- 1Department of Physical Therapy, Faculty of Associated Medical Sciences, Chiang Mai University, Chiang Mai, Thailand
- 2Environmental-Occupational Health Sciences and Non Communicable Diseases Research Center, Research Institute for Health Sciences, Chiang Mai University, Chiang Mai, Thailand
Background: Despite surviving Coronavirus disease 2019 (COVID-19), its long-term impact is of concern. Low cardiorespiratory fitness is a strong predictor of all-cause mortality, and likely affected by multisystem impairments following COVID-19 infection. Accumulating evidence has identified the impact of COVID-19 on cardiorespiratory fitness level. However, the findings have been controversial. Conclusive evidence is still needed.
Objectives: This review aimed to systematically summarize and synthesize whether the SARS-CoV-2 infection diminishes cardiorespiratory fitness in COVID-19 survivors.
Design: The study design was a systematic review and meta-analysis.
Methods: A search was carried out using PubMed, CINAHL, Scopus, Embase and the Cochrane Library, together with reference lists (searching from their inception to January 2023). Observational studies investigating the impact of COVID-19 on outcomes relevant to cardiorespiratory fitness (i.e., peak oxygen uptake) were included. Weighted mean difference (WMD) and 95% confidence interval (CI) were used to identify a pooled effect estimate. Use of a random effects model was considered as the main method. Grading of Recommendation Assessment, Development and Evaluation approach was employed to determine the certainty of evidence. This meta-analysis was registered with PROSPERO (registration number: CRD42023393108).
Results: Seven eligible studies (4 cross-sectional, 2 cohort, and 1 case–control studies) involving 4,773 participants were included in this meta-analysis. A pooled effect estimates showed that patients in the surviving COVID-19 group had a significant reduction in peak oxygen uptake when compared to their counterparts in the non-COVID-19 group (WMD −6.70, 95%CI −9.34 to −4.06, low certainty). A subgroup analysis by age found that COVID-19 survivors in the young- to middle-aged and middle- to older-aged subgroups had significant reductions in peak oxygen uptake when compared to their counterparts in the non-COVID-19 group (WMD −5.31, 95%CI −7.69 to −2.94, low certainty; WMD −15.63, 95%CI −28.50 to −2.75, very low certainty, respectively). Subgroup analyses by symptom found that patients with moderate to severe symptoms in the surviving COVID-19 group had significantly lower peak oxygen uptake than their counterparts in the non-COVID-19 group (WMD −15.63, 95%CI −28.50 to −2.75, very low certainty).
Conclusion: The current meta-analysis concluded that patients in the COVID-19 survivors had poorer cardiorespiratory fitness than their counterparts in the non-COVID-19 group, but there is considerable uncertainty of evidence. Poorer cardiorespiratory fitness is likely to be more pronounced in COVID-19 survivors who are getting older and had severe symptoms, but it is uncertain whether such finding has a valuable in clinical context.
Systematic Review Registration: https://www.crd.york.ac.uk/PROSPERO/, CRD42023393108.
1. Introduction
Coronavirus disease 2019 (COVID-19), caused by the severe acute respiratory syndrome coronavirus 2 (SARS-CoV-2), has been of crucial health concern worldwide (1). A reported 757 million infected people, and more than 6 million deaths have been addressed from this pandemic (2). People infected with SARS-CoV-2 experience a wide range of symptoms, from asymptomatic to symptomatic (3). Since SARS-CoV-2 is the main pathogen of the human respiratory system, the most common clinical presentations include cough, fatigue and dyspnea (4). Apart from respiratory tract infection, COVID-19 plays a role in multiorgan impairment, such as impaired cardiac, vascular and skeletal muscle systems (5–7). Despite surviving COVID-19, its long-term impact is also of concern. Persistent symptoms that lasted for 3 months after the onset of illness are defined as long COVID-19 (8). Various reports have documented that long-term organ damages are attributed to immune-mediated response, inflammation, and viral persistence following COVID-19 infection (9). Such physiological alterations drive several specific long-term symptoms such as difficulty breathing, chest pain, tachycardia, and muscle weakness (10). Evidence has also shown a declined lung function in patients who recovered from COVID-19 infection, even in mild symptomatic and asymptomatic cases (11–13). In addition, a reduction in muscle strength has been observed in patients with long-COVID-19 syndrome (14). Approximately 36% of COVID-19 survivors experience long-term symptoms that persist at least 30 days after the onset of the COVID-19 infection (10) and some recovered patients undergo COVID-19-acquired complications (15). Persistence in these impairments is consequently linked to a reduction in functional capacity and decrease in quality of life (16, 17).
Cardiorespiratory fitness (CRF), used as a quantified measure of functional capacity, indicates an integrated function of respiratory, cardiovascular and musculoskeletal systems. It is well-established that low CRF is a strong predictor of all-cause mortality, including cardiovascular disease (CVD) (18, 19). American Heart Association has suggested CRF measurement in clinical practice since it is not only an independently CVD risk predictor, but also a complemented factor to traditional risk to improve CVD risk prediction (19). According to this, CRF in long COVID-19 patients has been recognized increasingly. Due to the sequelae of long-term multisystem impairments following SARS-CoV-2 infection, patients with long COVID-19 are likely to undergo increased CVD risk as a consequence of reduced CRF. A recent evidence synthesis has documented that severe symptoms were associated with advancing age (20). In addition, a very recent study has revealed that the more worsening symptoms, the lower CRF level (21). As the evidence suggests, it is likely that older age is a driving factor in further reducing CRF. A growing body of evidence has identified the impact of COVID-19 on CRF level. However, findings among existing studies have been controversial. While some evidence has revealed a decrease in CRF in confirmed COVID-19 patients (22, 23), other proof has failed to confirm low CRF in this group of patients (24). Conclusive evidence as to whether COVID-19 compromises the level of CRF is still needed. Thus, this study mainly aimed to systematically summarize and synthesize whether the SARS-CoV-2 infection diminishes CRF in COVID-19 survivors. Based on the evidence, we hypothesized that COVID-19 survivors would have a lower CRF level than individuals with non-COVID-19, especially in older age and severe symptom subgroups.
