- 1Functional Environmental Microbiology, School of Natural Sciences, National University of Ireland Galway, Galway, Ireland
- 2Teagasc, Environmental Research Centre, Johnstown Castle, Wexford, Ireland
- 3Information and Computational Sciences, James Hutton Institute, Dundee, Scotland
- 4University College Dublin, Veterinary Science Centre, Dublin, Ireland
- 5Teagasc, Ashtown Food Research Centre, Ashtown, Ireland
- 6Microbial Ecology Laboratory, School of Natural Sciences, National University of Ireland Galway, Galway, Ireland
Manure and slurry may contain a range of bacterial, viral, and parasitic pathogens and land application of these organic fertilizers typically occurs without prior treatment. In-situ treatment through farm-based anaerobic digestion (AD) of such organic fertilizers co-digested with food-production wastes is multi-beneficial due to energy recovery, increased farm incomes and noxious gas reduction. Before risk assessment can be carried out at field scale an investigation of the fate of relevant target pathogens during the actual AD process must be undertaken, requiring the development of practical test systems for evaluation of pathogen survival. The present study examines miniature (50 mL) and laboratory (10 L) scale AD systems. Treatments included slurry co-digested with fats, oils, and grease (FOG) under typical operating and pasteurization conditions used in farm-based AD, in batch-fed miniature and laboratory mesophilic (37°C) continuously stirred tank reactors. Biogas production, pH, chemical oxygen demand, volatile solids, and ammonia concentration were measured throughout the trial, as were fecal indicator bacteria (FIB) i.e., total coliforms, Escherichia coli, and Enterococcus species. The miniature and laboratory bioreactors performed similarly in terms of physicochemical parameters and FIB die-off. In the absence of pasteurization, after 28 days, enterococci numbers were below the <1,000 cfu g−1 threshold required for land application, while E. coli was no longer detectable in the digestate. For comparison, FIB survival in slurry was examined and after 60 days of storage, none of the FIB tested was <1,000 cfu g−1, suggesting that slurry would not be considered safe for land application if FIB thresholds required for AD digestate were to be applied. Taken together we demonstrate that (i) miniature-scale bioreactors are valid proxies of farm-based AD to carry out targeted pathogen survival studies and (ii) in situ AD treatment of slurry prior to land application reduces the level of FIB, independently of pasteurization, which in turn might be indicative of a decreased potential pathogen load to the environment and associated public health risks.
Introduction
Approximately 1.4 billion tons of manure are produced in Europe each year, 80% of which is in the form of slurry, predominantly from cattle (Crowe et al., 2000; Foged et al., 2011). Manure and slurry represents valuable organic fertilizers, but typically contain a broad range of bacterial, viral, and parasitic pathogens (Bicudo and Goyal, 2003; Alam and Zurek, 2006; Ferens and Hovde, 2011). Human and animal pathogens commonly isolated from manure include E. coli O157, Salmonella, Listeria, Campylobacter, Cryptosporidium, Ascaris, Mycobacterium avium subspecies paratuberculosis, and Giardia (Nicholson et al., 2004; Olson et al., 2004; Grewal et al., 2006). These pathogens can be transferred to the environment as bioaerosols during landspreading (Millner, 2009; Dungan, 2010), ingested directly from grass or vegetables (Baloda et al., 2001; Braden and Tauxe, 2013), or may be washed off into connected water bodies, posing a significant threat to human and animal health (Douwes et al., 2003; Gerba and Smith, 2005; Venglovsky et al., 2009). Furthermore, manure is a potent source of noxious and greenhouse gases (GHG), which are released to the atmosphere during storage in slatted tanks and subsequent landspreading (Chadwick et al., 2011). A number of methods for limiting the impact of manure storage and landspreading, both in terms of GHG capture or mitigation and pathogen reduction have been examined, including aeration, and acidification during storage, animal diet manipulation, or alternative landspreading techniques (Nicholson et al., 2004; Franz et al., 2005; Webb et al., 2010). Typically these proposed solutions, however, consider either pathogens or GHG in isolation. Composting, for example, is suggested as an effective solution to reduce pathogens in manure (Ros et al., 2006; Vinnerås, 2007; Mc Carthy et al., 2011; Millner et al., 2014), with scant reference to gaseous N or CH4 loss to the environment (Rao et al., 2007). Conversely, methods for reduction of ammonia or other GHG losses from manure, such as acidification, rarely consider the fate of pathogens during such treatments (Kai et al., 2008; Petersen et al., 2012). In fact, in this context, the recommended direct incorporation of slurry into soil might lead to increased pathogen survival, as it inevitably reduces UV exposure (Avery et al., 2004; Hutchison et al., 2004).
Rather than tackling pathogen survival or GHG emissions from manure in isolation, a technological solution that addresses both would clearly be preferable. To that end, biogas production as a treatment for manure holds great promise (Monteny et al., 2006). In addition to the obvious benefits of energy recovery, noxious gas and GHG mitigation, farm-based AD could potentially reduce pathogen loads in the environment and the associated public health risks (Olsen and Larsen, 1987; Kearney et al., 1993; Sahlström, 2003; Jiang et al., 2018). Pathogen survival may be significantly impacted, positively or negatively, by a variety of factors. These include: pH, ammonia production, microbial competition, initial pathogen load, operating conditions of farm-based AD plants and addition of co-digestion substrates such as food production waste with varying pathogen risks (Smith et al., 2005; Orzi et al., 2015). Indeed, the AD of slurry alone is hindered by an imbalanced C:N ratio resulting in low potential methane yields of 25–30 m3 ton−1 (Weiland, 2010). To overcome this limitation, co-digestion of slurry with locally sourced organic waste is typically implemented. This in turn helps to balance the C:N ratio and thus improves the relatively low methane yield of slurry alone, whilst taking advantage of its inherent buffering capacity, microbial populations, nutrients, and moisture content (Hamelin et al., 2014; Moset et al., 2017; Neshat et al., 2017).
Congealed fats, oils, and grease (FOG) are a significant problematic food production waste internationally, causing environmental and human health issues when allowed to form “fatbergs” in municipal sewage systems (Wallace et al., 2017). Grease-traps required for licensing in the food-processing industry as well as those in restaurants mitigate the problem, but create large quantities of organic waste, which requires further treatment. The typical biogas yield of FOG (4–8 m3 kg VS −1) dwarfs that of slurry alone (0.148 m3 kg VS −1), making co-digestion of FOG with slurry in farm-based AD plants a sustainable treatment option, cheaply increasing methane output (Møller et al., 2004; Weiland, 2010; Long et al., 2012). In Ireland, successful implementation of grease-trap legislation provides a steady supply of organic waste in the form of FOG, which is used as a feedstock in the majority of Irish farm-based AD plants. The co-digestion of slurry with organic waste, however, typically requires some pasteurization treatment to be carried out as stipulated by the legislation. In that context, two pasteurization processes are available in Ireland, set out by (i) the European Union Commission (Directive No. 142/2011) as 60 continuous minutes at 70°C and (ii) the Irish Department of Agriculture, Food and the Marine as a total of 96 h at 60°C (DAFM, 2014). Pasteurization can be applied either pre- or post-AD processing with the corresponding digestate quality being assessed using fecal indicator bacteria, typically E. coli and/or enterococci. According to Regulation (EC) No.1069/2009 and Regulation (EU) No. 142/2011, for AD digestate to be deemed safe for landspreading FIB levels must <1,000 cfu g−1. As highlighted by Dennehy et al. (2018), further investigations into the effect of AD processing on pathogen loads must be carried out to determine the need for pasteurization. In addition, in order to meaningfully and accurately carry out risk assessment of digestate landspreading, the determination of the fate of relevant target pathogens during AD processing is necessary. Although previous studies of farm-based AD have reported reductions in target pathogen numbers (Olsen and Larsen, 1987; Kearney et al., 1993; Sahlström, 2003; Dennehy et al., 2018; Jiang et al., 2018), investigations into pathogen survival are typically hampered by difficulties in cultivating sufficient pathogen quantities and the public health concerns associated with spiking large volume bioreactors. Some solutions have been deployed in an effort to overcome this, including the containment of pathogens using sentinel chambers or filters held in steel baskets and submerged into digesters (Gray and Hake, 2004; Wagner et al., 2008). While this may successfully contain the pathogens and thus reduce the associated public health risks, such an experimental set-up greatly limits the interactions of the target pathogens with the surrounding matrix. There is, therefore a crucial need to develop an alternative solution closely mimicking real-life scenarios whereby interactions between pathogens and the AD liquor are not hindered.
