- 1Abu Dhabi Public Health Center, Abu Dhabi, United Arab Emirates
- 2Department of Pathology and Infectious Diseases, Khalifa University, Abu Dhabi, United Arab Emirates
- 3Emirates Health Services Establishment, Dubai, United Arab Emirates
- 4Public Health Sector, Ministry of Health and Prevention, Dubai, United Arab Emirates
- 5Biotechnology Research Center, Khalifa University, Abu Dhabi, United Arab Emirates
- 6Infection Research Unit, Khalifa University, Abu Dhabi, United Arab Emirates
- 7College of Medicine, Mohammed Bin Rashid University of Medicine and Health Sciences, Dubai, United Arab Emirates
- 8School of Dentistry, Cardiff University, Cardiff, United Kingdom
- 9Department of Medical Microbiology and Immunology, RAK Medical and Health Sciences University, Ras Al Khaimah, United Arab Emirates
- 10College of Natural and Health Sciences, Zayed University, Dubai, United Arab Emirates
Introduction: Acinetobacter spp., in particular A. baumannii, are opportunistic pathogens linked to nosocomial pneumonia (particularly ventilator-associated pneumonia), central-line catheter-associated blood stream infections, meningitis, urinary tract infections, surgical-site infections, and other types of wound infections. A. baumannii is able to acquire or upregulate various resistance determinants, making it frequently multidrug-resistant, and contributing to increased mortality and morbidity. Data on the epidemiology, levels, and trends of antimicrobial resistance of Acinetobacter spp. in clinical settings is scarce in the Gulf Cooperation Council (GCC) and Middle East and North Africa (MENA) regions.
Methods: A retrospective 12-year analysis of 17,564 non-duplicate diagnostic Acinetobacter spp. isolates from the United Arab Emirates (UAE) was conducted. Data was generated at 317 surveillance sites by routine patient care during 2010–2021, collected by trained personnel and reported by participating surveillance sites to the UAE National AMR Surveillance program. Data analysis was conducted with WHONET.1
Results: Species belonging to the A. calcoaceticus-baumannii complex were mostly reported (86.7%). They were most commonly isolated from urine (32.9%), sputum (29.0%), and soft tissue (25.1%). Resistance trends to antibiotics from different classes during the surveillance period showed a decreasing trend. Specifically, there was a significant decrease in resistance to imipenem, meropenem, and amikacin. Resistance was lowest among Acinetobacter species to both colistin and tigecycline. The percentages of multidrug-resistant (MDR) and possibly extensively drug-resistant (XDR) isolates was reduced by almost half between the beginning of the study in 2010 and its culmination in 2021. Carbapenem-resistant Acinetobacter spp. (CRAB) was associated with a higher mortality (RR: 5.7), a higher admission to ICU (RR 3.3), and an increased length of stay (LOS; 13 excess inpatient days per CRAB case), as compared to Carbapenem-susceptible Acinetobacter spp.
Conclusion: Carbapenem-resistant Acinetobacter spp. are associated with poorer clinical outcomes, and higher associated costs, as compared to carbapenem-susceptible Acinetobacter spp. A decreasing trend of MDR Acinetobacter spp., as well as resistance to all antibiotic classes under surveillance was observed during 2010 to 2021. Further studies are needed to explore the reasons and underlying factors leading to this remarkable decrease of resistance over time.
1 Introduction
The extremely diversified Acinetobacter genus includes more than 50 species of nonpigmented, Gram-negative, oxidase-positive or oxidase-negative coccobacilli, the bulk of which are nonpathogenic, environmental organisms (1). The species Acinetobacter baumannii, Acinetobacter calcoaceticus, and Acinetobacter lwoffii are the most commonly detected, while Acinetobacter haemolyticus, Acinetobacter johnsonii, Acinetobacter nosocomialis, Acinetobacter pittii, and Acinetobacter schindleri are sporadically encountered (2–4). Typically, the four species A. calcoaceticus, A. baumannii, A. pittii, and A. nosocomialis form together the so called A. calcoaceticus–A. baumannii complex, being closely related and difficult to routinely distinguish; and recently, two new species, Acinetobacter seifertii and Acinetobacter dijkshoorniae were also included within that complex (5).
The 1960s and 1970s witnessed the emergence of infections caused by Acinetobacter species, coinciding with the use of sophisticated intensive care (6, 7). Initially thought of as a commensal opportunist with moderate virulence and little clinical significance, Acinetobacter infections increased in incidence and severity over the past few decades, an increase concomitant with rising prevalence of procedures including mechanical ventilation, central venous catheterization, and broad-spectrum antimicrobial therapy (8, 9). Nowadays, infections by Acinetobacter species, especially A. baumannii, are widely disseminated across hospitals, with highest density in intensive care units (ICUs), accounting for at least 20% of hospital-acquired infections in these wards (10), with estimates of resulting overall mortality exceeding 40% (11). In particular, A. baumannii group includes opportunistic pathogens that cause ventilator-associated pneumonia (VAP), central-line catheter-associated bloodstream infections (CLABSI), meningitis, urinary tract infections, surgical-site and wound infections, among others (12, 13). In one study, A. baumannii accounted for at least 9% of all Gram-negative infections and about 22% of infections in the ICU (14). Moreover, it is incriminated in over 7% of hospital-acquired pneumonia in the ICU and about 2% of nosocomial bloodstream infections (15). In a report issued in 2016 by the National Healthcare Safety Network in the US, the most frequent antimicrobial-resistant pathogens associated with health care-associated infections were reviewed. Acinetobacter species accounted for over 12% of VAP, 8.8% of CLABSI, 1.3% of catheter-associated urinary tract infections, and 1.3% of surgical site infections among gram-negative bacteria (16).
Along with the rise of Acinetobacter infections, major classes of antibiotics are threatened to lose their effectiveness against this pathogen, given its complex and varied resistance mechanisms (17). Acinetobacter exhibits exceptional capacity to retain a multidrug-resistant (MDR) phenotype, further complicating therapy, through a wide variety of pathways such as antibiotic-hydrolyzing enzymes, efflux pump alterations, impermeability, and antibiotic target mutations (18). As such, Acinetobacter spp. are capable of hydrolyzing β-lactams through the four different classes (A to D) of Ambler enzymes; produce aminoglycoside-modifying enzymes; expel multiple antibiotics by efflux pumps; alter carbapenem and aztreonam access through porin mutations; and modify key antibiotic targets like penicillin-binding proteins, DNA gyrase, and lipopolysaccharide (19–22). Accordingly, infections caused by Acinetobacter currently present a challenge to clinicians, and the available therapeutic options remain extremely limited (23). Due to accumulated mechanisms of resistance, Acinetobacter has been classified as MDR, extensively drug resistant (XDR) and pan-drug resistant (PDR), according to the published classification by Magiorakos et al. (24) for healthcare-associated, antimicrobial resistant bacteria. These phenotypes pose a real exertion to antimicrobial chemotherapy (25), and are associated with considerable mortality (26, 27).
In the Gulf Cooperation Council (GCC) and Middle East and North Africa (MENA) regions, accumulating data indicate the prevalence of MDR Acinetobacter infections. For example, Aedh and Colleagues (28) recently demonstrated alarming levels of resistance among MDR Acinetobacter in Saudi ICUs, with gentamicin and colistin being the most sensitive antibiotics. The rate of resistance to antibiotics from β-lactam, fluoroquinolone, and aminoglycoside groups was above 50%, while only trimethoprim-sulfamethoxazole was active against 50% of the isolates. Also in Qatar, different lineages of carbapenemase-producing, MDR Acinetobacter were reported (29). In Kuwait, independent research groups have previously documented expansion of MDR Acinetobacter across different hospitals, with polyclonal nature and transferrable resistance determinants (30–32). As far as the United Arab Emirates (UAE) is concerned, the first preliminary analysis of resistant A. baumannii from the Emirate of Dubai in 2021 reported multiple carbapenemase genes that have horizontally spread (33). An earlier study described MDR Acinetobacter with heterogeneous, sporadic types isolated from 5 different hospitals in Abu Dhabi (34). Moreover, in a local follow-up analysis from a single tertiary hospital at Al-Ain Emirate, a drop in imipenem susceptibility in Acinetobacter species from 99% in 2004 to only 32.5% in 2008 was noticed (35). Such evidence did shed a light on specific resistance mechanisms among local Acinetobacter species and created a background underscoring the need for further surveillance and control. However, large-scale, UAE-wide epidemiological studies of this group of bacteria are still lacking, and trends in antimicrobial resistance remain to be investigated. Of note, these trends have been increasing in reports from various regions (36–40), although some studies indicate a decreasing trend, such as that reported by Logan and colleagues (41), where cephalosporin-resistant A. baumannii decreased significantly between 2008 and 2012 in pediatric infections. The decrease was attributed to calls for improvement in infection control practices during that period, as well as to the concomitant release of an expert guidance on implementation of antimicrobial stewardship in critical care.
The UAE is a country in the GGC region well known for its cosmopolitan atmosphere, being a host for several nationalities and cultures, and a growing role as an international travel, tourism, finance, and health industry hub (42). Such blended and diversified population increases the risk for dissemination of resistant pathogens, and Acinetobacter are not an exception. Nevertheless, a consolidated, time-trend analysis of the evolution and changes in Acinetobacter resistance traits over a long period has not been previously realized in the UAE. While challenges in Acinetobacter species persist, given its nosocomial, resilient nature, longitudinal, retrospective, surveillance studies of such pathogen in a specific region remain necessary (17). Such studies highlight patterns and trends of infection and antibiotic resistance which eventually provide direction for strengthening infection control strategies in healthcare settings in this region (43), and should be beneficial to follow up Acinetobacter resistance patterns in the UAE.
The current investigation was realized to describe the longitudinal changes in Acinetobacter species resistance trends, as reported by the national antimicrobial resistance surveillance system that covers all the seven UAE Emirates. The specific objective of this follow-up study was to explore the nationwide status of Acinetobacter species resistance and evolving nosocomial patterns. It lays out the most prevalent Acinetobacter spp. observed at UAE healthcare facilities, along with the prevalence of their MDR, XDR, and PDR phenotypes, and represents the first documentation of a 12-year resistance portfolio in this pathogen across the whole country, from 2010 until 2021.
2 Materials and methods
2.1 Study design and data source
A multi-institutional retrospective observational study was conducted between 2010 and 2021 in the UAE using data extracted from the WHONET microbiology laboratory database software2 supported by the Global AMR Surveillance System protocol (GLASS, World Health Organization). Data was generated, collected, cleaned and analyzed through the UAE national AMR Surveillance program described by Thomsen et al. (44).