2. Methods
2.1. Search strategy
The systematic review and meta-analysis proceeded in accordance with the Preferred Reporting Items for Systematic reviews and Meta-Analyses (PRISMA) guidelines (Supplementary Table S1) (25). Five search engines including PubMed, CINAHL, Scopus, Embase, and the Cochrane Library (from their inception to January 2023), together with reference lists were used. All relevant studies were identified using the following search terms: (“covid” OR “coronavirus”) AND (“cardiorespiratory fitness” OR “physical fitness” OR “cardiopulmonary fitness” OR “fitness performance” OR “cardiorespiratory performance”) (Supplementary Table S2). This meta-analysis was registered in the PROSPERO database (https://www.crd.york.ac.uk/PROSPERO/, registration number: CRD42023393108).
2.2. Study selection
BC and SK screened the title and abstract independently. To obtain eligible studies, all of them were screened based on the PICOS criteria: We included observational studies investigating the impact of COVID-19 on outcomes relevant to CRF [i.e., peak oxygen uptake (VO2peak)], participants aged >18 years old, no gender restrictions, no language restrictions, and both published and unpublished studies. Studies conducted in an animal model and other study designs were excluded (Supplementary Table S3). Any disagreement between two reviewers was resolved by the third reviewer (SN).
2.3. Risk of bias (quality) assessment
Assessing risk of bias (ROB) was performed by BC and SK independently. The ROB of case–control and cohort studies were graded using the Newcastle-Ottawa Scale (NOS), while cross-sectional studies were evaluated using the Appraisal (AXIS) tool (26). The NOS contains eight items that are interpreted as good, fair or poor quality according to the scores assessed. The AXIS tool consists of 20-point questionnaires including five sections (introduction, methods, results, discussion and others) scored as “yes”, “no” or “do not know”. Discrepancy between the reviewers was resolved by the third reviewer (SN).
2.4. Assessment of certainty of evidence
Certainty of evidence was evaluated by two independent reviewers (BC and SK) using the Grading of Recommendation Assessment, Development and Evaluation (GRADE) approach (27). GRADE was determined based on the following domains: risk of bias, inconsistency, indirectness, imprecision, and publication bias. The certainty of evidence can be classified into four levels: high, moderate, low, and very low to indicate how confident that an effect estimate is close to the true effect.
2.5. Data extraction
SN and PS extracted the following information independently: author, year of publication, study design, participant details, continent, setting and CRF outcome (VO2peak). All extracted data were recorded in a Microsoft Excel program. Incomplete outcome data were resolved by sending an email to the authors of the study. If no response within a week, the incomplete publication was excluded. Any disagreement was resolved by consensus between the two reviewers.
2.6. Statistical analyses
CRF outcome was analyzed using a participant number, and mean and standard deviation (SD) in the exposure (i.e., confirmed COVID-19 group, outcome value after COVID-19 infection) and non-exposure groups (i.e., matched-control group, outcome value prior to COVID-19 infection). A pooled meta-analysis was identified by weighted mean difference (WMD) and 95% confidence interval (CI). Use of the random effect model was considered as a main method for analysis if there was substantial heterogeneity across studies presenting as I2 > 50%, with a p value of Cochrane’s Q < 0.1 (28). Finding out possible sources of substantial heterogeneity was attempted by performing subgroup analysis, which was carried out by age ranges and symptoms. To ensure robustness, generating sensitivity analysis was considered based on unclear characteristics of the outcome (i.e., estimated value of VO2peak) and participants (i.e., unmatched-control participants). Publication bias was measured by funnel plot, contour-enhanced funnel plot, Begg’s test, and Egger’s test. All analyses were performed using STATA Statistical Software, version 14.2 (StataCorp LP, United States).
3. Results
A total of 1,419 studies were retrieved from the five engine searches. Of these, 465 duplicates were removed and the 954 remaining studies were screened from title and abstract. Fifty remaining studies and two additional ones from reference lists were reviewed for more details. Among these, 45 were excluded due to no full text available (n = 1), other study types (n = 21), non-COVID-19 participants (n = 1), incomplete data (n = 2), no relevant outcome (n = 8), and no group for comparison (n = 12) (Supplementary Table S4). Thus, seven studies were finally eligible for meta-analysis. The PRISMA flow diagram is shown in Figure 1.
Details of the included studies are illustrated in Table 1. Seven eligible studies, involving 4,773 participants, were mostly cross-sectional (22, 24, 29, 30), while others were case–control (23), retrospective cohort (31, 32), and prospective cohort studies. The mean age of all participants was 40 years, and the majority were male (80.1%). Most of the studies were conducted in Europe (23, 24, 29–32), and one only in South America (22). The study settings comprised four studies containing hospital-based participants (22, 23, 29, 30), two including community-based participants (24, 31), and one that collected combined hospital and community-based participants (32). The symptoms of the participants varied, ranging from asymptomatic to severe symptomatic from COVID-19. All of the studies reported VO2peak as CRF outcome (22, 24, 29, 30, 32), except for two that reported estimated VO2peak (23, 31).
