- Urban Horticulture Institute, Horticulture Section, School of Integrative Plant Science, Cornell University, Ithaca, NY, United States
Urban green infrastructure includes both natural inputs and artificial supplements, including irrigation, synthetic substrates, and drainage layers. Green infrastructure aims to make cities more resilient and less dependent on outside resource inputs through more efficient use. Over the past 2 decades, these constructed ecosystems have expanded to include green roofs, elevated urban parks, and rooftop vegetable farms. This paper outlines opportunities and challenges for advancing the science of these constructed ecosystems with particular emphasis on rooftop agriculture. Although in concept rooftop agriculture could contribute to urban food security, water management, and biodiversity, research comparing design and management strategies across climate zones and regional economies is necessary to fully integrate ecological understanding into urban planning policy.
Introduction
Cities are recognized as having environmental footprints extending far beyond their political borders, consuming resources and producing wastes in ways that can globally impact nature and human well-being (Vitousek et al., 1997; Alberti et al., 2003; Grimm et al., 2008). Beginning in the 1990s, this recognition has led to studies of urban biogeochemical cycles, while practices of urban planning and design have applied this knowledge, and explored diverse options for restoring ecological functionality to the built environment (Palmer et al., 2004; Kennedy et al., 2011; Pataki et al., 2011; Pickett et al., 2011). For example, horticultural technologies support the establishment and maintenance of soil-plant systems, such as green roofs, bio-retention basins, and other green spaces constructed on built surfaces, including roofs, pavements, and street-level portions of underground structures (Dunnett and Hitchmough, 2004; Dunnett and Clayden, 2007; Oberndorfer et al., 2007; Driscoll et al., 2015; Lundholm, 2015).
Major strides have been made in the practice of growing drought-tolerant succulents, grasses, and shrubs by using synthetic substrates on top of built surfaces with little or no supplemental irrigation and nutrients (Figure 1A) (Dunnett and Hitchmough, 2004; Dunnett and Kingsbury, 2008; Dunnett et al., 2008; Dvorak and Volder, 2010; MacIvor and Lundholm, 2011; Kotsiris et al., 2012; Nektarios et al., 2012, 2014, 2015; Ntoulas et al., 2013b; Van Mechelen et al., 2015). Design approaches have even expanded to include large-scale (e.g., > 2 ha) elevated urban parks (Figure 1B) and rooftop agriculture (Figure 1C) (Harada et al., 2017; Houston and Zuñiga, 2019). Since the late 1990's, these constructed ecosystems have become integral components of urban “green infrastructure” projects (Lundholm, 2015). The diverse goals of these green infrastructure projects include stormwater management, energy savings, biodiversity restoration, air pollution abatement, crop production, and recycling food waste through composting (Oberndorfer et al., 2007; Berndtsson, 2010; Rowe, 2011; Ahern et al., 2014). There are growing bodies of research relevant to performance measurements and improvements of constructed ecosystems in the fields of plant and soil science, hydrology, and biogeochemistry, while further research is needed for developing best management practices that directly inform urban planning and policy (Pataki et al., 2011; Driscoll et al., 2015; Pataki, 2015).
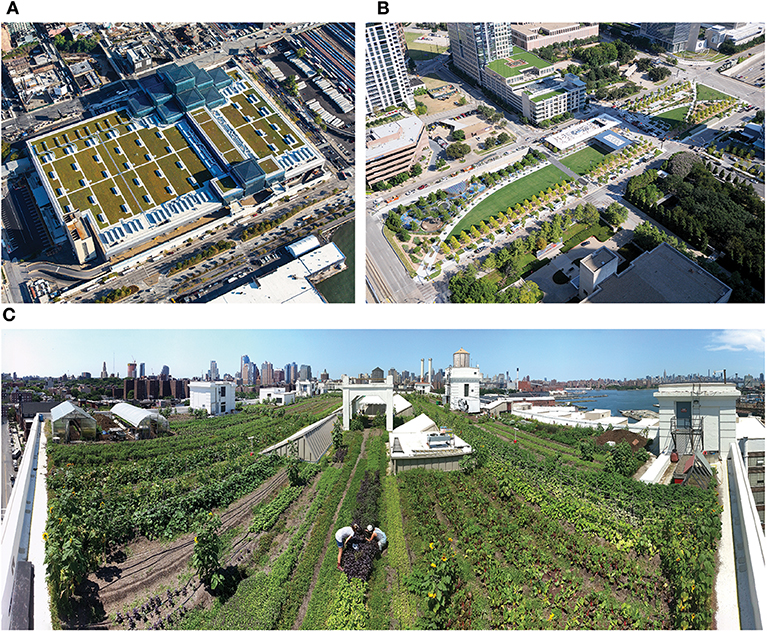
Figure 1. Examples of constructed ecosystems on built surfaces. (A) A 2.7-hectare green roof growing drought-tolerant sedum species on the Javits Center in New York City, New York (Image © Javits Center). (B) Klyde Warren Park: a 2.1-hectar public park, growing trees, shrubs, lawns, and ornamental herbs on the capping structure over Woodall Rodgers Freeway in Dallas, Texas (Image © OJB Landscape Architecture). (C) The Brooklyn Grange: a 0.6-hectar rooftop farm growing vegetables on top of an 11-story building in the former Brooklyn Navy Yard in New York City, New York.
Novel Ecosystems
An overarching framework of ecological communities represented in green infrastructure is useful for delineating and understanding constructed urban ecosystems. In terms of the intensity of ecosystem modification, urban green infrastructure projects range from remnant natural ecosystems to entirely constructed ecosystems (Figure 2). Urban ecosystems are often described as “novel ecosystems” which have no analog in the non-urban environments traditionally studied in the field of ecology (Hobbs et al., 2006; Kowarik, 2011; Perring et al., 2013). For example, remnant natural ecosystems in the urban environment can have distinct species composition and dynamics as the result of unintentional human influence such as the legacy of industrial activities (Kowarik, 2005, 2011). Although the difference between novel and constructed ecosystems is still debated, the concept of novel ecosystem has been used to describe constructed ecosystems as the direct outcome of urban planning and design (Lundholm, 2015; Ahern, 2016; Higgs, 2017). For example, biogeochemical properties of constructed ecosystems are engineered using artificial components such as synthetic substrates, drainage layers, and water-proofing membranes, which alter ecological processes such as movements of water and nutrients across spatiotemporal scales (Berndtsson, 2010; Pataki et al., 2011; Rowe et al., 2014; Fassman-Beck et al., 2015; Harada et al., 2018a,b). These artificial components are studied from various points of view in the disciplines of horticulture, controlled-environment agriculture, and civil and environmental engineering, all of which need to be included in collaborative research if the goal is increased understanding and improved performance of constructed ecosystems (Ampim et al., 2010; Sloan et al., 2012; Harada et al., 2017).
