- 1South African Medical Research Council, Vaccines and Infectious Diseases Analytics Unit, University of the Witwatersrand, Johannesburg, South Africa
- 2Bill and Melinda Gates Foundation, Seattle, WA, United States
- 3London School of Hygiene and Tropical Medicine, London, United Kingdom
- 4Warwick Medical School, University of Warwick, Coventry, United Kingdom
- 5Department of Obstetrics and Gynaecology, University of the Witwatersrand, Johannesburg, South Africa
- 6Department of Clinical Microbiology, Christian Medical College, Vellore, India
Invasive group B streptococcal (GBS) disease is the commonest perinatally-acquired bacterial infection in newborns; the burden is higher in African countries where intrapartum antibiotic prophylaxis strategies are not feasible. In sub-Saharan Africa, almost one in four newborns with GBS early-onset disease will demise, and one in ten survivors have moderate or severe neurodevelopmental impairment. A maternal GBS vaccine to prevent invasive GBS disease in infancy is a pragmatic and cost-effective preventative strategy for Africa. Hexavalent polysaccharide protein conjugate and Alpha family surface protein vaccines are undergoing phase II clinical trials. Vaccine licensure may be facilitated by demonstrating safety and immunological correlates/thresholds suggestive of protection against invasive GBS disease. This will then be followed by phase IV effectiveness studies to assess the burden of GBS vaccine preventable disease, including the effect on all-cause neonatal infections, neonatal deaths and stillbirths.
Introduction
Progress to reduce under five mortality rates by 2030, as part of the sustainable developmental goals, has been modest for many African countries (1), and children living in sub-Saharan Africa are ten-fold more likely to die in the first month after birth compared to high-income countries (1, 2). Many of the deaths in this age group are at and/or around birth; in 2021, of the five-million deaths in children less than 5 years of age, 46% occurred in the neonatal period (1, 2). In this neonatal period, the leading causes of death are from preterm related complications (17%), intrapartum related events (11%) and pneumonia, sepsis or meningitis (8%) (2).
In terms of specific infectious causes, Streptococcus agalactiae, commonly referred to as Group B Streptococcus (GBS), is the commonest reported cause of early-onset disease (EOD; i.e.: disease in the first 6 days after birth) in neonates (3–7). However, adverse perinatal outcomes are not limited to EOD in neonates, and late-onset disease (LOD) also occurs mostly within the neonatal period. Whilst considerable mortality is associated with EOD and LOD, survivors may also develop moderate or severe neurodevelopmental impairment (NDI) (8–10). Prior to birth, there may be miscarriages and stillbirth from invasive GBS disease in-utero (11, 12), and GBS colonisation has been associated with preterm labour (13, 14). Pregnant women may also develop GBS sepsis (15).
Burden of disease in Africa
Worldwide, based on maternal GBS colonization, and using Bayesian modelling, there were an estimated 394,000 cases of invasive GBS disease (EOD and LOD) and 58,300 (95%CI: 26,500–125,800) deaths in early infancy (8). The majority of cases of invasive GBS disease, 231,800 (95%CI: 114,100–455,000) cases, were EOD. The burden of disease and death from GBS EOD is high in African countries, with 90,800 (95%CI: 43,000–186,600) of all EOD cases in sub-Saharan Africa (8) and the incidence of EOD almost double the worldwide estimate (16). Consequently, almost half of all early-onset GBS deaths occur in sub-Saharan Africa, where the case fatality is also high (23%, vs. 6% in developed countries) (8). Factors driving the incidence of EOD in African countries include higher prevalence of maternal GBS colonization, and limited or no intrapartum antibiotic prophylaxis (IAP). IAP is commonly given in high-income countries, based on either clinical risk-based screening or microbiological screening to prevent EOD (16, 17). It should also be noted that there are data gaps in Africa, with less than a quarter of African countries contributing data to estimates (8, 16).
The adverse consequences of EOD GBS disease in neonates are not limited to disease and death. There may also be neurodevelopmental impairment (NDI). In high-income countries, survivors of invasive GBS disease (sepsis and/or meningitis) compared to matched controls have a two-fold increased risk of moderate or severe NDI by 10 years of age (9). There is a paucity of long-term outcome data from Africa, but in a recent multi-country matched cohort study undertaken in South Africa, India, Mozambique, Kenya, and Argentina, 38% (8.8% were moderate or severe) of survivors (n = 138) of invasive GBS disease had NDI compared to 22% of non-GBS (n = 390) children (10). Specifically, survivors of GBS EOD commonly developed NDI (10, 18).
In addition to this burden, there is also a substantial burden from GBS causing stillbirth from infections in-utero, with 20,300 (95%CI: 9,000–40,500) out of 46,200 (95%CI: 20,300–111,300) GBS stillbirths in 2020 in sub-Saharan Africa (8). Furthermore, babies born with intrapartum hypoxia from invasive GBS disease may present with neonatal encephalopathy (19, 20).
Maternal GBS colonization increases the risk of preterm labour (13), although data are limited; of the 15 million preterm births that occurred globally in 2020, approximately 518,100 (95%CI: 36,900–1,142,300) were estimated to be associated with GBS, most of which were from sub-Saharan Africa (8). Prematurely born infants also have an increased risk of acquiring invasive GBS disease (21); and being premature and acquiring invasive GBS disease was independenty associated with death and NDI in survivors (18, 22–24).
The acute healthcare cost and impact on the family of a neonate having a sepsis and/or meningitis is important. In a recent study from South Africa (a third of participants had EOD), the mean household and hospital cost of having a child with culture-confirmed GBS, E-coli or Staphyloccal infection was 52 and 684 international dollars, respectively (25). Average hospitalization cost for confirmed sepsis ranged from $55 to $129 in most high-income countries (26). Extra care for a child with invasive GBS disease is also required, and if the child has NDI this will be long-term. In cases of infant deaths from GBS EOD, parents of infants experience grief and express frustrations over the death of the child, and fears of future pregnancy (27).
Microbiology and pathogenesis of EOD
Streptococcus agalactiae is an encepsulated Gram positive coccus exclusively in the “B” Lancefield grouping of Streptococci. Approximately 18% (95%CI: 17–19%) of pregnant women are gastrointestinal and/ or genitourinary tract colonised with GBS globally (28); colonization rates are reported to be higher (22–25%) in Southern Africa (28–30), and approximately half of pregnant women are colonized at some point during their pregnancy (31). Of the 20 million pregnant women that had rectovaginal colonization in 2020, 6 (30%) million were living in sub-Saharan Africa (8).
There are ten GBS serotypes (Ia, Ib, II-X), serotype III being the commonest cause of invasive disease followed by Ia and V (16). Geographical differences in the prevalence of serotypes have been reported, and in Africa, serotype III is the commonest for EOD (16); most of the hypervirulent clonal complex 17 is serotype III (32).
There are several risk factors for EOD, GBS colonisation of the pregnant women is the only unequivocal risk factor (33). Other risk factors for EOD include prolonged rupture of membranes (>18 h; PROM), maternal fever during labor, premature labour, previous infant with invasive GBS disease, maternal GBS bacteriuria, chorioamnionitis, absence of screening for GBS colonisation, unavailability of intrapartum antibiotic prophylaxix (IAP), unavailability of a skilled birth attendant (8, 22, 34).
GBS colonization is usually asymptomatic, and maternal GBS colonization status is unknown to most African pregnant women as this is not routinely tested for in pregnancy. During the prenatal or perinatal period, ascending GBS into amniotic cavity can adversely affect the pregnant women resulting in chorioamnionitis or invasive maternal disease (15). For the foetus or newborn, the possible outcomes are EOD (pneumonia, sepsis, meningitis and/ or neonatal encephalopathy), stillbirth, or prematurity (11–13, 19, 20, 24) (Figure 1).