2.2 Identification and enrollment of national AMR surveillance sites
Starting 2010, UAE institutions were incorporated into the UAE national AMR surveillance program based on epidemiological needs assessment, readiness, and willingness of facilities to participate, availability of high-quality electronic AMR data, lab accreditation status, and qualification of staff. Hospitals, centers, and clinics representing all seven Emirates of the UAE joined the AMR surveillance network gradually over the years.
2.3 Bacterial population and variables of the study
All Acinetobacter spp. isolated from clinical samples during routine patient care by medical professionals in the National AMR surveillance sites, were included in this study from January 2010 to December 2021. Only the first isolate from each patient for each species per reporting period was included. Excluded from analysis were screening and quality control isolates, duplicate isolates, infection control related isolates, environmental isolates, and isolates from primary contaminated sources (pedibag).
The associated patient demographic information, clinical data, and microbiologic laboratory results were extracted from the national WHONET laboratory database software. The demographic variables included age, sex, nationality; clinical variables revealed the type of facility reporting the isolate (hospital/center/clinic), patient location, location type, specimen collection date, types of infection/specimen source; and microbiology variables revealed types of organism and antibiotic susceptibility testing results. The infection was considered to originate outside the center for outpatients or those presenting with the infection at the emergency department.
2.4 Bacterial identification
Bacterial identification was performed at the national AMR surveillance sites by medical professionals. The participating centers used at least one commercial, automated system for identification of bacteria, including VITEK® (BioMérieux SA, Craponne, France), BD Phoenix™ (Becton Dickinson, New Jersey, United States) and MicroScan WalkAway (Beckman Coulter, Brea, CA, United States). Only one lab relied on manual systems like API® (Analytical Profile Index. BioMérieux SA, Craponne, France) solely for identification.
2.5 Antimicrobial susceptibility testing
Antimicrobial susceptibility testing was performed at the National AMR surveillance sites using at least one commercial, automated system for routine antimicrobial susceptibility testing. Only two laboratories used manual testing methods (disk diffusion/Kirby Bauer). All labs followed CLSI guidelines for antimicrobial susceptibility testing of bacteria (CLSI-M100) (45). The criteria for interpretation of susceptibility testing results for tigecycline were adapted from the European Committee on Antimicrobial Susceptibility Testing (EUCAST 2022) guidelines (46). Any Acinetobacter spp. resistant to either imipenem, or meropenem, or both was considered as carbapenem-resistant Acinetobacter spp. (CRAB). To assess the multidrug-resistant (MDR) phenotype of the isolates the standard definition by Magiorakos et al. was used (24). To assess the extensively drug-resistant (XDR) and pandrug-resistant (PDR) phenotypes, a slightly modified version of the standard definition by Magiorakos et al. was used (24). Magiorakos’ et al. definitions for XDR and PDR phenotypes for Acinetobacter spp. includes 9 antimicrobial categories with 22 antibiotic agents. For technical reasons, associated costs, and local formulary requirements, participating laboratories would not routinely test all 22 antibiotics, i.e., some antibiotics were only very rarely (netilmicin, levofloxacin, ticarcillin/clavulanic acid, ampicillin/sulbactam, colistin, polymyxin B, tetracycline, doxycycline) or not at all (doripenem) tested. As such, the following, slightly modified definitions were used for ‘possible XDR’ and ‘possible PDR’ isolates (modifications highlighted in italics):
- ‘Possible XDR’: Non-susceptibility to at least one agent routinely tested by clinical labs in all but two or fewer antimicrobial categories, (i.e., bacterial isolates remain susceptible to only one or two categories).
- ‘Possible PDR’: Non-susceptibility to all agents routinely tested by clinical labs in all antimicrobial categories (i.e., no agents were tested as susceptible for that organism).
2.6 Statistical tests
Statistical significance of temporal trends for antimicrobial resistance percentages was calculated if data from at least 5 years was available. If fewer than 30 isolates per year were reported, or data was not available for all years within the considered period, trend analysis was not conducted. Statistical significance of trends is expressed as a p-value, calculated by a Chi-square for trend test (extended Mantel–Haenszel), using SPSS or Epi Info™. For testing the statistical significance of the difference for mortality and ICU admission a Chi2-test was used. For testing the statistical significance of the difference for length of stay (LOS), the weighted log-rank survival analysis was used. This was done to take care of differences in sample size between the groups. A p-value of less than 0.05 was considered statistically significant.
3 Results
3.1 Distribution of reporting sites for national AMR surveillance
The UAE national AMR surveillance program was initiated in 2010 in the Abu Dhabi Emirate with 6 hospitals and 16 centers/clinics enrolled as AMR surveillance sites. Additional sites were recruited over the years, starting with 22 participating sites located only in the Emirate of Abu Dhabi in 2010, which is the first year during which the study was initiated, and reaching in 2021 a total of 317 surveillance sites, including 87 hospitals and 230 centers/clinics and representing all seven Emirates of the country. Figure 1 represents the distribution of reporting sites for National AMR Surveillance from 2010 to 2021, by year and Emirate.
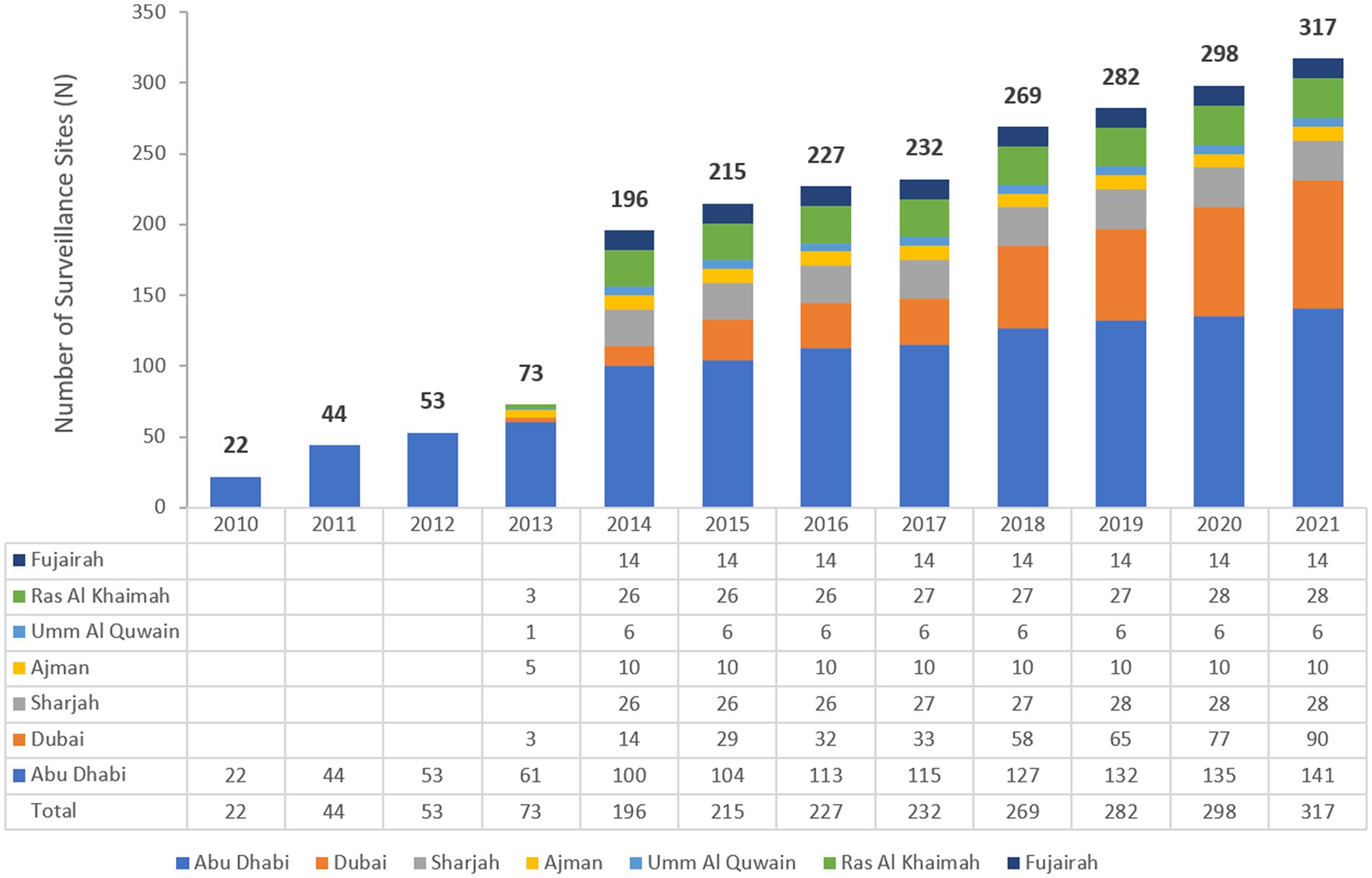
Figure 1. Number of AMR Surveillance sites by Emirate over the years of the surveillance period (2010–2021) in the UAE.
3.2 Bacterial population
From 2010 to 2021, a total of 17,564 non-repetitive Acinetobacter spp. was isolated from an equivalent number of patients over the surveillance period. Figure 2 represents the number of Acinetobacter spp. isolates included per year.
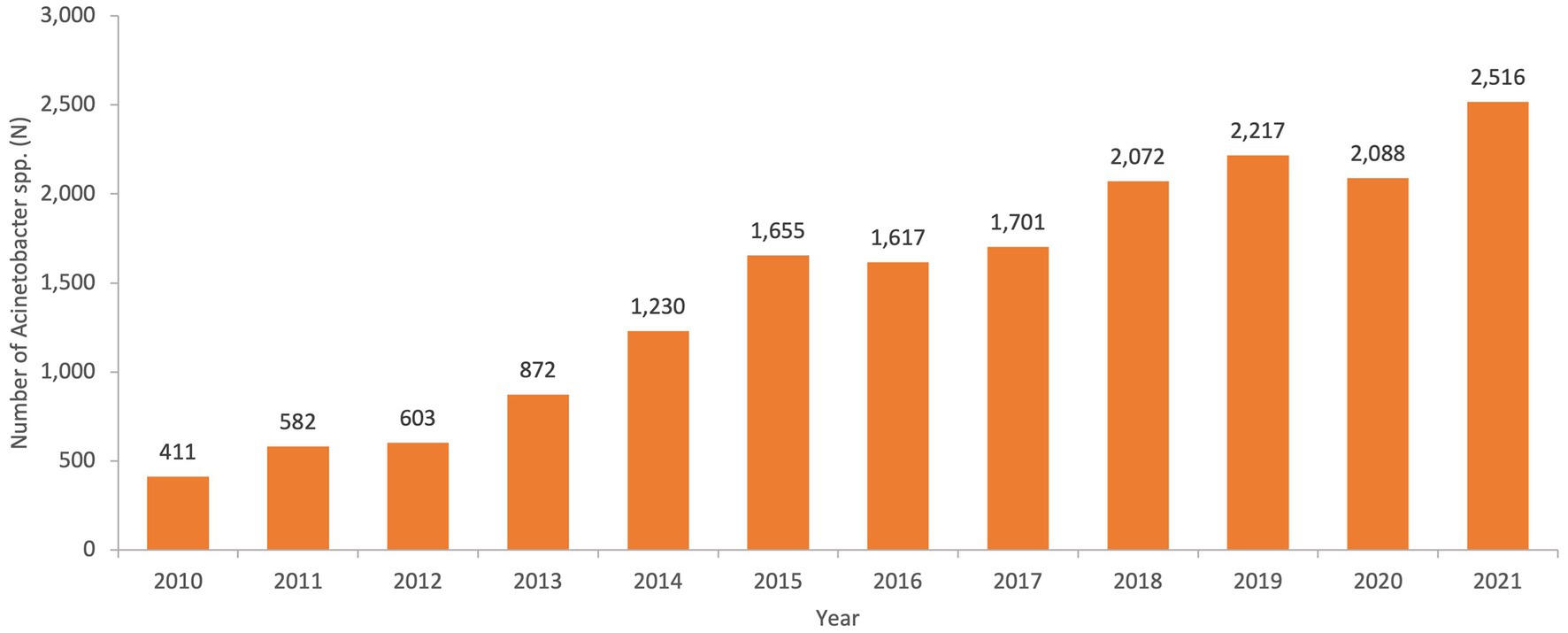
Figure 2. Numbers of non-repetitive Acinetobacter spp. isolated per year over the surveillance period in the UAE.