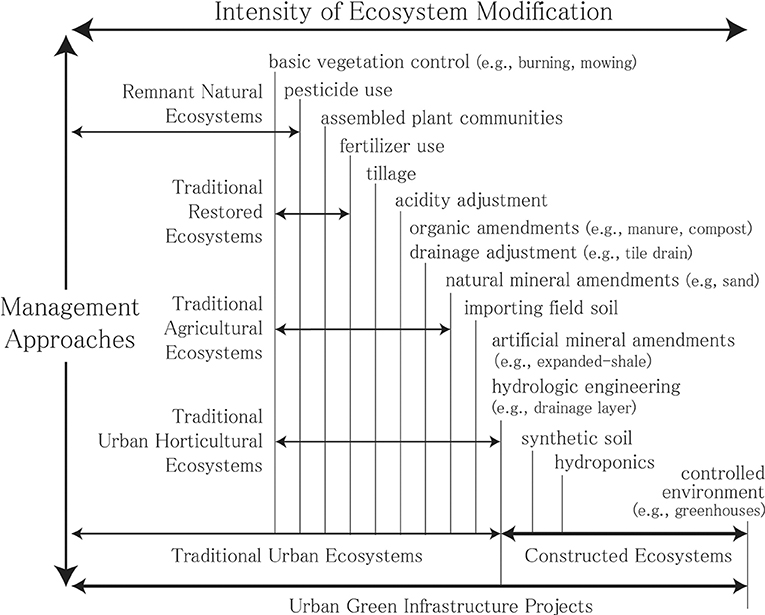
Figure 2. Types of Urban Ecosystems and Intensity of Ecosystem Modification. (adapted from Figure 2 in Sloan et al., 2012).
Advancing Urban Ecology Through Constructed Ecosystems
Planning and design of constructed ecosystems could offer opportunities for advancing the “ecology of cities” which is the science of coupled human-natural systems in the urban environment (Grimm et al., 2000; Pickett et al., 2001; McPhearson et al., 2016). In green infrastructure projects, design strategies intended to assure specific ecological processes and environmental goals often lack evidence. For example, green roofs and rooftop agriculture intended to reduce the nutrient load in runoff may in fact increase the load due to fertilizer application, because the present state of knowledge and technology are insufficient to allow precision nutrient management (Driscoll et al., 2015; Harada et al., 2018a). Socio-ecological assumptions are often implicit in constructed ecosystems, which could drive interdisciplinary studies of feedback loops between society, science, and transformation of the urban environments (Tanner et al., 2014; McPhearson et al., 2016). “Adaptive experiments” or “designed experiments” are among the approaches of embedding experiments and applying science to urban ecosystems by involving ecologists in design and management practices from the onset (Cook et al., 2004; Felson and Pickett, 2005; Kotsiris et al., 2013; Ntoulas et al., 2013a; Ahern et al., 2014). Constructed ecosystems are ideal for collaborative ecological research because components of constructed ecosystems such as plant selection, substrate properties, and drainage systems can be experimentally manipulated and replicated through design and management practices (Felson and Pickett, 2005; Felson et al., 2013). Design constraints could also be an advantage for experiments. For example, engineers are often constrained by abrupt hydrologic boundaries, shallow substrates, and centralized drainage systems because of budget limitations, regulations, professional guidelines, and load bearing capacity of buildings and underground structures. In such simple and discreet hydrological systems, inputs and drainage losses of water and nutrients can be studied most completely as in the forested catchments in the Hubbard Brook Long-Term Ecological Research (Likens, 2013). This approach would serve as a foundation for pursuing precise water and nutrient management in constructed ecosystems, which reduces the drainage loss of water and nutrients, while maintaining satisfactory crop yield and quality (Harada et al., 2017). Water and nutrient management is important for the practices of rooftop farming.
Integration of Rooftop Intensive Agriculture in Urban Ecosystems
Rooftop Farming
Urban agriculture is a growing movement which aims to address the diverse goals of urban sustainability, including food security, food equity, efficient food supply chains, stormwater management, mitigation of urban heat island effects, and waste management using compostable waste (Brown and Jameton, 2000; Brown and Bailkey, 2002; Mougeot, 2006; Lovell, 2010; Lovell and Taylor, 2013; Ackerman et al., 2014; Russo et al., 2017). In economically developed countries, urban planning practices treated agriculture as a temporary activity for vacant lots before conversion to more profitable residential, commercial, and industrial land uses (Alonso, 1964; Van Veenhuizen and Danso, 2007). However, urban agriculture is becoming a long-term enterprise by increasing and stabilizing profits through (1) intensive production of fresh and perishable vegetables that have high long distance transportation costs from rural farms; (2) unique marketing strategies such as organic cultivation and production of heirloom and exotic varieties; (3) diversifying crop selection, and/or (4) incorporating non-cropping services such as tourism, environmental education, green job training, culinary events, nature therapy, and creation of lively neighborhood (van der Schans and Wiskerke, 2012; Plakias, 2016; Pölling et al., 2016, 2017).
Limited space and competitive real-estate markets are impediments for in-ground agriculture, while farms retrofitted to roofs occupy otherwise underutilized space in the built environment (Specht et al., 2014; Thomaier et al., 2015; Whittinghill and Starry, 2016). New York City alone has 15,482 ha of rooftop surface, equal to 445 times the size of existing community gardens (Ackerman et al., 2013). Converting even a small portion of this space to agriculture presents important opportunities for advancing urban agriculture.
In addition to private investments, rooftop farming can combine policy supports and public funding from green building and green infrastructure initiatives. Since 2011, for example, Community-Based Green Infrastructure Program of NYC Department of Environmental Protection provides grants for construction of green infrastructure projects including rooftop farms as a part of 20-year green infrastructure masterplan (NYC DEP, 2010, 2011), while since 2013, NYC's new zoning code, “Zone Green,” allows modification of buildings for enhancing urban sustainability, including the construction of rooftop farms (NYC DCP, 2012). One of the outcomes is the Brooklyn Grange, a 0.6 ha intensive vegetable production farm atop an 11-story building in the former Brooklyn Navy Yard, NYC (Figure 1C). The Brooklyn Grange yields 11,000- 13,000 metric tons year−1 of organic vegetables, while expanding relationships with local residents, schools, restaurant owners, and non-profit organizations through vegetable sales, green job training, environmental education, and waste collection for composting (Plakias, 2016; Harada et al., 2018a).