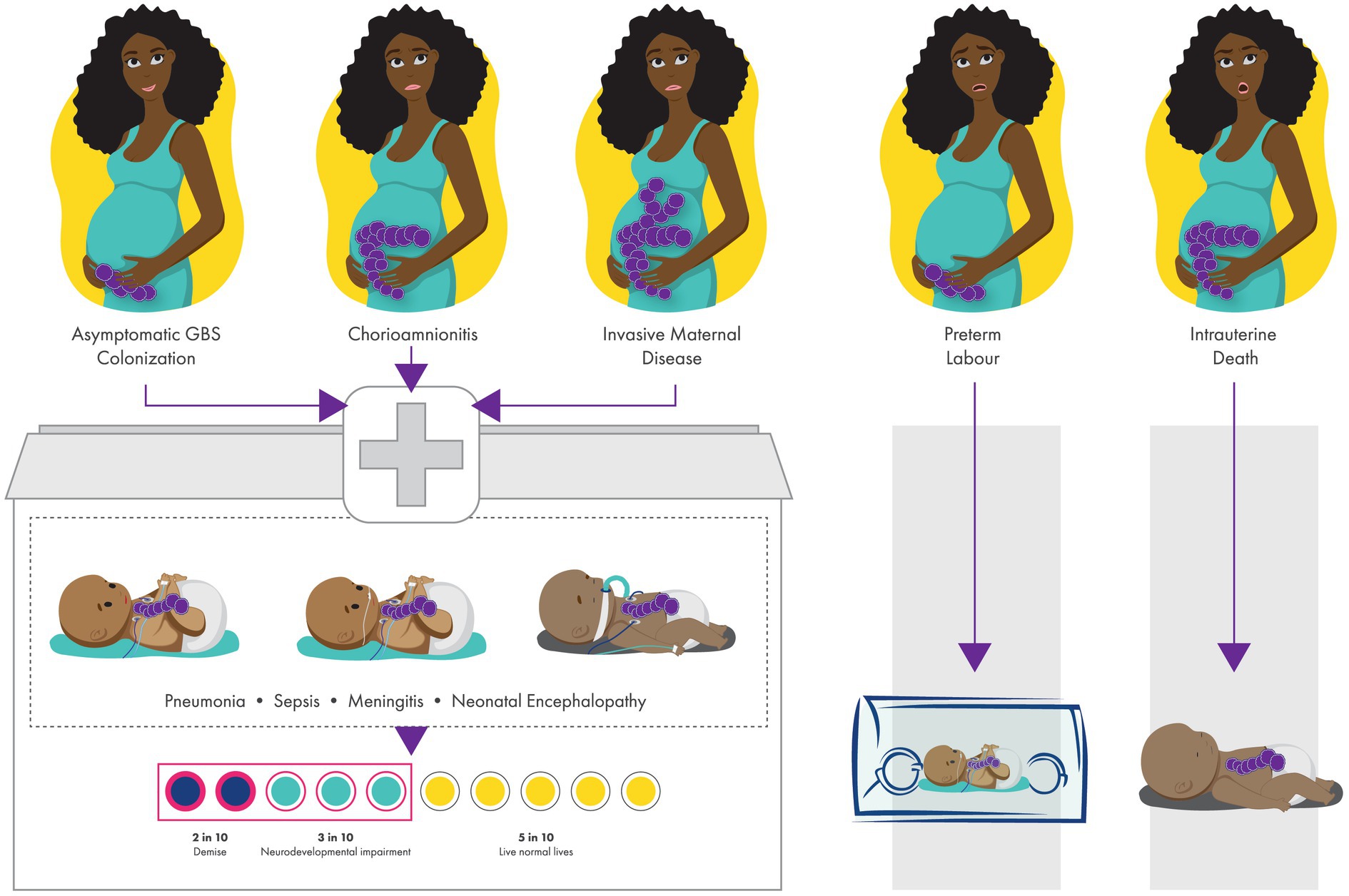
Figure 1. Perinatal and birth outcomes of newborns with invasive group B streptococcal (GBS) disease. GBS colonisation can lead to invasive early-onset neonatal disease (pneumonia, sepsis, meningitis, neonatal encephalopathy), and can also result in maternal infection (chorioamnionitis and invasive maternal disease), preterm birth and stillbirth. In sub-Saharan Africa, 23% will demise and 38% of survivors from low- and middle-income countries have neurodevelopmental impairment.
Current preventative strategies
Currently, prevention of invasive GBS EOD is dependant on the availability of intravenous antibiotics that can be administered to a pregnant women at least 4 h before delivery by adequately trained health care workers (35). There are two screening approaches (microbiological or clinical risk-based) to determine which pregnant women should get IAP. Many high-income countries use the microbiological screening approach in which pregnant women have a vaginal-rectal swab in the 36 or 37th week of gestation, and are offered IAP if they are GBS colonized (36). This strategy resulted in a 80% reduction in EOD in the United States from the early 1990’s to 2010 (37). The risk-based approach targets pregnant women that have selected clinical risk factors (premature labour, PROM, maternal fever or previous child with GBS). Both strategies have limitations; microbiological screening is insensitive and colonisation status changes during pregnancy. Clinical risk factors are non-specific, and only identified with close monitoring and comprehensive assessments, otherwise sensitivity is low. Microbiological screening also requires processing of specimens, logistical support and timely feedback of results. Microbiolgical screening is likely more effective at reducing EOD than risk-based approaches (38), but implementation challenges make either strategy challenging to implement in resource limited African settings. In African countries, IAP is not provided due to an absence of microbiological screening, and (i) a large proportion of home deliveries, (ii) no intravenous treatment available at primary health care facilities where a large proportion of women deliver, (iii) inability to administer antibiotics 4 h before delivery because of late presentations to labour ward, or staff shortages that delay medical interventions, and (iv) failure to recognise pregnant women with risk factors when they are present (39). Importantly, the risk of EOD to a neonate born to GBS colonized pregnant women is 1.1% with no IAP compared to 0.3% where IAP coverage is high (34).
Point of care / real-time molecular PCR testing in labour wards with IAP to colonized women has also been associated with a reduction in EOD (40). The sensitivity and specificity of PCR testing to detect GBS colonization in pregnant women has been reported as 93 and 97%, respectively (41). The advantages of these point of care testing would be a non-reliance on laboratory service and the immendiate availability of results to the midwife / obstetrician, but this will need to be offset by the high cost of these tests.
Recent studies have investigated the role of probiotics, garlic and zinc in the prevention of GBS colization but results are inconclusive (42–44).
Maternal GBS vaccination
Maternal vaccination is now recognized as the optimal strategy to reduce disease in pregnant women, and foetus or young infant. There is considerable precedent for vaccines in pregnancy providing protection at the most critical time after birth, and WHO has recommended tetanus, pertussis, influenza and Covid-19 vaccinations during pregnancy (45). Vaccinating pregnant women against pertussis and influenza has been shown reduce disease in infants of vaccinated mothers, and has been associated with a 75% reduction in stillbirths and a 30% reduction in preterm birth (46–48). Other vaccines such as those for Cholera and Typhoid are recommended in specific situations, and a number of maternal vaccines are currently under development (45).
