- 1Department of Hygiene and Public Health, Nippon Medical School, Tokyo, Japan
- 2Faculty of Pharmaceutical Sciences, Sanyo-onoda City University, Sanyo-Onoda, Japan
- 3Medical Biochemistry, Tohoku University Graduate School of Medicine, Sendai, Japan
Air pollution is associated with significant adverse health effects. Recent studies support the idea that inhalation of fine particles can instigate extrapulmonary effects on the cardiovascular system through several pathways. The systemic transfer of ultrafine particles (UFPs) or soluble particle components (organic compounds and metals) is of particular concern. An integral role of reactive oxygen species (ROS)-dependent pathways has been suggested in systemic inflammatory responses and vascular dysfunction at the molecular level. Accumulating lines of evidence suggest that fine particles affect fetal development, giving rise to low birth weight and a reduction in fetal growth, and also affect the immune, cardiovascular, and central nervous systems. Oxidative stress plays an important role in fine particles toxicity; pre-treatment with antioxidants partially suppresses the developmental toxicity of fine particles. On the other hand, Nuclear factor erythroid-derived 2-like 2 (Nfe2l2), also known as NRF2, is a transcription factor essential for inducible and/or constitutive expression of phase II and antioxidant enzymes. Studies using Nrf2-knockout mice revealed that NRF2 dysfunction is intimately involved in the pathogenesis of various human diseases. Multiple single nucleotide polymorphisms (SNPs) have been detected in human NRF2 locus. An NRF2 gene SNP (−617C > A; rs6721961), located in the upstream promoter region, affects the transcriptional level of NRF2 and thereby the protein level and downstream gene expression. It has been reported that the SNP-617 is associated with various diseases. The onset and exacerbation of the diseases are regulated by genetic predisposition and environmental factors; some people live in the air-polluted environment but are not affected and remain healthy, suggesting the presence of individual differences in the susceptibility to air pollutants. NRF2 polymorphisms may also be associated with the fetal effects of fine particles exposure. Screening high-risk pregnant women genetically susceptible to oxidative stress and prevention by antioxidant interventions to protect fetal development in air-polluted areas should be considered. This article reviews the recent advances in our understanding of the fetal health effects of fine particles and describes potential chemoprevention via the NRF2 pathway to prevent the developmental and reproductive toxicity of fine particles.
Introduction
The strongest evidence from many epidemiological studies linking air pollution to human health centers on particulate components (Dockery et al., 1993; Gehring et al., 2010; Krishnan et al., 2012; Xia et al., 2013). Fine particles are classified according to their aerodynamic diameter into size fractions such as particulate matter 2.5 (PM2.5, diameter fine inhalable particles, with diameters that are generally 2.5 micrometers and smaller) and ultrafine (nano-sized) particles (UFPs, fine inhalable particles, with diameters that are generally 0.1 micrometers and smaller) (Araujo and Nel, 2009). Recent studies support the hypothesis that inhalation of fine particles can instigate extrapulmonary effects on the cardiovascular system through several pathways. Many epidemiological studies have attempted to clarify the association between PM2.5 and extrapulmonary disorders such as ischemic cardiovascular diseases (Lipsett et al., 2011; Hayes et al., 2020), arteriosclerosis (Hoffmann et al., 2007; Kaufman et al., 2016), neurological disorders (Zanobetti et al., 2014; Grande et al., 2020), diabetes (Raaschou-Nielsen et al., 2013; Balti et al., 2014), fetal development, and reproduction (Volk et al., 2013; DeFranco et al., 2016) (Table 1). PM2.5 in the atmosphere contain a lot of UFPs. Systemic transfer of UFPs or soluble particle components (organic compounds and metals) is of most concern in this context. An integral role of reactive oxygen species (ROS)-dependent pathways has been suggested in systemic pro-inflammatory responses and vascular dysfunction at the molecular level (Brook et al., 2010). Although epidemiological studies on the health effects of UFPs also are needed, exposure assessment for atmospheric UFPs is complex (Sioutas et al., 2005) and emerging evidence on UFPs health effects has mainly led by experimental studies using animals (Sugamata et al., 2006; Shimizu et al., 2009; Takeda et al., 2009; Takahashi et al., 2010; Kubo-Irie et al., 2014; Onoda et al., 2014; Shimizu et al., 2014; El-Sayed et al., 2015; Mitsunaga et al., 2016; Onoda et al., 2017) and cells (Xia et al., 2004; Mo et al., 2009; Li et al., 2013).
On the other hand, Nuclear factor erythroid-derived 2-like 2 (Nfe2l2), also known as NRF2, is a transcription factor essential for the inducible and/or constitutive expression of phase II and antioxidant enzymes (Itoh et al., 1997). Recent studies using Nrf2-knockout mice revealed that NRF2 dysfunction is intimately involved in the pathogenesis of various human diseases after exposure to diesel exhaust (DE), and Nrf2 knockout mice are highly sensitive to oxidative stress caused by DE (Li et al., 2008; Li et al., 2010; Li et al., 2017; Li et al., 2020). Most DE particles (DEPs) are contained in fine particulates and contain nano-sized carbon particles at their core (Araujo and Nel, 2009). In this regard, it is interesting to note that multiple single nucleotide polymorphisms (SNPs) have been detected in human NRF2 locus. An NRF2 gene SNP (−617C > A; rs6721961), located in the upstream promoter region, affects the transcriptional level of NRF2 and thereby the protein level and downstream gene expression (Yamamoto et al., 2004). It has been reported that the SNP-617 is associated with various diseases (Marzec et al., 2007; von Otter et al., 2010; Ungvári et al., 2012; Suzuki et al., 2013; Okano et al., 2013; Shimoyama et al., 2014; Wang et al., 2015). It is speculated that the reduction of protein levels and downstream gene expression by the NRF2 gene SNP may increase susceptibility to oxidative stress caused by fine particulates exposure.