Rooftop farming could enjoy further opportunities through the NYC's new green building policy known as “Climate Mobilization Act,” which takes effect in 2024, mandating improvements in building energy performance including vegetated roofs (New York City Council, 2019). However, the scientific community has little hard data on the environmental and economic performance of rooftop farming. Empirical studies of water and nutrient budgets for operational rooftop farms could serve as a starting point for understanding and improving the performance of rooftop farming.
Hypothetical City-Scale Effects
Among the studies of rooftop farming using experimental systems, city-scale production capacity of rooftop farming was estimated only once (Orsini et al., 2014). They report that outdoor hydroponic systems (57% of site area) and planters using synthetic substrates (43% of site area) could maximize the yield of lettuce (Lactuca sativa L.), black cabbage (Brassica oleracea Acephala Group), chicory (Cichorium intybus L.), tomato (Solanum lycopersicum L.), eggplant (Solanum melongena L.), chili pepper (Capsicum annum L.), cantaloupe (Cucumis melo L.), and watermelon (Citrullus lanatus Thumb.) in the flat roof areas (82 ha) of Bologna, Italy, thereby providing 77% of the city's fresh vegetable demand (16,169 metric tons year−1). The study did not estimate city-scale environmental impacts, such as potable water consumption for irrigation, fertilizer input, and drainage loss of water and nutrients (Orsini et al., 2014). Although measurements from experimental systems provide useful insights for understanding the performance of rooftop farming, it is important to study operational rooftop farms because efficiency of water and nutrient management is often higher in small-scale well-managed experimental plots than that in operational farms (see Cassman et al., 2002).
Among the studies of operational rooftop farms, only Harada et al. (2018a,b) report the budget of water and nitrogen of the Brooklyn Grange Navy Yard Farm in NYC, which can be used for estimating the city-scale effect of rooftop farming as summarized in Supplementary Table S1, S2. If all suitable rooftops in NYC (1,246 ha) were occupied by rooftop farms like the Brooklyn Grange, the city-scale potable water consumption and nitrogen discharge from wastewater treatment plants to surface water could increase by 0.3 and 0.6%, respectively, while producing 27,344 metric tons year−1 of fresh vegetables, equivalent to fresh vegetable consumption of 3.8 × 105 people, or 4% of the estimated city-scale vegetable consumption (Supplementary Table S1, S2). Leafy vegetables are one of the most important fresh vegetables grown in urban agriculture including rooftop farming (Ackerman et al., 2013; Baudoin et al., 2017; Harada et al., 2018a), and city-scale demand of greens mix (leafy lettuce and mustard greens) is largest among leafy vegetables grown at the Brooklyn Grange (Supplementary Table S2). If all cropped area were dedicated to mixed greens, then the city-scale rooftop farming could produce 38% of the NYC's demand (Supplementary Table S2).
Although the NYC's city-scale suitable rooftop area was 15 times of that in Orsini et al. (2014), fresh vegetable production was only 1.1 times of that in Orsini et al. (2014) due to the relatively low yield at the Brooklyn Grange. Average yield per unit are of the entire roof (including both cropped and uncropped areas) at the Brooklyn Grange is only 14% of that reported by Orsini et al. (2014) (15.2 kg m−2 year−1), in part, because the growing season was longer (year-around) in Bologna than at the Brooklyn Grange (226 days). Also, the percentage of cropped area in the entire roof is smaller at the Brooklyn Grange (47%) than that reported by Orsini et al. (2014) (65%), while even when the uncropped area is excluded, the average yield at the Brooklyn Grange is only 20% of that reported by Orsini et al. (2014). Other factors for relatively low yield at the Brooklyn Grange include crop selection, design of the production system, and the less intensive management in larger operational farms than that in small experimental plots.
Biodiversity Conservation
Within the context of city-scale ecosystems, isolated patches of urban green space, including urban agriculture and green roofs, can be hotspots for biodiversity (Cook-Patton and Bauerle, 2012; Forman, 2014; Williams et al., 2014; Borysiak et al., 2017; Lepczyk et al., 2017). Declining wildlife populations in farmland and rural areas due to pesticide use increased the importance of cities as wildlife refuge, while organic cultivation in rooftop farming can increase plant, insect, and bird habitat in densely built environments and contribute to urban corridor networks (Gilbert, 1989; Chamberlain et al., 2000; Orsini et al., 2014; Bretzel et al., 2017; Dang, 2017; Hall et al., 2017). Insect pollinators are important indicators of biodiversity across land uses including urban environments, while beekeeping contributes directly to food production (Tommasi et al., 2004; Broadway, 2009; Plakias, 2016; Bretzel et al., 2017; Hall et al., 2017). Studies of green roofs report that management, plant selection, and substrate properties have strong influence on species composition and abundance for wild flora, birds, invertebrates, and the substrate microbial community, emphasizing the need of empirical studies specific to rooftop farming (Dunnett et al., 2008; Dvorak and Volder, 2010; Fernández Cañero and González Redondo, 2010; McGuire et al., 2013, 2015; Williams et al., 2014; MacIvor and Ksiazek, 2015; Bretzel et al., 2017; Ksiazek et al., 2018; Aloisio et al., 2019).
Stormwater Management
Estimated city-scale evapotranspiration (ET) summarized in Supplementary Table S1 indicates that the potential of stormwater retention by rooftop farming, equals 2.3 X that of the urban forest in NYC. The Brooklyn Grange uses a synthetic substrate for growing vegetables, while the base material of the substrate is heat-expanded shale, which can increase drainage by lowering water-holding capacity of the substrate (Rowe et al., 2014; Ntoulas et al., 2015; Harada et al., 2018b). Although the Brooklyn Grange retained little stormwater during the growing seasons, it should be noted that precipitation exceeded ET at the Brooklyn Grange, which means that stormwater discharge and irrigation demands could be eliminated while maintaining satisfactory yield through enhanced water-holding capacity of the substrate and recirculating drainage (Harada et al., 2018b). Outdoor hydroponic systems can also be used for rooftop farming, which could eliminate drainage discharge by using closed-circuit systems for nutrient solution, and incorporating stormwater recycle systems (Orsini et al., 2014; Sanyé-Mengual et al., 2015b; Rodríguez-Delfín et al., 2017; Tsirogiannis et al., 2017). However, water management using electric pumps requires further research because pumps' electricity consumption can be the largest environmental cost of rooftop farming (Sanyé-Mengual et al., 2015b).