Thus the aims of this study were to: (i) propose and validate the use of miniature-scale (50 mL) bioreactors as proxies for 10 L bioreactors; (ii) determine FIB survival under typical operating and pasteurization conditions used in farm-based AD systems; and (iii) assess the suitability of AD as a means of reducing the environmental impact of slurry management.
Materials and Methods
Feedstock Selection, Collection, and Storage
In order to determine feedstock composition and operating conditions, a characterization of current Irish AD facilities was carried out. All Irish farm-based AD plants currently operate at mesophilic temperatures and process slurry co-digested with food production waste, including FOG (Auer et al., 2016). By visiting these AD facilities and utilizing knowledge gained in Auer et al., the following operation conditions and feedstock composition were determined.
First, a cattle slurry:FOG ratio of 2:1 was used, with a view to replicating full-scale farm-based AD. The FOG was sourced from the Bioenergy and Organic Fertilizer Services (BEOFS) AD plant in Camphill, County Kilkenny, Ireland, collected in a 25 L drum, stored at 4°C, and mixed thoroughly before use. Cattle slurry for feeding the bioreactors was collected from a dairy farm in County Galway, Ireland in October, 2016. The slatted housing storage tanks were agitated to homogenize the slurry before collection of the sample using a bucket attached to a pole, in accordance with Brennan et al. (2011) and Peyton et al. (2016). Slurry was stored in a 25 L sealed container at 4°C for 2 days prior to use as feedstock, at which time it was mixed thoroughly. In order to establish levels of farm to farm variation, dairy cattle slurry was collected from two additional farms in County Galway during October 2016. For comparison between digestate and stored slurry, triplicate slurry samples for each farm were stored in a shed at ambient Irish environmental temperatures during October–December, to mimic on-farm storage.
Inoculum Development
Digestate from the BEOFS full-scale mesophilic continuously-stirred tank reactors (CSTR) co-digesting FOG with slurry was used as the starting inoculum, as it was adapted to the chosen substrate. This inoculum was found, through biomethane potential assays (BMP, data not shown), to be sub-optimal for biogas production. Therefore, augmentation with a mixture of slurry and methanogenic anaerobic granular sludge was deemed necessary to bolster both hydrolysis and methanogenesis. A series of specific methanogenic assays (SMA) were carried out using non-gaseous (acetate, ethanol, propionate, butyrate) and gaseous substrates (H2/CO2) as described by Coates et al. (1996). Based on the SMA results, a 2:1:1 ratio of granular sludge:BEOFS:slurry was selected as the optimum inoculum mixture (Figure S1).
Miniature- and Laboratory-Scale Bioreactors Operation
Three 10-L CSTRs (R1–R3) were operated at 37°C in batch with a 28-day solid retention time. Prior to operation, the inoculum and starting liquor were adjusted to pH 7 by adding NaHCO3. The organic loading rate for each bioreactor was 30 g VS L−1 in a 2:1 inoculum to feedstock ratio with a 7 L working volume. Submerged, motor-propelled axial stirrers with large scale paddles were centrally installed in the bioreactor ceilings, with an externally positioned motor, as is typical of agricultural biogas plants (Weiland, 2010). Miniature batch tests (33 mL in 50 mL glass bottles) using identical inoculum and feedstock ratios to the 10 L bioreactors were run simultaneously at 37°C under shaking conditions in a New Brunswick Scientific Innova°44 incubator and destructively sampled in triplicate at regular intervals (days 0, 7, 14, 21, 28), for comparison. Their contents, as well as samples collected from the 10 L bioreactors were analyzed as described below.
Analytical Methods
Biogas volume from the 10 L and 50 mL bioreactors was determined using the water displacement method and 10 mL syringes attached with a stopcock, respectively. Methane content of the biogas was analyzed using a Varian gas chromatograph equipped with a flame ionization detector. The carrier gas was nitrogen and the flow rate was 25 mL min−1. Analysis of TS and VS was performed gravimetrically according to standard methods (APHA., 2005). Soluble chemical oxygen demand (sCOD) was determined by analyzing the supernatant of centrifuged samples. Total chemical oxygen demand (tCOD) and sCOD analyses were performed according to the Standing Committee of Analysts. (1985). NH3 concentrations (mg L−1) were determined using the HACH AmVer High-Range Ammonia test, available from HACH.
Pasteurization
In addition to the unpasteurized 50 mL bioreactors used for comparison with the 10 L CSTRs, four pasteurization conditions were examined at the miniature scale to determine the impact on bioreactor performance and FIB survival. At each time point, two pre-AD pasteurization conditions (P1: 60°C for 96 h; P2: 70°C for 1 h) were used on the food production waste, and two post-AD pasteurization conditions (P3: 60°C for 96 h; P4: 70°C for 1 h) were applied to the digestate. These assays were carried out in triplicate for each time point, totaling 75 miniature-scale assays. Water baths set to the appropriate temperatures were used for pasteurization, and temperature probes were employed to ensure the designated temperature was achieved.
Fecal Indicator Bacteria Monitoring
In line with the EU Regulation, total coliforms, E. coli, and enterococci numbers were monitored throughout the trial. Most probable numbers (MPN) of total coliforms and Escherichia coli were quantified using IDEXX Colisure with Quanti-Tray/2000 incubated at 35°C for 24 h. MPN of enterococci were determined using IDEXX Enterolert kit with Quanti-Tray/2000 incubated at 41°C for 24 h. Slurry and digestate samples were diluted as necessary to fall within the detection range (1 - 2419.6 cfu 100 mL−1) in sterilized phosphate buffered saline (Colisure) and sterilized distilled water (Enterolert).
Assessing Treatment Effects on FIB Die-Off With Bayesian Hierarchical Modeling
Bayesian hierarchical modeling was used to compare the effects of vessel volume and pasteurization conditions on FIB die-off. Weak Cauchy-distributed priors were used for the pooled parameter estimates of the regression, to allow for outliers (Gelman and Hill, 2006). Stan version 2.17.0 (Carpenter et al., 2017) was used to generate samples from the model using the Rstan interface (Stan Development Team, 2017). The data, model, analysis scripts, and interpretation of the results can be found at https://github.com/nickp60/SI_Nolan_etal_2018. A difference in parameter estimates was considered significant if the 95% confidence intervals were exclusive.
Results
Slurry Characterization
The slurry collected from the three farms was tested prior to AD, for initial FIB levels as well as total solids and volatile solids (Table 1). TS and VS were consistent across the samples tested, whilst coliforms and E. coli numbers were highest in samples from Farm C. In all cases, enterococci numbers were lower than coliforms and E. coli.