3.1. Risk of bias (quality) assessment
Of four cross-sectional studies, one met 16 out of 20 assessment criteria (24), two met 15 (22, 30), and one met 14 (29) (Table 2). Of these, no study had provided sample size justification. For case–control and cohort studies, one had good quality (23), while the other two had fair quality (31, 32) (Table 3).
3.2. Long-term impact of COVID-19 on cardiorespiratory fitness
CRF was reported in all of the studies as amount of VO2peak. From the seven studies, pooled effect estimates showed that patients surviving COVID-19 (COVID-19 group) had a significant 6.70 milliliter (mL) reduction in VO2peak, compared to their counterparts in the non-COVID-19 group (WMD −6.70, 95%CI −9.34 to −4.06, I2 93.9%, 7 studies, low certainty) (Figure 2).
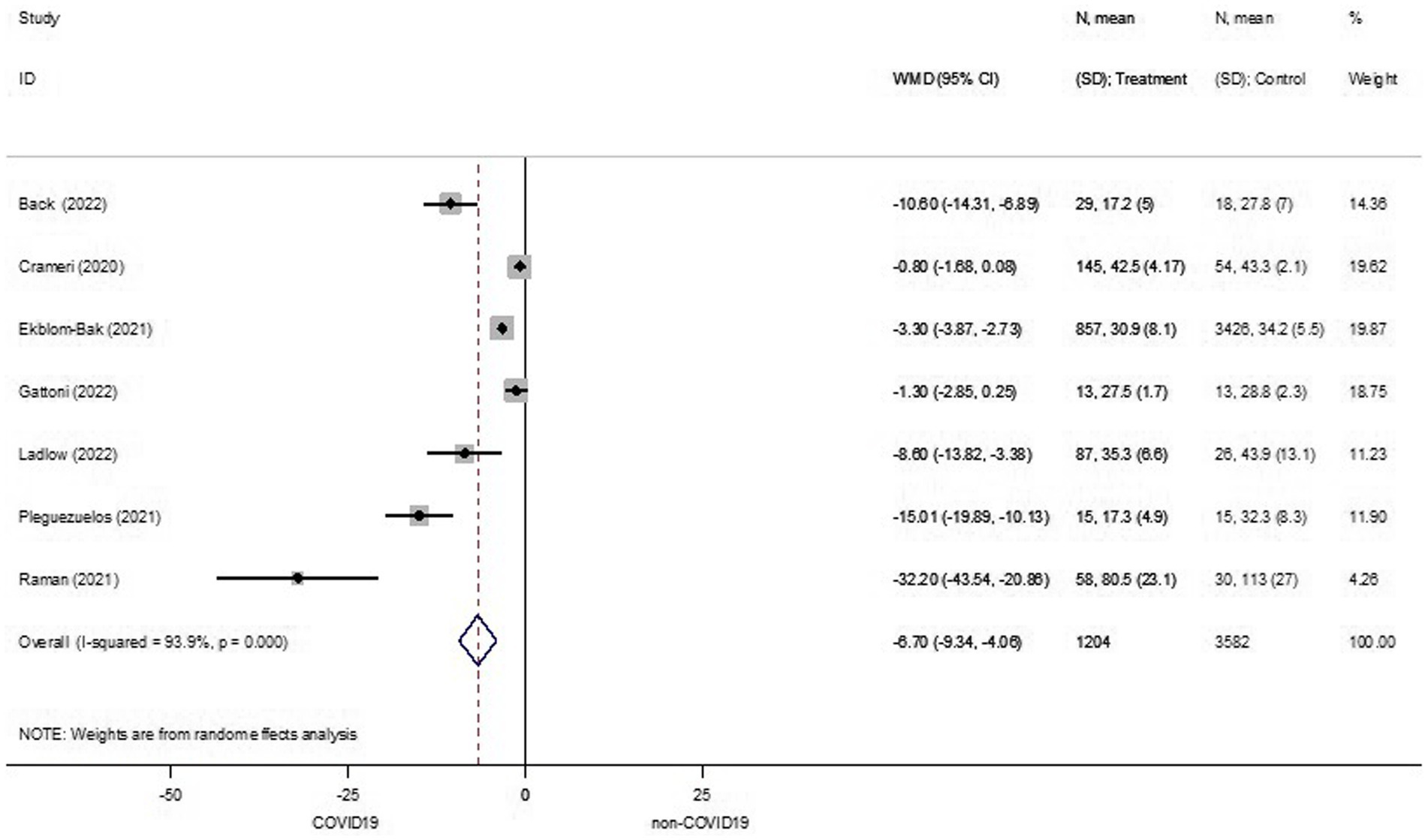
Figure 2. Cardiorespiratory fitness between COVID-19 and non-COVID-19 groups. ID, identification; N, number of participants; SD, standard deviation; WMD, weighted mean difference.