Rooftop Greenhouses
Another option for rooftop intensive agriculture is rooftop greenhouses which could achieve higher levels of yield, water use efficiency, and stormwater retention than those of rooftop farming. For example, Sanjuan-Delmás et al. (2018) report the tomato yield of 19.6 kg m−2 year−1 in rooftop greenhouse in the city near Barcelona, Spain, which exceeds yields of rooftop farms (5.1–14.3 kg m−2 year−1) (Orsini et al., 2014; Grard et al., 2015; Harada et al., 2018a; Boneta et al., 2019). Rainwater was collected from roofs of the greenhouse and adjacent building, contributing to 80–90% of total water supply. While nutrient discharge exceeded that of a conventional greenhouse, this could be reduced by using a closed-circuit hydroponic system (Sanjuan-Delmás et al., 2018).
However, construction costs for rooftop greenhouse (299–764 USD m−2) can be higher than those for commercial rooftop farming (54–150 USD m−2) (calculated as 1 Euro = 1.12 USD) (Mandel, 2013; Sanyé-Mengual et al., 2015a; Proksch, 2016). Furthermore, it could be a challenge for rooftop greenhouses to compete with conventional greenhouses in terms of economic and environmental returns. For example, Sanyé-Mengual et al. (2015a) estimated that life-cycle production cost of rooftop greenhouses for tomato is 2.8 times that of conventional greenhouse (a steel-framed high tunnel with vertical sidewalls), requiring yield of 55 kg m−2 year−1 for rooftop greenhouses to achieve higher economic and environmental returns than those of conventional greenhouse in Barcelona, Spain.
In terms of life-cycle costs of vegetable production, greenhouse cropping systems could be superior to outdoor agriculture in arid and cold regions that have high irrigation demands and short growing seasons, while life-cycle costs specific to rooftop greenhouses highly depend on regional economy, design, microclimate, and distance to major agricultural areas, which require further research (Cuéllar and Webber, 2010; Barbosa et al., 2015; Sanyé-Mengual et al., 2015a; Van Ginkel et al., 2017). While providing opportunities for sustainable food production, rooftop greenhouses do not contribute to habitat creation and environmental education relevant to wildlife biodiversity.
Conclusions
Constructed urban ecosystems can be different from traditional subjects of ecology in terms of environmental and economic performance, but they are direct outcomes of urban planning and design intentions, which are important and testable subjects for understanding and improving coupled human-natural systems. Among those constructed ecosystems are rooftop intensive vegetable production systems aiming to achieve diverse goals of sustainability within the practices of urban green infrastructure projects, while the scientific community has little information for instructing and navigating urban planning and design. Future research could be motivated by the following questions:
(1) What are the optimal levels of yield, water and nutrient use efficiency, and stormwater management for balancing environmental and economic costs of rooftop intensive agriculture?
(2) How do economic and environmental returns of rooftop intensive agriculture differ in specific system design, climate zone, regional economy, and distance to major agricultural areas?
(3) What are the specific contributions of rooftop farming to enhancing urban biodiversity?
(4) What are the best ways of involving scientists and designing experiments in rooftop intensive agriculture for understanding and improving social ecological systems?
(5) How could urban planning and design integrate different options of rooftop intensive agriculture and other green spaces for achieving diverse goals of urban sustainability?
Data Availability Statement
All datasets generated for this study are included in the article/Supplementary Material.
Author Contributions
YH: experimental design, sample collection, sample analyses, data analyses, and manuscript preparation. TW: experimental design and manuscript preparation.
Conflict of Interest
The authors declare that the research was conducted in the absence of any commercial or financial relationships that could be construed as a potential conflict of interest.
Acknowledgments
This study was based on the United States Department of Agriculture (USDA) Hatch Grant (1001972), Sustainable Agriculture Research and Education (SARE) Partnership Grant Projects (ONE16-276), and the Toward Sustainability Foundation.
Supplementary Material
The Supplementary Material for this article can be found online at: https://www.frontiersin.org/articles/10.3389/fsufs.2020.00076/full#supplementary-material
References
Ackerman, K., Conard, M., Culligan, P., Plunz, R., Sutto, M-P., and Whittinghill, L. (2014). Sustainable food systems for future cities: the potential of urban agriculture. Econ. Soc. Rev. 45, 189–206. Available online at: https://www.esr.ie/article/view/136
Ackerman, K., Dahlgren, E., and Xu, X. (2013). Sustainable Urban Agriculture: Confirming Viable Scenarios for Production. New York State Energy Research and Development Authority [NYSERDA], Final Report No.13-07.
Ahern, J. (2016). Novel urban ecosystems: concepts, definitions and a strategy to support urban sustainability and resilience. Landsc. Arch. Front. 4, 10–22. Available online at: http://journal.hep.com.cn/laf/EN/Y2016/V4/I1/10
Ahern, J., Cilliers, S., and Niemelä, J. (2014). The concept of ecosystem services in adaptive urban planning and design: a framework for supporting innovation. Landsc. Urban Plan. 125, 254–259. doi: 10.1016/j.landurbplan.2014.01.020
Alberti, M., Marzluff, J. M., Shulenberger, E., Bradley, G., Ryan, C., and Zumbrunnen, C. (2003). Integrating humans into ecology: opportunities and challenges for studying urban ecosystems. BioScience 53, 1169–1179. doi: 10.1641/0006-356820030531169:IHIEOA2.0.CO;2
Aloisio, J. M., Palmer, M. I., Tuininga, A. R., and Lewis, J. D. (2019). Plant colonization of green roofs is affected by composition of established native plant communities. Front. Ecol. Evol. 6:238. doi: 10.3389/fevo.2018.00238
Alonso, W. (1964). Location and Land Use. Toward a General Theory of Land Rent. (Cambridge : Harvard University Press).