Many studies have suggested that fine particulates air pollution is related to developmental and reproductive (Gilboa et al., 2005; Shimizu et al., 2009; Takeda et al., 2009; Takahashi et al., 2010; Dadvand et al., 2013; Volk et al., 2013; Yokota et al., 2013; Onoda et al., 2014; Kubo-Irie et al., 2014; Symanski et al., 2014; El-Sayed et al., 2015; Tachibana et al., 2015; DeFranco et al., 2016; Mitsunaga et al., 2016; Yokota et al., 2016; Carré et al., 2017; Chen et al., 2017; Martens et al., 2017; Onoda et al., 2017). The impact of air pollutants on the next generation is of great concern, and preventive measures are required. This article reviews the recent advances in our understanding of the fetal health effects of fine particulates and discusses the potential chemoprevention via the NRF2 pathway to prevent the developmental and reproductive toxicity of fine particulates.
Characteristics of Fine Particulates
Particulate matter is a complex mixture of organic and inorganic chemicals, including metals and particulates (Mo et al., 2009), and is composed of heterogeneous compounds of different sizes, chemical compositions, surface areas, concentrations, and sources. Fine particles are classified according to their aerodynamic diameter into size fractions such as PM2.5 (particulate matter of diameter <2.5 μm) and UFPs (particulate matter of diameter <0.1 μm). These particles are derived from various sources and by various mechanisms as shown in Table 2 (Araujo and Nel, 2009). These are just physical definitions, and the chemical components and the subsequent toxic characteristics of fine particles vary by country or region. Differences in energy structure, economic development, climate classification, etc., determine the type of air pollution and the chemical composition of fine particles (Pan et al., 2014). At the cellular level, fine particles various mechanisms involve free radical production (by transition metals and organic compounds), oxidative stress, cytokine release, inflammation, etc., (Araujo and Nel, 2009).

TABLE 2. Classification of fine particles based on size *.
Several studies have shown that UFPs are more toxic than larger particles (Li et al., 2003; Donaldson et al., 2005). UFPs are important because when compared with larger particles, they have order of higher particle number concentration and surface area, and larger concentrations of adsorbed or condensed toxic air pollutants (oxidant gases, organic compounds, transition metals) per unit mass (Sioutas et al., 2005). Also facilitates their intake during breathing, and their mass ratio greatly enhances their chemical/catalytic reactivity compared to large-sized particles (Mikami et al., 2014). UFPs are not as easily phagocytized by alveolar macrophages as larger particles. They may enter the blood circulation, and translocation to extra-pulmonary tissues (Frampton, 2001; Sioutas et al., 2005; Brook et al., 2010). UFPs, with their high surface area, can carry large amounts of adsorbed or condensed toxic air pollutants, such as oxidant gas, organic compounds and transition metals (Oberdörster and Utell, 2002). It is also reported that the induction of mitochondrial dysfunction caused by DEPs and UFPs are mediated by adsorbed chemicals quinones and aromatics rather than the particles themselves (Xia et al., 2004).
DE emissions, are a major source of UFPs in urban environments, and it is these particles that have the capacity to induce the most significant health effects (Wåhlin et al., 2001; Miller and Newby, 2020). Previous studies have shown that DE exposure can have many adverse effects on the cardiovascular system, both acutely and chronically (Miller and Newby, 2020). DEPs have a complex structure characterized by nano-sized carbon particles at their core with adsorbed organic compounds such as polyaromatic hydrocarbons (PAHs) and quinones. The PAHs and their oxygenated derivatives (e.g., quinones) have attracted attention because they are able to participate in the redox cycle and generate ROS in target cells (Takizawa, 2004). Therefore, DEPs have been extensively used in studies as a model air pollutant. However, it has been also reported that standardized DEPs such as standard reference material (SRM)-2,975 are not suitable to represent traffic emissions and typical ambient particulate matter used in toxicological studies (Farahani et al., 2021). Since the actual composition of air pollutants depends on the region, the DEPs used in the research has a limit as a model of air pollutants.