Vaccination of pregnant women to prevent invasive GBS disease in infancy is an important, pragmatic, future preventative strategy for Africa and other low- and middle-income countries (LMICs). The WHO has provided a comprehensive value assessment for a GBS vaccine (49–51). A GBS vaccine would also likely prevent stillbirths and LOD (51), and may reduce GBS-associated preterm birth. A maternal GBS vaccine would need to be safe, effective, affordable, and accepted by policymakers as important, with demand from pregnant women and their partners. Studies from high-income countries suggest there is likely to be demand, but studies from countries in Africa are needed; in a study from the United Kingdom, after receiving information about GBS, two thirds of women reported that they would consider taking a GBS vaccine during pregnancy, underpinning the need for interventions to support uptake (52).
In terms of health impact and cost-effectiveness, a maternal GBS vaccine with 80% efficacy could prevent, worldwide, 127,000 (uncertainty range (UR): 63,300 – 248,000) EOD cases, 87,300 (UR: 38,100 – 209,000) LOD cases, 31,100 (UR: 14,400 – 66,400) deaths, 17,900 (UR: 6,380 – 49,900) cases of moderate and severe NDI, 185,000 (UR: 13,500 – 407,000) preterm births, and 23,000 (UR: 10,000 – 56,400) stillbirths per year (53). The effect will be greatest in sub-Saharan where two-fifths of the above will be prevented by vaccinating a fifth of women (53). A GBS vaccine is likely to be cost effective in most settings, including African countries (54–56). Asssuming the cost of the vaccine was $50, $15 and $3.50 in high, upper-middle, and in low/lower-middle-income countries, respectively, a single dose of the vaccine would cost $1.7 billion but save $385 million in healthcare costs worldwide (53).
The mechanism of protection against invasive disease through maternal vaccination is from an increase in type-specific antibody levels in the vaccinated pregnant women that can be transplacentally transferred to the foetus over the weeks (most tranfer occurs in the last trimester) until delivery. The transplacental transfer of type-specific maternal IgG antibodies (IgG1 > IgG4 > IgG3 > IgG2) is an active process from the maternal to foetal circulation using the neonatal Fc receptor (FcRn) (45). Protein based vaccines induce mostly IgG1 and IgG3 responses, whereas capsular polysaccharide vaccines induce IgG2. The rate of IgG transfer is negatively affected by infections such as malaria and HIV, which are common in some areas of African countries. For a maternal GBS vaccine to be effective, the vaccine would need to be: (i) immunogenic and elicit functional responses, and (ii) administered at the appropriate gestational age for optimal concentrations of IgG to be transported across the placenta to the foetus (this is dependant on IgG subclass, transfer rate and gestational time to birth). Importantly, a vaccine to prevent invasive GBS disease can reduce the emergence of antimicrobial resistance; recent data from the US shows a low but increasing proportion of isolates with reduced beta-lactam susceptibility (57).
Vaccine licensure pathway and current landscape
In 1976, Baker and Kasper reported that low levels of maternal antibody titer was associated with invasive GBS disease (58). Both the GBS capsular polysaccharide (CPS) and many surface proteins have antigenic properties. Many studies have reported the association between higher levels of natural acquired maternal antibodies against GBS CPS and surface protein, and decreased acquisition of GBS colonization or a decreased risk of invasive GBS disease (59–64). Using Bayesian analysis, varying thresholds (“correlates of protection”) have been proposed (62). A maternal IgG antibody concentration of ≥1 μg/mL for serotypes Ia, III and V was proposed as a threshold of protection amongst pregnant women in the US by Baker and colleagues in 2014 (65). This same threshold reduced the risk of disease by 80% for serotypes Ia and III from eight European countries (66). In South Africa, however, a higher threshold of protection was proposed (≥2.3 μg/mL and ≥ 3.4 μg/mL for serotypes Ia and III was associated with a 90% risk reduction in disease) (63). Importantly, benchmarking a correlate of protection to infant antibody levels, and supplementing that with maternal levels and transplcental tranfer ratios may be necessary because of the unpredictability of transplacental transfer. An infant antibody level between 1 and 3 μg/mL protected 90% of infants from invasive GBS disease caused by serotype Ia and III in South Africa (63, 67). Determination of single protective threshold for all GBS serotypes would be ideal.
There have been a number of monovalent, bivalent, trivalent (Ia, Ib, III) and hexavalent (Ia, Ib, II, III, IV and V) CPS phase I and II vaccine trials over the past few decades, some of which were undertaken in pregnant women (68–70). These trials showed higher IgG antibody reponses with CPS protein conjugate vaccines compared to CPS only vaccines. Investigators also explored using the tetanus toxoid versus CRM197, a non-toxic mutant of diphtheria toxin, as carrier protein – findings were similar (68). Furthermore, CPS protein conjugate vaccines induced peak responses 4–8 weeks after vaccination and began to wane 6 months after vaccination (68). The first GBS vaccine trial undertaken in pregnant women was conducted in the United States in 1988 using an unconjugated monovalent serotype III CPS vaccine, and then followed this up with a serotype-III CPS protein conjugate vaccine in 2003 (71, 72). Subsequently, phase Ib/II trivalent CPS protein conjugate vaccine trials have explored safety, different dosing schedules, a second dose, and immunogenicity in HIV-infected compared to uninfected pregnant women (73–77). These GBS CPS conjugate vaccines were safe in pregnancy. In South Africa, a trivalent GBS vaccine administered to pregnant women showed higher geometric mean concentrations (GMCs) than placebo for serotypes Ia, Ib and III, and a significant difference was noted with higher vaccine doses for serotype Ia, but not for Ib and III. Cord-maternal antibody ratios were 49–79% (74). Similarly, infants of vaccinated mothers had higher serotype-specific GMCs than placebo, and serotypes Ia and III levels decreased to 26–35% from birth to 3 months of age (78). Therefore, a GBS polysaccharide conjugate vaccine is likely to protect young infants from invasive GBS disease. Additionally, a phase II, GBS serotype III conjugate vaccine administered to nonpregnant women was 36–43% effective at reducing first acquisition of GBS vaginal/rectal colonization (79). Furthermore, the vaccine is likely to increase sIgA breastmilk antibody concentrations; serotype-specific natural sIgA antibody was independently associated with protection against LOD (80).
Given the serotype diversity globally and the increased prevalence of serotype IV and V in some regions, a Pfizer hexavalent (Ia, Ib, II, III, IV, and V; referred to as GBS6) vaccine is undergoing clinical trials. The phase I/II, placebo-controlled, dose-escalation trial in 365 healthy men and non-pregnant women aged 18–49 years who received GBS6 (5 μg, 10 μg, 20 μg) with aluminium phosphate (AlPO4) or placebo reported adverse events in 23–48% of participants depending on dosing and inclusion of AlPO4 (81). None of three serious adverse events reported during the study were considered to be related to the vaccine. Serotype-specific IgG GMCs peaked at 2 weeks after vaccination and remained higher (10 to 56-fold rise) than placebo at six-months. Most (75%) vaccinated participants had an antibody concentration ≥ 1 μg/mL for serotypes Ia, II, III, IV, and V at 1 month after vaccination. A threshold of ≥1 μg/mL for serotype Ib was present in 40–57% of participants. GBS6 is being evaluated in an ongoing phase II, placebo-controlled, study in pregnant women assessing the safety, tolerability and immunogenicity (NCT03765073). Another hexavalent vaccine candidate developed by Inventprise is in the preclinical phase.