Ampim, P. A., Sloan, J. J., Cabrera, R. I., Harp, D. A., and Jaber, F. H. (2010). Green roof growing substrates: types, ingredients, composition and properties. J. Environ. Hortic. 28, 244–252. doi: 10.24266/0738-2898-28.4.244
Barbosa, G., Gadelha, F., Kublik, N., Proctor, A., Reichelm, L., Weissinger, E., et al. (2015). Comparison of land, water, and energy requirements of lettuce grown using hydroponic vs. conventional agricultural methods. Int. J. Environ. Res. Public Health 12, 6879–6891. doi: 10.3390/ijerph120606879
Baudoin, W., Desjardins, Y., Dorais, M., Charrondière, U., Herzigova, L., El-Behairy, U., et al. (2017). “Rooftop gardening for improved food and nutrition security in the urban environment,” in Rooftop Urban Agriculture, eds F. Orsini, M. Dubbeling, H. de Zeeuw, and G. Gianquinto (Cham: Springer), 219–233.
Berndtsson, J. C. (2010). Green roof performance towards management of runoff water quantity and quality: a review. Ecol. Eng. 36, 351–360. doi: 10.1016/j.ecoleng.2009.12.014
Boneta, A., Rufí-Salís, M., Ercilla-Montserrat, M., Gabarrell, X., and Rieradevall, J. (2019). Agronomic and environmental assessment of a polyculture rooftop soilless urban home garden in a Mediterranean city. Front. Plant Sci. 10:341. doi: 10.3389/fpls.2019.00341
Borysiak, J., Mizgajski, A., and Speak, A. (2017). Floral biodiversity of allotment gardens and its contribution to urban green infrastructure. Urban Ecosyst. 20, 323–335. doi: 10.1007/s11252-016-0595-4
Bretzel, F., Vannucchi, F., Benvenuti, S., and Rumble, H. (2017). “Biodiversity of flora and fauna,” in Rooftop Urban Agriculture, eds F. Orsini, M. Dubbeling, H. de Zeeuw, and G. Gianquinto (Cham: Springer), 235–252.
Broadway, M. (2009). Growing urban agriculture in North American cities: the example of Milwaukee. Focus Geogr. 52, 23–30. doi: 10.1111/j.1949-8535.2009.tb00251.x
Brown, K. H., and Bailkey, M. (2002). Urban Agriculture and Community Food Security in the United States: Farming From the City Center to the Urban Fringe. Urban Agriculture Committee of the Community Food Security Coalition.
Brown, K. H., and Jameton, A. L. (2000). Public health implications of urban agriculture. J. Public Health Policy 21, 20–39. doi: 10.2307/3343472
Cassman, K. G., Dobermann, A., and Walters, D. T. (2002). Agroecosystems, nitrogen-use efficiency, and nitrogen management. Ambio 31, 132–140. doi: 10.1579/0044-7447-31.2.132
Chamberlain, D. E., Fuller, R. J., Bunce, R. G. H., Duckworth, J. C., and Shrubb, M. J. (2000). Changes in the abundance of farmland birds in relation to the timing of agricultural intensification in England and wales. J. Appl. Ecol. 37, 771–788. doi: 10.1046/j.1365-2664.2000.00548.x
Cook, W. M., Casagrande, D. G., Hope, D., Groffman, P. M., and Collins, S. L. (2004). Learning to roll with the punches: adaptive experimentation in human-dominated systems. Front. Ecol. Environ. 2, 467–474. doi: 10.1890/1540-929520040020467:LTRWTP2.0.CO;2
Cook-Patton, S. C., and Bauerle, T. L. (2012). Potential benefits of plant diversity on vegetated roofs: a literature review. J. Environ. Manage. 106, 85–92. doi: 10.1016/j.jenvman.2012.04.003
Cuéllar, A. D., and Webber, M. E. (2010). Wasted food, wasted energy: the embedded energy in food waste in the United States. Environ. Sci. Technol. 44, 6464–6469. doi: 10.1021/es100310d
Dang, M. (2017). “Designing green corridors network within cities: a case study in vienna,” in Rooftop Urban Agriculture, eds F. Orsini, M. Dubbeling, H. de Zeeuw, and G. Gianquinto (Cham: Springer), 291–306.
Driscoll, C. T., Eger, C. G., Chandler, D. G., Davidson, C. I., Roodsari, B. K., Flynn, C. D., et al. (2015). Green Infrastructure: Lessons from Science and Practice. A Publication of the Science. Policy Exchange.
Dunnett, N., and Clayden, A. (2007). Rain Gardens: Managing Water Sustainably in the Garden and Designed Landscape. Portland, OR: Timber Press.
Dunnett, N., and Hitchmough, J. (2004). The Dynamic Landscape: Design, Ecology and Management of Naturalistic Urban Planting. New York, NY: Taylor & Francis.
Dunnett, N., and Kingsbury, N. (2008). Planting Green Roofs and Living Walls. Portland, OR: Timber press.
Dunnett, N., Nagase, A., and Hallam, A. (2008). The dynamics of planted and colonising species on a green roof over six growing seasons 2001–2006: influence of substrate depth. Urban Ecosyst. 11, 373–384. doi: 10.1007/s11252-007-0042-7
Dvorak, B., and Volder, A. (2010). Green roof vegetation for North American ecoregions: a literature review. Landsc. Urban Plan. 96, 197–213. doi: 10.1016/j.landurbplan.2010.04.009
Fassman-Beck, E., Hunt, W., Berghage, R., Carpenter, D., Kurtz, T., Stovin, V., et al. (2015). Curve number and runoff coefficients for extensive living roofs. J. Hydrol. Eng. 21:04015073. doi: 10.1061/(ASCE)HE.1943-5584.0001318
Felson, A. J., Oldfield, E. E., and Bradford, M. A. (2013). Involving ecologists in shaping large-scale green infrastructure projects. Bioscience 63, 882–890. doi: 10.1525/bio.2013.63.11.7
Felson, A. J., and Pickett, S. T. A. (2005). Designed experiments: new approaches to studying urban ecosystems. Front. Ecol. Environ. 3, 549–556. doi: 10.1890/1540-929520050030549:DENATS2.0.CO;2
Fernández Cañero, R., and González Redondo, P. (2010). Green roofs as a habitat for birds: a review. J. Anim. Vet. Adv. 9, 2041–2052. doi: 10.3923/javaa.2010.2041.2052
Grard, B. J-P., Bel, N., Marchal, N., Madre, F., Castell, J-F., Cambier, P., et al. (2015). Recycling urban waste as possible use for rooftop vegetable garden. Fut. Food J. Food Agric. Soc. 3, 21–34. Available online at: http://www.thefutureoffoodjournal.com/index.php/FOFJ/article/view/119
Grimm, N. B., Faeth, S. H., Golubiewski, N. E., Redman, C. L., Wu, J., Bai, X., et al. (2008). Global change and the ecology of cities. Science 319, 756–760. doi: 10.1126/science.1150195
Grimm, N. B., Morgan Grove, J., Pickett, S. T., and Redman, C. L. (2000). Integrated approaches to long-termstudies of urban ecological systems: urban ecological systems present multiple challenges to ecologists—pervasive human impact and extreme heterogeneity of cities, and the need to integrate social and ecological approaches, concepts, and theory. BioScience 50, 571–584. doi: 10.1641/0006-356820000500571:IATLTO2.0.CO;2
Hall, D. M., Camilo, G. R., Tonietto, R. K., Ollerton, J., Ahrné, K., Arduser, M., et al. (2017). The city as a refuge for insect pollinators. Conserv. Biol. 31, 24–29. doi: 10.1111/cobi.12840
Harada, Y., Whitlow, T. H., Bassuk, N. L., and Russell-Anelli, J. (2017). “Biogeochemistry of rooftop farm soils,” in Urban Soils, eds R. Lal and B. A. Stewart (Portland, OR: Taylor & Francis Group), 275–294.
