
123. Salva O, Doreski PA, Giler CS, Quinodoz DC, Guzmán LG, Muñoz SE, et al. Reversal of SARS-CoV2-induced hypoxia by nebulized sodium ibuprofenate in a compassionate use program. Infect Dis Ther. (2021) 10:2511–24. doi: 10.1007/s40121-021-00527-2
124. Delić N, Matetic A, Domjanović J, Kljaković-Gašpić T, Šarić L, Ilić D, et al. Effects of different inhalation therapy on ventilator-associated pneumonia in ventilated COVID-19 patients: a randomized controlled trial. Microorganisms. (2022) 10:1118. doi: 10.3390/microorganisms10061118
125. Schreiber S, Ronfani L, Ghirardo S, Minen F, Taddio A, Jaber M, et al. Nasal irrigation with saline solution significantly improves oxygen saturation in infants with bronchiolitis. Acta Paediatr. (2016) 105:292–6. doi: 10.1111/apa.13282
126. Bueno Campaña M, Olivares Ortiz J, Notario Muñoz C, Rupérez Lucas M, Fernández Rincón A, Patiño Hernández O, et al. High flow therapy versus hypertonic saline in bronchiolitis: randomised controlled trial. Arch Dis Child. (2014) 99:511–5. doi: 10.1136/archdischild-2013-305443
127. US Food Drug Administration. Is Rinsing Your Sinuses With Neti Pots Safe? DTB team. Available online at: https://www.fda.gov/consumers/consumer-updates/rinsing-your-sinuses-neti-pots-safethan (accessed September 28, 2022).
128. Valderramas SR, Atallah AN. Effectiveness and safety of hypertonic saline inhalation combined with exercise training in patients with chronic obstructive pulmonary disease: a randomized trial. Respir Care. (2009) 54:327-33.
129. Nicolson CH, Stirling RG, Borg BM, Button BM, Wilson JW, Holland AE. The long-term effect of inhaled hypertonic saline 6% in non-cystic fibrosis bronchiectasis. Respir Med. (2012) 106:661-7. doi: 10.1016/j.rmed.2011.12.021
130. Buchanan RM, Ibrahim K, Roberts HC, Stuart B, Webley F, Eminton Z, et al. Nasal irrigation and gargling with hypertonic saline to prevent transmission of SARS-CoV-2 to care home residents. A feasibility cluster trial. J Nursing Home Res. (2021) 7:13–6. doi: 10.14283/jnhrs.2021.3
131. Tiong V, Hassandarvish P, Bakar SA, Mohamed NA, Wan Sulaiman WS, Baharom N, et al. The effectiveness of various gargle formulations and salt water against SARS-CoV-2. Sci Rep. (2021) 11:20502. doi: 10.1038/s41598-021-99866-w
132. Pfeifer M, Ewig S, Voshaar T, Randerath WJ, Bauer T, Geiseler J, et al. Position paper for the state-of-the-art application of respiratory support in patients with COVID-19. Respiration. (2020) 99:521–41. doi: 10.1159/000509104
133. Gouvernement, du Québec. Self-Care Guide - COVID-19. Available online at: https://www.quebec.ca/en/health/health-issues/a-z/2019-coronavirus/self-care-guide-covid-19 (accessed January 9, 2021).