According to substantial heterogeneity across the studies, subgroup analyses were carried out based on age ranges and symptoms. Age ranges were separated into four subgroups, including young-aged, middle-aged, young to middle-aged, and middle to older aged. The young-aged subgroup in the COVID-19 group had a similar VO2peak to their counterparts in the non-COVID-19 group (WMD −2.01, 95%CI −4.19 to 0.17, I2 76.3%, 3 studies, low certainty) (Supplementary Figure S1). In line with the young-aged subgroup, the middle-aged subgroup in the COVID-19 group had the same VO2peak as their counterparts in the non-COVID-19 group (WMD −8.89, 95%CI −20.36 to 2.57, I2 95.4%, 2 studies, low certainty) (Supplementary Figure S1). The young- to middle-aged subgroup had a significant reduction of 5.31 mL in VO2peak in the COVID-19 group when compared to the non-COVID-19 group (WMD −5.31, 95%CI −7.69 to −2.94, I2 93.1%, 6 studies, low certainty) (Supplementary Figure S2). The middle- to older-aged subgroup also had a significantly lower VO2peak in the COVID-19 group when compared to the non-COVID-19 group (WMD −15.63, 95%CI −28.50 to −2.75, I2 95.7%, 3 studies, very low certainty) (Supplementary Figure S3). Subgroup analyses by symptom included mild to moderate, moderate to severe, and severe subgroups. Among the mild to moderate and severe subgroups, VO2peak was equal between those in the COVID-19 and non-COVID-19 groups (WMD −5.79, 95%CI −14.90 to 3.32, I2 95.1%, 2 studies, low certainty; WMD −8.89, 95%CI −20.36 to 2.57, I2 95.4%, 2 studies, very low certainty, respectively) (Supplementary Figures S4, S5). However, patients who had moderate to severe COVID-19 symptoms had significantly lower VO2peak than those in the non-COVID-19 group (WMD −15.63, 95%CI −28.50 to −2.75, I2 95.7%, 3 studies, very low certainty) (Supplementary Figure S6).
Two of the studies reported an estimated VO2peak rather than a measured one. Due to uncertain characteristics of outcome, a sensitivity analysis was performed to ensure robustness by removing (23, 31). After these omissions, patients in the COVID-19 group had significantly reduced VO2peak than their counterparts in the non-COVID-19 one (WMD −12.25, 95%CI −20.24 to −4.25, I2 95.5%, 5 studies, low certainty) (Supplementary Figure S7). Such sensitivity analysis showed a trend toward decreased VO2peak in the COVID-19 group when compared to the non-COVID-19 one. Sensitivity analysis was performed further by eliminating (32), due to unmatched-control participants. After this exclusion, the pooled effect estimates revealed a significantly lower VO2peak in the COVID-19 group than in the non-COVID one (WMD −6.43, 95%CI −9.21 to −3.66, I2 94.6%, 6 studies, low certainty) (Supplementary Figure S8).
3.3. Publication bias of the included studies
Assessment of publication bias of the studies was carried out (Supplementary Figure S9). There was a possible publication bias where asymmetry of funnel plot was expressed (Supplementary Figure S9A). However, contour-enhanced funnel plot revealed no publication bias, as most missing studies were in an area of high statistical significance (p < 0.01) (33) (Supplementary Figure S9B). In addition, Begg’s test and Egger’s test had no significant differences (p = 0.051 and 0.096, respectively) (Supplementary Figure S9C).
4. Discussion
This review aimed to systematically summarize and synthesize whether the SARS-CoV-2 infection diminishes CRF in patients who have recovered from COVID-19. The main findings of this review were (1) the COVID-19 survivors had poorer CRF when compared to the non-COVID-19 one, (2) poorer CRF was presented in the young- to middle-aged subgroup and was more pronounced in the middle- to older-aged subgroup, and (3) the COVID-19 survivors that had moderate to severe symptoms had lower CRF than the non-COVID-19 group, while the COVID-19 survivors with severe symptoms alone had a similar CRF level when compared with the non-COVID-19 group.
The long-term impact of COVID-19 on health status is pronounced despite recovering from it. Persistent multiorgan impairment is possibly associated with a declined CRF, which is a factor determining reduced functional ability, poor quality of life (16, 17), and increased all-cause mortality (18, 19). The meta-analysis in this study confirms poorer CRF as indicated by lower VO2peak in patients recovering from COVID-19 when compared to those in the non-COVID-19 group. A reduction in VO2peak is possibly attributed to either one or an interplay among impaired pulmonary, cardiovascular and skeletal musculature systems (34). Given available evidence, prolonged impaired diffusing capacity of the lungs for carbon monoxide (DLCO), as a consequence of COVID-19-induced alveolar-capillary damage, plays a major role in impaired pulmonary function in discharged COVID-19 patients (35). Functional changes in the cardiac system, as reflected by a lower stroke volume (36–38), chronotropic incompetence and lower end-diastolic volume, have been observed further in COVID-19 patients (38). A recent review provided additional information that COVID-19 patients experience muscular damage, which is produced by disease-induced changes (i.e., inflammatory effects, cytokine storm and muscle catabolism), pharmacotherapy (i.e., corticotherapy) and prolonged immobility (39).