Harada, Y., Whitlow, T. H., Templer, P. H., Howarth, R. W., Walter, M. T., Bassuk, N. L., et al. (2018a). Nitrogen biogeochemistry of an urban rooftop farm. Front. Ecol. Evol. 6:153. doi: 10.3389/fevo.2018.00153
Harada, Y., Whitlow, T. H., Todd Walter, M., Bassuk, N. L., Russell-Anelli, J., and Schindelbeck, R. R. (2018b). Hydrology of the Brooklyn Grange, an urban rooftop farm. Urban Ecosyst. 21, 673–689. doi: 10.1007/s11252-018-0749-7
Hobbs, R. J., Arico, S., Aronson, J., Baron, J. S., Bridgewater, P., Cramer, V. A., et al. (2006). Novel ecosystems: theoretical and management aspects of the new ecological world order. Glob. Ecol. Biogeogr. 15, 1–7. doi: 10.1111/j.1466-822X.2006.00212.x
Houston, D., and Zuñiga, M. E. (2019). Put a park on it: how freeway caps are reconnecting and greening divided cities. Cities 85, 98–109. doi: 10.1016/j.cities.2018.08.007
Kennedy, C., Pincetl, S., and Bunje, P. (2011). The study of urban metabolism and its applications to urban planning and design. Environ. Pollut. 159, 1965–1973. doi: 10.1016/j.envpol.2010.10.022
Kotsiris, G., Nektarios, P. A., Ntoulas, N., and Kargas, G. (2013). An adaptive approach to intensive green roofs in the Mediterranean climatic region. Urban For. Urban Green. 12, 380–392. doi: 10.1016/j.ufug.2013.05.001
Kotsiris, G., Nektarios, P. A., and Paraskevopoulou, A. T. (2012). Lavandula angustifolia growth and physiology is affected by substrate type and depth when grown under Mediterranean semi-intensive green roof conditions. HortScience 47, 311–317. doi: 10.21273/HORTSCI.47.2.311
Kowarik, I. (2005). “Wild urban woodlands: towards a conceptual framework,” in Wild Urban Woodlands, eds I. Kowarik and S. Körner (Berlin; Heidelberg: Springer), 1–32.
Kowarik, I. (2011). Novel urban ecosystems, biodiversity, and conservation. Environ. Pollut. 159, 1974–1983. doi: 10.1016/j.envpol.2011.02.022
Ksiazek, K., Tonietto, R., and Ascher, J. S. (2018). Ten bee species new to green roofs in the Chicago area. Great Lakes Entomol. 47:13. Available online at: https://scholar.valpo.edu/tgle/vol47/iss1/13
Lepczyk, C. A., Aronson, M. F., Evans, K. L., Goddard, M. A., Lerman, S. B., and MacIvor, J. S. (2017). Biodiversity in the city: fundamental questions for understanding the ecology of urban green spaces for biodiversity conservation. BioScience 67, 799–807. doi: 10.1093/biosci/bix079
Likens, G. E. (2013). Biogeochemistry of a Forested Ecosystem. New York, NY: Springer Science & Business Media.
Lovell, S. T. (2010). Multifunctional urban agriculture for sustainable land use planning in the United States. Sustainability 2, 2499–2522. doi: 10.3390/su2082499
Lovell, S. T., and Taylor, J. R. (2013). Supplying urban ecosystem services through multifunctional green infrastructure in the United States. Landsc. Ecol. 28, 1447–1463. doi: 10.1007/s10980-013-9912-y
Lundholm, J. T. (2015). The ecology and evolution of constructed ecosystems as green infrastructure. Front. Ecol. Evol. 3:106. doi: 10.3389/fevo.2015.00106
MacIvor, J. S., and Ksiazek, K. (2015). “Invertebrates on green roofs,” in Green Roof Ecosystems, ed R. Sutton (Cham: Springer), 333–355.
MacIvor, J. S., and Lundholm, J. (2011). Performance evaluation of native plants suited to extensive green roof conditions in a maritime climate. Ecol. Eng. 37, 407–417. doi: 10.1016/j.ecoleng.2010.10.004
Mandel, L. (2013). EAT UP: The Inside Scoop on Rooftop Agriculture. Gabriola, BC: New Society Publishers.
McGuire, K. L., Payne, S. G., Orazi, G., and Palmer, M. I. (2015). “Bacteria and fungi in green roof ecosystems,” in Green Roof Ecosystems, eds R. Sutton (Cham: Springer), 175–191.