There are few reasons for the considerable delays in phase III trials of GBS conjugate vaccines. Firstly, the burden of invasive disease needed to be better established. A geographic diversity in the GBS capsular serotypes causing disease were observed, and vaccines were increased from trivalent to hexavalent, aiming to cover 98% of serotypes causing disease in young infants (16). Secondly, the full value proposition of vaccination that extends beyond invasive neonatal disease needed to be defined. This includes the potential impact of vaccination on all-cause neonatal sepsis, neonatal mortality and stillbirths. Thirdly, licensure of the vaccine through conventional phase III clinical trials with clinical outcomes of efficacy would mean that a sample of 30–180,000 pregnant women would be required in a setting with a high burden of disease (82, 83). Therefore, licensure of a GBS vaccine would be facilitated, if acceptable to regulators, through benchmarking the efficacy endpoint on a threshold of protection that is required to protect against invasive infant GBS disease, and following that with phase IV clinical effectiveness studies (83). This approach has been previously explored for meningocococcal and pneumococcal vaccines (84, 85).
Protein based vaccines that are highly conserved and provide a broad spectrum of protection against most serotype-causing disease strains are also under development. Amongst the leading candidates are antigens tartgetted at the Alpha family (Alp 1–4, Alpha C and Rib) of surface proteins. A protein based GBS vaccine that consists of the N-terminal domains of AlphaC and Rib (GBS-NN) is immunogenic and safe in non-pregnant adults (86). Similarly, a second generation vaccine (AlpN) that includes Rib, Alpha C, Alp1, Alp2 and Alp3 has been shown to be immunogenic and safe in non-pregnant adults (87). Currently, a phase 2 (NCT05154578) study evaluating the immunogenicity and safety of the AlpN vaccine in pregnant women is underway in South Africa, Denmark, Uganda and United Kingdom. Thresholds for protection have also been proposed for some of these surface protein vaccine candidates (64).
The GBS vaccine pipeline has been recently described (70, 88); leading candidates are the Pfizer hexavalent CPS protein conjugate vaccine and the Minervax AlpN protein-based vaccine. Monovalent, bivalent or trivalent investigational CPS protein conjugate vaccine that reached phase II evaluation have been suspended. Notably, few CPS protein conjugate and protein-based vaccine are in pre-clinical trials.
Considerations for GBS vaccination in African populations
There are specific considerations for vaccines in African populations. The prevalence of HIV amongst pregnant women in some sub-Saharan African countries is high. In South Africa, the antenatal HIV prevalence rate has remained at 30% over the last two decades (89). Despite advancements in the prevention of mother to child programmes of HIV, HIV-infected pregnant women have an increased risk of having a baby with invasive GBS disease (90, 91). HIV-exposed neonates have lower serotype-specific and surface protein antibody levels at birth compared to HIV-unexposed neonates (92, 93). This may in part be from lower naturally acquired antibody levels in HIV-infected pregnant women and secondly, from inefficient transplacental transfer of antibody (92, 93). Notably, in Malawi and South Africa, a 5 μg trivalent (serotypes Ia, Ib, and III) GBS vaccine was found to be less immunogenic in HIV-infected compared to HIV-uninfected pregnant women (75). Therefore, higher doses or alternative dosing schedules will need to be considered in HIV-infected pregnant women to optimise antibody responses and tranplacental transfer.
The second concern in African populations is that malaria is endemic to many regions. Similar to HIV, malaria may affect the transplacental transfer of antibody from the pregnant women to the foetus, this needs to be evaluated in the context of a GBS vaccine (94).
Thirdly, prematurity is prevalent in Africa, and vaccinated pregnant women need an optimal gestational period after vaccination to achieve adequate antibody transfer to the foetus. Approximately half of maternal antibody levels are present in the foetus at the beginning of the third trimester (95). Therefore, early preterm neonates will be at increased risk of disease. This is a major challenge for most African countries where IAP for pregnant women presenting in preterm labour in not readily available.
Lastly, some of the highest burden of GBS disease is in sub-Saharan Africa and therefore ethical considerations to undertaking clinical trials in these settings need to consider implementation and sustainability of the vaccine after licensure (96).
Post GBS vaccine licensure
If licensure is based on a correlate of protection, this will need to be followed by vaccine effectiveness studies, vaccine probe studies or national / regional surveillance programmes to measure the impact of vaccination on culture-confirmed GBS disease and stillbirths, all-cause sepsis, all-cause meningitis, all neonatal deaths and all stillbirths, and the effect of the vaccine on preterm labour. In addition, safety monitoring; particularly foetal and birth outcomes will need to continue. For a successful GBS vaccine, health-care worker (obstetrician/midwife) engagement is crucial. To overcome vaccine hesitancy, public engagement and awareness programmes are required by governments and policymakers. Importantly, as more maternal vaccines are recommended, an antenatal extended programme of immunisation (EPI) for pregnant women needs to be developed and understanding vaccine interactions and timing during pregnancy needs to be clearly defined.
Conclusion
The burden of invasive GBS disease, the commonest cause of EOD in neonates, is high in Africa, where IAP preventative strategies are not feasible, although data gaps remain. However, after many decades of GBS vaccine development, maternal GBS vaccines are moving into late stage clinical trials. If these vaccine candidates are successful and a GBS vaccine is licensed based on a correlate of protection, studies of effectiveness will need to follow to assess the burden of GBS vaccine preventable disease. These studies should be complemented by routine clinical surveillance (97). This will require sentinel surveillance sites with standardized laboratory methods and monitoring systems to investigate neonates with signs of infection and confirmation of GBS, similar to those used in the estimation of pneumococcal and rotavirus vaccination effectiveness (98). These WHO surveillance sites were designed to measure baseline burden of disease or cost-effectiveness, estimate VE after vaccine introduction, monitor trends in serotype/ genotype distribution and serotype replacement after vaccine introduction, and detect other infectious diseases. Such sites exist in African countries, but sustainability is challenging.
Data availability statement
The original contributions presented in the study are included in the article/supplementary material, further inquiries can be directed to the corresponding author.
Author contributions
ZD wrote the first draft. AS, VB, and GK provided critical review. All authors contributed to the article and approved the submitted version.
Conflict of interest
AS is employed by the Bill and Melinda Gates Foundation.
The remaining authors declare that the research was conducted in the absence of any commercial or financial relationships that could be construed as a potential conflict of interest.
Publisher’s note
All claims expressed in this article are solely those of the authors and do not necessarily represent those of their affiliated organizations, or those of the publisher, the editors and the reviewers. Any product that may be evaluated in this article, or claim that may be made by its manufacturer, is not guaranteed or endorsed by the publisher.
References
1. Sharrow, D, Hug, L, Liu, Y, Lindt, N, and You, D. Levels and Trends in Child Mortality. (United Nations Children’s Fund). (2022).