Fine Particles Affect Fetal Health
Studies support an association for fine particles and fetal health (Table 3). Exposure to PM2.5 affects development and reproduction as have been documented in epidemiological reports (Dadvand et al., 2013; Volk et al., 2013; Symanski et al., 2014; DeFranco et al., 2016; Martens et al., 2017) and supported by data from animal-model experiments (Yokota et al., 2013; Tachibana et al., 2015; Yokota et al., 2016; Chen et al., 2017). Epidemiological reports revealed that exposure to fine particulates during pregnancy is associated with autism (Volk et al., 2013) and causes changes in reproductive function (Carré et al., 2017). Volk, et al. (2013) Reported that exposure to traffic-related air pollution, such as PM2.5, during pregnancy and the first year of life was associated with autism. This study is based on a case-control study and includes data from 279 children with autism and 245 children with typical development in California (Volk et al., 2013). Exposure to fine particulates during pregnancy is also associated with biological aging (Martens et al., 2017), preterm birth (Symanski et al., 2014; DeFranco et al., 2016), and low birth weight (Dadvand et al., 2013). Similarly, animal-model experiments suggested that exposure to DE during pregnancy alters energy metabolism (Chen et al., 2017) and nervous function (Tachibana et al., 2015; Yokota et al., 2016). In animal model experiments, exposure to UFPs smaller than 0.1 µm diameter appeared critical for the development and reproduction, such as changes in reproductive function (Takeda et al., 2009; Kubo-Irie et al., 2014) and immune responses (Shimizu et al., 2014; El-Sayed et al., 2015). Sugamata, et al. (2006) reported that maternal exposure to DE which contain nano-sized carbon particles at their core, alters the ultrastructure of perivascular macrophages (PVMs) and surrounding tissues in the brain of mouse offspring. Onoda et al. (2014) found that maternal exposure to ultrafine carbon black altered the phenotype of PVMs and astrocytes close to blood vessels in offspring mice. This results suggest that maternal ultrafine carbon black exposure may associated with increased risk of dysfunction in the offspring brain (Onoda et al., 2014). Umezawa et al. (2014) also reported that the degree of the risk on offspring depends on the type of nanoparticles. Furthermore, many other animal studies also reported that exposure to UFPs provokes fetal brain dysfunction (Shimizu et al., 2009; Takeda et al., 2009; Takahashi et al., 2010; Mitsunaga et al., 2016; Onoda et al., 2017). Ambient fine particles contain a large proportion of UFPs; due to their small size, UFPs have high physicochemical reactivity and show unique behaviors in vivo including the following three potential pathways: 1) release of proinflammatory mediators from lung cells, 2) affect autonomic nervous system balance by particle interactions with lung receptors or nerves, and 3) translocation of UFPs and soluble particle components into the systemic circulation. Therefore, inhaled UFPs can reach the alveolar region and extrapulmonary organs (Brook et al., 2010). It is also possible that the UPFs contained in the fine particles may affect the foetation. UFPs also have higher permeability than large-sized particles; this facilitates the translocation of particles from mother to infant (Mikami et al., 2014).
Mechanisms of Fine Particles Biological Activity: Role of Oxidative Stresses Induced by Fine Particles
There is increasing evidence that fine particulate pollutants induce inflammatory responses, and these proinflammatory effects have been linked to the ability of fine particulate, such as DEPs, to generate ROS and oxidative stress in bronchial epithelial cells (Takizawa et al., 1999; Hashimoto et al., 2000), macrophages (Li et al., 2004). Li et al. (2003) demonstrated that the increased biological potency of UFPs is related to the content of redox cycling organic chemicals and their ability to damage mitochondria. UFPs were collected by ambient particle concentrators in the Los Angeles basin in California and used to study their chemical composition in parallel with assays for generation of ROS and ability to induce oxidative stress in macrophages and epithelial cells. UFPs exposure induces oxidative stress by promoting cellular heme oxygenase-1 (HO-1) expression; HO-1 depletes intracellular glutathione and is a sensitive marker of oxidative stress. The results showed that HO-1 expression directly correlated with the high organic carbon and polycyclic aromatic hydrocarbon (PAH) content of UFPs; PAHs have been identified in placental tissue and umbilical cord blood from neonates. Detection of damaged DNA in cord blood indicates that exposure to these pollutants in the environment can cause fetal damage (RavindraMittal and Van Grieken, 2001). Oxidative stress likely plays an important role in nanoparticle toxicity, as pre-treatment with antioxidants partially suppresses the developmental toxicity of nanoparticles (Wang et al., 2010). PAHs, usually bound to fine particles and UFPs, increase the risk of intrauterine growth retardation. The permeability and increased ROS generation (which induces oxidative stress in cells) of small particles are the mechanisms underlying these harmful effects (Wåhlin et al., 2001).
NRF2 as a Key Trascription Factor Preventing Cellular Damage in Response to Oxidative Stress
Nrf2 is a key transcription factor that is essential for the induction and/or constitutive expression of phase II enzymes and antioxidants in response to ROS or electrophile (Itoh et al., 1997). Nrf2 possesses a Neh2 domain, which is recognized by Keap1 (Kelch-like ECH-associated protein 1) and acts as a degron–an amino acid sequence, which signals degradation (Itoh et al., 1999) Upon exposure to oxidative and electrophilic stress, Nrf2 is activated, and accumulates in nuclei, forms a heterodimer with a member of small Maf proteins, and binds to antioxidant ⁄ electrophile responsive elements (ARE⁄EpRE) located in its target genes (Rushmore et al., 1991; Prestera et al., 1995). This leads to the induction of a battery antioxidants (Ishii et al., 1993) and phase II detoxifying enzyme genes (Itoh et al., 1997) (Figure 1). Cytoprotective pathways are induced by the Nrf2 transcription signal pathway at the lowest levels of oxidative stress from DEPs and can induce the transcription of antioxidant genes in the earliest level of defense. Nrf2 regulates antioxidant defense that is constituted as the main defense action against the pro-inflammatory and oxidizing effects of DEPs (Li et al., 2004). Xiao et al. (2003) showed the hierarchical oxidative stress model in response to redox cycling DEPs components in vitro: Cytoprotective pathways are induced by the Nrf2 transcription signal pathway at the lowest levels of oxidative stress, and this may constitute the first tier of a hierarchical oxidative stress response, as is in the production of heme oxygenase (HO)-1. If these enzymes fail to neutralize the effects of ROS, proinflammatory effects constitute a second tier or superimposed level of oxidative stress. The final tier or superimposed level of oxidative stress is cytotoxicity, including the initiation of programmed cell death. (Xiao et al., 2003). DNA adduct formation is accelerated in the lungs of Nrf2 knockout mice exposed to DE inhalation (Aoki et al., 2001). Previous studies suggest that oxidative stress induced by DE inhalation is associated with airway inflammation (Li et al., 2008), allergic asthma (Li et al., 2010), pulmonary fibrosis (Li et al., 2017), and airway innate immune responses (Li et al., 2020) evidenced in experiments using Nrf2 knockout mice. Nrf2 also played an important role in mediating the adjuvant effect of UFPs at the level of functional dendritic cells (Li et al., 2013).