In the current meta-analysis, poorer CRF in surviving COVID-19 patients is also related to age and disease severity. Regarding age groups, our study revealed lower VO2peak in all age ranges in the surviving COVID-19 group, especially in the middle-aged to older adult subgroup. Previous evidence documented that an increase in 1-metabolic equivalent (MET), as reflected by a VO2peak of 3.5 mL/kg/min, associated with a 13% decrease in all-cause mortality and 15% reduction in cardiovascular disease in a healthy population (40), and 12% improvement in survival rate of the older adult (41). Based on previous evidence, this review found that a 5.31 mL reduction in VO2peak in the young- to middle-aged subgroup suggests a 20% increase in all-cause mortality, 23% increase in cardiovascular disease, and 18% reduction in survival rate. These negative impacts are more pronounced in middle- to older aged COVID-19 patients, as reflected by a 15.63 mL decrease in VO2peak. The degree of severity in COVID-19 symptoms is also accounted for as a factor contributing to long-covid impact. This study found a lower VO2peak in the COVID-19 group with moderate to severe symptoms. We note that COVID-19 survivors in advancing age had more severe symptoms whereas younger had less worsening symptoms. Lower CRF as a consequence of severe symptoms among middle- to older aged group may be explained that progressive deterioration of protective immune response is accompanied with increasing age, resulting in susceptibility to infection and experiencing more tissue-damaging inflammation (42). Obesity and physical activity status have additionally been proposed as factors for worsening symptoms (21, 43). Among included studies, COVID-19 survivors with advancing age and more severe symptoms were obese (23, 29, 30). On the other hand, younger participants with less severe symptoms had normal weight (24, 31). Our findings are in line with the previous evidence illustrating a lower CRF in hospitalized COVID-19 individuals with obesity. Excessive fat causes lower immune response via a systemic activation of hyperinflammatory promotion and the secretion of proinflammatory mediators (44). COVID-19 survivors with advancing age and worsening symptoms further had low level of physical activity. This finding is in line with a recent study that reported low perception of performing activity reflected by a poorer physical component score of health-related quality of life in severe COVID-19 patients (45). Large epidemiology has also documented that physical activity was inversely associated with severe COVID-19 (46). Physical activity is accounted as an efficient intervention for reversing an inflammatory process (47) and cardioprotective effect in COVID-19 survivors (43). In the context of current review, sufficient physical activity (≥150 min a week of moderate intensity) can impede viral entry to the targeted cell by increase in plasma soluble angiotensin-converting enzyme 2 (sACE2), which can bind to SARS-CoV-2 (43). A previous study has also supported a cardioprotective effect of a higher physical activity level as they found a significantly greater ventilatory efficient and tissue oxygen uptake in elite athletes than recreational athletes (48). Interestingly, the meta-analysis in this study failed to identify lower CRF in patients with severe symptoms when compared to the non-COVID-19 group. It is possible that those patients were in the middle-aged range, in which patients are likely to recover more quickly (23, 29). Based on the findings in this study, it was speculated that the detrimental effect of COVID-19 infection on CRF is down to coexistence of age and symptoms rather than symptoms alone.
Although the current meta-analysis showed poorer CRF among COVID-19 survivors, confidence of evidence may be reduced as it had low certainty of evidence. Low certainty suggests COVID-19 survivors may have poorer CRF than non-COVID-19 group. Decrease in the evidence certainty is attributed to serious inconsistency due to considerably heterogeneity and serious imprecision due to wide ranges of confident interval. In addition, the confidence that poorer CRF in COVID-19 survivors with getting older and more severe symptoms are likely to reduce due to very low certainty. It is uncertain whether older and severe symptoms are prone to diminish CRF in COVID-19 survivors. Factors contributing to rating down the certainty are serious inconsistency, serious imprecision, and serious risk of bias. For the serious risk of bias, body mass index distinction between groups is accounted as a potential confounding factor (23, 29) which downgrades the certainty of evidence. BMI has been identified as one of predictors of VO2peak (49). Unequal BMI may distort the true effect of COVID-19 on CRF level. Thus, it should be interpreted with caution.
This is the first study to synthesize the long-term impact of COVID-19 on CRF alteration. Possible biases were eliminated by carrying out comprehensive and exhaustive searches, reviewing all available languages, and performing a review process independently by the reviewers. Furthermore, the effect estimates on CRF are likely valid, as quality of all the studies was fair to good (for case–control and cohort studies) and met the ≥70% criteria required (for cross-sectional studies). Despite the strength of this study, there were some limitations that should be considered. First, apparent heterogeneity (I2 = 93.9%) across the studies may have limited reporting, as it might have had some clinical or methodological heterogeneity. This was resolved with the randoms effect model and subgroup analysis that explored the possible source of heterogeneity. Second, two of the included studies reported an estimated VO2peak, which might reflect an unreal VO2peak. Third, COVID-19 symptoms and their duration were not identified clearly in some of the studies, which may account for confounding factors influencing statistical analysis. Last, the precise effect may be different from the estimated effect due to low to very low certainty of evidence.
5. Conclusion
The current meta-analysis concluded that the COVID-19 group had poorer CRF than the non-COVID-19 group. Poorer CRF is likely to be more pronounced in the COVID-19 group that is getting older and having severe symptoms. The findings in this study pointed out the need to include the measurement of CRF status in clinical practice, provide suitable intervention for improving CRF and quality of life, and reduce all-cause mortality.
For a practical implication, the available evidence was limited to conclusively recommend or deny carrying out CRF assessment in COVID-19 survivors. Low certainty of evidence limits confidence about poorer CRF in COVID-19 survivors. Very low certainty of evidence also restricts the confidence of lower CRF in COVID-19 survivors who are getting older and having severe symptoms. Further well-designed research and much more data are required to prove this issue.
Data availability statement
The original contributions presented in the study are included in the article/Supplementary material, further inquiries can be directed to the corresponding author.
Author contributions
BC, SK, PS, and SN: conceptual framework, and drafting and revising manuscript. BC and SK: search strategy. BC, SK, and SN: study selection, risk of bias, and quality assessment. PS and SN: data extraction. BC and SN: statistical analysis. All authors contributed to the article and approved the submitted version.
Acknowledgments
This study was partially supported by Chiang Mai University. We would like to thank Voravit Suwanvanichkij for English editing.