3.3 Species distribution
Among the 17,564 Acinetobacter spp. analyzed, the vast majority belonged to the A. calcoaceticus–A. baumannii complex that includes A. calcoaceticus, A. baumannii, A. pittii, and A. nosocomialis. The overall percentages over the study period are shown in Table 1. More than 86.7% of the total number of Acinetobacter spp. collected during the surveillance period belongs to that complex.
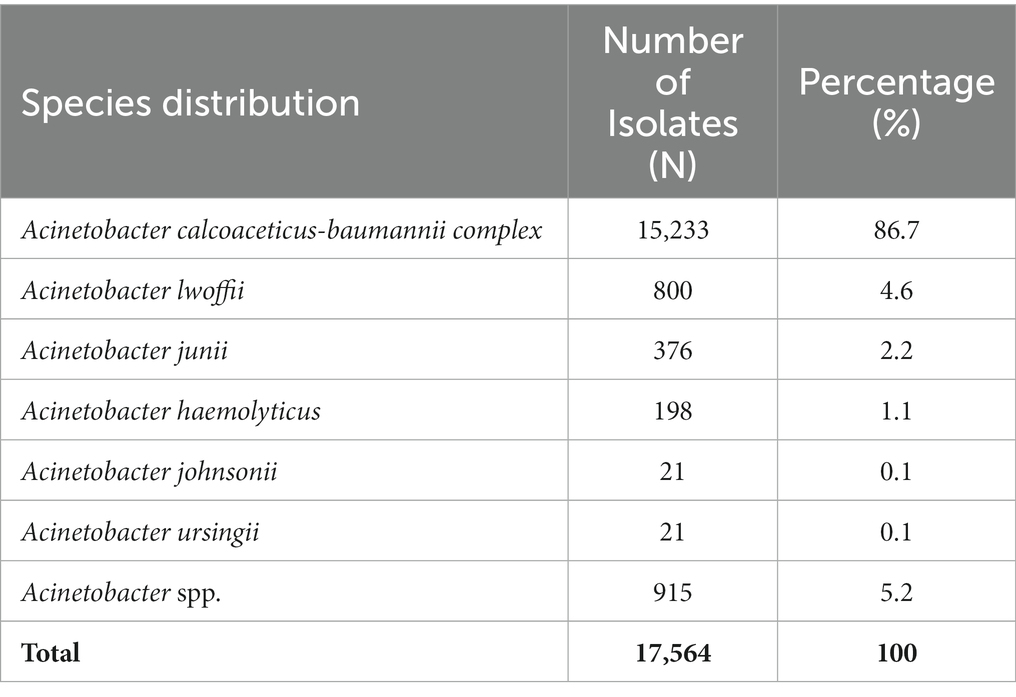
Table 1. Acinetobacter species distribution as number and percentage of isolates across the study period (2010–2021).
3.4 Distribution of Acinetobacter spp. patients by age, gender, nationality status, and Emirate
Acinetobacter spp. strains were mostly associated with adults with a net decrease in the newborn and pediatric population since 2016 (Figure 3). Strains of Acinetobacter spp. were almost equally affecting males and females, with a 51% attributed to males.
The nationality status of patients revealed a total of 23.1% of nationals and 36.3% of expatriates. For the remaining 40.6% of patients, the nationality status was missing. The majority of patients was detected in the Emirate of Abu Dhabi as shown in Figure 4, and those accounted for over half of the patients during which Acinetobacter isolates were recovered.
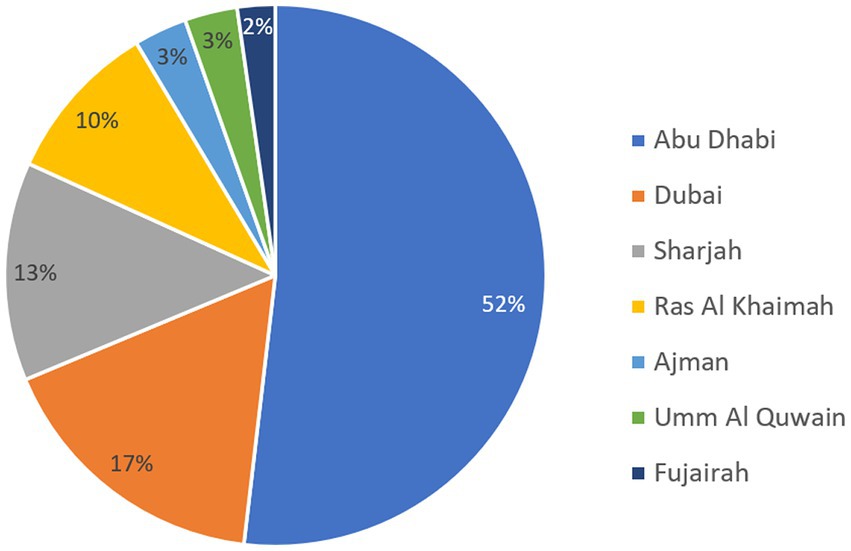
Figure 4. Distribution of patients carrying Acinetobacter spp. by Emirate over the surveillance period.
3.5 Distribution of Acinetobacter spp. by sample type group
Most of Acinetobacter spp. strains were isolated from urine (32.9%), followed by the respiratory tract samples (29.0%) and the soft tissue (25.1%) groups. The distribution of Acinetobacter isolates by clinical sample type is shown in Figure 5.
3.6 Distribution of Acinetobacter spp. by location (inpatients/outpatients) and department
Most strains of Acinetobacter spp. (65.2%) were detected in clinical settings (in hospitals rather than community settings and emergency wards) and were enrolled in general medical wards (26.8%) followed by ICUs (15.8%) and surgery departments (15.6%). A proportion of 34.8% of studied isolates originated in outpatient basis, being recovered either in the community, from outpatient centers and clinics, or in the hospital emergency departments.
3.7 Trend of antimicrobial susceptibility profiles of Acinetobacter spp.
The sensitivity of all Acinetobacter spp. recovered during a period of the study, from 2014 to 2021, to antimicrobial agents from the β-lactam group and other groups is shown in Figures 6 and 7.
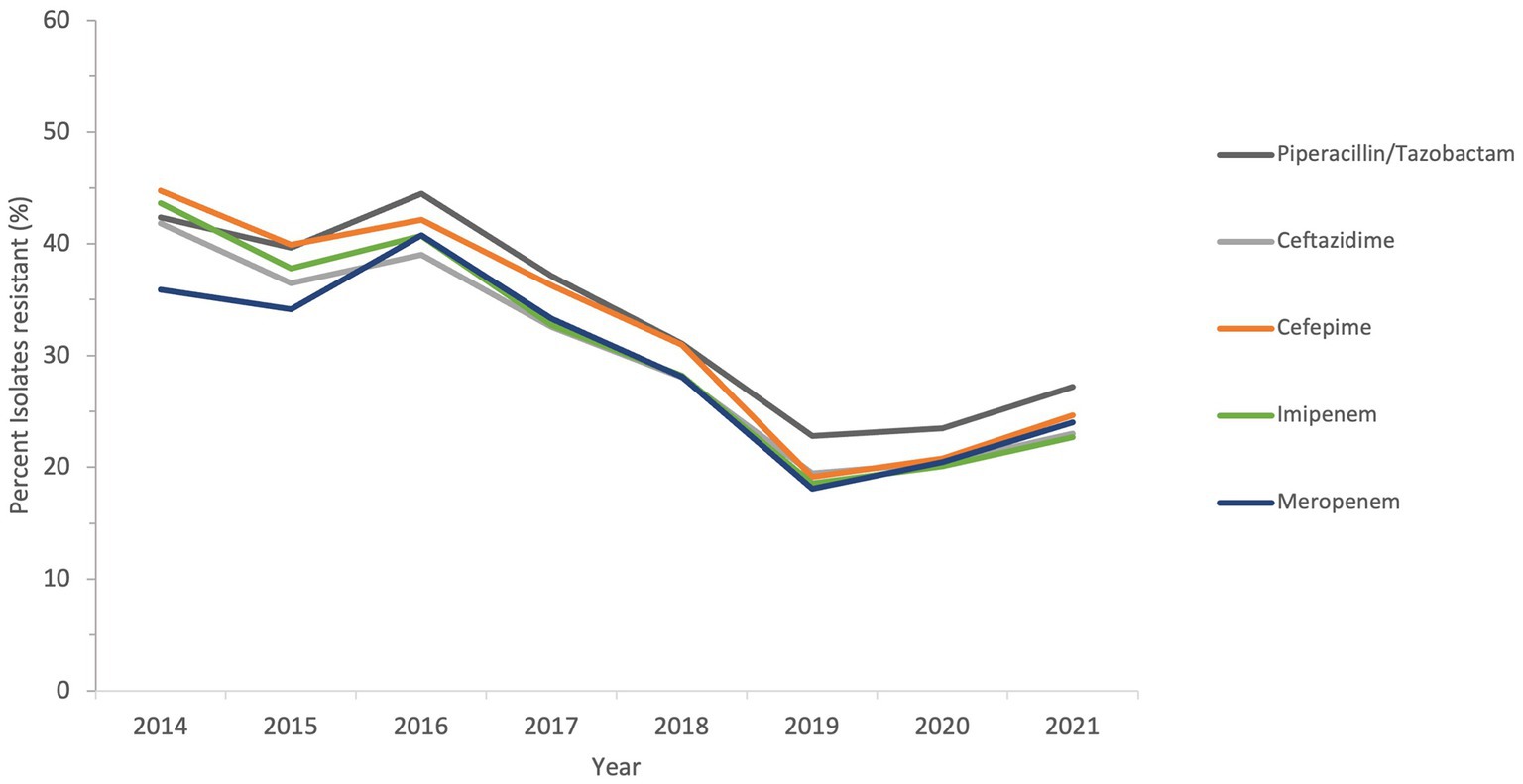
Figure 6. Susceptibility trends of Acinetobacter spp. to five different β-lactams over a selected period of the study (from 2014 to 2021).