134. Kramer A, Eggers M, Hübner N-O, Steinmann E, Walger P, Exner M, et al. German Virucidal gargling and virucidal nasal spray. GMS Hyg Infect Control. (2021) 16:Doc02. doi: 10.3205/dgkh000373
135. House SA, Gadomski AM, Ralston SL. Evaluating the placebo status of nebulized normal saline in patients with acute viral bronchiolitis: a systematic review and meta-analysis. JAMA Pediatr. (2020) 174:250-9. doi: 10.1001/jamapediatrics.2019.5195
136. Khan SY, O'Driscoll BR. Is nebulized saline a placebo in COPD? BMC Pulm Med. (2004) 4:9. doi: 10.1186/1471-2466-4-9
137. Webster KE, O'Byrne L, MacKeith S, Philpott C, Hopkins C, Burton MJ. Interventions for the prevention of persistent post-COVID-19 olfactory dysfunction. Cochr Database Syst Rev. (2021) 7:CD013877. doi: 10.1002/14651858.CD013877
138. Seikai T, Takada A, Hasebe A, Kajihara M, Okuya K, Sekiguchi Yamada T, et al. Gargling with povidone iodine has a short-term inhibitory effect on SARS-CoV-2 in patients with COVID-19. J Hosp Infect. (2022) 123:179-81. doi: 10.1016/j.jhin.2022.01.001
139. Ivanova N, Sotirova Y, Gavrailov G, Nikolova K, Andonova V. Advances in the prophylaxis of respiratory infections by the nasal and the oromucosal route: relevance to the fight with the SARS-CoV-2 pandemic. Pharmaceutics. (2022) 14:530. doi: 10.3390/pharmaceutics14030530
140. Battistoni J. Président des médecins généralistes, France, DSG, letter of 22 March 2020. In: Reported by Anne Xaillé Les spray naseaux sont déconseillés Top Santé (2020). Available online at: https://www.topsante.com/medecine/maladies-infectieuses/zoonoses/coronavirus-le-lavage-de-nez-au-serum-physiologique-est-deconseille-635976 (accessed September 28, 2022).
141. Klein JM. Président du conseil national des ORL. In:Leboucq F, , editor. CheckNews. Covid-19 : est-il vrai qu'il faut éviter les lavages de nez? Libération 1/4/2020. Available online at: https://www.liberation.fr/checknews/2020/04/01/covid-19-est-il-vrai-qu-il-faut-eviter-les-lavages-de-nez_1783733/ (accessed September 28, 2022).
142. Nederlandse Vereniging voor Keel-Neus-Oorheelkunde en Heelkunde van het Hoofd-Halsgebied. Neusspoelen en Covid-19. KNO (Official Site of Dutch Ear-Nose-Throat specialists) (2020). Available online at: https://www.kno.nl/neusspoelen-en-covid-19/ (accessed September 28, 2022).
143. Direction Générale de la Santé (DCS), France. In: Bresson A, editor. Coronavirus : pourquoi le lavage de nez est déconseillé. PARENTS. (2020). Available online at: https://www.parents.fr/actualites/etre-parent/coronavirus-pourquoi-le-lavage-de-nez-est-deconseille-433124 (accessed September 28, 2022).
144. LUNGENÄRZTE im Netz. Einfaches Inhalieren kann die Ansteckungsgefahr mit Coronaviren deutlich senken. Meldung: News-Archiv (2021). Available online at: https://www.lungenaerzte-im-netz.de/news-archiv/meldung/article/einfaches-inhalieren-kann-die-ansteckungsgefahr-mit-coronaviren-deutlich-senken/ (accessed September 28, 2022).
Keywords: saline, COVID-19, nasal irrigation, gargling, nebulization, nasal spray, respiratory infection
Citation: Huijghebaert S, Parviz S, Rabago D, Baxter A, Chatterjee U, Khan FR, Fabbris C, Poulas K and Hsu S (2023) Saline nasal irrigation and gargling in COVID-19: a multidisciplinary review of effects on viral load, mucosal dynamics, and patient outcomes. Front. Public Health 11:1161881. doi: 10.3389/fpubh.2023.1161881
Received: 08 February 2023; Accepted: 18 May 2023;
Published: 16 June 2023.
Edited by:
Senthil Kumaran Satyanarayanan, China Medical University Hospital, TaiwanReviewed by:
Alessandra Fioretti, Tinnitus Center, European Hospital, ItalyBenjamin Florian Koch, Goethe University Frankfurt, Germany
Copyright © 2023 Huijghebaert, Parviz, Rabago, Baxter, Chatterjee, Khan, Fabbris, Poulas and Hsu. This is an open-access article distributed under the terms of the Creative Commons Attribution License (CC BY). The use, distribution or reproduction in other forums is permitted, provided the original author(s) and the copyright owner(s) are credited and that the original publication in this journal is cited, in accordance with accepted academic practice. No use, distribution or reproduction is permitted which does not comply with these terms.
*Correspondence: Suzy Huijghebaert, Uy5IdWlqZ2hlYmFlcnRAZ21haWwuY29t
†ORCID: Suzy Huijghebaert orcid.org/0000-0002-5477-6838