Conflict of interest
The authors declare that the research was conducted in the absence of any commercial or financial relationships that could be construed as a potential conflict of interest.
Publisher’s note
All claims expressed in this article are solely those of the authors and do not necessarily represent those of their affiliated organizations, or those of the publisher, the editors and the reviewers. Any product that may be evaluated in this article, or claim that may be made by its manufacturer, is not guaranteed or endorsed by the publisher.
Supplementary material
The Supplementary material for this article can be found online at: https://www.frontiersin.org/articles/10.3389/fpubh.2023.1215486/full#supplementary-material
References
1. World Health Organization. Coronavirus disease 2019 (COVID-19) situation report 51. Geneva. Available at: https://www.who.int/docs/default-source/coronaviruse/situation-reports/20200311-sitrep-51-covid-19.pdf?sfvrsn=1ba62e57_10: WHO (2020).
2. World Health Organization. Coronavirus disease (COVID-19) pandemic. Available at: https://www.who.int/emergencies/diseases/novel-coronavirus-2019 ().
3. Kowalik, MM, Trzonkowski, P, Łasińska-Kowara, M, Mital, A, Smiatacz, T, and Jaguszewski, M. COVID-19—toward a comprehensive understanding of the disease. Cardiol J. (2020) 27:99–114. doi: 10.5603/CJ.a2020.0065
4. Huang, C, Wang, Y, Li, X, Ren, L, Zhao, J, and Hu, Y. Clinical features of patients infected with 2019 novel coronavirus in Wuhan. Lancet. (2020) 395:497–506. doi: 10.1016/S0140-6736(20)30183-5
5. Rath, D, Petersen-Uribe, Á, Avdiu, A, Witzel, K, Jaeger, P, Zdanyte, M, et al. Impaired cardiac function is associated with mortality in patients with acute COVID-19 infection. Clin Res Cardiol. (2020) 109:1491–9. doi: 10.1007/s00392-020-01683-0
6. Gille-Johnson, P, Kessler, I, and Tehrani, S. Impaired microvascular function in patients with critical COVID-19. Crit Care Explor. (2022) 4:e0643. doi: 10.1097/CCE.0000000000000643
7. Dos Santos, PK, Sigoli, E, Bragança, LJG, and Cornachione, AS. The musculoskeletal involvement after mild to moderate COVID-19 infection. Front Physiol. (2022) 13:813924. doi: 10.3389/fphys.2022.813924
8. World Health Organization. Coronavirus disease (COVID-19): post COVID-19 condition. Geneva. Available at: https://www.who.int/news-room/questions-and-answers/item/coronavirus-disease-(covid-19)-post-covid-19-condition#:~:text=Post%20COVID%2D19%20condition%20is,falls%20ill%20with%20COVID%2D19: WHO (2023).
9. Davis, HE, McCorkell, L, Vogel, JM, and Topol, EJ. Long COVID: major findings, mechanisms and recommendations. Nat Rev Microbiol. (2023) 21:133–46. doi: 10.1038/s41579-022-00846-2
10. Cirulli, E, Barrett, KMS, Riffle, S, Bolze, A, Neveux, I, Dabe, S, et al. Long-term COVID-19 symptoms in a large unselected population. medrxiv. (2020). doi: 10.1101/2020.10.07.20208702
11. van den Borst, B, Peters, JB, Brink, M, Schoon, Y, Bleeker-Rovers, CP, Schers, H, et al. Comprehensive health assessment 3 months after recovery from acute coronavirus disease 2019 (COVID-19). Clin Infect Dis. (2021) 73:e1089–98. doi: 10.1093/cid/ciaa1750
12. Sonnweber, T, Sahanic, S, Pizzini, A, Luger, A, Schwabl, C, Sonnweber, B, et al. Cardiopulmonary recovery after COVID-19: an observational prospective multicentre trial. Eur Respir J. (2021) 57:2003481. doi: 10.1183/13993003.03481-2020
13. Iversen, KK, Afzal, S, Ahlström, MG, Nordestgaard, BG, Schneider, UV, Nielsen, L, et al. Lung function decline in relation to COVID-19 in the general population: a matched cohort study with prepandemic assessment of lung function. J Infect Dis. (2022) 225:1308–16. doi: 10.1093/infdis/jiab636
14. Ramírez-Vélez, R, Legarra-Gorgoñon, G, Oscoz-Ochandorena, S, García-Alonso, Y, García-Alonso, N, Oteiza, J, et al. Reduced muscle strength in patients with long-COVID-19 syndrome is mediated by limb muscle mass. J Appl Physiol (1985). (2023) 134:50–8. doi: 10.1152/japplphysiol.00599.2022
15. World Health Organization. (2020). What we know about long-term effects of COVID-19 (coronavirus update 36). Available at: https://www.who.int/publications/m/item/update-36-long-term-effects-of-covid-19 ().