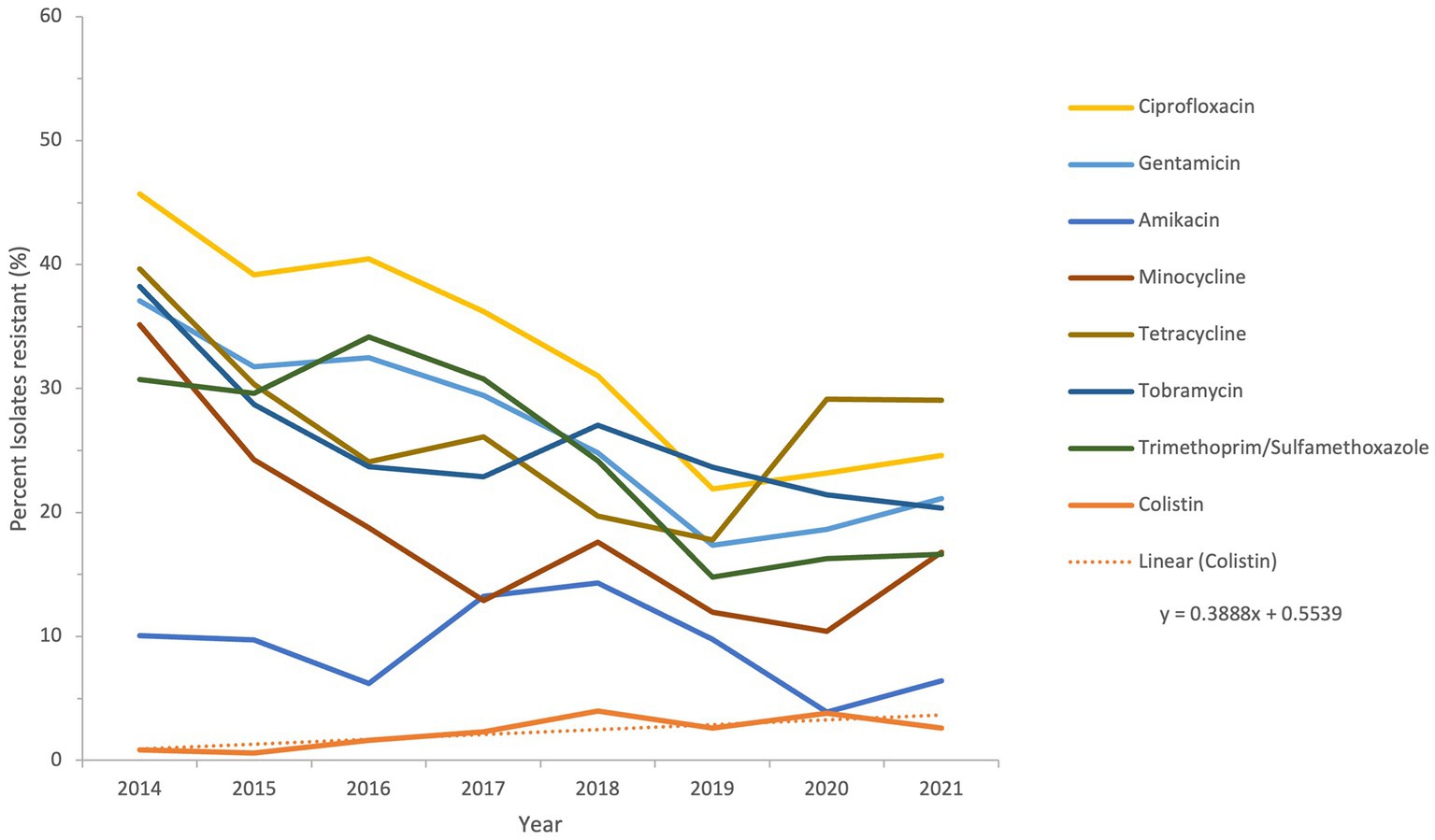
Figure 7. Susceptibility trends of Acinetobacter spp. to antibiotics other than β-lactams over a selected period of the study (from 2014 to 2021).
The resistance of isolates to multiple antibiotics showed a decreasing trend over the study period, as depicted from the general profiles in Figures 6 and 7. Specifically, resistance to imipenem and meropenem as well as to amikacin showed a statistically significant decrease over the past 12 years with a p value of zero. Resistance to colistin was low, showing an upper limit of 4% in 2018. Tigecycline resistance levels were the lowest, with maximum upper limit of 0.2% in 2019 and 2021, while they persisted at zero with isolates remaining highly sensitive to this antibiotic for all other study years.
The percentage of strains that exhibited MDR phenotype, as shown in Figure 8, being resistant to three or more classes of antibiotics, such as ciprofloxacin, gentamicin, tetracycline, trimethoprim/sulfamethoxazole, ceftazidime, piperacillin/tazobactam, imipenem, and meropenem, ranged between 48.7 in 2010 and 20.6 in 2019 and 2020, then raised again to 24.7 in 2021. The maximum percentage of possible XDR strains was reported in 2010 at 45.7%, and of possible PDR strains in 2016 at 16.2%. These figures were cut down to 22.3 and 8%, respectively, in 2021. As an overall trend, MDR, possible XDR, and possible PDR strains generally declined over the study period especially starting from the year 2016, as shown in Figure 8.
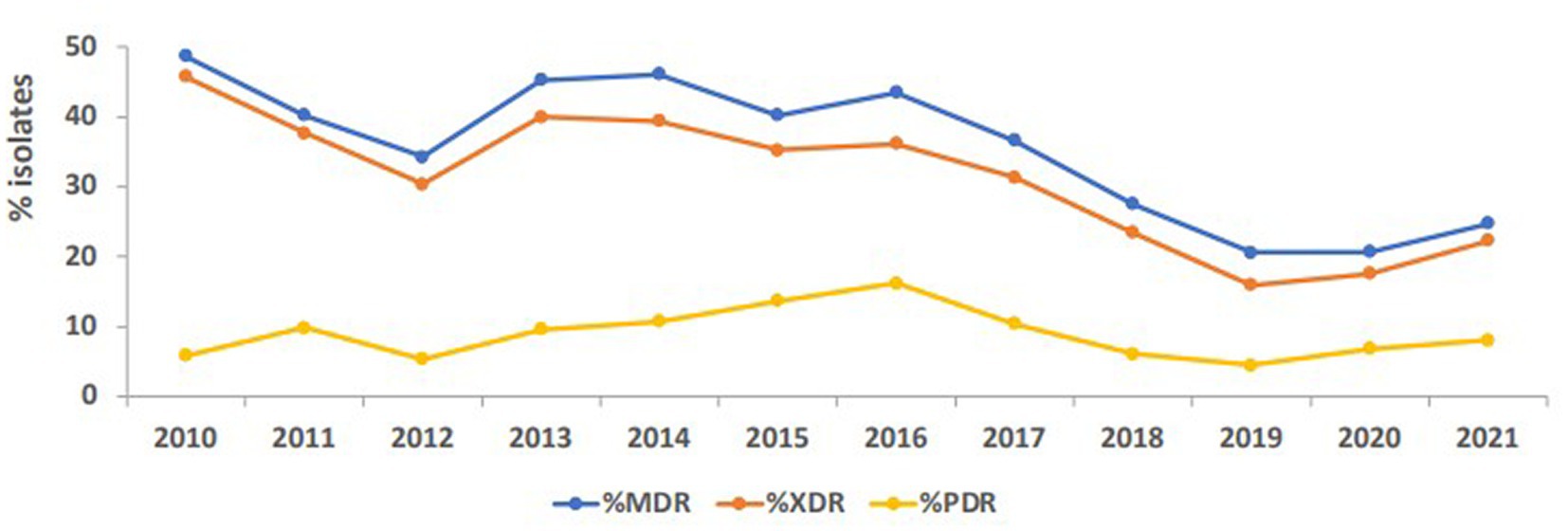
Figure 8. Trends of Acinetobacter spp. exhibiting multidrug-resistant (MDR), possible extensively drug-resistant (XDR) and possible pandrug-resistant (PDR) phenotypes over the study period.
3.8 Mortality rate
A subgroup analysis including the nine clinical institutions that reported mortality was performed. In these institutions, a total of 4,306 patients were associated with non-CRAB of whom 272 patients died (mortality rate is 6.32%), while a total of 1,649 patients were associated with CRAB, of whom 593 patients died (mortality rate is 35.96%). The difference in mortality between CRAB patients (35.96%) and non-CRAB patients (6.32%) is statistically significant (RR 5.69, 95% C.I. 4.99, 6.50, p < 0.01).
3.9 Admission to intensive care unit
A total of 9,132 patients were associated with non-CRAB of whom 1,109 patients were admitted to ICU (ICU admission rate is 12.14%), while a total of 3,800 patients were associated with CRAB, of whom 1,510 patients were admitted to ICU (ICU admission rate: 39.740%). The difference in ICU admission rate is statistically significant (RR 3.27, 95% C.I. 3.06, 3.50, p < 0.01).
3.10 Length of stay (LOS)
A subgroup analysis including those patients for whom the date of admission as well as the date of discharge was known was performed. For patients in the non-CRAB group (n = 1,321) the median length of stay was 8 days, while for patients in the CRAB group (n = 715) the median length of stay was 21 days. Comparative differences in LOS were done using Kaplan–Meier curves and are shown graphically in Supplementary Figure 1. To assess if the observed difference in LOS was statistically significant, we performed a weighted log-rank test. This test showed that there was a significant difference in LOS between CRAB and non-CRAB patients (p < 0.001).
Based on a total of n = 4,921 patients associated with CRAB during the observation period (2010–2021), a total of 63,973 excess days of hospitalization is estimated attributable to CRAB. For the year 2021 only, a total of 7,384 excess hospitalization days is estimated attributable to CRAB.