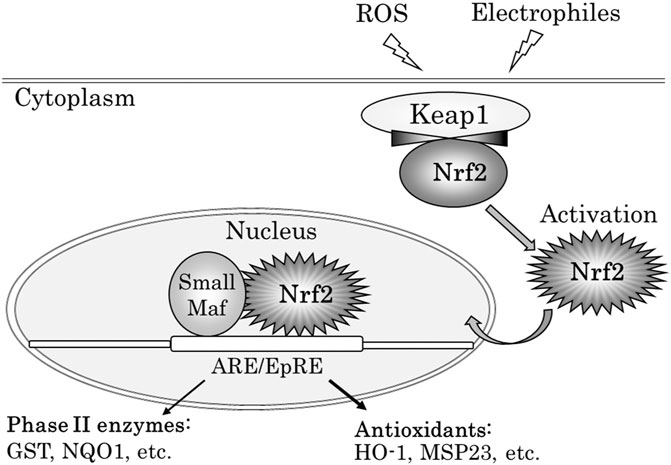
FIGURE 1. Activation of Nrf2 with ROS or electrophiles, and expression of Phase II enzyme genes and antioxidant stress protein genes via ARE/EpRE. Under non-stressed conditions, the transcription factor Nrf2 is constitutively degraded by binding to Keap1. ROS or electrophilic attack leads to the dissociation of Nrf2 from Keap1. Activated Nrf2 protein is then translocated into the nucleus and many genes encoding detoxifying and antioxidant enzymes are expressed. ROS: reactive oxygen species, ARE: antioxidant response element, EpRE: electrophile responsive element, GST: glutathione S-transferase, NQO1: NAD(P)H quinone dehydrogenase 1, HO-1: heme oxygenase-1, MSP23: macrophage 23-kDa stress protein.
NRF2 Polymorphism
Multiple single nucleotide polymorphisms (SNPs) have been identified in human NRF2 (Yamamoto et al., 2004). The NRF2 gene SNP (-617C > A; rs6721961) located in the upstream promoter region affects the transcriptional level of NRF2 and thus the protein level and downstream gene expression. Suzuki et al. (2013) reported that minor A/A homozygotes of NRF2 rSNP-617 exhibit significantly decreased NRF2 gene expression. SNP-617 was found to be associated with a higher risk of oxidant-induced acute lung injury in humans (Yamamoto et al., 2004; Marzec et al., 2007). Individuals with NRF2 polymorphisms have been reported to be at greater risk of developing acute lung injury (Marzec et al., 2007), Parkinson’s disease (von Otter et al., 2010), diabetes mellitus (Wang et al., 2015), chronic obstructive pulmonary disease (Hua et al., 2010), breast cancer (Hartikainen et al., 2012), cerebrovascular disease (Kunnas et al., 2016), and vascular stiffness (Shimizu et al., 2020). The presence of NRF2 polymorphisms correlates significantly with the incidence of non-small cell lung cancer, especially in smokers (Suzuki et al., 2013), and is also related to air pollution and childhood asthma (Ungvári et al., 2012) (Table 4). Thus, personalized medicine based on NRF2 polymorphisms might be effective to treat environmental pollutant-induced diseases.
As mentioned above, the NRF2 transcription factor controls cellular adaptation/protection to ROS and electrophiles by inducing antioxidant and detoxification genes. Under non-stressed conditions, the transcription factor Nrf2 is constitutively degraded by binding to Keap1 (Itoh et al., 1997; Itoh et al., 1999; Kobayashi et al., 2004). Oxidative stress and/or electrophilic attack leads to the dissociation of Nrf2 from Keap1 (Kobayashi et al., 2006); the Nrf2 protein is then translocated into the nucleus (Iso et al., 2016), and many genes encoding detoxifying and antioxidant enzymes are regulated by Nrf2 (Motohashi and Yamamoto, 2004; Kobayashi and Yamamoto, 2006; Suzuki et al., 2020). Notably, changes in Nrf2 transcript level alter the Nrf2 protein level, even in the basal state, in which Keap1 actively degrades Nrf2. When the appropriate Nrf2 transcript is supplied, the Nrf2 protein is maintained at low levels by Keap1-mediated degradation under basal conditions, and constant levels of Nrf2 protein are accumulated after inactivation by Keap1. When Nrf2 transcription levels are low, Nrf2 protein is reduced in both basal and stress-induced states. When Nrf2 transcription levels are high, large amounts of Nrf2 are produced in both basal and stress-induced states (Suzuki et al., 2013). This study suggests that the transcription level of the NRF2 gene is indeed important for the roles that NRF2 plays in cytoprotection (Okano et al., 2013).
A Personalized Prevention of Fine Particles-Induced Oxidative Stress Based on NRF2 Polymorphism
The onset and exacerbation of diseases are regulated by genetic predisposition and environmental factors; some people living in an air-polluted environment are not affected by it and remain healthy. This suggests that there are individual differences in the susceptibility to air pollutants. Many reports mentioned above suggest that NRF2 polymorphisms may be associated with the development and replication of oxidative stress caused by nanoparticle exposure. It has been reported that the NRF2 SNP homozygous allele (–617A/A) is a useful biomarker for clinical diagnosis (Okano et al., 2013). In air-polluted areas, it is possible to screen high-risk pregnant women genetically susceptible to oxidative stress using NRF2 SNP homozygous allele (-617A/A) biomarker; personalized prevention of UFPs-induced oxidative stress at the developmental and reproductive stages, by antioxidant interventions based on NRF2 polymorphisms, may be feasible. Activation of NRF2 represses pro-inflammatory reactions induced by oxidative stress and ameliorates various inflammatory diseases. Many NRF2-activating compounds have been developed or are currently being refined (Suzuki et al., 2020). Figure 2 shows a schematic diagram of the potential of the NRF2 pathway to prevent developmental and reproductive toxicity caused by fine particles.