McGuire, K. L., Payne, S. G., Palmer, M. I., Gillikin, C. M., Keefe, D., Kim, S. J., et al. (2013). Digging the New York city skyline: soil fungal communities in green roofs and city parks. PLoS ONE 8:e58020. doi: 10.1371/journal.pone.0058020
McPhearson, T., Pickett, S. T., Grimm, N. B., Niemelä, J., Alberti, M., Elmqvist, T., et al. (2016). Advancing urban ecology toward a science of cities. BioScience 66, 198–212. doi: 10.1093/biosci/biw002
Nektarios, P., Ntoulas, N., Kotopoulis, G., Ttoulou, T., and Ilia, P. (2014). Festuca arundinacea drought tolerance and evapotranspiration when grown on two extensive green roof substrate depths and under two irrigation regimes. Eur. J. Hortic. Sci 79, 142–149. Available online at: https://www.pubhort.org/ejhs/2014/file_4341620.pdf
Nektarios, P., Ntoulas, N., and Nydrioti, E. (2012). Turfgrass use on intensive and extensive Green roofs. Acta Hortic. 938, 121–128. doi: 10.17660/ActaHortic.2012.938.15
Nektarios, P. A., Ntoulas, N., Nydrioti, E., Kokkinou, I., Bali, E-M., and Amountzias, I. (2015). Drought stress response of Sedum sediforme grown in extensive green roof systems with different substrate types and depths. Sci. Hortic. 181, 52–61. doi: 10.1016/j.scienta.2014.10.047
New York City Council (2019). Council to Vote on Climate Mobilization Act Ahead of Earth Day. Available online at: https://council.nyc.gov/press/2019/04/18/1730/ (accessed April 30, 2019).
Ntoulas, N., Nektarios, P. A., Charalambous, E., and Psaroulis, A. (2013a). Zoysia matrella cover rate and drought tolerance in adaptive extensive green roof systems. Urban For. Urban Green 12, 522–531. doi: 10.1016/j.ufug.2013.07.006
Ntoulas, N., Nektarios, P. A., Kapsali, T-E., Kaltsidi, M-P., Han, L., and Yin, S. (2015). Determination of the physical, chemical, and hydraulic characteristics of locally available materials for formulating extensive green roof substrates. HortTechnology 25, 774–784. doi: 10.21273/HORTTECH.25.6.774
Ntoulas, N., Nektarios, P. A., and Nydrioti, E. (2013b). Performance of Zoysia matrella ‘Zeon’in shallow green roof substrates under moisture deficit conditions. HortScience 48, 929–937. doi: 10.21273/HORTSCI.48.7.929
NYC DCP (2012). Zone Green Text Amendment. Available online at: http://www1.nyc.gov/assets/planning/download/pdf/plans/zone-green/zone_green.pdf (accessed April 01, 2016).
NYC DEP (2010). NYC Green Infrastructure Plan: A Sustainable Strategy for Clean Waterways. Available online at: http://www.nyc.gov/html/dep/html/stormwater/nyc_green_infrastructure_plan.shtml (accessed April 01, 2016).
NYC DEP (2011). DEP Awards $3.8 Million in Grants for Community-Based Green Infrastructure Program Projects. Available online at: http://www.nyc.gov/html/dep/html/press_releases/11-46pr.shtml#.VvIn6fkrKUk (accessed April 01, 2016).
Oberndorfer, E., Lundholm, J., Bass, B., Coffman, R. R., Doshi, H., Dunnett, N., et al. (2007). Green roofs as urban ecosystems: ecological structures, functions, and services. BioScience 57, 823–833. doi: 10.1641/B571005
Orsini, F., Gasperi, D., Marchetti, L., Piovene, C., Draghetti, S., Ramazzotti, S., et al. (2014). Exploring the production capacity of rooftop gardens (RTGs) in urban agriculture: the potential impact on food and nutrition security, biodiversity and other ecosystem services in the city of Bologna. Food Secur. 6, 781–792. doi: 10.1007/s12571-014-0389-6
Palmer, M., Bernhardt, E., Chornesky, E., Collins, S., Dobson, A., Duke, C., et al. (2004). Ecology for a crowded planet. Science 304, 1251–1252. doi: 10.1126/science.1095780
Pataki, D. E. (2015). Grand challenges in urban ecology. Front. Ecol. Evol. 3:57. doi: 10.3389/fevo.2015.00057
Pataki, D. E., Carreiro, M. M., Cherrier, J., Grulke, N. E., Jennings, V., Pincetl, S., et al. (2011). Coupling biogeochemical cycles in urban environments: ecosystem services, green solutions, and misconceptions. Front. Ecol. Environ. 9, 27–36. doi: 10.1890/090220
Perring, M. P., Manning, P., Hobbs, R. J., Lugo, A. E., Ramalho, C. E., and Standish, R. J. (2013). Novel urban ecosystems and ecosystem services. in Novel Ecosystems: Intervening in the New Ecological World Order, eds R. J. Hobbs, E. S. Higgs, and C. M. Hall (Oxford: Wiley-Blackwell), 310–325. doi: 10.1002/9781118354186.ch38
Pickett, S. T., Cadenasso, M. L., Grove, J. M., Boone, C. G., Groffman, P. M., Irwin, E., et al. (2011). Urban ecological systems: scientific foundations and a decade of progress. J. Environ. Manage. 92, 331–362. doi: 10.1016/j.jenvman.2010.08.022
Pickett, S. T., Cadenasso, M. L., Grove, J. M., Nilon, C. H., Pouyat, R. V., Zipperer, W. C., et al. (2001). Urban ecological systems: linking terrestrial ecological, physical, and socioeconomic components of metropolitan areas. Ann. Rev. Ecol. Syst. 32, 127–157. doi: 10.1146/annurev.ecolsys.32.081501.114012
Plakias, A. C. (2016). The Farm on the Roof: What Brooklyn Grange Taught us About Entrepreneurship, Community, and Growing a Sustainable Business. New York, NY: Penguin Publishing Group.
Pölling, B., Mergenthaler, M., and Lorleberg, W. (2016). Professional urban agriculture and its characteristic business models in Metropolis Ruhr, Germany. Land Use Policy 58, 366–379. doi: 10.1016/j.landusepol.2016.05.036
Pölling, B., Sroka, W., and Mergenthaler, M. (2017). Success of urban farming's city-adjustments and business models—findings from a survey among farmers in Ruhr Metropolis, Germany. Land Use Policy 69, 372–385. doi: 10.1016/j.landusepol.2017.09.034
Proksch, G. (2016). Creating Urban Agricultural Systems: An Integrated Approach to Design. London: Taylor & Francis.
Rodríguez-Delfín, A., Gruda, N., Eigenbrod, C., Orsini, F., and Gianquinto, G. (2017). “Soil based and simplified hydroponics rooftop gardens,” in Rooftop Urban Agriculture, eds F. Orsini, M. Dubbeling, H. de Zeeuw, and G. Gianquinto (Cham: Springer), 61–81.