Miniature- (50 ml) and Laboratory-Scale (10 L) Bioreactor Performance Is Similar
The recorded performance data in the comparative trial displayed similar trends for miniature- and laboratory-scale bioreactors. The pH for both bioreactor scales remained between 7.6 and 8.1 throughout the experiment (Figure S2). Volatile solids (VS) degradation was comparable for the 50 mL and 10 L bioreactors with 64 and 61% VS removal, respectively within the first 7 days (Figure 1A). Similar trends in ammonia concentration (Figure 1B) were also observed across the two scales, with an increase over the first 2 weeks of the trial from 937 to 1,233 mg L−1 in the 10 L bioreactors and from 865 to 1,038 mg L−1 in the 50 mL bioreactors. This increase likely results from the breakdown of organic compounds. As ammonia concentration has been identified as an important factor in pathogen reduction (Watcharasukarn et al., 2009, the similarity between the scaled bioreactors is of particular relevance.
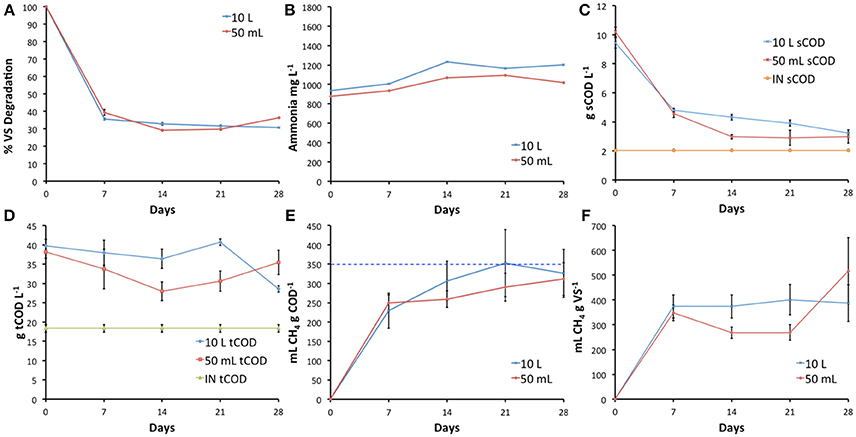
Figure 1. Comparison of means (n = 3) of key process performance indicators in 10 L and 50 mL mesophilic continuously stirred bioreactors, with standard deviation error bars. (A) Volatile solids degradation %. (B) Ammonia concentration (mg L−1). (C) Soluble COD removal (g L−1). (D) Total COD removal (g L−1). (E) Volume of methane produced per gram of COD removed, with dashed line denoting theoretical methane yield (Batstone et al., 2002). (F) Volume of methane produced per gram VS.
Soluble and total chemical oxygen demand (sCOD and tCOD) concentrations were also consistent across the two bioreactor scales (Figures 1C,D). Soluble COD and tCOD removal primarily occurred within the first 7 days, reaching a maximum of 87–88% by Day 28 for both bioreactor scales (Figures 1C,D). The majority of methane production occurred within 14 days, reaching 77.5 and 82% of the total recorded in the 10 L and 50 mL bioreactors within that time frame (Figure S3A). Although similar methane production trends were observed at both bioreactor scales, the larger scale bioreactors approached the theoretical yield proposed by Batstone et al. (2002) of 350 mL CH4 g−1 of COD at Day 21 compared to Day 28 for the 50 mL bioreactors (Figure 1E). This could partly be attributed to the more thorough mixing occurring in the larger bioreactors.
Bayesian Hierarchical Modeling
A Bayesian hierarchical model was developed to compare the effects of vessel volume and pasteurization conditions on FIB survival. In short, both the initial effect (from day 0 to day 7) and the latter effect (from day 7 to day 28) of the conditions were considered in relation to the underlying behavior of the data. This piece-wise approach was able to accurately model both the initial perturbation (the addition of feedstock to the inoculum) and the recovery of the system (https://github.com/nickp60/SI_Nolan_etal_2018).
Fecal Indicator Bacteria Survival Is Comparable in 50 ml and 10 L Bioreactors
Fecal indicator bacteria levels should be reduced to <1,000 cfu g−1 for the safe landspreading of digestate (Regulation (EC) No.1069/2009 and Regulation (EU) No. 142/2011). Total coliforms survival showed similar trends in both 50 mL and 10 L bioreactors, with a 3.7 and 4.3 log10 reduction after 7 days (Figure 2A). A similar trend in E coli die-off was also observed in both bioreactor scales (Figure 2B). The initial 3.5–4.3 log10 reductions of both coliforms and E. coli occurring within 7 days (Figures 2A,B), followed by relatively stable survival until 21 days suggests the presence of resilient cells with increased ability to survive under mesophilic AD conditions. Although enterococci numbers were slightly above 1,000 cfu g−1 after 21 days, greater than 3.0 log10 reduction was observed after 28 days in both bioreactor scales (Figure 2C). The parameter estimates obtained from piece-wise modeling of the FIB die-off data showed well-overlapping confidence intervals, indicating no significant difference between the two bioreactor volumes.
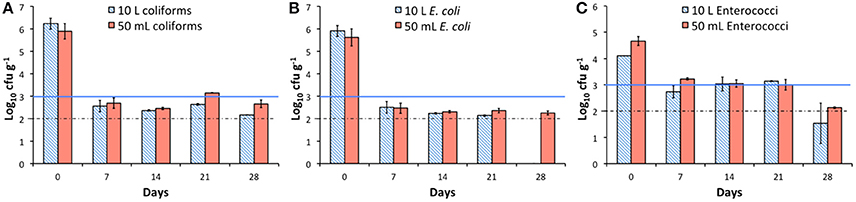
Figure 2. Comparison of average fecal indicator bacteria survival in 10 L and 50 mL bioreactors (n = 3), with standard deviation error bars. (A) Total coliforms; (B) E. coli; (C) Enterococci. Blue line denotes EU digestate standard requirement, dashed line represents the limit of detection.
Pre-pasteurization Impacts Scod Removal and Methane Yield
Two pre-AD (P1: 60°C for 96 h and P2: 70°C for 60 min) and two post-AD pasteurization regimes (P3: 60°C for 96 h and P4: 70°C for 60 min) were tested at the miniature scale. Volatile solids degradation was relatively consistent across all conditions, as was ammonia concentration (Figures 3A,B). For both total COD and soluble COD, the rate of removal within the first 7 days was notably higher for AD of feedstock that had been pre-pasteurized at 60°C for 96 h (89 vs. 74–80% for sCOD, Figure 3; and 93 vs. 82–85% for tCOD; data not shown). The impact of P1 on COD removal was observed at the first time point only, as by Day 14, the other conditions displayed similar results (Figure 3C). Although the total volume of methane produced for P1 was similar to the other conditions, high levels of COD removal combined with low biogas quality (22–40% CH4 Day 2, 54–68% CH4 Day 5; Figure S3B) resulted in lower yields of 146 mL CH4 g COD−1 by Day 7 (Figure 3D), compared with 227 mL CH4 g COD−1 for no pasteurization. Pre- pasteurization at the EU standard (P2) improved methane yield, approaching the maximum theoretical methane yield of 350 mL CH4 g COD−1 within 7 days (Figure 3D; Batstone et al., 2002). As expected, the two post-AD conditions had no impact on the AD process itself and the results for key performance indicator data recorded for P3 and P4 (Figure 3) were comparable to those of the unpasteurized condition presented in Figure 1.