2. Perin, J, Mulick, A, Yeung, D, Villavicencio, F, Lopez, G, Strong, KL, et al. Global, regional, and national causes of under-5 mortality in 2000-19: an updated systematic analysis with implications for the sustainable development goals. Lancet Child Adolesc Health. (2022) 6:106–15. doi: 10.1016/S2352-4642(21)00311-4
3. Schrag, SJ, Farley, MM, Petit, S, Reingold, A, Weston, EJ, Pondo, T, et al. Epidemiology of invasive early-onset neonatal Sepsis, 2005 to 2014. Pediatrics. (2016) 138:e20162013. doi: 10.1542/peds.2016-2013
4. Thigpen, MC, Whitney, CG, Messonnier, NE, Zell, ER, Lynfield, R, Hadler, JL, et al. Bacterial meningitis in the United States, 1998-2007. N Engl J Med. (2011) 364:2016–25. doi: 10.1056/NEJMoa1005384
5. Johansson Gudjónsdóttir, M, Elfvin, A, Hentz, E, Adlerberth, I, Tessin, I, and Trollfors, B. Changes in incidence and etiology of early-onset neonatal infections 1997-2017 - a retrospective cohort study in western Sweden. BMC Pediatr. (2019) 19:490. doi: 10.1186/s12887-019-1866-z
6. Singh, T, Barnes, EH, and Isaacs, D. Early-onset neonatal infections in Australia and New Zealand, 2002-2012. Arch Dis Child Fetal Neonatal Ed. (2019) 104:F248–52. doi: 10.1136/archdischild-2017-314671
7. Mashau, RC, Meiring, ST, Dramowski, A, Magobo, RE, Quan, VC, Perovic, O, et al. Culture-confirmed neonatal bloodstream infections and meningitis in South Africa, 2014-19: a cross-sectional study. Lancet Glob Health. (2022) 10:e1170–8. doi: 10.1016/S2214-109X(22)00246-7
8. Gonçalves, BP, Procter, SR, Paul, P, Chandna, J, Lewin, A, Seedat, F, et al. Group B streptococcus infection during pregnancy and infancy: estimates of regional and global burden. Lancet Glob Health. (2022) 10:e807–19. doi: 10.1016/S2214-109X(22)00093-6
9. Horváth-Puhó, E, van Kassel, MN, Gonçalves, BP, de Gier, B, Procter, SR, Paul, P, et al. Mortality, neurodevelopmental impairments, and economic outcomes after invasive group B streptococcal disease in early infancy in Denmark and the Netherlands: a national matched cohort study. Lancet Child Adolesc Health. (2021) 5:398–407. doi: 10.1016/S2352-4642(21)00022-5
10. Paul, P, Chandna, J, Procter, SR, Dangor, Z, Leahy, S, Santhanam, S, et al. Neurodevelopmental and growth outcomes after invasive group B streptococcus in early infancy: a multi-country matched cohort study in South Africa, Mozambique, India, Kenya, and Argentina. EClinicalMedicine. (2022) 47:101358. doi: 10.1016/j.eclinm.2022.101358
11. Seale, AC, Blencowe, H, Bianchi-Jassir, F, Embleton, N, Bassat, Q, Ordi, J, et al. Stillbirth with group B streptococcus disease worldwide: systematic review and Meta-analyses. Clin Infect Dis. (2017) 65:S125–32. doi: 10.1093/cid/cix585
12. Madhi, SA, Briner, C, Maswime, S, Mose, S, Mlandu, P, Chawana, R, et al. Causes of stillbirths among women from South Africa: a prospective, observational study. Lancet Glob Health. (2019) 7:e503–12. doi: 10.1016/S2214-109X(18)30541-2
13. Bianchi-Jassir, F, Seale, AC, Kohli-Lynch, M, Lawn, JE, Baker, CJ, Bartlett, L, et al. Preterm birth associated with group B streptococcus maternal colonization worldwide: systematic review and Meta-analyses. Clin Infect Dis. (2017) 65:S133–42. doi: 10.1093/cid/cix661
14. Tano, S, Ueno, T, Mayama, M, Yamada, T, Takeda, T, Uno, K, et al. Relationship between vaginal group B streptococcus colonization in the early stage of pregnancy and preterm birth: a retrospective cohort study. BMC Pregnancy Childbirth. (2021) 21:141. doi: 10.1186/s12884-021-03624-9
15. Hall, J, Adams, NH, Bartlett, L, Seale, AC, Lamagni, T, Bianchi-Jassir, F, et al. Maternal disease with group B streptococcus and serotype distribution worldwide: systematic review and Meta-analyses. Clin Infect Dis. (2017) 65:S112–24. doi: 10.1093/cid/cix660
16. Madrid, L, Seale, AC, Kohli-Lynch, M, Edmond, KM, Lawn, JE, Heath, PT, et al. Infant group B streptococcal disease incidence and serotypes worldwide: systematic review and Meta-analyses. Clin Infect Dis. (2017) 65:S160–72. doi: 10.1093/cid/cix656
17. Le Doare, K, O'Driscoll, M, Turner, K, Seedat, F, Russell, NJ, Seale, AC, et al. Intrapartum antibiotic chemoprophylaxis policies for the prevention of group B streptococcal disease worldwide: systematic review. Clin Infect Dis. (2017) 65:S143–51. doi: 10.1093/cid/cix654
18. Nakwa, FL, Lala, SG, Madhi, SA, and Dangor, Z. Neurodevelopmental impairment at 1 year of age in infants with previous invasive group B streptococcal Sepsis and meningitis. Pediatr Infect Dis J. (2020) 39:794–8. doi: 10.1097/INF.0000000000002695
19. Tann, CJ, Martinello, KA, Sadoo, S, Lawn, JE, Seale, AC, Vega-Poblete, M, et al. Neonatal encephalopathy with group B streptococcal disease worldwide: systematic review, Investigator Group datasets, and Meta-analysis. Clin Infect Dis. (2017) 65:S173–89. doi: 10.1093/cid/cix662
20. Car, KP, Nakwa, F, Solomon, F, Velaphi, SC, Tann, CJ, Izu, A, et al. The association between early-onset sepsis and neonatal encephalopathy. J Perinatol. (2022) 42:354–8. doi: 10.1038/s41372-021-01290-5
21. Lin, FY, Weisman, LE, Troendle, J, and Adams, K. Prematurity is the major risk factor for late-onset group B streptococcus disease. J Infect Dis. (2003) 188:267–71. doi: 10.1086/376457
22. Dangor, Z, Lala, SG, Cutland, CL, Koen, A, Jose, L, Nakwa, F, et al. Burden of invasive group B streptococcus disease and early neurological sequelae in south African infants. PLoS One. (2015) 10:e0123014. doi: 10.1371/journal.pone.0123014
23. Horváth-Puhó, E, Snoek, L, van Kassel, MN, Gonçalves, BP, Chandna, J, Procter, SR, et al. Prematurity modifies the risk of long-term neurodevelopmental impairments after invasive group B streptococcus infections during infancy in Denmark and the Netherlands. Clin Infect Dis. (2022) 74:S44–53. doi: 10.1093/cid/ciab774
24. Lohrmann, F, Hufnagel, M, Kunze, M, Afshar, B, Creti, R, Detcheva, A, et al. Neonatal invasive disease caused by Streptococcus agalactiae in Europe: the DEVANI multi-center study. Infection. Ahead of Print (2022):1–11. doi: 10.1007/s15010-022-01965-x
25. Aerts, C, Leahy, S, Mucasse, H, Lala, S, Bramugy, J, Tann, CJ, et al. Quantifying the acute care costs of neonatal bacterial Sepsis and meningitis in Mozambique and South Africa. Clin Infect Dis. (2022) 74:S64–9. doi: 10.1093/cid/ciab815
26. Salman, O, Procter, SR, McGregor, C, Paul, P, Hutubessy, R, Lawn, JE, et al. Systematic review on the acute cost-of-illness of Sepsis and meningitis in neonates and infants. Pediatr Infect Dis J. (2020) 39:35–40. doi: 10.1097/INF.0000000000002500
27. McLeod, E, Mason, C, and Swainston, K. Parents experiences of having an infant with early onset group B streptococcus infection. Br J Health Psychol. (2022) 27:777–88. doi: 10.1111/bjhp.12572
28. Russell, NJ, Seale, AC, O'Driscoll, M, O'Sullivan, C, Bianchi-Jassir, F, Gonzalez-Guarin, J, et al. Maternal colonization with group B streptococcus and serotype distribution worldwide: systematic review and Meta-analyses. Clin Infect Dis. (2017) 65:S100–11. doi: 10.1093/cid/cix658
29. Kwatra, G, Cunnington, MC, Merrall, E, Adrian, PV, Ip, M, Klugman, KP, et al. Prevalence of maternal colonisation with group B streptococcus: a systematic review and meta-analysis. Lancet Infect Dis. (2016) 16:1076–84. doi: 10.1016/S1473-3099(16)30055-X
30. Gizachew, M, Tiruneh, M, Moges, F, and Tessema, B. Streptococcus agalactiae maternal colonization, antibiotic resistance and serotype profiles in Africa: a meta-analysis. Ann Clin Microbiol Antimicrob. (2019) 18:14. doi: 10.1186/s12941-019-0313-1
31. Kwatra, G, Adrian, PV, Shiri, T, Buchmann, EJ, Cutland, CL, and Madhi, SA. Serotype-specific acquisition and loss of group B streptococcus recto-vaginal colonization in late pregnancy. PLoS One. (2014) 9:e98778. doi: 10.1371/journal.pone.0098778
32. McGee, L, Chochua, S, Li, Z, Mathis, S, Rivers, J, Metcalf, B, et al. Multistate, population-based distributions of candidate vaccine targets, clonal complexes, and resistance features of invasive group B streptococci within the United States, 2015-2017. Clin Infect Dis. (2021) 72:1004–13. doi: 10.1093/cid/ciaa151
33. Verani, JR, McGee, L, and Schrag, SJ. Prevention of perinatal group B streptococcal disease-revised guidelines from CDC, 2010. MMWR Recomm Rep. (2010) 59:1–36.