4 Discussion
This is the first comprehensive analysis across the UAE that shows the significance and magnitude of Acinetobacter infections in clinical settings and the fluctuations in their antimicrobial resistance patterns. The present research utilized an extensive dataset collected over a considerable duration, allowing precise observation of subtle variations in antimicrobial resistance among Acinetobacter. This level of inclusive analysis has not been previously replicated in the country. The samples analyzed in this study consisted of non-repetitive Acinetobacter of laboratory confirmed identity and antibiotic resistance profile, indicating authenticity of the microbiological material used and accuracy of the generated data. Perhaps the most thought-provoking finding in this study is the observation of a decrease in antibiotic resistance in Acinetobacter over about 12 years, and this was evident despite an increase in the number of participating sites from 22 to 317, distributed across all the seven Emirates.
The UAE accommodates a diverse community comprising more than 200 nationalities. Emirati nationals make up approximately 10% of the overall population, highlighting the UAE’s status as one of the countries with a significant expatriate presence. Among the expatriate groups, Indians and Pakistanis represent the largest segments, accounting for 27.5 and 12.7% of the total population, respectively. However, our results show that about 23% of Acinetobacter samples were recovered from Emirati nationals, while 6.1 and 4.5% were recovered from Indian and Pakistani expatriates, respectively. These proportions of the total sample pool should be interpreted cautiously, since 40.6% of the samples attributed from patients for whom their nationality was not coded in the data, hence not available. With the expatriate-inclusive and multicultural setting, expected to prevail for the forthcoming years, the UAE may be an interesting niche to compare how trends of resistance in Acinetobacter differ by nationality, shedding a light on cultural and social factors contributing to resistance in a multidisciplinary research perspective, as previously suggested (47, 48). However, given that a massive 40.6% of our samples originated from patients with unknown nationality, this investigation could not be realized with our data, but remains tempting to explore. Moreover, the majority of patients (52%) from whom samples for the study were recovered were residents of the Emirate of Abu Dhabi, which also included the majority of participating centers (44.2%). Obviously, this conforms with the fact that Abu Dhabi has been the first Emirate to start AMR surveillance, and it also is the largest Emirate in terms of area, where is occupies over 80% of the nation’s land. However, Dubai, rather than Abu Dhabi, is the most populated Emirate, and samples from Dubai residents accounted for a much lower 17% only of those analyzed in this study. As such, these results must be cautiously interpreted.
As shown in Table 1, the majority of recovered species belong to A. calcoaceticus-baumannii complex, which alone occupied 86.7% of the total sample size. The remaining proportion was formed collectively from all other species, with A. lwoffii and A. junii accounting for 4.6 and 2.2% of all isolates collected throughout the study period, in addition to other species. Ubiquitous Acinetobacter species were initially thought of as commensals that are non-pathogenic to immunocompetent subjects (49); globally, reports on hospital outbreaks caused by Acinetobacter spp. have been mostly linked to A. baumanni, which is the most virulent species (50). However, several species of these ubiquitous bacteria have emerged as core pathogens in hospitalized patients and fomites, and can cause life-threatening nosocomial infections in compromised hosts (51). The spread of antimicrobial resistance to these species has further increased concerns regarding them and placed them as a special risk (52), especially in the ICU (53–55). With over 15% of different non-baumannii species isolated throughout the duration of the study, this may shed a light on the presence and long-term maintenance of these strains among patients and, accordingly, the need for precise documentation and tight control of different factors responsible for their dissemination in the UAE.
A consistent minimum of about 70% of species were recovered from adult patients, while pediatric samples declined from 2016 till the end of the study to reach about 12%. Reports of resistant Acinetobacter infections in children in different hospital settings have been previously published (56–58), even in children under 1 year with community acquisition of A. baumanni in their upper respiratory tract (59). This indicates possible epidemiological insights into the existence of Acinetobacter not only in critical settings, but also in non-serious community-based infections in the pediatric population. An in-depth analysis of Acinetobacter infections in infants and children in the UAE has not been yet realized. However, the decreasing rates of infection since 2016 are interesting, and warrant a further focused exploration of the epidemiology and resistance patterns of Acinetobacter in this population.
Urinary and respiratory samples contributed to the largest collection of studied samples, with percentages of 33 and 29%, respectively, from the bulk collection of Acinetobacter. Although described as a cause of catheter-associated urinary tract infections (60), most of the literature has focused on Acinetobacter in pneumonia and bloodstream infections; nevertheless, its role as a uropathogen cannot be neglected (61), and its isolation in urine samples in this study warrants attention. The second most common clinical specimen was from the respiratory tract, and this is in parallel to evidence indicating Acinetobacter abundance in respiratory samples with an incidence from 13 to 68% (62). Of note, the proportion of outpatient samples was about 35%, shedding a light on Acinetobacter reservoirs outside hospital settings, where they can be culprits in community-acquired pneumonia, infections in survivors from natural disasters, and infected war wounds (63). The actual presence of this organism in various environmental locations and being transferred to patients in the community is not possible to confer from our findings and needs further attention.
Over the full duration of the study, the rates of resistance of Acinetobacter to all tested antibiotics did not increase beyond 50%. This is in contrast to a recent report from the Gulf region, where in KSA, a neighboring country, the rates of resistance to all antibiotic in A. baumannii was above 50%, except for gentamycin and colistin (28). Also, in Oman, the rates of A. baumannii resistance to different antibiotics ranged from 50 to 83% (64). Previously, some studies detected the molecular epidemiology of A. baumannii resistance in the region, like detection of OXA-23 and OXA-24 from isolates collected from the Gulf Cooperation Council countries (65), detection of OXA-23, NDM-1 and GES-11 in isolates from Dubai (33), and detection of MDR and XDR strains in Abu Dhabi (34). In this study, a follow-up of resistance trends to different antibiotics consistently at a nationwide level showed that the rates of resistance declined from 2011 to 2021. This was true for all tested β-lactam antibiotics, whose resistance declined from a range of 40–50% at the beginning of the study to 20–30% in the last 3 years of follow-up. For other antibiotic classes, the trends of resistance were more heterogeneous in the first few years (due to smaller sample size), but all declined toward the end of the study. Perhaps the statistically significant decline in imipenem, meropenem, and amikacin is one of the most interesting findings of this analysis, and warrants to investigate the positive practices in the UAE that culminated into such a result. Similarly, the proportions of MDR and possible XDR strains were almost reduced by half, indicating that some isolates have regained their sensitivity or at least ceased being nonsusceptible over the course of the follow-up years. The mild increase in the levels of MDR, possible XDR, and possible PDR strains toward the end of the study period may have been affected by the COVID-19, as reported elsewhere (66, 67), and was probably driven by high rates of antimicrobial utilization and disruption of infection control measures occurring as collateral effects of the global pandemic (68). It is worth mentioning that trends in Acinetobacter species resistance were reported to have similar declining portraits in other parts of the world. For instance, a national study from the US showed a decline in carbapenem resistance (from 43 to 36%), MDR prevalence (from 49 to 36%), and XDR prevalence (from 21 to 10%) from 2010 to 2018. Similar epidemiological data of resistance decline in Acinetobacter exist from KSA (69), Germany (70), and Brazil (71). Hence, the decrease in resistance trends in Acinetobacter in the UAE mirrors other resistance trend observations in the region and elsewhere, and emphasize the need for continuing infection control and stewardship efforts and the development of new therapeutic options. While the trends for antimicrobial resistance to some antibiotics showed a slight rise after 2019, probably associated with factors related to the COVID-19 pandemic (72), the tends remained lower than those observed at the beginning of the study.
Looking into some specific antibiotics and the decrease in resistance among the studied isolates, it is noticeable that, for instance, imipenem and meropenem resistance rates in 2021 were less than 30%. This is much lower than rates of resistance reported in nearby countries like Jordan, where carbapenem resistance rate in 2022 was 99% (73). Moghnieh and Colleagues (74), in their narrative review on resistant Gram-negative pathogens in the region also described rates of carbapenem resistance in Acinetobacter above 80% not only in Jordan, but also in Lebanon and Iraq. The rates of carbapenem resistance in other countries including Turkey, Greece, Italy, and Spain, are much higher, with reported incidences of 50–80, 85, 60, and 45%, respectively (75, 76). For antibiotics from the non-β-lactam class, the highest resistance rates in 2021 were for tetracycline and ciprofloxacin, but both showed rates below 30%. This is in contrast to higher rates observed in Iran (tetracycline resistance 86%) (77), Egypt (ciprofloxacin resistance 42%) (78). However, the rates in this study were higher than those observed in Pakistan for ciprofloxacin (2.5%) and close to those for tetracycline (25%) (79). There are positive insights from the decline in resistance observed over the study duration, and comparison to other data from other countries reveals diverse resistance rates. However, the observed decline does not preclude the need for ongoing surveillance of Acinetobacter infections and continued assessment of effective prevention strategies, to build on the observed resistance mitigation for future attainments.
The two antibiotics tigecycline and colistin remained effective throughout the study period. According to previous evidence (80–84), combinations of these two antibiotics or combination of at least one of them with a third antibiotic have been used in treatment of MDR Acinetobacter infections, with variable success. However, both antibiotics remain among the most effective antimicrobial agents against Acinetobacter isolates in vitro (85), and their value needs to be preserved. As such, antimicrobial usage and consumption surveillance should aim at monitoring the use of colistin and tigecycyline, in presence of reports indicating resistance in Acinetobacter mainly mediated by the tet(X) gene against tigecycline (86, 87), and by the loss or modification of lipopolysaccharide or plasmid-encoded mcr genes against colistin (88, 89). The preservation of effectiveness of these two antibiotics in the UAE during this study, albeit with some rise in colistin resistance in 2018 and 2020, should provide an exemplar on maintaining the effect of last-resort antibiotics in clinical settings of high resistance.
Nevertheless, the mortality rate, according to our observations, was about 5.7-fold higher in patients infected with CRAB compared to those infected with non-CRAB Acinetobacter spp. Patients with an infection associated with CRAB were 3.3-fold more likely to be admitted to ICU, and their median length of stay was increased by 13 days, as compared to patients with non-CRAB infections. This is consistent with other findings that indicated high mortality rate and poor outcomes in patients with CRAB (90, 91) and highlights need for surveillance and control for better health outcomes.
5 Conclusion
This 12-year follow-up of the resistance trends in Acinetobacter species in the UAE indicated a decline in antimicrobial resistance and in proportions of Acinetobacter isolates with MDR and XDR profiles. The useful surveillance techniques, infection control strategies, and stewardship implemented over this span of time should be all reinforced. Further to these findings, continued epidemiological enquiry and genetic evolution analysis of Acinetobacter are required, to sustain the observed decline in resistance and to provide new strategies for prevention and control.
Data availability statement
The datasets presented in this article are not readily available because the National AMR Surveillance database managed by the UAE Ministry of Health and Prevention (MOHAP) contains confidential health information. Requests to access the datasets should be directed to https://mohap.gov.ae/.