16. Eisner, MD, Iribarren, C, Yelin, EH, Sidney, S, Katz, PP, Ackerson, L, et al. Pulmonary function and the risk of functional limitation in chronic obstructive pulmonary disease. Am J Epidemiol. (2008) 167:1090–101. doi: 10.1093/aje/kwn025
17. van der Sar-van der Brugge, S, Talman, S, Boonman-de Winter, L, de Mol, M, Hoefman, E, van Etten, RW, et al. Pulmonary function and health-related quality of life after COVID-19 pneumonia. Respir Med. (2021) 176:106272. doi: 10.1016/j.rmed.2020.106272
18. Blair, SN, Kohl, HW 3rd, Paffenbarger, RS Jr, Clark, DG, Cooper, KH, and Gibbons, LW. Physical fitness and all-cause mortality. A prospective study of healthy men and women. JAMA. (1989) 262:2395–401. doi: 10.1001/jama.1989.03430170057028
19. Ross, R, Blair, SN, Arena, R, Church, TS, Després, JP, Franklin, BA, et al. Importance of assessing cardiorespiratory fitness in clinical practice: a case for fitness as a clinical vital sign: a scientific statement from the American Heart Association. Circulation. (2016) 134:e653–99. doi: 10.1161/CIR.0000000000000461
20. Dessie, ZG, and Zewotir, T. Mortality-related risk factors of COVID-19: a systematic review and meta-analysis of 42 studies and 423, 117 patients. BMC Infect Dis. (2021) 21:855. doi: 10.1186/s12879-021-06536-3
21. Lemos, MM, Cavalini, GR, Pugliese Henrique, CR, Perli, VAS, de Moraes, MG, Marchiori, LLM, et al. Body composition and cardiorespiratory fitness in overweight or obese people post COVID-19: a comparative study. Front Physiol. (2022) 13:949351. doi: 10.3389/fphys.2022.949351
22. Back, GD, Oliveira, MR, Camargo, PF, Goulart, CL, Oliveira, CR, Wende, KW, et al. Mild-to-moderate COVID-19 impact on the cardiorespiratory fitness in young and middle-aged populations. Braz J Med Biol Res. (2022) 55:e12118. doi: 10.1590/1414-431X2022e12118
23. Ekblom-Bak, E, Väisänen, D, Ekblom, B, Blom, V, Kallings, LV, Hemmingsson, E, et al. Cardiorespiratory fitness and lifestyle on severe COVID-19 risk in 279, 455 adults: a case control study. Int J Behav Nutr Phys Act. (2021) 18:135. doi: 10.1186/s12966-021-01198-5
24. Crameri, GAG, Bielecki, M, Züst, R, Buehrer, TW, Stanga, Z, and Deuel, JW. Reduced maximal aerobic capacity after COVID-19 in young adult recruits, Switzerland, may 2020. Euro Surveill. (2020) 25:2001542. doi: 10.2807/1560-7917.ES.2020.25.36.2001542
25. Page, MJ, McKenzie, JE, Bossuyt, PM, Boutron, I, Hoffmann, TC, Mulrow, CD, et al. The PRISMA 2020 statement: an updated guideline for reporting systematic reviews. BMJ. (2021) 372:n71. doi: 10.1136/bmj.n71
26. Ma, LL, Wang, YY, Yang, ZH, Huang, D, Weng, H, and Zeng, XT. Methodological quality (risk of bias) assessment tools for primary and secondary medical studies: what are they and which is better? Mil Med Res. (2020) 7:7. doi: 10.1186/s40779-020-00238-8
27. Schünemann, HJ, Cuello, C, Akl, EA, Mustafa, RA, Meerpohl, JJ, Thayer, K, et al. GRADE guidelines: 18. How ROBINS-I and other tools to assess risk of bias in nonrandomized studies should be used to rate the certainty of a body of evidence. J Clin Epidemiol. (2019) 111:105–14. doi: 10.1016/j.jclinepi.2018.01.012
28. Higgins, JPT, Thompson, SG, Deeks, JJ, and Altman, DG. Measuring inconsistency in meta-analyses. BMJ. (2003) 327:557–60. doi: 10.1136/bmj.327.7414.557
29. Pleguezuelos, E, Del Carmen, A, Llorensi, G, Carcole, J, Casarramona, P, Moreno, E, et al. Severe loss of mechanical efficiency in COVID-19 patients. J Cachexia Sarcopenia Muscle. (2021) 12:1056–63. doi: 10.1002/jcsm.12739
30. Raman, B, Cassar, MP, Tunnicliffe, EM, Filippini, N, Griffanti, L, Alfaro-Almagro, F, et al. Medium-term effects of SARS-CoV-2 infection on multiple vital organs, exercise capacity, cognition, quality of life and mental health, post-hospital discharge. EClinicalMedicine. (2021) 31:100683. doi: 10.1016/j.eclinm.2020.100683
31. Gattoni, C, Conti, E, Casolo, A, Nuccio, S, Baglieri, C, Capelli, C, et al. COVID-19 disease in professional football players: symptoms and impact on pulmonary function and metabolic power during matches. Physiol Rep. (2022) 10:e15337. doi: 10.14814/phy2.15337
32. Ladlow, P, O’Sullivan, O, Bennett, AN, Barker-Davies, R, Houston, A, Chamley, R, et al. The effect of medium-term recovery status after COVID-19 illness on cardiopulmonary exercise capacity in a physically active adult population. J Appl Physiol. (2022) 132:1525–35. doi: 10.1152/japplphysiol.00138.2022
33. Peters, JL, Sutton, AJ, Jones, DR, Abrams, KR, and Rushton, L. Contour-enhanced meta-analysis funnel plots help distinguish publication bias from other causes of asymmetry. J Clin Epidemiol. (2008) 61:991–6. doi: 10.1016/j.jclinepi.2007.11.010