34. Russell, NJ, Seale, AC, O'Sullivan, C, Le Doare, K, Heath, PT, Lawn, JE, et al. Risk of early-onset neonatal group B streptococcal disease with maternal colonization worldwide: systematic review and meta-analyses. Clin Infect Dis. (2017) 65:S152–9. doi: 10.1093/cid/cix655
35. Fairlie, T, Zell, ER, and Schrag, S. Effectiveness of intrapartum antibiotic prophylaxis for prevention of early-onset group B streptococcal disease. Obstet Gynecol. (2013) 121:570–7. doi: 10.1097/AOG.0b013e318280d4f6
36. Dhudasia, MB, Flannery, DD, Pfeifer, MR, and Puopolo, KM. Updated guidance: prevention and management of perinatal group B streptococcus infection. NeoReviews. (2021) 22:e177–88. doi: 10.1542/neo.22-3-e177
37. Schrag, SJ, and Verani, JR. Intrapartum antibiotic prophylaxis for the prevention of perinatal group B streptococcal disease: experience in the United States and implications for a potential group B streptococcal vaccine. Vaccine. (2013) 31:D20–6. doi: 10.1016/j.vaccine.2012.11.056
38. Gopal Rao, G, Townsend, J, Stevenson, D, Nartey, G, Hiles, S, Bassett, P, et al. Early-onset group B streptococcus (EOGBS) infection subsequent to cessation of screening-based intrapartum prophylaxis: findings of an observational study in West London, UK. BMJ Open. (2017) 7:e018795. doi: 10.1136/bmjopen-2017-018795
39. Nishihara, Y, Dangor, Z, French, N, Madhi, S, and Heyderman, R. Challenges in reducing group B streptococcus disease in African settings. Arch Dis Child. (2017) 102:72–7. doi: 10.1136/archdischild-2016-311419
40. El Helali, N, Habibi, F, Azria, E, Giovangrandi, Y, Autret, F, Durand-Zaleski, I, et al. Point-of-care intrapartum group B streptococcus molecular screening: effectiveness and costs. Obstet Gynecol. (2019) 133:276–81. doi: 10.1097/AOG.0000000000003057
41. Ramesh Babu, S, McDermott, R, Farooq, I, Le Blanc, D, Ferguson, W, McCallion, N, et al. Screening for group B streptococcus (GBS) at labour onset using PCR: accuracy and potential impact - a pilot study. J Obstet Gynaecol. (2018) 38:49–54. doi: 10.1080/01443615.2017.1328490
42. Hanson, L, VandeVusse, L, Malloy, E, Garnier-Villarreal, M, Watson, L, Fial, A, et al. Probiotic interventions to reduce antepartum group B streptococcus colonization: a systematic review and meta-analysis. Midwifery. (2022) 105:103208. doi: 10.1016/j.midw.2021.103208
43. Torres, KAM, Lima, S, Torres, LMB, Gamberini, MT, and Silva Junior, PID. Garlic: an alternative treatment for group B streptococcus. Microbiol Spectr. (2021) 9:e0017021. doi: 10.1128/Spectrum.00170-21
44. Francis, JD, Guevara, MA, Lu, J, Madhi, SA, Kwatra, G, Aronoff, DM, et al. The antimicrobial activity of zinc against group B streptococcus is strain-dependent across diverse sequence types, capsular serotypes, and invasive versus colonizing isolates. BMC Microbiol. (2022) 22:23. doi: 10.1186/s12866-021-02428-3
45. Etti, M, Calvert, A, Galiza, E, Lim, S, Khalil, A, Le Doare, K, et al. Maternal vaccination: a review of current evidence and recommendations. Am J Obstet Gynecol. (2022) 226:459–74. doi: 10.1016/j.ajog.2021.10.041
46. Giles, ML, Davey, MA, and Wallace, EM. Associations between maternal immunisation and reduced rates of preterm birth and stillbirth: a population based retrospective cohort study. Front Immunol. (2021) 12:704254. doi: 10.3389/fimmu.2021.704254
47. Amirthalingam, G, Andrews, N, Campbell, H, Ribeiro, S, Kara, E, Donegan, K, et al. Effectiveness of maternal pertussis vaccination in England: an observational study. Lancet (London, England). (2014) 384:1521–8. doi: 10.1016/S0140-6736(14)60686-3
48. Nunes, MC, Cutland, CL, Jones, S, Hugo, A, Madimabe, R, Simoes, EA, et al. Duration of infant protection against influenza illness conferred by maternal immunization: secondary analysis of a randomized clinical trial. JAMA Pediatr. (2016) 170:840–7. doi: 10.1001/jamapediatrics.2016.0921
49. World Health Organization. Group B Streptococcus Vaccine Development Technology Roadmap. Priority Activities for Development, Testing, Licensure and Global Availability of Group B Streptococcus Vaccines. WHO. (2017).
50. World Health Organization. WHO Preferred Product Characteristics for Group B Streptococcus Vaccines. WHO. (2017).
51. World Health Organization. Group B Streptococcus Vaccine: Full Value of Vaccine Assessment. Executive Summary ; WHO. (2021).