Ethics statement
Ethical approval for this study was provided by the Ministry of Health and Prevention Research Ethics Committee (MOHAP/DXB-REC/ D.D.D/No.131 / 2021; MOHAP/DXB-REC/J.J.J./No. 86/2023), Dubai Scientific Research Ethics Committee (DSREC-GL17-2023), and Abu Dhabi Health Research and Technology Ethics Committee (DOH/ZHCD/2023/1316).
Author contributions
CM, JT, NA, HA, DE, AS, and GM: conceptualization. JT, CM, NA, HA, DE, AS, GM, and The UAE AMR surveillance consortium: data collection. JT and CM: formal analysis. JT, CM, NA, HA, DE, AS, GM: data interpretation. CM and JT: manuscript preparation. CM, JT, NA, HA, DE, AS, and GM: manuscript review and editing. All authors contributed to the article and approved the submitted version.
The UAE AMR surveillance consortium
Funding
The Article Processing Charge (APC) was funded by the UAE Ministry of Health and Prevention (MOHAP).
Acknowledgments
The authors wish to thank the UAE Ministry of Health and Prevention, regional health authorities (DHA, DoH/ADPHC), AMR Focal points at participating sites, and all healthcare professionals and other experts involved in national AMR surveillance in the UAE for their continuous collaboration and support.
Conflict of interest
The authors declare that the research was conducted in the absence of any commercial or financial relationships that could be construed as a potential conflict of interest.
Publisher’s note
All claims expressed in this article are solely those of the authors and do not necessarily represent those of their affiliated organizations, or those of the publisher, the editors and the reviewers. Any product that may be evaluated in this article, or claim that may be made by its manufacturer, is not guaranteed or endorsed by the publisher.
Supplementary material
The Supplementary material for this article can be found online at: https://www.frontiersin.org/articles/10.3389/fpubh.2023.1245131/full#supplementary-material
Footnotes
References
1. Al Atrouni, A, Joly-Guillou, M-L, Hamze, M, and Kempf, M. Reservoirs of non-baumannii Acinetobacter species. Front Microbiol. (2016) 7:49. doi: 10.3389/fmicb.2016.00049
2. Chang, WN, Lu, CH, Huang, CR, and Chuang, YC. Community-acquired Acinetobacter meningitis in adults. Infection. (2000) 28:395–7. doi: 10.1007/s150100070013
3. Visca, P, Seifert, H, and Towner, KJ. Acinetobacter infection--an emerging threat to human health. IUBMB Life. (2011) 63:1048–54. doi: 10.1002/iub.534
4. Wong, D, Nielsen, TB, Bonomo, RA, Pantapalangkoor, P, Luna, B, and Spellberg, B. Clinical and pathophysiological overview of Acinetobacter infections: a century of challenges. Clin Microbiol Rev. (2017) 30:409–47. doi: 10.1128/CMR.00058-16
5. Toh, BEW, Paterson, DL, Kamolvit, W, Zowawi, H, Kvaskoff, D, Sidjabat, H, et al. Species identification within Acinetobacter calcoaceticus-baumannii complex using MALDI-TOF MS. J Microbiol Methods. (2015) 118:128–32. doi: 10.1016/j.mimet.2015.09.006
6. Stirland, RM, Hillier, VF, and Steyger, MG. Analysis of hospital bacteriological data. J Clin Pathol Suppl Coll Pathol. (1969) 3:82–6. doi: 10.1136/jcp.s2-3.1.82
7. Abrutyn, E, Goodhart, GL, Roos, K, Anderson, R, and Buxton, A. Acinetobacter calcoaceticus outbreak associated with peritoneal dialysis. Am J Epidemiol. (1978) 107:328–35. doi: 10.1093/oxfordjournals.aje.a112548
8. Spellberg, B, and Bonomo, RA. The deadly impact of extreme drug resistance in Acinetobacter baumannii. Crit Care Med. (2014) 42:1289–91. doi: 10.1097/CCM.0000000000000181
9. Maragakis, LL, and Perl, TM. Acinetobacter baumannii: epidemiology, antimicrobial resistance, and treatment options. Clin Infect Dis. (2008) 46:1254–63. doi: 10.1086/529198
10. Ayobami, O, Willrich, N, Harder, T, Okeke, IN, Eckmanns, T, and Markwart, R. The incidence and prevalence of hospital-acquired (carbapenem-resistant) Acinetobacter baumannii in Europe, eastern Mediterranean and Africa: a systematic review and meta-analysis. Emerg Microbes Infect. (2019) 8:1747–59. doi: 10.1080/22221751.2019.1698273
11. Mohd Sazlly Lim, S, Zainal Abidin, A, Liew, SM, Roberts, JA, and Sime, FB. The global prevalence of multidrug-resistance among Acinetobacter baumannii causing hospital-acquired and ventilator-associated pneumonia and its associated mortality: a systematic review and meta-analysis. J Infect. (2019) 79:593–600. doi: 10.1016/j.jinf.2019.09.012
12. Sieniawski, K, Kaczka, K, Rucińska, M, Gagis, L, and Pomorski, L. Acinetobacter baumannii nosocomial infections. Pol Przegl Chir. (2013) 85:483–90. doi: 10.2478/pjs-2013-0075
13. Howard, A, O’Donoghue, M, Feeney, A, and Sleator, RD. Acinetobacter baumannii. Virulence. (2012) 3:243–50. doi: 10.4161/viru.19700
14. Uwingabiye, J, Frikh, M, Lemnouer, A, Bssaibis, F, Belefquih, B, Maleb, A, et al. Acinetobacter infections prevalence and frequency of the antibiotics resistance: comparative study of intensive care units versus other hospital units. Pan Afr Med J. (2016) 23:191. doi: 10.11604/pamj.2016.23.191.7915
15. Kim, UJ, Kim, HK, An, JH, Cho, SK, Park, K-H, and Jang, H-C. Update on the epidemiology, treatment, and outcomes of Carbapenem-resistant Acinetobacter infections. Chonnam Med J. (2014) 50:37–44. doi: 10.4068/cmj.2014.50.2.37
16. Weiner, LM, Webb, AK, Limbago, B, Dudeck, MA, Patel, J, Kallen, AJ, et al. Antimicrobial-resistant pathogens associated with healthcare-associated infections: summary of data reported to the National Healthcare Safety Network at the Centers for Disease Control and Prevention, 2011-2014. Infect Control Hosp Epidemiol. (2016) 37:1288–301. doi: 10.1017/ice.2016.174
17. Gonzalez-Villoria, AM, and Valverde-Garduno, V. Antibiotic-resistant Acinetobacter baumannii increasing success remains a challenge as a nosocomial pathogen. J Pathog. (2016) 2016:7318075. doi: 10.1155/2016/7318075
18. Ayoub Moubareck, C, and Hammoudi, HD. Insights into Acinetobacter baumannii: a review of microbiological, virulence, and resistance traits in a threatening nosocomial pathogen. Antibiotics (Basel). (2020) 9. doi: 10.3390/antibiotics9030119
19. Lee, C-R, Lee, JH, Park, M, Park, KS, Bae, IK, Kim, YB, et al. Biology of Acinetobacter baumannii: pathogenesis, antibiotic resistance mechanisms, and prospective treatment options. Front Cell Infect Microbiol. (2017) 7:55. doi: 10.3389/fcimb.2017.00055
20. Nowak, P, and Paluchowska, P. Acinetobacter baumannii: biology and drug resistance - role of carbapenemases. Folia Histochem Cytobiol. (2016) 54:61–74. doi: 10.5603/FHC.a2016.0009
21. Chakravarty, B. Genetic mechanisms of antibiotic resistance and virulence in Acinetobacter baumannii: background, challenges and future prospects. Mol Biol Rep. (2020) 47:4037–46. doi: 10.1007/s11033-020-05389-4
22. Carretero-Ledesma, M, García-Quintanilla, M, Martín-Peña, R, Pulido, MR, Pachón, J, and McConnell, MJ. Phenotypic changes associated with Colistin resistance due to lipopolysaccharide loss in Acinetobacter baumannii. Virulence. (2018) 9:930–42. doi: 10.1080/21505594.2018.1460187
23. Karakonstantis, S, Gikas, A, Astrinaki, E, and Kritsotakis, EI. Excess mortality due to pandrug-resistant Acinetobacter baumannii infections in hospitalized patients. J Hosp Infect. (2020) 106:447–53. doi: 10.1016/j.jhin.2020.09.009
24. Magiorakos, A-P, Srinivasan, A, Carey, RB, Carmeli, Y, Falagas, ME, Giske, CG, et al. Multidrug-resistant, extensively drug-resistant and pandrug-resistant bacteria: an international expert proposal for interim standard definitions for acquired resistance. Clin Microbiol Infect. (2012) 18:268–81. doi: 10.1111/j.1469-0691.2011.03570.x