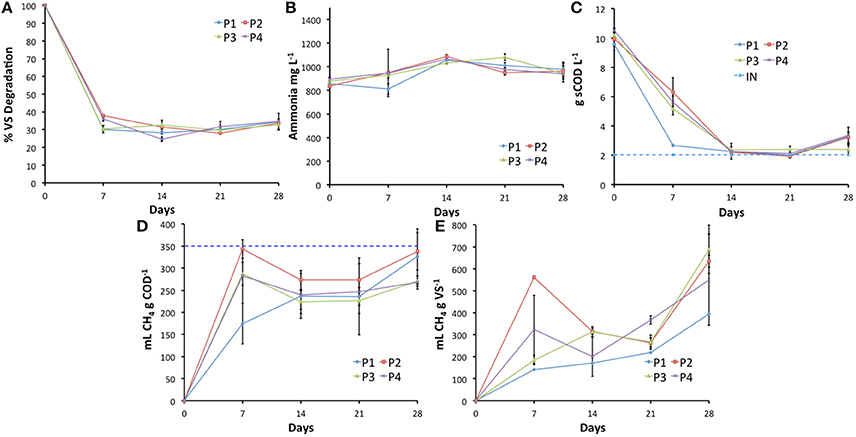
Figure 3. Comparison of means (n = 3) of key process performance indicators following four pasteurization treatments, with standard deviation error bars. (A) Volatile solids degradation %; (B) Ammonia concentration (mg L−1); (C) Soluble COD removal (g L−1); (D) Volume of methane produced per gram of COD removed, with dashed line denoting theoretical methane yield (Batstone et al., 2002); (E) Volume of methane produced per gram VS.
Post-AD Pasteurization Decreases Fecal Indicator Bacteria Survival
Pre-AD pasteurization (P1 and P2) was carried out on the food production waste prior to mixing with slurry and feeding into bioreactors, as is standard practice. This resulted in a reduction in E. coli (1.19–1.33 log10) numbers on Day 0, particularly for P1, but had minimal impact on total coliform numbers compared with no pasteurization (Figures 2, 4). Overall, the effect of pre-pasteurization treatments (P1 and P2) on FIB survival was not statistically significant. Post-AD treatment under Irish and EU transformation parameters (P3 and P4) resulted in lower coliform and E. coli numbers in the digestate, when compared with unpasteurized (Figures 2, 4). When comparing pre-pasteurization with post pasteurization, the post-pasteurized treatments showed significantly lower coliform counts; whilst the other indicators shared similar trends (P1 and P2; Figure 4). At all post-AD pasteurization time-points, coliforms and E. coli were below the limit of detection in the majority of replicates, while enterococci numbers were below 1,000 cfu g−1 within 7 days (Figure 4).
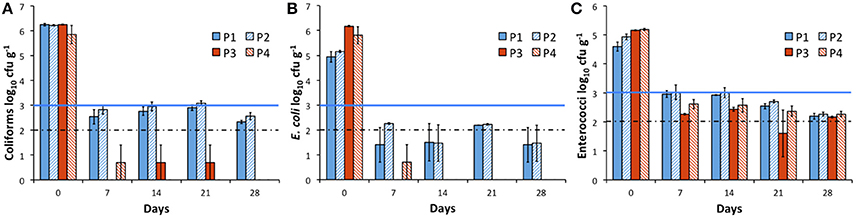
Figure 4. Comparison of means (n = 3) of fecal indicator bacteria survival, presented as log10 colony forming units per gram following four pasteurization treatments, with standard deviation error bars. P1: Pre-AD, 60°C for 96 h; P2: Pre-AD, 70°C for 1 h; P3: Post-AD, 60°C for 96 h; P4: Post-AD, 70°C for 1 h. (A) Total coliforms; (B) E. coli; (C) Enterococci. Blue line denotes EU digestate standard requirement, dashed line represents the limit of detection.
AD Treatment Effectively Reduces Fecal Indicator Bacteria Levels Compared to Stored Slurry
Cattle slurry from three dairy farms was stored in a shed at ambient environmental temperature for 56 days (between 4 and 13°C in Galway, Ireland). Over the first 7 days of storage there was a 0.32 and 0.36 log10 reduction in coliforms and E. coli numbers respectively, and a slight increase in enterococci numbers. Hence, within 7 days of AD treatment, the resulting digestate was superior to stored slurry in terms of FIB inactivation (Figure 5). It is worth noting that an initial dilution factor of 1–1.5 log10 is evident in the digestate when compared with unprocessed slurry. This is due to the mixing of slurry with FOG and microbial inoculum prior to AD processing. After 2 months of storage, none of the FIB tested in slurry had dropped below the EU minimum digestate quality standards of 1,000 cfu g−1 (Figure 5).
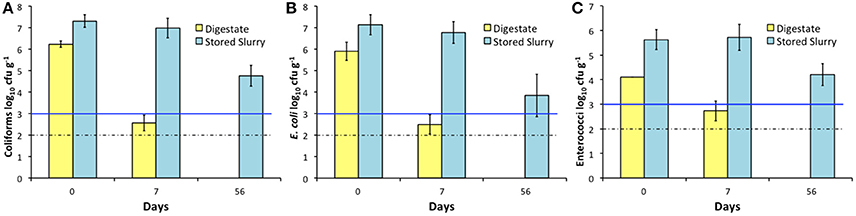
Figure 5. Comparison of FIB removal in 10 L mesophilic continuously stirred tank bioreactors (n = 3) and stored slurry (n = 9), with standard deviation error bars. All FIB survived 56 days storage at ambient temperature at levels exceeding EU limits; 1 week of AD processing was sufficient to bring FIB counts beneath the EU limits, and eventually beneath the limit of detection. Blue line denotes EU digestate standard requirement, dashed line represents the limit of detection. (A) Total coliforms (log10 cfu g−1); (B) E. coli (log10 cfu g−1); (C) Enterococci (log10 cfu g−1).
Discussion
Systematic examination of the fate of key viral, bacterial and protozoan pathogens in farm-based anaerobic co-digestion of various wastes is hampered by availability of sufficient pathogenic biomass as well as health and safety concerns associated with spiking large-volume bioreactors. This makes the use of larger scale bioreactors for pathogen survival studies impractical. Here, we carried out a comparative trial across two bioreactor scales, of 50 mL and 10 L, in order to assess the potential use of miniature-scale AD bioreactors as proxies for larger scales. Across all the major physicochemical parameters recorded, both bioreactor scales displayed similar trends. The volatile solids removals obtained in the present study were in line with those reported in the literature (64–67%—Neves et al., 2009; Luste et al., 2012). The majority (61–64%) of the volatile solids degradation at both scales occurred within 7 days (Figure 1A), demonstrating the potential for reduced retention time of the substrate in the bioreactors. Reported methane yields vary significantly, depending on feedstock mixtures and ratios, retention time, and temperatures, but a range between 200 and 489 mL g VS−1 is typical of co-digestion containing manure as the primary constituent with food production waste (200–350 mL g VS−1–Neves et al., 2009; 470 mL g VS−1–Creamer et al., 2010; 260 mL g VS−1–Luste et al., 2012; 320–489 mL g VS−1 Dennehy et al., 2016). The range of 220–488 mL CH4 g VS−1 recorded in the present work falls within those previously reported. Here we demonstrate, at 50 mL and 10 L bioreactor scales, that mesophilic AD of slurry co-digested with FOG effectively reduces coliforms and E. coli numbers within 7 days (Figures 2A,B). Similarly, whilst examining the effect of varying ratios of pig slurry co-digested with food waste in dry-AD, Jiang et al. (2018) recently reported coliform and E. coli inactivation within 7 days, identifying free VFA concentration as a primary factor in inactivation. Dennehy et al. (2018) found similarly reduced levels of E. coli (1.2–2.2 log10 cfu g−1) in mesophilic CSTR co-digesting pig manure with food waste, although higher total coliform values were reported (4–6 log10). The higher total coliforms reported by Dennehy et al. (2018) may be due to reduced mixing (1 h per day), decreased hydraulic retention time and feeding regime employed (daily feeding vs. batch) when compared to the present study.