52. McQuaid, F, Jones, C, Stevens, Z, Meddaugh, G, O'Sullivan, C, Donaldson, B, et al. Antenatal vaccination against group B streptococcus: attitudes of pregnant women and healthcare professionals in the UK towards participation in clinical trials and routine implementation. Acta Obstet Gynecol Scand. (2018) 97:330–40. doi: 10.1111/aogs.13288
53. Procter, SR, Gonçalves, BP, Paul, P, Chandna, J, Seedat, F, Koukounari, A, et al. Maternal immunisation against group B streptococcus: a global analysis of health impact and cost-effectiveness. PLoS Med. (2023) 20:e1004068. doi: 10.1371/journal.pmed.1004068
54. Kim, SY, Nguyen, C, Russell, LB, Tomczyk, S, Abdul-Hakeem, F, Schrag, SJ, et al. Cost-effectiveness of a potential group B streptococcal vaccine for pregnant women in the United States. Vaccine. (2017) 35:6238–47. doi: 10.1016/j.vaccine.2017.08.085
55. Russell, LB, Kim, SY, Cosgriff, B, Pentakota, SR, Schrag, SJ, Sobanjo-Ter Meulen, A, et al. Cost-effectiveness of maternal GBS immunization in low-income sub-Saharan Africa. Vaccine. (2017) 35:6905–14. doi: 10.1016/j.vaccine.2017.07.108
56. Ahmed, N, Giorgakoudi, K, Usuf, E, Okomo, U, Clarke, E, Kampmann, B, et al. Potential cost-effectiveness of a maternal group B streptococcal vaccine in the Gambia. Vaccine. (2020) 38:3096–104. doi: 10.1016/j.vaccine.2020.02.071
57. Kobayashi, M, McGee, L, Chochua, S, Apostol, M, Alden, NB, Farley, MM, et al. Low but increasing prevalence of reduced Beta-lactam susceptibility among invasive group B streptococcal isolates, US population-based surveillance, 1998-2018. Open Forum Infect Dis. (2021) 8:ofaa634. doi: 10.1093/ofid/ofaa634
58. Baker, CJ, and Kasper, DL. Correlation of maternal antibody deficiency with susceptibility to neonatal group B streptococcal infection. N Engl J Med. (1976) 294:753–6. doi: 10.1056/NEJM197604012941404
59. Kwatra, G, Adrian, PV, Shiri, T, Buchmann, EJ, Cutland, CL, and Madhi, SA. Natural acquired humoral immunity against serotype-specific group B streptococcus rectovaginal colonization acquisition in pregnant women. Clin Microbiol Infect. (2015) 21:568.e13–21. doi: 10.1016/j.cmi.2015.01.030
60. Dangor, Z, Kwatra, G, Izu, A, Lala, SG, and Madhi, SA. Review on the association of group B streptococcus capsular antibody and protection against invasive disease in infants. Expert Rev Vaccines. (2015) 14:135–49. doi: 10.1586/14760584.2014.953939
61. Dangor, Z, Lala, SG, Kwatra, G, and Madhi, SA. Group B streptococcus: developing a correlate of protection for a vaccine against neonatal infections. Curr Opin Infect Dis. (2016) 29:262–7. doi: 10.1097/QCO.0000000000000266
62. Le Doare, K, Kampmann, B, Vekemans, J, Heath, PT, Goldblatt, D, Nahm, MH, et al. Serocorrelates of protection against infant group B streptococcus disease. Lancet Infect Dis. (2019) 19:e162–71. doi: 10.1016/S1473-3099(18)30659-5
63. Madhi, SA, Izu, A, Kwatra, G, Jones, S, Dangor, Z, Wadula, J, et al. Association of group B streptococcus serum serotype-specific anti-capsular IgG concentration and risk reduction for invasive group B streptococcus disease in south African infants: an observational birth-cohort, matched case-control study. Clin Infect Dis. (2020) 73:e1170–80. doi: 10.1093/cid/ciaa1873
64. Dangor, Z, Kwatra, G, Pawlowski, A, Fisher, PB, Izu, A, Lala, SG, et al. Association of infant rib and Alp1 surface protein N-terminal domain immunoglobulin G and invasive group B streptococcal disease in young infants. Vaccine. (2023) 41:1679–83. doi: 10.1016/j.vaccine.2023.01.071
65. Baker, CJ, Carey, VJ, Rench, MA, Edwards, MS, Hillier, SL, Kasper, DL, et al. Maternal antibody at delivery protects neonates from early onset group B streptococcal disease. J Infect Dis. (2014) 209:781–8. doi: 10.1093/infdis/jit549
66. Fabbrini, M, Rigat, F, Rinaudo, CD, Passalaqua, I, Khacheh, S, Creti, R, et al. The protective value of maternal group B streptococcus antibodies: quantitative and functional analysis of naturally acquired responses to capsular polysaccharides and pilus proteins in European maternal sera. Clin Infect Dis. (2016) 63:746–53. doi: 10.1093/cid/ciw377
67. Dangor, Z, Kwatra, G, Izu, A, Khan, M, Lala, SG, and Madhi, SA. Infant serotype specific anti-capsular immunoglobulin G antibody and risk of invasive group B streptococcal disease. Vaccine. (2021) 39:6813–6. doi: 10.1016/j.vaccine.2021.10.022
68. Dzanibe, S, and Madhi, SA. Systematic review of the clinical development of group B streptococcus serotype-specific capsular polysaccharide-based vaccines. Expert Rev Vaccines. (2018) 17:635–51. doi: 10.1080/14760584.2018.1496021
69. Carreras-Abad, C, Ramkhelawon, L, Heath, PT, and Le Doare, K. A vaccine against group B streptococcus: recent advances. Infect Drug Resist. (2020) 13:1263–72. doi: 10.2147/IDR.S203454
70. Delara, M, Vadlamudi, NK, and Sadarangani, M. Strategies to prevent early and late-onset group B Streptococcal infection via interventions in pregnancy. Pathogens. (2023) 12:229. doi: 10.3390/pathogens12020229
71. Baker, CJ, Rench, MA, Edwards, MS, Carpenter, RJ, Hays, BM, and Kasper, DL. Immunization of pregnant women with a polysaccharide vaccine of group B streptococcus. N Engl J Med. (1988) 319:1180–5. doi: 10.1056/NEJM198811033191802
72. Baker, CJ, Rench, MA, and McInnes, P. Immunization of pregnant women with group B streptococcal type III capsular polysaccharide-tetanus toxoid conjugate vaccine. Vaccine. (2003) 21:3468–72. doi: 10.1016/S0264-410X(03)00353-0
73. Donders, GG, Halperin, SA, Devlieger, R, Baker, S, Forte, P, Wittke, F, et al. Maternal immunization with an investigational trivalent group B streptococcal vaccine: a randomized controlled trial. Obstet Gynecol. (2016) 127:213–21. doi: 10.1097/AOG.0000000000001190
74. Madhi, SA, Cutland, CL, Jose, L, Koen, A, Govender, N, Wittke, F, et al. Safety and immunogenicity of an investigational maternal trivalent group B streptococcus vaccine in healthy women and their infants: a randomised phase 1b/2 trial. Lancet Infect Dis. (2016) 16:923–34. doi: 10.1016/S1473-3099(16)00152-3
75. Heyderman, RS, Madhi, SA, French, N, Cutland, C, Ngwira, B, Kayambo, D, et al. Group B streptococcus vaccination in pregnant women with or without HIV in Africa: a non-randomised phase 2, open-label, multicentre trial. Lancet Infect Dis. (2016) 16:546–55. doi: 10.1016/S1473-3099(15)00484-3
76. Swamy, GK, Metz, TD, Edwards, KM, Soper, DE, Beigi, RH, Campbell, JD, et al. Safety and immunogenicity of an investigational maternal trivalent group B streptococcus vaccine in pregnant women and their infants: results from a randomized placebo-controlled phase II trial. Vaccine. (2020) 38:6930–40. doi: 10.1016/j.vaccine.2020.08.056
77. Fabbrini, M, Rigat, F, Tuscano, G, Chiarot, E, Donders, G, Devlieger, R, et al. Functional activity of maternal and cord antibodies elicited by an investigational group B streptococcus trivalent glycoconjugate vaccine in pregnant women. J Infect. (2018) 76:449–56. doi: 10.1016/j.jinf.2018.01.006