25. Tuon, FF, Rocha, JL, and Merlini, AB. Combined therapy for multi-drug-resistant Acinetobacter baumannii infection--is there evidence outside the laboratory? J Med Microbiol. (2015) 64:951–9. doi: 10.1099/jmm.0.000144
26. Kofteridis, DP, Andrianaki, AM, Maraki, S, Mathioudaki, A, Plataki, M, Alexopoulou, C, et al. Treatment pattern, prognostic factors, and outcome in patients with infection due to pan-drug-resistant gram-negative bacteria. Eur J Clin Microbiol Infect Dis. (2020) 39:965–70. doi: 10.1007/s10096-019-03784-9
27. Assimakopoulos, SF, Karamouzos, V, Eleftheriotis, G, Lagadinou, M, Bartzavali, C, Kolonitsiou, F, et al. Efficacy of Fosfomycin-containing regimens for treatment of bacteremia due to Pan-drug resistant Acinetobacter baumannii in critically ill patients: a case series study. Pathogens. (2023) 12:286. doi: 10.3390/pathogens12020286
28. Aedh, AI, Al-Swedan, AD, Mohammed, AA, Alwadai, BM, Alyami, AY, Alsaaed, EA, et al. Occurrence of multidrug-resistant strains of Acinetobacter spp.: An emerging threat for nosocomial-borne infection in Najran region, KSA. Trop Med Infect Dis. (2023) 8:108. doi: 10.3390/tropicalmed8020108
29. Rolain, J-M, Loucif, L, Al-Maslamani, M, Elmagboul, E, Al-Ansari, N, Taj-Aldeen, S, et al. Emergence of multidrug-resistant Acinetobacter baumannii producing OXA-23 Carbapenemase in Qatar. New Microbes New Infect. (2016) 11:47–51. doi: 10.1016/j.nmni.2016.02.006
30. Al-Hashem, G, Rotimi, VO, and Albert, MJ. Antimicrobial resistance of serial isolates of Acinetobacter baumannii colonizing the rectum of adult intensive care unit patients in a teaching Hospital in Kuwait. Microb Drug Resist. (2021) 27:64–72. doi: 10.1089/mdr.2020.0106
31. Nasser, K, Mustafa, AS, Khan, MW, Purohit, P, Al-Obaid, I, Dhar, R, et al. Draft genome sequences of six multidrug-resistant clinical strains of Acinetobacter baumannii, isolated at two major hospitals in Kuwait. Genome Announc. (2018) 6:e00264–18. doi: 10.1128/genomeA.00264-18
32. Vali, L, Dashti, K, Opazo-Capurro, AF, Dashti, AA, Al Obaid, K, and Evans, BA. Diversity of multi-drug resistant Acinetobacter baumannii population in a major hospital in Kuwait. Front Microbiol. (2015) 6:743. doi: 10.3389/fmicb.2015.00743
33. Ayoub Moubareck, C, Hammoudi Halat, D, Nabi, A, AlSharhan, MA, AlDeesi, ZO, Han, A, et al. Detection of OXA-23, GES-11 and NDM-1 among carbapenem-resistant Acinetobacter baumannii in Dubai: a preliminary study. J Glob Antimicrob Resist. (2020) 24:27–8. doi: 10.1016/j.jgar.2020.11.016
34. Sonnevend, Á, Ghazawi, A, Al Munthari, N, Pitout, M, Hamadeh, MB, Hashmey, R, et al. Characteristics of epidemic and sporadic strains of Acinetobacter baumannii isolated in Abu Dhabi hospitals. J Med Microbiol. (2013) 62:582–90. doi: 10.1099/jmm.0.055681-0
35. Al-Kaabi, MR, Tariq, WU-Z, and Hassanein, AA. Rising bacterial resistance to common antibiotics in Al Ain, United Arab Emirates. East Mediterr Health J. (2011) 17:479–84. doi: 10.26719/2011.17.6.479
36. Mera, RM, Miller, LA, Amrine-Madsen, H, and Sahm, DF. Acinetobacter baumannii 2002-2008: increase of carbapenem-associated multiclass resistance in the United States. Microb Drug Resist. (2010) 16:209–15. doi: 10.1089/mdr.2010.0052
37. Reddy, T, Chopra, T, Marchaim, D, Pogue, JM, Alangaden, G, Salimnia, H, et al. Trends in antimicrobial resistance of Acinetobacter baumannii isolates from a metropolitan Detroit health system. Antimicrob Agents Chemother. (2010) 54:2235–8. doi: 10.1128/AAC.01665-09
38. Ku, W-W, Kung, C-H, Lee, C-H, Tseng, C-P, Wu, P-F, Kuo, S-C, et al. Evolution of carbapenem resistance in Acinetobacter baumannii: an 18-year longitudinal study from a medical center in northern Taiwan. J Microbiol Immunol Infect. (2015) 48:57–64. doi: 10.1016/j.jmii.2013.07.005
39. Soroush, S, Haghi-Ashtiani, MT, Taheri-Kalani, M, Emaneini, M, Aligholi, M, Sadeghifard, N, et al. Antimicrobial resistance of nosocomial strain of Acinetobacter baumannii in Children’s medical Center of Tehran: a 6-year prospective study. Acta Med Iran. (2010) 48:178–84.
40. Mhondoro, M, Ndlovu, N, Bangure, D, Juru, T, Gombe, NT, Shambira, G, et al. Trends in antimicrobial resistance of bacterial pathogens in Harare, Zimbabwe, 2012-2017: a secondary dataset analysis. BMC Infect Dis. (2019) 19:746. doi: 10.1186/s12879-019-4295-6
41. Logan, LK, Gandra, S, Trett, A, Weinstein, RA, and Laxminarayan, R. Acinetobacter baumannii resistance trends in children in the United States, 1999-2012. J Pediatric Infect Dis Soc. (2019) 8:136–42. doi: 10.1093/jpids/piy018
42. Moubareck, CA, Mouftah, SF, Pál, T, Ghazawi, A, Halat, DH, Nabi, A, et al. Clonal emergence of Klebsiella pneumoniae ST14 co-producing OXA-48-type and NDM carbapenemases with high rate of colistin resistance in Dubai, United Arab Emirates. Int J Antimicrob Agents. (2018) 52:90–5. doi: 10.1016/j.ijantimicag.2018.03.003
43. Patel, S, Jhass, A, Slee, A, Hopkins, S, and Shallcross, L. Variation in approaches to antimicrobial use surveillance in high-income secondary care settings: a systematic review. J Antimicrob Chemother. (2021) 76:1969–77. doi: 10.1093/jac/dkab125
44. Thomsen, J, Abdulrazzaq, NM, and Alrand, H, The UAE AMR Surveillance Consortium. Surveillance of antimicrobial resistance in the united emirates: the early implementation phase. Front Public Health. (2023) 11:1247627. doi: 10.3389/fpubh.2023.1247627
45. Clinical laboratory and standards Instiute (CLSI). Performance standards for antimicrobial susceptibility testing. 30th ed. Wayne, PA: Clinical Laboratory and Standards Institute (CLSI) (2020).
46. Eucast. Breakpoint tables and dosages v 12.0 (2022). Available at: https://www.eucast.org/eucast_news/news_singleview?tx_ttnews%5Btt_news%5D=464&cHash=ea8540c0fbdaa71b3bbcb3bf765239de [Accessed April 29, 2023]
47. Vedadhir, AA, Rodrigues, C, and Lambert, H. Social science research contributions to antimicrobial resistance: protocol for a scoping review. Syst Rev. (2020) 9:24. doi: 10.1186/s13643-020-1279-y
48. Minssen, T, Outterson, K, Rogers Van Katwyk, S, Batista, PHD, Chandler, CIR, Ciabuschi, F, et al. Social, cultural and economic aspects of antimicrobial resistance. Bull World Health Organ. (2020) 98:823–823A. doi: 10.2471/BLT.20.275875
49. Adewoyin, MA, and Okoh, AI. The natural environment as a reservoir of pathogenic and non-pathogenic Acinetobacter species. Rev Environ Health. (2018) 33:265–72. doi: 10.1515/reveh-2017-0034
50. Peleg, AY, Seifert, H, and Paterson, DL. Acinetobacter baumannii: emergence of a successful pathogen. Clin Microbiol Rev. (2008) 21:538–82. doi: 10.1128/CMR.00058-07
51. Adegoke, AA, Mvuyo, T, and Okoh, AI. Ubiquitous Acinetobacter species as beneficial commensals but gradually being emboldened with antibiotic resistance genes. J Basic Microbiol. (2012) 52:620–7. doi: 10.1002/jobm.201100323
52. Lupo, A, Haenni, M, and Madec, J-Y. Antimicrobial resistance in Acinetobacter spp. and Pseudomonas spp. Microbiol Spectr. (2018) 6. doi: 10.1128/microbiolspec.ARBA-0007-2017
53. Mijovic, G, Pejakov, L, and Vujosevic, D. Antibiotic susceptibility of Acinetobacter species in intensive care unit in Montenegro. J Chemother. (2016) 28:273–6. doi: 10.1179/1973947815Y.0000000037
54. Prasai, A, Pant, A, Neupane, A, Pant, S, and Pradhan, S. Extensive drug resistant Acinetobacter species isolates in sputum sample of patient admitted in intensive care unit of a tertiary care Centre: a descriptive cross-sectional study. JNMA J Nepal Med Assoc. (2021) 59:860–3. doi: 10.31729/jnma.7088
55. Indhar, F, Durrani, MA, Bux, A, and Sohail, M. Carbapenemases among Acinetobacter species isolated from NICU of a tertairy care hospital in Karachi. J Pak Med Assoc. (2017) 67:1547–51.