Using Bayesian hierarchical modeling provided a flexible framework for assessing the statistical significance of the indicator die-off rates. As the vast majority of change in FIB numbers occurred within the initial 7 days, taking a piecewise approach allowed assessment of both the initial effect of the feedstock addition under the different pasteurization schemes, and also the long-term effect on FIB counts in the system as it stabilized over time. We hope that by releasing both the data and models used to assess the data, such an approach will become a regular tool in assessing bioreactor performance, particularly in relation to pathogen survival.
The results obtained for both bioreactor scales indicate higher enterococci survival in mesophilic anaerobic co-digestion of slurry with FOG, compared with coliforms or E. coli. This observation is in agreement with the previously reported examination of four full-scale Swedish biogas plants, one thermophilic and three mesophilic, co-digesting manure with kitchen, and food-processing waste, where higher numbers of enterococci than coliforms were consistently found in the digestate, despite the use of pre-AD pasteurization in all four plants (Bagge et al., 2005). Furthermore, the enterococci survival results of Bagge et al. (2005) mirror closely those of Dennehy et al. (2018), whereby ~3 log10 cfu g−1 were consistently recorded, using a continuously fed system and three different ratios of pig manure to food waste. Based on these observations, enterococci are recommended as a better indicator for pathogen survival during AD processes (Larsen et al., 1994; Sahlström, 2003).
Numerous studies have examined the impact of pre-AD pasteurization on process performance, typically anticipating improved methane yield caused by preliminary hydrolysis of the feedstock (Luste and Luostarinen, 2010). The corresponding results have however varied widely, ranging from a methane production reduction of up to 34% during the co-digestion of slaughterhouse waste (SHW) with the organic fraction of municipal solid waste (Cuetos et al., 2010) to no significant effect during the AD of SHW (Hejnfelt and Angelidaki, 2009; Ware and Power, 2016), through 14–25% improvements during the co-digestion of SHW and slurry (Paavola et al., 2006; Luste and Luostarinen, 2010). Edström et al. (2003) initially reported a 400% increase in BMPs of pasteurized vs. unpasteurized SHW, although this yield was not achieved in laboratory or pilot-scale trials. The variability of these results is likely due to differences in biochemical properties of the feedstocks used, as demonstrated in a study examining the effects of pre-treatment on five different components of SHW (Luste et al., 2009). In the present study, the methane output when FOG was pre-pasteurized at 70°C for 1 h was statistically higher than the other conditions in the first 7 days of this trial (Figure 3D,E), although Carrere et al. (2016) advise against extrapolating such results to full-scale plants without complex modeling. Although methanogenesis appears to have been impacted differentially by the two pre-AD pasteurization conditions tested, FIB survival was similar for both conditions. Slightly higher FIB numbers were recorded after 28 days in systems processing pre-pasteurized feedstock (P1 and P2; Figure 4). This may be indicative of reduced competition for resources, whereby pre-pasteurization reduced the microbial populations in the feedstock, enabling increased FIB survival and/or regrowth of resilient strains or cells.
A number of pasteurization conditions were examined by Coultry et al. (2013) to determine the energy consumption and consequent economic impact on viability of AD plants. Pre-AD pasteurization was demonstrated to be prohibitively expensive; most notably, the energy required to meet the Irish national transformation standard (P1) equates to 4,544% of the digester's output, which is an 80-fold increase in energy consumption when compared with the already prohibitive EU requirement (P2). These numbers are likely to be lower in practice however, as only the imported materials are pasteurized before mixing in with indigenous slurry, reducing the pasteurization treatment efficacy as seen in the FIB survival results for P1 and P2. The energy cost of post-AD pasteurization is mitigated by the mesophilic digestate, but was still found by Coultry et al. (2013) to be substantial, at 30 and 1,893% of the digester's annual energy output for EU (P4) and national standards (P3) respectively. Although some measures could be taken to reduce these costs, such as separation of liquid and solids, they are clearly a substantial burden to the economic viability of bioreactor operation. This burden hinders adoption of farm-based AD and is worth reconsideration in light of the reduction in FIB numbers in unpasteurized trials and the absence of hygienization requirements for unprocessed slurry. The FIB survival rates monitored in the stored slurry are in line with previous studies such as that of Nicholson et al. (2005), who found that E. coli O157, Salmonella and Campylobacter survived for up to 3 months during dairy slurry storage. Similarly, Mycobacterium avium subspecies paratuberculosis has been found to survive beyond 56 days in stored slurry at ambient temperatures (Grewal et al., 2006). Furthermore, survival of pathogens in stored slurry increases with temperatures below 10°C, such as those typical of winter storage months in north-western European climates (Kudva et al., 1998). In terms of potential pathogen load to the environment, as assessed via the monitoring of FIB levels, we have demonstrated that mesophilic anaerobic co-digestion of slurry with food production waste is superior to simple slurry storage without treatment. Moreover, the slight increase in numbers of enterococci over the first 7 day period of slurry storage (Figure 5) highlights the potential risk of pathogens thriving in this environment. Based on these findings, if the EU standard for digestate was applied to slurry (<1,000 cfu g−1), all livestock farms would be required to adopt some form of treatment.
Previous studies have examined the agronomic benefits of anaerobic digestion (AD) of slurry. Benefits include increased homogeneity and decreased viscosity, due to the reduction in volatile solids, resulting in more uniform landspreading (Massé et al., 1997). As detailed by Massé et al. (2011), other studies have demonstrated the added fertilizer value of digestate compared with slurry and mineral fertilizer, resulting from improved plant N uptake and increases in N and P mineralization (Massé et al., 2007; Chantigny et al., 2009). When these agronomic improvements, energy production, waste reduction and mitigation of GHG emissions are considered together with reduced pathogen load to the environment, widespread adoption of AD as a means of slurry amendment prior to landspreading should be encouraged (Clemens et al., 2006). The enterococci survival observed in this study highlights however the scope for future work to improve pathogen inactivation during farm-based AD. Optimization of operational conditions for FIB reduction is currently underway. Future work focusing on landspreading field trials will be necessary to assess further comparative risk from digestate and unprocessed slurry.
Conclusion
In this study we demonstrate that (i) miniature 50 mL bioreactors are valid proxies of farm-based AD to carry out targeted pathogen survival investigations and (ii) in situ AD treatment of slurry prior to land application reduces the level of FIB compared to slurry storage alone, independently of pasteurization, which in turn might be indicative of a decreased potential pathogen load to the environment and associated public health risks. While pathogen indicator die-off was observed, enterococci survival highlights the opportunity for process optimization with a focus on hygienization.
Author Contributions
FA and SN designed the research. SN and AA ran the bioreactors. NW and LP carried out the modeling. SN and FA analyzed the data. SN, FA, NW, and LP wrote the paper with input from VO, FB, OF, KR, and DB.
Funding
This work was carried out as part of the FIRM Project, 14 F847, The comparative public health risks associated with spreading anaerobic digestate, animal manure, and slurry on land: Science, policy and practice, funded by the Irish Department of Agriculture, Food and Marine. NW was funded by a joint studentship by NUI Galway and the James Hutton Institute.
Conflict of Interest Statement
The authors declare that the research was conducted in the absence of any commercial or financial relationships that could be construed as a potential conflict of interest.