78. Madhi, SA, Koen, A, Cutland, CL, Jose, L, Govender, N, Wittke, F, et al. Antibody kinetics and response to routine vaccinations in infants born to women who received an investigational trivalent group B streptococcus polysaccharide CRM197-conjugate vaccine during pregnancy. Clin Infect Dis. (2017) 65:1897–904. doi: 10.1093/cid/cix666
79. Hillier, SL, Ferrieri, P, Edwards, MS, Ewell, M, Ferris, D, Fine, P, et al. A phase 2, randomized, control trial of group B streptococcus (GBS) type III capsular polysaccharide-tetanus toxoid (GBS III-TT) vaccine to prevent vaginal colonization with GBS III. Clin Infect Dis. (2019) 68:2079–86. doi: 10.1093/cid/ciy838
80. Dangor, Z, Khan, M, Kwatra, G, Izu, A, Nakwa, F, Ramdin, T, et al. The association between breast Milk group B streptococcal capsular antibody levels and late-onset disease in young infants. Clin Infect Dis. (2020) 70:1110–4. doi: 10.1093/cid/ciz360
81. Absalon, J, Segall, N, Block, SL, Center, KJ, Scully, IL, Giardina, PC, et al. Safety and immunogenicity of a novel hexavalent group B streptococcus conjugate vaccine in healthy, non-pregnant adults: a phase 1/2, randomised, placebo-controlled, observer-blinded, dose-escalation trial. Lancet Infect Dis. (2021) 21:263–74. doi: 10.1016/S1473-3099(20)30478-3
82. Madhi, SA, Dangor, Z, Heath, PT, Schrag, S, Izu, A, Sobanjo-Ter Meulen, A, et al. Considerations for a phase-III trial to evaluate a group B streptococcus polysaccharide-protein conjugate vaccine in pregnant women for the prevention of early- and late-onset invasive disease in young-infants. Vaccine. (2013) 31:D52–7. doi: 10.1016/j.vaccine.2013.02.029
83. Vekemans, J, Crofts, J, Baker, CJ, Goldblatt, D, Heath, PT, Madhi, SA, et al. The role of immune correlates of protection on the pathway to licensure, policy decision and use of group B streptococcus vaccines for maternal immunization: considerations from World Health Organization consultations. Vaccine. (2019) 37:3190–8. doi: 10.1016/j.vaccine.2019.04.039
84. Frasch, CE, Borrow, R, and Donnelly, J. Bactericidal antibody is the immunologic surrogate of protection against meningococcal disease. Vaccine. (2009) 27:B112–6. doi: 10.1016/j.vaccine.2009.04.065
85. World Health Organization Immunization. Vaccines and Biologicals W. Measuring Impact of Streptococcus Pneumoniae and Haemophilus influenzae type b Conjugate Vaccination. Geneva, Switzerland: Expanded Programme on Immunization (EPI) of the Department of Immunization, Vaccines and Biologicals (2012). 36 p.
86. Fischer, P, Pawlowski, A, Cao, D, Bell, D, Kitson, G, Darsley, M, et al. Safety and immunogenicity of a prototype recombinant alpha-like protein subunit vaccine (GBS-NN) against group B streptococcus in a randomised placebo-controlled double-blind phase 1 trial in healthy adult women. Vaccine. (2021) 39:4489–99. doi: 10.1016/j.vaccine.2021.06.046
87. Gonzalez-Miro, M, Pawlowski, A, Lehtonen, J, Cao, D, Larsson, S, Darsley, M, et al. Safety and immunogenicity of the group B streptococcus vaccine AlpN in a placebo-controlled double-blind phase 1 trial. iScience. (2023) 26:106261. doi: 10.1016/j.isci.2023.106261
88. Paul, P, Gonçalves, BP, Le Doare, K, and Lawn, JE. 20 million pregnant women with group B streptococcus carriage: consequences, challenges, and opportunities for prevention. Curr Opin Pediatr. (2023) 35:223–30. doi: 10.1097/MOP.0000000000001223
89. Woldesenbet, SA, Kufa, T, Lombard, C, Manda, S, Ayalew, K, Cheyip, M, et al. The 2017 National Antenatal Sentinel HIV Survey. National Department of Health: South Africa (2019).
90. Cools, P, van de Wijgert, J, Jespers, V, Crucitti, T, Sanders, EJ, Verstraelen, H, et al. Role of HIV exposure and infection in relation to neonatal GBS disease and rectovaginal GBS carriage: a systematic review and meta-analysis. Sci Rep. (2017) 7:13820. doi: 10.1038/s41598-017-13218-1
91. Manzanares, Á, Prieto-Tato, LM, Escosa-García, L, Navarro, M, Guillén, S, Penin, M, et al. Increased risk of group B streptococcal sepsis and meningitis in HIV-exposed uninfected infants in a high-income country. Eur J Pediatr. (2023) 182:575–9. doi: 10.1007/s00431-022-04710-6
92. Dangor, Z, Kwatra, G, Izu, A, Adrian, P, van Niekerk, N, Cutland, CL, et al. HIV-1 is associated with lower group B streptococcus capsular and surface-protein IgG antibody levels and reduced transplacental antibody transfer in pregnant women. J Infect Dis. (2015) 212:453–62. doi: 10.1093/infdis/jiv064
93. Dzanibe, S, Adrian, PV, Mlacha, SZK, Dangor, Z, Kwatra, G, and Madhi, SA. Reduced transplacental transfer of group B streptococcus surface protein antibodies in HIV-infected mother-Newborn dyads. J Infect Dis. (2017) 215:415–9. doi: 10.1093/infdis/jiw566
94. Cumberland, P, Shulman, CE, Maple, PA, Bulmer, JN, Dorman, EK, Kawuondo, K, et al. Maternal HIV infection and placental malaria reduce transplacental antibody transfer and tetanus antibody levels in newborns in Kenya. J Infect Dis. (2007) 196:550–7. doi: 10.1086/519845
95. Palmeira, P, Quinello, C, Silveira-Lessa, AL, Zago, CA, and Carneiro-Sampaio, M. IgG placental transfer in healthy and pathological pregnancies. Clin Dev Immunol. (2012) 2012:985646:1–13. doi: 10.1155/2012/985646
96. White, A, and Madhi, SA. Ethical considerations for designing GBS maternal vaccine efficacy trials in low-middle income countries. Vaccine. (2015) 33:6396–400. doi: 10.1016/j.vaccine.2015.07.108
97. Seale, AC, Bianchi-Jassir, F, Russell, NJ, Kohli-Lynch, M, Tann, CJ, Hall, J, et al. Estimates of the burden of group B streptococcal disease worldwide for pregnant women, stillbirths, and children. Clin Infect Dis. (2017) 65:S200–19. doi: 10.1093/cid/cix664
Keywords: Group B Streptococcus, Streptococcus agalactiae, early-onset disease, neonatal sepsis, perinatal infections
Citation: Dangor Z, Seale AC, Baba V and Kwatra G (2023) Early-onset group B streptococcal disease in African countries and maternal vaccination strategies. Front. Public Health. 11:1214844. doi: 10.3389/fpubh.2023.1214844
Edited by:
Lisa Frigati, Tygerberg Hospital, South AfricaReviewed by:
Tania Rivera-Hernandez, National Council of Science and Technology (CONACYT), MexicoCopyright © 2023 Dangor, Seale, Baba and Kwatra. This is an open-access article distributed under the terms of the Creative Commons Attribution License (CC BY). The use, distribution or reproduction in other forums is permitted, provided the original author(s) and the copyright owner(s) are credited and that the original publication in this journal is cited, in accordance with accepted academic practice. No use, distribution or reproduction is permitted which does not comply with these terms.
*Correspondence: Ziyaad Dangor, eml5YWFkLmRhbmdvckB3aXRzLmFjLnph