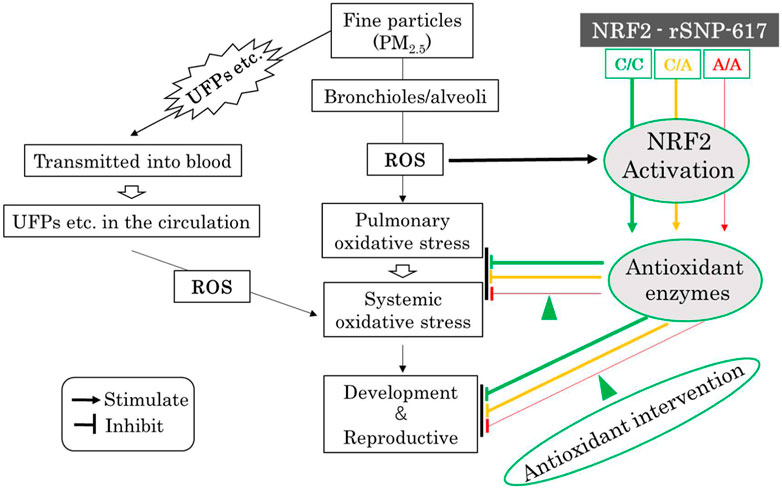
FIGURE 2. Hypothesis schematic diagram of the potential NRF2 pathways to prevent developmental and reproductive toxicity of fine particles. The colored lines show the putative NRF2 signaling pathway, with the signals being weaker in the order green, orange, and red. ROS: reactive oxygen species, UFPs: ultrafine particles, C/C: wild-type homozygous (c.–617C/C) alleles, C/A: SNP heterozygous (c.–617C/A) alleles, A/A: SNP homozygous (c.–617A/A) alleles; Green arrowhead: antioxidant intervention (NRF2-activating compounds, etc.).
Conclusion
This review is a literature review and has not been statistically analyzed. Regarding the health effects of fine particulates, the effects of component analysis have not been analyzed. Although there are these limits, the information summarized in this report highlights the importance of the NRF2-antioxidant pathway and proposes a hypothesis for preventing developmental and reproductive processes implicated in oxidative stress caused by exposure to fine particles in the atmosphere.
Author Contributions
Y-JL wrote the manuscript. KT, MY and TK critically revised the article for important intellectual content. All authors contributed to the manuscript and approved the submitted version.
Funding
This work was supported by a Grant-in-Aid for Scientific Research (No. 21590668) from the Ministry of Education, Culture, Sports, Science and Technology (MEXT) of Japan, and the Center for Environmental Health Science for the Next Generation, Research Institute for Science and Technology, Tokyo University of Science of Japan. This work was also supported by the Environmental Restoration and Conservation Agency of Japan.
Conflict of Interest
The authors declare that the research was conducted in the absence of any commercial or financial relationships that could be construed as a potential conflict of interest.
Publisher’s Note
All claims expressed in this article are solely those of the authors and do not necessarily represent those of their affiliated organizations, or those of the publisher, the editors and the reviewers. Any product that may be evaluated in this article, or claim that may be made by its manufacturer, is not guaranteed or endorsed by the publisher.
Acknowledgments
We gratefully thank all our research collaborators, especially Dr. Masakazu Umezawa (Tokyo University of Science), Dr. Arata Azuma (Nippon Medical School), Dr. Hajime Takizawa (Kyorin University), Dr. Isamu Sugawara (Research Institute of Tuberculosis), Dr. Satoru Takahashi (University of Tsukuba), and Dr. Shoji Kudoh (Anti-Tuberculosis Association) for their cooperation and critical comments. This work supported by the staff of the Department of Hygiene and Public Health (Nippon Medical School).