Supplementary Material
The Supplementary Material for this article can be found online at: https://www.frontiersin.org/articles/10.3389/fsufs.2018.00041/full#supplementary-material
Figure S1. Comparison of methanogenic activity performance of various ratios of granular sludge:BEOFS digestate:slurry for development of inoculum (n = 3). FOG: Fats, oils and grease; PRO: Propionate; BUT: Butyrate; ETH: Ethanol; ACE: Acetate.
Figure S2. Recorded pH for (A) 10 L and 50 mL bioreactors processing unpasteurized slurry and FOG; (B) 50 mL bioreactors testing pasteurization conditions.
Figure S3. Mean methane percentages for 10 L and 50 mL (A) and P1-P4 (B) at all timepoints (n = 3), with standard deviation error bars. Detailed information about the models used, in addition to the data and analysis script, can be found at http://nickp60.github.io/SI_Nolan_etal_2018.
References
Alam, M. J., and Zurek, L. (2006). Seasonal prevalence of Escherichia coli O157:H7 in beef cattle feces. J. Food Prot. 69, 3018–3020. doi: 10.4315/0362-028X-69.12.3018
Auer, A., Vande Burgt, N. H., Abram, F., Barry, G., Fenton, O., Markey, B. K., et al. (2016). Agricultural anaerobic digestion power plants in Ireland and Germany: policy and practice J. Sci. Food Agric. 97, 719–723. doi: 10.1002/jsfa.8005
APHA. (2005). Standard Methods for the Examination of Water and Wastewater. 21st Edn. Washington, DC: APHA-AWWA-WEF. Water Environment Research, 453–456.
Avery, L. M., Hill, P., Killham, K., and Jones, D. L. (2004). Escherichia coli O157 survival following the surface and sub-surface application of human pathogen contaminated organic waste to soil. Soil Biol. Biochem. 36, 2101–2103. doi: 10.1016/j.soilbio.2004.05.018
Bagge, E., Sahlström, L., and Albihn, A. (2005). The effect of hygienic treatment on the microbial flora of biowaste at biogas plants. Water Res. 39, 4879–4886. doi: 10.1016/j.watres.2005.03.016
Baloda, S. B., Christensen, L., and Trajcevska, S. (2001). Persistence of a Salmonella enterica serovar typhimurium DT12 clone in a piggery and in agricultural soil amended with Salmonella-contaminated slurry. Appl. Environ. Microbiol. 67, 2859–2862. doi: 10.1128/AEM.67.6.2859-2862.2001
Batstone, D. J., Keller, J., Angelidaki, I., Kalyuzhnyi, S. V., Pavlostathis, S. G., Rozzi, A., et al. (2002). The IWA Anaerobic Digestion Model No 1 (ADM1). Water Sci. Technol. 45, 65–73. doi: 10.2166/wst.2002.0292
Bicudo, J. R., and Goyal, S. M. (2003). Pathogens and manure management systems: a review. Environ. Technol. 24, 115–130. doi: 10.1080/09593330309385542
Braden, C. R., and Tauxe, R. V. (2013). Emerging trends in foodborne diseases. Infect. Dis. Clin. North Am. 27, 517–533. doi: 10.1016/j.idc.2013.06.001
Brennan, R. B., Fenton, O., Grant, J., and Healy, M. G. (2011). Impact of chemical amendment of dairy cattle slurry on phosphorus, suspended sediment and metal loss to runoff from a grassland soil. Sci. Total Environ. 409, 5111–5118. doi: 10.1016/j.scitotenv.2011.08.016
Carpenter, B., Gelman, A., Hoffman, M., Lee, D., Goodrich, B., Betancourt, M., et al. (2017). Stan: a probabilistic programming language. J. Stat. Softw. 76, 1–32. doi: 10.18637/jss.v076.i01
Carrere, H., Antonopoulou, G., Affes, R., Passos, F., Battimelli, A., Lyberatos, G., et al. (2016). Review of feedstock pretreatment strategies for improved anaerobic digestion: from lab-scale research to full-scale application. Bioresour. Technol. 199, 386–397. doi: 10.1016/j.biortech.2015.09.007
Chadwick, D., Sommer, S., Thorman, R., Fangueiro, D., Cardenas, L., Amon, B., et al. (2011). Manure management: implications for greenhouse gas emissions. Anim. Feed Sci. Technol. 166–167, 514–531. doi: 10.1016/j.anifeedsci.2011.04.036
Chantigny, M. H., MacDonald, J. D., Beaupré, C., Rochette, P., Angers, D. A., Massé, D., et al. (2009). Ammonia volatilization following surface application of raw and treated liquid swine manure. Nutr. Cycl. Agroecosyst. 85, 275–286. doi: 10.1007/s10705-009-9266-7
Clemens, J., Trimborn, M., Weiland, P., and Amon, B. (2006). Mitigation of greenhouse gas emissions by anaerobic digestion of cattle slurry. Agric. Ecosyst. Environ. 112, 171–177. doi: 10.1016/j.agee.2005.08.016
Coates, J. D., Coughlan, M. F., and Colleran, E. (1996). Simple method for the measurement of the hydrogenotrophic methanogenic activity of anaerobic sludges. J. Microbiol. Methods 26, 237–246. doi: 10.1016/0167-7012(96)00915-3
Coultry, J., Walsh, E., and McDonnell, K. P. (2013). Energy and economic implications of anaerobic digestion pasteurization regulations in Ireland. Energy 60, 125–128. doi: 10.1016/j.energy.2013.07.059
Creamer, K. S., Chen, Y., Williams, C. M., and Cheng, J. J. (2010). Stable thermophilic anaerobic digestion of dissolved air flotation (DAF) sludge by co-digestion with swine manure. Bioresour. Technol. 101, 3020–3024. doi: 10.1016/j.biortech.2009.12.029
Crowe, M., Fanning, A., Nolan, K., Carty, G., and Howley, D. (2000). National Waste Report 1998. Wexford: Environmental Protection Agency, Co.
Cuetos, M. J., Gómez, X., Otero, M., and Morán, A. (2010). Anaerobic digestion and co-digestion of slaughterhouse waste (SHW): influence of heat and pressure pre-treatment in biogas yield. Waste Manag. 30, 1780–1789. doi: 10.1016/j.wasman.2010.01.034
Dennehy, C., Lawlor, P. G., Croize, T., Jiang, Y., Morrison, L., Gardiner, G. E., et al. (2016). Synergism and effect of high initial volatile fatty acid concentrations during food waste and pig manure anaerobic co-digestion. Waste Manag. 56, 173–180. doi: 10.1016/j.wasman.2016.06.032
Dennehy, C., Lawlor, P. G., McCabe, M. S., Cormican, P., Sheahan, J., Jiang, Y., et al. (2018). Anaerobic co-digestion of pig manure and food waste; effects on digestate biosafety, dewaterability, and microbial community dynamics. Waste Manag. 71, 532–541. doi: 10.1016/j.wasman.2017.10.047
Douwes, J., Thorne, P., Pearce, N., and Heederik, D. (2003). Bioaerosol health effects and exposure assessment: progress and prospects. Ann. Occup. Hyg. 47, 187–200. doi: 10.1093/annhyg/meg032
Department of Agriculture Food the Marine (DAFM) (2014) Approval and Operations of Biogas Plants Transforming Animal By-Products and Derived Products in Ireland (CN11). Available online at: https://www.agriculture.gov.ie/media/migration/foodindustrydevelopmenttrademarkets/animalby-products/applicationformsconditionsforabpprocessingoperations/conditionsforms/CN11ApprovalOperationBiogasPlants200617.pdf (Accessed 10 February, 2018).