56. Zhu, Y, Zhang, X, Wang, Y, Tao, Y, Shao, X, Li, Y, et al. Insight into carbapenem resistance and virulence of Acinetobacter baumannii from a children’s medical Centre in eastern China. Ann Clin Microbiol Antimicrob. (2022) 21:47. doi: 10.1186/s12941-022-00536-0
57. Xu, CF, and Xu, W. Drug resistance of Acinetobacter baumannii in pediatric intensive care unit. Zhonghua Er Ke Za Zhi. (2021) 59:651–7. doi: 10.3760/cma.j.cn112140-20210117-00053
58. Jalal, D, Elzayat, MG, Diab, AA, El-Shqanqery, HE, Samir, O, Bakry, U, et al. Deciphering multidrug-resistant Acinetobacter baumannii from a pediatric Cancer Hospital in Egypt. mSphere. (2021) 6:e0072521. doi: 10.1128/mSphere.00725-21
59. Peña-Tuesta, I, Del Valle-Vargas, C, Petrozzi-Helasvuo, V, Aguilar-Luis, MA, Carrillo-Ng, H, Silva-Caso, W, et al. Community acquired Acinetobacter baumannii in pediatric patients under 1 year old with a clinical diagnosis of whooping cough in Lima. Peru BMC Res Notes. (2021) 14:412. doi: 10.1186/s13104-021-05826-y
60. Ding, R, Li, X, Zhang, X, Zhang, Z, and Ma, X. The epidemiology of symptomatic catheter-associated urinary tract infections in the intensive care unit: a 4-year single center retrospective study. Urol J. (2019) 16:312–7. doi: 10.22037/uj.v0i0.4256
61. Di Venanzio, G, Flores-Mireles, AL, Calix, JJ, Haurat, MF, Scott, NE, Palmer, LD, et al. Urinary tract colonization is enhanced by a plasmid that regulates uropathogenic Acinetobacter baumannii chromosomal genes. Nat Commun. (2019) 10:2763. doi: 10.1038/s41467-019-10706-y
62. Jain, M, Sharma, A, Sen, MK, Rani, V, Gaind, R, and Suri, JC. Phenotypic and molecular characterization of Acinetobacter baumannii isolates causing lower respiratory infections among ICU patients. Microb Pathog. (2019) 128:75–81. doi: 10.1016/j.micpath.2018.12.023
63. Eveillard, M, Kempf, M, Belmonte, O, Pailhoriès, H, and Joly-Guillou, M-L. Reservoirs of Acinetobacter baumannii outside the hospital and potential involvement in emerging human community-acquired infections. Int J Infect Dis. (2013) 17:e802–5. doi: 10.1016/j.ijid.2013.03.021
64. Sannathimmappa, MB, Nambiar, V, and Aravindakshan, R. Antibiotic resistance pattern of Acinetobacter baumannii strains: a retrospective study from Oman. Saudi J Med Med Sci. (2021) 9:254–60. doi: 10.4103/sjmms.sjmms_855_20
65. Zowawi, HM, Sartor, AL, Sidjabat, HE, Balkhy, HH, Walsh, TR, Al Johani, SM, et al. Molecular epidemiology of carbapenem-resistant Acinetobacter baumannii isolates in the Gulf cooperation council states: dominance of OXA-23-type producers. J Clin Microbiol. (2015) 53:896–903. doi: 10.1128/JCM.02784-14
66. Lai, C-C, Chen, S-Y, Ko, W-C, and Hsueh, P-R. Increased antimicrobial resistance during the COVID-19 pandemic. Int J Antimicrob Agents. (2021) 57:106324. doi: 10.1016/j.ijantimicag.2021.106324
67. Segala, FV, Bavaro, DF, Di Gennaro, F, Salvati, F, Marotta, C, Saracino, A, et al. Impact of SARS-CoV-2 epidemic on antimicrobial resistance: a literature review. Viruses. (2021) 13:2110. doi: 10.3390/v13112110
68. Ayoub Moubareck, C, and Hammoudi, HD. The collateral effects of COVID-19 pandemic on the status of Carbapenemase-producing pathogens. Front Cell Infect Microbiol. (2022) 12:823626. doi: 10.3389/fcimb.2022.823626
69. Balkhy, HH, El-Saed, A, Alshamrani, MM, Alsaedi, A, Al Nasser, W, El Gammal, A, et al. Ten-year resistance trends in pathogens causing healthcare-associated infections; reflection of infection control interventions at a multi-hospital healthcare system in Saudi Arabia, 2007-2016. Antimicrob Resist Infect Control. (2020) 9:21. doi: 10.1186/s13756-020-0678-0
70. Wohlfarth, E, Kresken, M, Higgins, PG, Stefanik, D, Wille, J, Hafner, D, et al. Study group “antimicrobial resistance” of the Paul-Ehrlich-Society for Infection Therapy. The evolution of carbapenem resistance determinants and major epidemiological lineages among carbapenem-resistant Acinetobacter baumannii isolates in Germany, 2010-2019. Int J Antimicrob Agents. (2022) 60:106689. doi: 10.1016/j.ijantimicag.2022.106689
71. Tognim, MCB, Andrade, SS, Silbert, S, Gales, AC, Jones, RN, and Sader, HS. Resistance trends of Acinetobacter spp. in Latin America and characterization of international dissemination of multi-drug resistant strains: five-year report of the SENTRY antimicrobial surveillance program. Int J Infect Dis. (2004) 8:284–91. doi: 10.1016/j.ijid.2003.11.009
72. Ghosh, S, Bornman, C, and Zafer, MM. Antimicrobial resistance threats in the emerging COVID-19 pandemic: where do we stand? J Infect Public Health. (2021) 14:555–60. doi: 10.1016/j.jiph.2021.02.011
73. al-Tamimi, M, Albalawi, H, Alkhawaldeh, M, Alazzam, A, Ramadan, H, Altalalwah, M, et al. Multidrug-resistant Acinetobacter baumannii in Jordan. Microorganisms. (2022) 10:849. doi: 10.3390/microorganisms10050849
74. Moghnieh, RA, Moussa, JA, Aziz, MA, and Matar, GM. Phenotypic and genotypic characterisation of cephalosporin-, carbapenem- and colistin-resistant gram-negative bacterial pathogens in Lebanon, Jordan and Iraq. J Global Antimicrob Resist. (2021) 27:175–99. doi: 10.1016/j.jgar.2021.08.005
75. Kempf, M, Rolain, J-M, Azza, S, Diene, S, Joly-Guillou, M-L, Dubourg, G, et al. Investigation of Acinetobacter baumannii resistance to carbapenems in Marseille hospitals, south of France: a transition from an epidemic to an endemic situation. APMIS. (2013) 121:64–71. doi: 10.1111/j.1600-0463.2012.02935.x
76. Kempf, M, and Rolain, J-M. Emergence of resistance to carbapenems in Acinetobacter baumannii in Europe: clinical impact and therapeutic options. Int J Antimicrob Agents. (2012) 39:105–14. doi: 10.1016/j.ijantimicag.2011.10.004
77. Nowroozi, J, Akhavan Sepahi, A, Tahmasebinejad Kamarposhti, L, Razavipour, R, and Mazhar, F. Evaluation of ciprofloxacin (gyrA, parC genes) and tetracycline (tetB gene) resistance in nosocomial Acinetobacter baumannii infections. Jundishapur J Microbiol. (2014) 7:e8976. doi: 10.5812/jjm.8976
78. Zaki, MES, Abou ElKheir, N, and Mofreh, M. Molecular study of quinolone resistance determining regions of gyrA gene and parC genes in clinical isolates of Acintobacter baumannii resistant to fluoroquinolone. Open Microbiol J. (2018) 12:116–22. doi: 10.2174/1874285801812010116
79. Khurshid, M, Rasool, MH, Siddique, MH, Azeem, F, Naeem, M, Sohail, M, et al. Molecular mechanisms of antibiotic co-resistance among carbapenem resistant Acinetobacter baumannii. J Infect Dev Ctries. (2019) 13:899–905. doi: 10.3855/jidc.11410
80. Kengkla, K, Kongpakwattana, K, Saokaew, S, Apisarnthanarak, A, and Chaiyakunapruk, N. Comparative efficacy and safety of treatment options for MDR and XDR Acinetobacter baumannii infections: a systematic review and network meta-analysis. J Antimicrob Chemother. (2018) 73:22–32. doi: 10.1093/jac/dkx368
81. Kooli, I, Brahim, HB, Kilani, M, Gannouni, C, Aouam, A, Toumi, A, et al. Successful treatment of postoperative multidrug-resistant Acinetobacter baumannii meningitis by tigecycline. J Glob Antimicrob Resist. (2016) 5:62–3. doi: 10.1016/j.jgar.2015.12.003
82. Liu, J, Shu, Y, Zhu, F, Feng, B, Zhang, Z, Liu, L, et al. Comparative efficacy and safety of combination therapy with high-dose sulbactam or colistin with additional antibacterial agents for multiple drug-resistant and extensively drug-resistant Acinetobacter baumannii infections: a systematic review and network meta-analysis. J Glob Antimicrob Resist. (2021) 24:136–47. doi: 10.1016/j.jgar.2020.08.021
83. Ni, W, Wang, Y, Ma, X, He, Y, Zhao, J, Guan, J, et al. In vitro and in vivo efficacy of cefiderocol plus tigecycline, colistin, or meropenem against carbapenem-resistant Acinetobacter baumannii. Eur J Clin Microbiol Infect Dis. (2022) 41:1451–7. doi: 10.1007/s10096-022-04503-7
84. Sipahi, OR, Mermer, S, Demirdal, T, Ulu, AC, Fillatre, P, Ozcem, SB, et al. Tigecycline in the treatment of multidrug-resistant Acinetobacter baumannii meningitis: results of the Ege study. Clin Neurol Neurosurg. (2018) 172:31–8. doi: 10.1016/j.clineuro.2018.06.008
85. Khurshid, M, Rashid, A, Husnain, M, Rasool, MH, Waqas, U, Saeed, M, et al. In-vitro assessment of the therapeutic potential of Polymyxins and Tigecycline against Multidrugresistant Acinetobacter isolates from infected wounds. J Ayub Med Coll Abbottabad. (2020) 32:459–64.
86. Cheng, Y, Li, Y, Yu, R, Ma, M, Yang, M, and Si, H. Identification of novel tet(X3) variants resistant to Tigecycline in Acinetobacter species. Microbiol Spectr. (2022) 10:e0133322. doi: 10.1128/spectrum.01333-22
87. Fang, L-X, Chen, C, Cui, C-Y, Li, X-P, Zhang, Y, Liao, X-P, et al. Emerging high-level Tigecycline resistance: novel tetracycline Destructases spread via the Mobile Tet(X). Bioessays. (2020) 42:e2000014. doi: 10.1002/bies.202000014
88. Novović, K, and Jovčić, B. Colistin resistance in Acinetobacter baumannii: molecular mechanisms and epidemiology. Antibiotics (Basel). (2023) 12:516. doi: 10.3390/antibiotics12030516
89. Snyman, Y, Reuter, S, Whitelaw, AC, Stein, L, Maloba, MRB, and Newton-Foot, M. Characterisation of mcr-4.3 in a colistin-resistant Acinetobacter nosocomialis clinical isolate from Cape Town, South Africa. J Glob Antimicrob Resist. (2021) 25:102–6. doi: 10.1016/j.jgar.2021.03.002
90. Vivo, A, Fitzpatrick, MA, Suda, KJ, Jones, MM, Perencevich, EN, Rubin, MA, et al. Epidemiology and outcomes associated with carbapenem-resistant Acinetobacter baumannii and carbapenem-resistant Pseudomonas aeruginosa: a retrospective cohort study. BMC Infect Dis. (2022) 22:491. doi: 10.1186/s12879-022-07436-w
91. Zhen, X, Stålsby Lundborg, C, Sun, X, Gu, S, and Dong, H. Clinical and economic burden of Carbapenem-resistant infection or colonization caused by Klebsiella pneumoniae, Pseudomonas aeruginosa, Acinetobacter baumannii: a multicenter study in China. Antibiotics (Basel). (2020) 9:514. doi: 10.3390/antibiotics9080514
Keywords: Acinetobacter, United Arab Emirates, multidrug-resistance, national surveillance, antimicrobial resistance
Citation: Thomsen J, Abdulrazzaq NM, AlRand H, The UAE AMR Surveillance Consortium, Everett DB, Senok A, Menezes GA and Ayoub Moubareck C (2024) Epidemiology and antimicrobial resistance trends of Acinetobacter species in the United Arab Emirates: a retrospective analysis of 12 years of national AMR surveillance data. Front. Public Health. 11:1245131. doi: 10.3389/fpubh.2023.1245131
Edited by:
Dalal Hammoudi Halat, Qatar University, QatarReviewed by:
Pawan Kumar Kanaujia, Mahayogi Gorakhnath University, IndiaCarlos Henrique Camargo, Adolfo Lutz Institute, Brazil
Copyright © 2024 Thomsen, Abdulrazzaq, AlRand, The UAE AMR Surveillance Consortium, Everett, Senok, Menezes and Ayoub Moubareck. This is an open-access article distributed under the terms of the Creative Commons Attribution License (CC BY). The use, distribution or reproduction in other forums is permitted, provided the original author(s) and the copyright owner(s) are credited and that the original publication in this journal is cited, in accordance with accepted academic practice. No use, distribution or reproduction is permitted which does not comply with these terms.
*Correspondence: Carole Ayoub Moubareck, Y2Fyb2xlQG1vdWJhcmVjay5jb20=; Jens Thomsen, anR3LnRob21zZW5Ad2ViLmRl
†These authors have contributed equally to this work and share first authorship
‡These authors have contributed equally to this work and share last authorship