References
Aoki, Y., Sato, H., Nishimura, N., Takahashi, S., Itoh, K., and Yamamoto, M. (2001). Accelerated DNA adduct formation in the lung of the Nrf2 knockout mouse exposed to diesel exhaust. Toxicol. Appl. Pharmacol. 173, 154–160. doi:10.1006/taap.2001.9176
Araujo, J. A., and Nel, A. E. (2009). Particulate matter and atherosclerosis: role of particle size, composition and oxidative stress. Part. Fibre Toxicol. 6, 24. doi:10.1186/1743-8977-6-24
Balti, E. V., Echouffo-Tcheugui, J. B., Yako, Y. Y., and Kengne, A. P. (2014). Air pollution and risk of type 2 diabetes mellitus: a systematic review and meta-analysis. Diabetes Res. Clin. Pract. 106, 161–172. doi:10.1016/j.diabres.2014.08.010
Brook, R. D., Rajagopalan, S., Pope, C. A., Brook, J. R., Bhatnagar, A., Diez-Roux, A. V., et al. (2010). Particulate Matter Air Pollution and Cardiovascular Disease. Circulation 121, 2331–2378. doi:10.1161/cir.0b013e3181dbece1
Carré, J., Gatimel, N., Moreau, J., Parinaud, J., and Léandri, R. (2017). Does air pollution play a role in infertility? a systematic review. Environ. Health 16, 82. doi:10.1186/s12940-017-0291-8
Chen, M., Liang, S., Zhou, H., Xu, Y., Qin, X., Hu, Z., et al. (2017). Prenatal and postnatal mothering by diesel exhaust PM2.5-exposed dams differentially program mouse energy metabolism. Part. Fibre Toxicol. 14, 3. doi:10.1186/s12989-017-0183-7
Dadvand, P., Parker, J., Bell, M. L., Bonzini, M., Brauer, M., Darrow, L. A., et al. (2013). Maternal exposure to particulate air pollution and term birth weight: a multi-country evaluation of effect and heterogeneity. Environ. Health Perspect. 121, 267–373. doi:10.1289/ehp.1205575
DeFranco, E., Moravec, W., Xu, F., Hall, E., Hossain, M., Haynes, E. N., et al. (2016). Exposure to airborne particulate matter during pregnancy is associated with preterm birth: a population-based cohort study. Environ. Health 15, 6. doi:10.1186/s12940-016-0094-3
Dockery, D. W., Pope, C. A., Xu, X., Spengler, J. D., Ware, J. H., Fay, M. E., et al. (1993). An association between air pollution and mortality in six U.S. cities. N. Engl. J. Med. 329, 1753–1759. doi:10.1056/nejm199312093292401
Donaldson, K., Tran, L., Jimenez, L., Duffin, R., Newby, D. E., Mills, N., et al. (2005). Combustion-derived nanoparticles: a review of their toxicology following inhalation exposure. Part. Fibre Toxicol. 2, 10. doi:10.1186/1743-8977-2-10
El-Sayed, Y. S., Shimizu, R., Onoda, A., Takeda, K., and Umezawa, M. (2015). Carbon black nanoparticle exposure during middle and late fetal development induces immune activation in male offspring mice. Toxicology 327, 53–61. doi:10.1016/j.tox.2014.11.005
Farahani, V. J., Pirhadi, M., and Sioutas, C. (2021). Are standardized diesel exhaust particles (DEP) representative of ambient particles in air pollution toxicological studies? Sci. Total Environ. 788, 147854. doi:10.1016/j.scitotenv.2021.147854
Frampton, M. W. (2001). Systemic and cardiovascular effects of airway injury and inflammation: ultrafine particle exposure in humans. Environ. Health Perspect. 109 (Suppl. 4), 529–532. doi:10.2307/3454664
Gehring, U., Wijga, A. H., Brauer, M., Fischer, P., de Jongste, J. C., Kerkhof, M., et al. (2010). Traffic-related air pollution and the development of asthma and allergies during the first 8 years of life. Am. J. Respir. Crit. Care Med. 181, 596–603. doi:10.1164/rccm.200906-0858oc
Gilboa, S. M., Mendola, P., Olshan, A. F., Langlois, P. H., Savitz, D. A., Loomis, D., et al. (2005). Relation between ambient air quality and selected birth defects, seven county study, Texas, 1997-2000. Am. J. Epidemiol. 162, 238–252. doi:10.1093/aje/kwi189
Grande, G., Ljungman, P. L. S., Eneroth, K., Bellander, T., and Rizzuto, D. (2020). Association between Cardiovascular Disease and Long-term Exposure to Air Pollution with the Risk of Dementia. JAMA Neurol. 77, 801–809. doi:10.1001/jamaneurol.2019.4914
Hartikainen, J. M., Tengström, M., Kosma, V.-M., Kinnula, V. L., Mannermaa, A., and Soini, Y. (2012). Genetic polymorphisms and protein expression of NRF2 and Sulfiredoxin predict survival outcomes in breast cancer. Cancer Res. 72, 5537–5546. doi:10.1158/0008-5472.can-12-1474
Hashimoto, S., Gon, Y., Takeshita, I., Matsumoto, K., Jibiki, I., Takizawa, H., et al. (2000). Diesel Exhaust Particles Activate p38 MAP Kinase to Produce Interleukin 8 and RANTES by Human Bronchial Epithelial Cells andN-Acetylcysteine Attenuates p38 MAP Kinase Activation. Am. J. Respir. Crit. Care Med. 161, 280–285. doi:10.1164/ajrccm.161.1.9904110
Hayes, R. B., Lim, C., Zhang, Y., Cromar, K., Shao, Y., Reynolds, H. R., et al. (2020). PM2.5 air pollution and cause-specific cardiovascular disease mortality. Int. J. Epidemiol. 49, 25–35. doi:10.1093/ije/dyz114
Hoffmann, B., Moebus, S., Möhlenkamp, S., Stang, A., Lehmann, N., Dragano, N., et al. (2007). Residential exposure to traffic is associated with coronary atherosclerosis. Circulation 116, 489–496. doi:10.1161/circulationaha.107.693622
Hua, C.-C., Chang, L.-C., Tseng, J.-C., Chu, C.-M., Liu, Y.-C., and Shieh, W.-B. (2010). Functional Haplotypes in the Promoter Region of Transcription FactorNrf2in Chronic Obstructive Pulmonary Disease. Dis. Markers 28, 185–193. doi:10.1155/2010/143142
Ishii, T., Yamada, M., Sato, H., Matsue, M., Taketani, S., Nakayama, K., et al. (1993). Cloning and characterization of a 23-kDa stress-induced mouse peritoneal macrophage protein. J. Biol. Chem. 268, 18633–18636. doi:10.1016/s0021-9258(17)46676-2
Iso, T., Suzuki, T., Baird, L., and Yamamoto, M. (2016). Absolute Amounts and Status of the Nrf2-Keap1-Cul3 Complex within Cells. Mol. Cell Biol. 36, 3100–3112. doi:10.1128/mcb.00389-16