Dungan, R. S. (2010). BOARD-INVITED REVIEW: fate and transport of bioaerosols associated with livestock operations and manures. J. Anim. Sci. 88, 3693–3706. doi: 10.2527/jas.2010-3094
Edström, M., Nordberg, A., and Thyselius, L. (2003). Anaerobic treatment of animal byproducts from slaughterhouses at laboratory and pilot scale. Appl. Biochem. Biotechnol. 109, 127–138. doi: 10.1385/ABAB:109:1-3:127
Ferens, W. A., and Hovde, C. J. (2011). Escherichia coli O157:H7: animal reservoir and sources of human infection. Foodborne Pathog. Dis. 8, 465–487. doi: 10.1089/fpd.2010.0673
Foged, H. L., Flotats, X., Blasi, A. B., Palatsi, J., Magri, A., and Schelde, K. M. (2011). Inventory of Manure Processing Activities in Europe. Technical Report No. I concerning “Manure Processing Activities in Europe” to the European Commission, Directorate-General Environment. Agro Business Park in cooperation with GIRO Centre Tecnològic.
Franz, E., van Diepeningen, A. D., de Vos, O. J., and van Bruggen, A. H. C. (2005). Effects of cattle feeding regimen and soil management type on the fate of Escherichia coli O157:H7 and salmonella enterica serovar typhimurium in manure, manure-amended soil, and lettuce. Appl. Environ. Microbiol. 71, 6165–6174. doi: 10.1128/AEM.71.10.6165-6174.2005
Gelman, A., and Hill, J. (2006). Data Analysis Using Regression and Multilevel/Hierarchical Models. New York, NY: Cambridge University Press.
Gerba, C. P., and Smith, J. E. (2005). Sources of pathogenic microorganisms and their fate during land application of wastes. J. Environ. Qual. 34, 42–48. doi: 10.2134/jeq2005.0042
Gray, D. M. D., and Hake, J. M. (2004). Pathogen Destruction Efficiency in High-Temperature Digestion. London: IWA Publishing.
Grewal, S. K., Rajeev, S., Sreevatsan, S., and Michel, F. C. (2006). Persistence of Mycobacterium avium subsp. Paratuberculosis and other zoonotic pathogens during simulated composting, manure packing, and liquid storage of dairy manure. Appl. Environ. Microbiol. 72, 565–574. doi: 10.1128/AEM.72.1.565-574.2006
Hamelin, L., Naroznova, I., and Wenzel, H. (2014). Environmental consequences of different carbon alternatives for increased manure-based biogas. Appl. Energy 114, 774–782. doi: 10.1016/j.apenergy.2013.09.033
Hejnfelt, A., and Angelidaki, I. (2009). Anaerobic digestion of slaughterhouse by-products. Biomass Bioenergy 33, 1046–1054. doi: 10.1016/j.biombioe.2009.03.004
Hutchison, M. L., Walters, L. D., Moore, A., Crookes, K. M., and Avery, S. M. (2004). Effect of length of time before incorporation on survival of pathogenic bacteria present in livestock wastes applied to agricultural soil. Appl. Environ. Microbiol. 70, 5111–5118. doi: 10.1128/AEM.70.9.5111-5118.2004
Jiang, Y., Dennehy, C., Lawlor, P. G., Hu, Z., Zhan, X., and Gardiner, G. E. (2018). Inactivation of enteric indicator bacteria and system stability during dry co-digestion of food waste and pig manure. Science of The Total Environment 612, 293–302. doi: 10.1016/j.scitotenv.2017.08.214
Kai, P., Pedersen, P., Jensen, J. E., Hansen, M. N., and Sommer, S. G. (2008). A whole-farm assessment of the efficacy of slurry acidification in reducing ammonia emissions. European Journal of Agronomy 28, 148–154. doi: 10.1016/j.eja.2007.06.004
Kearney, T. E., Larkin, M. J., Frost, J. P., and Levett, P. N. (1993). Survival of pathogenic bacteria during mesophilic anaerobic digestion of animal waste. J. Appl. Bacteriol. 75, 215–219. doi: 10.1111/j.1365-2672.1993.tb02768.x
Kudva, I. T., Blanch, K., and Hovde, C. J. (1998). Analysis of Escherichia coli O157:H7 survival in ovine or bovine manure and manure slurry. Appl. Environ. Microbiol. 64, 3166–74.
Larsen, H. E., Munch, B., and Schlundt, J. (1994). Use of indicators for monitoring the reduction of pathogens in animal waste treated in biogas plants. Int. J. Hygiene Environ. Med. 195, 544–555.
Long, J. H., Aziz, T. N., Reyes, F. L., and de los Ducoste, J. J. (2012). Anaerobic co-digestion of fat, oil, and grease (FOG): a review of gas production and process limitations. Proc. Saf. Environ. Prot. 90, 231–245. doi: 10.1016/J.PSEP.2011.10.001
Luste, S., Heinonen-Tanski, H., and Luostarinen, S. (2012). Co-digestion of dairy cattle slurry and industrial meat-processing by-products – Effect of ultrasound and hygienization pre-treatments. Bioresour. Technol. 104, 195–201. doi: 10.1016/j.biortech.2011.11.003
Luste, S., and Luostarinen, S. (2010). Anaerobic co-digestion of meat-processing by-products and sewage sludge - effect of hygienization and organic loading rate. Bioresour. Technol. 101, 2657–2664. doi: 10.1016/j.biortech.2009.10.071
Luste, S., Luostarinen, S., and Sillanpää, M. (2009). Effect of pre-treatments on hydrolysis and methane production potentials of by-products from meat-processing industry. J. Hazard. Mater. 164, 247–255. doi: 10.1016/j.jhazmat.2008.08.002
Massé, D. I., Croteau, F., and Masse, L. (2007). The fate of crop nutrients during digestion of swine manure in psychrophilic anaerobic sequencing batch reactors. Bioresour. Technol. 98, 2819–2823. doi: 10.1016/j.biortech.2006.07.040
Massé, D. I., Droste, R. L., Kennedy, K. J., Patni, N. K., and Munroe, J. A. (1997). Potential for the psychrophilic anaerobic treatment of swine manure using a sequencing batch reactor 39, 25–33.