34. The, WK, and Dickinson, W. Richards lecture. New concepts in assessing cardiovascular function. Circulation. (1988) 78:1060–71. doi: 10.1161/01.CIR.78.4.1060
35. Chen, R, Gao, Y, Chen, M, Jian, W, Lei, C, Zheng, J, et al. Impaired pulmonary function in discharged patients with COVID-19: more work ahead. Eur Respir J. (2020) 56:2002194. doi: 10.1183/13993003.02194-2020
36. Cassar, MP, Tunnicliffe, EM, Petousi, N, Lewandowski, AJ, Xie, C, Mahmod, M, et al. Symptom persistence despite improvement in cardiopulmonary health – insights from longitudinal CMR, CPET and lung function testing post-COVID-19. EClinicalMedicine. (2021) 41:101159. doi: 10.1016/j.eclinm.2021.101159
37. Baratto, C, Caravita, S, Faini, A, Perego, GB, Senni, M, Badano, LP, et al. Impact of COVID-19 on exercise pathophysiology: a combined cardiopulmonary and echocardiographic exercise study. J Appl Physiol. (2021) 130:1470–8. doi: 10.1152/japplphysiol.00710.2020
38. Szekely, Y, Lichter, Y, Sadon, S, Lupu, L, Taieb, P, Banai, A, et al. Cardiorespiratory abnormalities in patients recovering from coronavirus disease 2019. J Am Soc Echocardiogr. (2021) 34:1273–1284.e9. doi: 10.1016/j.echo.2021.08.022
39. Pescaru, CC, Marițescu, A, Costin, EO, Trăilă, D, Marc, MS, Trușculescu, AA, et al. The effects of COVID-19 on skeletal muscles, muscle fatigue and rehabilitation programs outcomes. Medicina (Kaunas). (2022) 58:1199. doi: 10.3390/medicina58091199
40. Kodama, S, Saito, K, Tanaka, S, Maki, M, Yachi, Y, Asumi, M, et al. Cardiorespiratory fitness as a quantitative predictor of all-cause mortality and cardiovascular events in healthy men and women: a meta-analysis. JAMA. (2009) 301:2024–35. doi: 10.1001/jama.2009.681
41. Myers, J, Prakash, M, Froelicher, V, Do, D, Partington, S, and Atwood, JE. Exercise capacity and mortality among men referred for exercise testing. N Engl J Med. (2002) 346:793–801. doi: 10.1056/NEJMoa011858
42. Weyand, CM, and Goronzy, JJ. Aging of the immune system. Mechanisms and therapeutic targets. Ann Am Thorac Soc. (2016) 13:S422–8. doi: 10.1513/AnnalsATS.201602-095AW
43. Hagiu, BA. Moderate exercise may prevent the development of severe forms of COVID-19, whereas high-intensity exercise may result in the opposite. Med Hypotheses. (2021) 157:110705. doi: 10.1016/j.mehy.2021.110705
44. Maffetone, PB, and Laursen, PB. The perfect storm: coronavirus (Covid-19) pandemic meets overfat pandemic. Front Public Health. (2020) 8:135. doi: 10.3389/fpubh.2020.00135
45. Alnasser, AHA, Al-Tawfiq, JA, Al Kalif, MSH, Alburaih, JAH, AlHamad, LAA, Abadi, AAA, et al. Impact of COVID-19 severity on health-related quality of life among Saudi adult patients. Infez Med. (2022) 30:223–30. doi: 10.53854/liim-3002-7
46. Sallis, R, Young, DR, Tartof, SY, Sallis, JF, Sall, J, Li, Q, et al. Physical inactivity is associated with a higher risk for severe COVID-19 outcomes: a study in 48 440 adult patients. Br J Sports Med. (2021) 55:1099–105. doi: 10.1136/bjsports-2021-104080
47. Sordi, AF, Lemos, MM, de Souza Marques, DC, Ryal, JJ, Priscila de Paula Silva Lalucci, M, Marques, MG, et al. Effects of a multi-professional intervention on body composition, physical fitness and biochemical markers in overweight COVID-19 survivors: a clinical trial. Front Physiol. (2023) 14:1219252. doi: 10.3389/fphys.2023.1219252
48. Wernhart, S, Weihe, E, Totzeck, M, Balcer, B, Rassaf, T, and Luedike, P. Cardiopulmonary profiling of athletes with post-exertional malaise after COVID-19 infection-a single-center experience. J Clin Med. (2023) 12:4348. doi: 10.3390/jcm12134348
Keywords: age, COVID-19, cardiorespiratory fitness, meta-analysis, peak oxygen uptake, SAR-CoV-2, symptom
Citation: Chuatrakoon B, Konghakote S, Sa-nguanmoo P and Nantakool S (2023) Long-term impact of SARS-CoV-2 infection on cardiorespiratory fitness: a meta-analysis. Front. Public Health. 11:1215486. doi: 10.3389/fpubh.2023.1215486
Edited by:
Agieshkumar Balakrishna Pillai, Sri Balaji Vidyapeeth University, IndiaReviewed by:
Manoharan P. S., Sri Balaji Vidyapeeth University, IndiaBraulio Branco, Centro Universitário Cesumar, Brazil
Pablo Valdés-Badilla, Universidad Católica del Maule, Chile
Copyright © 2023 Chuatrakoon, Konghakote, Sa-nguanmoo and Nantakool. This is an open-access article distributed under the terms of the Creative Commons Attribution License (CC BY). The use, distribution or reproduction in other forums is permitted, provided the original author(s) and the copyright owner(s) are credited and that the original publication in this journal is cited, in accordance with accepted academic practice. No use, distribution or reproduction is permitted which does not comply with these terms.
*Correspondence: Sothida Nantakool, c290aGlkYW5hbnRha29vbEBnbWFpbC5jb20=