Itoh, K., Chiba, T., Takahashi, S., Ishii, T., Igarashi, K., Katoh, Y., et al. (1997). An Nrf2/small Maf heterodimer mediates the induction of phase II detoxifying enzyme genes through antioxidant response elements. Biochem. Biophys. Res. Commun. 236, 313–322. doi:10.1006/bbrc.1997.6943
Itoh, K., Wakabayashi, N., Katoh, Y., Ishii, T., Igarashi, K., Engel, J. D., et al. (1999). Keap1 represses nuclear activation of antioxidant responsive elements by Nrf2 through binding to the amino-terminal Neh2 domain. Genes Dev. 13, 76–86. doi:10.1101/gad.13.1.76
Kaufman, J. D., Adar, S. D., Barr, R. G., Budoff, M., Burke, G. L., Curl, C. L., et al. (2016). Association between air pollution and coronary artery calcification within six metropolitan areas in the USA (the Multi-Ethnic Study of Atherosclerosis and Air Pollution): a longitudinal cohort study. The Lancet 388, 696–704. doi:10.1016/s0140-6736(16)00378-0
Kobayashi, A., Kang, M.-I., Okawa, H., Ohtsuji, M., Zenke, Y., Chiba, T., et al. (2004). Oxidative stress sensor Keap1 functions as an adaptor for Cul3-based E3 ligase to regulate proteasomal degradation of Nrf2. Mol. Cell Biol. 24, 7130–7139. doi:10.1128/mcb.24.16.7130-7139.2004
Kobayashi, A., Kang, M.-I., Watai, Y., Tong, K. I., Shibata, T., Uchida, K., et al. (2006). Oxidative and electrophilic stresses activate Nrf2 through inhibition of ubiquitination activity of Keap1. Mol. Cell Biol. 26, 221–229. doi:10.1128/mcb.26.1.221-229.2006
Kobayashi, M., and Yamamoto, M. (2006). Nrf2-Keap1 regulation of cellular defense mechanisms against electrophiles and reactive oxygen species. Adv. Enzyme Regul. 46, 113–140. doi:10.1016/j.advenzreg.2006.01.007
Krishnan, R. M., Adar, S. D., Szpiro, A. A., Jorgensen, N. W., Van Hee, V. C., Barr, R. G., et al. (2012). Vascular Responses to Long- and Short-Term Exposure to Fine Particulate Matter. J. Am. Coll. Cardiol. 60, 2158–2166. doi:10.1016/j.jacc.2012.08.973
Kubo-Irie, M., Uchida, H., Mastuzawa, S., Yoshida, Y., Shinkai, Y., Suzuki, K., et al. (2014). Dose-dependent biodistribution of prenatal exposure to rutile-type titanium dioxide nanoparticles on mouse testis. J. Nanopart. Res. 16, 2284. doi:10.1007/s11051-014-2284-7
Kunnas, T., Määttä, K., and Nikkari, S. T. (2016). Genetic Polymorphisms of Transcription Factor NRF2 and of its Host Gene Sulfiredoxin (SRXN1) are Associated with Cerebrovascular Disease in a Finnish Cohort, the TAMRISK Study. Int. J. Med. Sci. 13, 325–329. doi:10.7150/ijms.14849
Li, N., Alam, J., Venkatesan, M. I., Eiguren-Fernandez, A., Schmitz, D., Di Stefano, E., et al. (2004). Nrf2 is a key transcription factor that regulates antioxidant defense in macrophages and epithelial cells: protecting against the proinflammatory and oxidizing effects of diesel exhaust chemicals. J. Immunol. 173, 3467–3481. doi:10.4049/jimmunol.173.5.3467
Li, N., Sioutas, C., Cho, A., Schmitz, D., Misra, C., Sempf, J., et al. (2003). Ultrafine particulate pollutants induce oxidative stress and mitochondrial damage. Environ. Health Perspect. 111, 455–460. doi:10.1289/ehp.6000
Li, N., Wang, M., Barajas, B., Sioutas, C., Williams, M. A., and Nel, A. E. (2013). Nrf2 deficiency in dendritic cells enhances the adjuvant effect of ambient ultrafine particles on allergic sensitization. J. Innate Immun. 5, 543–554. doi:10.1159/000347060
Li, Y.-J., Shimizu, T., Shinkai, Y., Hirata, Y., Inagaki, H., Takeda, K., et al. (2017). Nrf2 Regulates the Risk of a Diesel Exhaust Inhalation-Induced Immune Response during Bleomycin Lung Injury and Fibrosis in Mice. Ijms 18 (3), 649. doi:10.3390/ijms18030649
Li, Y.-J., Shimizu, T., Shinkai, Y., Ihara, T., Sugamata, M., Kato, K., et al. (2020). Nrf2 Lowers the Risk of Lung Injury via Modulating the Airway Innate Immune Response Induced by Diesel Exhaust in Mice. Biomedicines 8, 443. doi:10.3390/biomedicines8100443
Li, Y. J., Takizawa, H., Azuma, A., Kohyama, T., Yamauchi, Y., Takahashi, S., et al. (2008). Disruption of Nrf2 enhances susceptibility to airway inflammatory responses induced by low-dose diesel exhaust particles in mice. Clin. Immunol. 128, 366–373. doi:10.1016/j.clim.2008.05.005
Li, Y. J., Takizawa, H., Azuma, A., Kohyama, T., Yamauchi, Y., Takahashi, S., et al. (2010). Nrf2 is closely related to allergic airway inflammatory responses induced by low-dose diesel exhaust particles in mice. Clin. Immunol. 137, 234–241. doi:10.1016/j.clim.2010.07.014
Lipsett, M. J., Ostro, B. D., Reynolds, P., Goldberg, D., Hertz, A., Jerrett, M., et al. (2011). Long-term exposure to air pollution and cardiorespiratory disease in the California teachers study cohort. Am. J. Respir. Crit. Care Med. 184, 828–835. doi:10.1164/rccm.201012-2082oc
Martens, D. S., Cox, B., Janssen, B. G., Clemente, D. B. P., Gasparrini, A., Vanpoucke, C., et al. (2017). Prenatal air pollution and newborns' predisposition to accelerated biological aging. JAMA Pediatr. 171, 1160–1167. doi:10.1001/jamapediatrics.2017.3024
Marzec, J. M., Christie, J. D., Reddy, S. P., Jedlicka, A. E., Vuong, H., Lanken, P. N., et al. (2007). Functional polymorphisms in the transcription factor NRF2 in humans increase the risk of acute lung injury. FASEB j. 21, 2237–2246. doi:10.1096/fj.06-7759com
Mikami, Y. (2014). “Adverse fetal effects of diesel exhaust induced oxidative stress in PM2.,” in 5-role of oxidative stress in health effects & prevention strategy. Editors Y. J. Li, M. Umezawa, H. Takizawa, K. Takeda, and T. Kawada (New York: Nova Science Publishers), 133–140.