Massé, D. I., Talbot, G., and Gilbert, Y. (2011). On farm biogas production: a method to reduce GHG emissions and develop more sustainable livestock operations. Anim. Feed Sci. Technol. 166–167, 436–445. doi: 10.1016/j.anifeedsci.2011.04.075
Mc Carthy, G., Lawlor, P. G., Coffey, L., Nolan, T., Gutierrez, M., and Gardiner, G. E. (2011). An assessment of pathogen removal during composting of the separated solid fraction of pig manure. Bioresour. Technol. 102, 9059–9067. doi: 10.1016/j.biortech.2011.07.021
Millner, P. D. (2009). Bioaerosols associated with animal production operations. Bioresour. Technol. 100, 5379–5385. doi: 10.1016/j.biortech.2009.03.026
Millner, P., Ingram, D., Mulbry, W., and Arikan, O. A. (2014). Pathogen reduction in minimally managed composting of bovine manure. Waste Manag. 34, 1992–1999. doi: 10.1016/j.wasman.2014.07.021
Møller, H. B., Sommer, S. G., and Ahring, B. K. (2004). Methane productivity of manure, straw and solid fractions of manure. Biomass Bioenergy 26, 485–495. doi: 10.1016/j.biombioe.2003.08.008
Monteny, G.-J., Bannink, A., and Chadwick, D. (2006). Greenhouse gas abatement strategies for animal husbandry. Agric. Ecosyst. Environ. 112, 163–170. doi: 10.1016/j.agee.2005.08.015
Moset, V., Fontaine, D., and Møller, H. B. (2017). Co-digestion of cattle manure and grass harvested with different technologies. Effect on methane yield, digestate composition and energy balance. Energy 141, 451–460. doi: 10.1016/j.energy.2017.08.068
Neshat, S. A., Mohammadi, M., Najafpour, G. D., and Lahijani, P. (2017). Anaerobic co-digestion of animal manures and lignocellulosic residues as a potent approach for sustainable biogas production. Renew. Sust. Energ. Rev. 79, 308–322. doi: 10.1016/j.rser.2017.05.137
Neves, L., Oliveira, R., and Alves, M. M. (2009). Fate of LCFA in the co-digestion of cow manure, food waste and discontinuous addition of oil. Water Res. 43, 5142–5150. doi: 10.1016/j.watres.2009.08.013
Nicholson, F. A., Chambers, B. J., Moore, A., Nicholson, R. J., and Hickman, G. (2004). Assessing and managing the risks of pathogen transfer from livestock manures into the food chain. Water Environ. J. 18, 155–160. doi: 10.1111/j.1747-6593.2004.tb00518.x
Nicholson, F. A., Groves, S. J., and Chambers, B. J. (2005). Pathogen survival during livestock manure storage and following land application. Bioresour. Technol. 96, 135–143. doi: 10.1016/j.biortech.2004.02.030
Olsen, E. J., and Larsen, E. H. (1987). Bacterial decimation times in anaerobic digestions of animal slurries. Biol. Wastes 21, 153–168. doi: 10.1016/0269-7483(87)90121-2
Olson, M. E., O'Handley, R. M., Ralston, B. J., McAllister, T. A., and Andrew Thompson, R. (2004). Update on Cryptosporidium and Giardia infections in cattle. Trends Parasitol. 20, 185–191. doi: 10.1016/j.pt.2004.01.015
Orzi, V., Scaglia, B., Lonati, S., Riva, C., Boccasile, G., Alborali, G. L., et al. (2015). The role of biological processes in reducing both odor impact and pathogen content during mesophilic anaerobic digestion. Sci. Total Environ. 526, 116–126. doi: 10.1016/j.scitotenv.2015.04.038
Paavola, T., Syväsalo, E., and Rintala, J. (2006). Co-digestion of manure and biowaste according to the EC Animal By-Products Regulation and Finnish national regulations. Water Sci. Technol. 53, 223–231. doi: 10.2166/wst.2006.253
Petersen, S. O., Andersen, A. J., and Eriksen, J. (2012). Effects of cattle slurry acidification on ammonia and methane evolution during storage. J. Environ. Qual. 41, 88. doi: 10.2134/jeq2011.0184
Peyton, D. P., Healy, M. G., Fleming, G. T. A., Grant, J., Wall, D., Morrison, L. O., et al. (2016). Nutrient, metal and microbial loss in surface runoff following treated sludge and dairy cattle slurry application to an Irish grassland soil. Sci. Total Environ. 541, 218–229. doi: 10.1016/j.scitotenv.2015.09.053
Rao, J. R., Watabe, M., Stewart, T. A., Millar, B. C., and Moore, J. E. (2007). Pelleted organo-mineral fertilisers from composted pig slurry solids, animal wastes and spent mushroom compost for amenity grasslands. Waste Manag. 27, 1117–1128. doi: 10.1016/j.wasman.2006.06.010
Ros, M., García, C., and Hernández, T. (2006). A full-scale study of treatment of pig slurry by composting: kinetic changes in chemical and microbial properties. Waste Manag. 26, 1108–1118. doi: 10.1016/j.wasman.2005.08.008
Sahlström, L. (2003). A review of survival of pathogenic bacteria in organic waste used in biogas plants. Bioresour. Technol. 87, 161–166. doi: 10.1016/S0960-8524(02)00168-2
Stan Development Team. (2017). RStan: The R Interface to Stan. R Package Version 2.16.2. Available online at: http://mc-stan.org
Standing Committee of Analysts. (1985). Chemical Oxygen Demand: Methods for the Examination of Waters and Associated Materials. 2nd Edn. Her London: Majesty's Stationary Office.
Smith, S. R., Lang, N. L., Cheung, K. H. M., and Spanoudaki, K. (2005). Factors controlling pathogen destruction during anaerobic digestion of biowastes. Waste Manag. 25, 417–425. doi: 10.1016/j.wasman.2005.02.010
Venglovsky, J., Sasakova, N., and Placha, I. (2009). Pathogens and antibiotic residues in animal manures and hygienic and ecological risks related to subsequent land application. Bioresour. Technol. 100, 5386–5391. doi: 10.1016/j.biortech.2009.03.068
Vinnerås, B. (2007). Comparison of composting, storage and urea treatment for sanitising of faecal matter and manure. Bioresour. Technol. 98, 3317–3321. doi: 10.1016/j.biortech.2006.07.011
Wagner, A. O., Gstraunthaler, G., and Illmer, P. (2008). Survival of bacterial pathogens during the thermophilic anaerobic digestion of biowaste: laboratory experiments and in situ validation. Anaerobe 14, 181–183. doi: 10.1016/j.anaerobe.2008.03.004
Wallace, T., Gibbons, D., O'Dwyer, M., and Curran, T. P. (2017). International evolution of fat, oil and grease (FOG) waste management – A review. J. Environ. Manage. 187, 424–435. doi: 10.1016/j.jenvman.2016.11.003
Ware, A., and Power, N. (2016). What is the effect of mandatory pasteurization on the biogas transformation of solid slaughterhouse wastes? Waste Manag. 48, 503–512. doi: 10.1016/j.wasman.2015.10.013
Watcharasukarn, M., Kaparaju, P., Steyer, J.-P., Krogfelt, K. A., and Angelidaki, I. (2009). Screening Escherichia coli, Enterococcus faecalis, and Clostridium perfringens as indicator organisms in evaluating pathogen-reducing capacity in biogas plants. Microb. Ecol. 58, 221–230. doi: 10.1007/s00248-009-9497-9
Webb, J., Pain, B., Bittman, S., and Morgan, J. (2010). The impacts of manure application methods on emissions of ammonia, nitrous oxide and on crop response—A review. Agriculture, Ecosystems and Environment 137, 39–46. doi: 10.1016/j.agee.2010.01.001
Keywords: anaerobic digestion, fats, oils and grease, fecal indicator bacteria survival, miniature bioreactors, slurry
Citation: Nolan S, Waters NR, Brennan F, Auer A, Fenton O, Richards K, Bolton DJ, Pritchard L, O'Flaherty V and Abram F (2018) Toward Assessing Farm-Based Anaerobic Digestate Public Health Risks: Comparative Investigation With Slurry, Effect of Pasteurization Treatments, and Use of Miniature Bioreactors as Proxies for Pathogen Spiking Trials. Front. Sustain. Food Syst. 2:41. doi: 10.3389/fsufs.2018.00041
Received: 08 May 2018; Accepted: 29 June 2018;
Published: 20 July 2018.
Edited by:
Tom Misselbrook, Rothamsted Research (BBSRC), United KingdomReviewed by:
Jaak Truu, University of Tartu, EstoniaClinton D. Church, Pasture Systems and Watershed Management Research Unit (USDA-ARS), United States
Copyright © 2018 Nolan, Waters, Brennan, Auer, Fenton, Richards, Bolton, Pritchard, O'Flaherty and Abram. This is an open-access article distributed under the terms of the Creative Commons Attribution License (CC BY). The use, distribution or reproduction in other forums is permitted, provided the original author(s) and the copyright owner(s) are credited and that the original publication in this journal is cited, in accordance with accepted academic practice. No use, distribution or reproduction is permitted which does not comply with these terms.
*Correspondence: Florence Abram, florence.abram@nuigalway.ie