Rowe, D. B. (2011). Green roofs as a means of pollution abatement. Environ. Pollut. 159, 2100–2110. doi: 10.1016/j.envpol.2010.10.029
Rowe, D. B., Kolp, M. R., Greer, S. E., and Getter, K. L. (2014). Comparison of irrigation efficiency and plant health of overhead, drip, and sub-irrigation for extensive green roofs. Ecol. Eng. 64, 306–313. doi: 10.1016/j.ecoleng.2013.12.052
Russo, A., Escobedo, F. J., Cirella, G. T., and Zerbe, S. (2017). Edible green infrastructure: an approach and review of provisioning ecosystem services and disservices in urban environments. Agric. Ecosyst. Environ. 242, 53–66. doi: 10.1016/j.agee.2017.03.026
Sanjuan-Delmás, D., Llorach-Massana, P., Nadal, A., Ercilla-Montserrat, M., Muñoz, P., Montero, J. I., et al. (2018). Environmental assessment of an integrated rooftop greenhouse for food production in cities. J. Clean. Prod. 177, 326–337. doi: 10.1016/j.jclepro.2017.12.147
Sanyé-Mengual, E., Oliver-Solà, J., Montero, J. I., and Rieradevall, J. (2015a). An environmental and economic life cycle assessment of rooftop greenhouse (RTG) implementation in Barcelona, Spain. Assessing new forms of urban agriculture from the greenhouse structure to the final product level. Int. J. Life Cycle Assess. 20, 350–366. doi: 10.1007/s11367-014-0836-9
Sanyé-Mengual, E., Orsini, F., Oliver-Solà, J., Rieradevall, J., Montero, J. I., and Gianquinto, G. (2015b). Techniques and crops for efficient rooftop gardens in Bologna, Italy. Agron. Sustain. Dev. 35, 1477–1488. doi: 10.1007/s13593-015-0331-0
Sloan, J. J., Ampim, P. A. Y., Basta, N. T., and Scott, R. (2012). Addressing the need for soil blends and amendments for the highly modified urban landscape. Soil Sci. Soc. Am. J. 76, 1133–1141. doi: 10.2136/sssaj2011.0224
Specht, K., Siebert, R., Hartmann, I., Freisinger, U. B., Sawicka, M., Werner, A., et al. (2014). Urban agriculture of the future: an overview of sustainability aspects of food production in and on buildings. Agric. Hum. Val. 31, 33–51. doi: 10.1007/s10460-013-9448-4
Tanner, C. J., Adler, F. R., Grimm, N. B., Groffman, P. M., Levin, S. A., Munshi-South, J., et al. (2014). Urban ecology: advancing science and society. Front. Ecol. Environ. 12, 574–581. doi: 10.1890/140019
Thomaier, S., Specht, K., Henckel, D., Dierich, A., Siebert, R., Freisinger, U. B., et al. (2015). Farming in and on urban buildings: present practice and specific novelties of zero-acreage farming (ZFarming). Renew. Agric. Food Syst. 30, 43–54. doi: 10.1017/S1742170514000143
Tommasi, D., Miro, A., Higo, H. A., and Winston, M. L. (2004). Bee diversity and abundance in an urban setting. Can. Entomol. 136, 851–869. doi: 10.4039/n04-010
Tsirogiannis, I. L., Orsini, F., and Luz, P. (2017). “Water management and irrigation systems,” in Rooftop Urban Agriculture, eds F. Orsini, M. Dubbeling, H. de Zeeuw, and G. Gianquinto (Cham: Springer), 129–146.
van der Schans, J. W., and Wiskerke, J. S. (2012). “Urban agriculture in developed economies,” in Sustainable Food Planning: Evolving Theory and Practice eds A. Viljoen and J. S. C. Wiskerke (Wageningen Academic Publishers), 245–258. doi: 10.3920/978-90-8686-187-3_21
Van Ginkel, S. W., Igou, T., and Chen, Y. (2017). Energy, water and nutrient impacts of California-grown vegetables compared to controlled environmental agriculture systems in Atlanta, GA. Resour. Conserv. Recycl. 122, 319–325. doi: 10.1016/j.resconrec.2017.03.003
Van Mechelen, C., Dutoit, T., and Hermy, M. (2015). Adapting green roof irrigation practices for a sustainable future: a review. Sustain. Cities Soc. 19, 74–90. doi: 10.1016/j.scs.2015.07.007
Van Veenhuizen, R., and Danso, G. (2007). Profitability and Sustainability of Urban and Periurban Agriculture. Food & Agriculture Org.
Vitousek, P. M., Mooney, H. A., Lubchenco, J., and Melillo, J. M. (1997). Human domination of earth's ecosystems. Science 277, 494–499. doi: 10.1126/science.277.5325.494
Whittinghill, L., and Starry, O. (2016). “Up on the roof: considerations for food production on rooftops,” in Sowing Seeds in the City: Ecosystem and Municipal Services, eds S. Brown, K. McIvor and E. Hodges Snyder (Dordrecht: Springer Netherlands), 325–338.
Keywords: green infrastructure (GI), urban biogeochemistry, urban ecology, green roof, rooftop agriculture, ecosystem services (ES), urban agriculture (ua)
Citation: Harada Y and Whitlow TH (2020) Urban Rooftop Agriculture: Challenges to Science and Practice. Front. Sustain. Food Syst. 4:76. doi: 10.3389/fsufs.2020.00076
Received: 09 November 2019; Accepted: 30 April 2020;
Published: 04 June 2020.
Edited by:
Johann G. Zaller, University of Natural Resources and Life Sciences Vienna, AustriaReviewed by:
Olawale O. Oladeji, Metropolitan Water Reclamation District of Greater Chicago (MWRDGC), United StatesGM Shafiullah, Murdoch University, Australia
Panagiotis Nektarios, Hellenic Mediterranean University, Greece
Copyright © 2020 Harada and Whitlow. This is an open-access article distributed under the terms of the Creative Commons Attribution License (CC BY). The use, distribution or reproduction in other forums is permitted, provided the original author(s) and the copyright owner(s) are credited and that the original publication in this journal is cited, in accordance with accepted academic practice. No use, distribution or reproduction is permitted which does not comply with these terms.
*Correspondence: Yoshiki Harada, eWg1MzVAY29ybmVsbC5lZHU=