Miller, M. R., and Newby, D. E. (2020). Air pollution and cardiovascular disease: car sick. Cardiovasc. Res. 116, 279–294. doi:10.1093/cvr/cvz228
Mitsunaga, F., Umezawa, M., Takeda, K., and Nakamura, S. (2016). Maternal administration of nanomaterials elicits hemoglobin upregulation in the neonatal brain of non-human primates. J. Toxicol. Sci. 41, 265–271. doi:10.2131/jts.41.265
Mo, Y., Wan, R., Chien, S., Tollerud, D. J., and Zhang, Q. (2009). Activation of endothelial cells after exposure to ambient ultrafine particles: the role of NADPH oxidase. Toxicol. Appl. Pharmacol. 236, 183–193. doi:10.1016/j.taap.2009.01.017
Motohashi, H., and Yamamoto, M. (2004). Nrf2-Keap1 defines a physiologically important stress response mechanism. Trends Mol. Med. 10, 549–557. doi:10.1016/j.molmed.2004.09.003
Oberdörster, G., and Utell, M. J. (2002). Ultrafine particles in the urban air: to the respiratory tract--and beyond? Environ. Health Perspect. 110, A440–A441. doi:10.1289/ehp.110-1240959
Okano, Y., Nezu, U., Enokida, Y., Lee, M. T. M., Kinoshita, H., Lezhava, A., et al. (2013). SNP (-617C>A) in ARE-Like Loci of the NRF2 Gene: A New Biomarker for Prognosis of Lung Adenocarcinoma in Japanese Non-smoking Women. PLoS One 8, e73794. doi:10.1371/journal.pone.0073794
Onoda, A., Takeda, K., and Umezawa, M. (2017). Dose-dependent induction of astrocyte activation and reactive astrogliosis in mouse brain following maternal exposure to carbon black nanoparticle. Part. Fibre Toxicol. 14, 4. doi:10.1186/s12989-017-0184-6
Onoda, A., Umezawa, M., Takeda, K., Ihara, T., and Sugamata, M. (2014). Effects of maternal exposure to ultrafine carbon black on brain perivascular macrophages and surrounding astrocytes in offspring mice. PLoS One 9, e94336. doi:10.1371/journal.pone.0094336
Pan, X. (2014). “The study on air PM2.5 pollution health effects-recent trends in china current conditions in PM2.,” in 5-role of oxidative stress in health effects & prevention strategy. Editors Y. J. Li, M. Umezawa, H. Takizawa, K. Takeda, and T. Kawada (New York: Nova Science Publishers), 37–45.
Prestera, T., Talalay, P., Alam, J., Ahn, Y. I., Lee, P. J., and Choi, A. M. K. (1995). Parallel induction of heme oxygenase-1 and chemoprotective phase 2 enzymes by electrophiles and antioxidants: regulation by upstream antioxidant-responsive elements (ARE). Mol. Med. 1, 827–837. doi:10.1007/bf03401897
Raaschou-Nielsen, O., Sørensen, M., Ketzel, M., Hertel, O., Loft, S., Tjønneland, A., et al. (2013). Long-term exposure to traffic-related air pollution and diabetes-associated mortality: a cohort study. Diabetologia 56, 36–46. doi:10.1007/s00125-012-2698-7
Ravindra,, , Mittal, A. K., and Van Grieken, R. (2001). Health risk assessment of urban suspended particulate matter with special reference to polycyclic aromatic hydrocarbons: a review. Rev. Environ. Health 16, 169–189. doi:10.1515/reveh.2001.16.3.169
Rushmore, T. H., Morton, M. R., and Pickett, C. B. (1991). The antioxidant responsive element. Activation by oxidative stress and identification of the DNA consensus sequence required for functional activity. J. Biol. Chem. 266, 11632–11639. doi:10.1016/s0021-9258(18)99004-6
Shimizu, M., Tainaka, H., Oba, T., Mizuo, K., Umezawa, M., and Takeda, K. (2009). Maternal exposure to nanoparticulate titanium dioxide during the prenatal period alters gene expression related to brain development in the mouse. Part. Fibre Toxicol. 6, 20. doi:10.1186/1743-8977-6-20
Shimizu, R., Umezawa, M., Okamoto, S., Onoda, A., Uchiyama, M., Tachibana, K., et al. (2014). Effect of maternal exposure to carbon black nanoparticle during early gestation on the splenic phenotype of neonatal mouse. J. Toxicol. Sci. 39, 571–578. doi:10.2131/jts.39.571
Shimizu, S., Mimura, J., Hasegawa, T., Shimizu, E., Imoto, S., Tsushima, M., et al. (2020). Association of single nucleotide polymorphisms in the NRF2 promoter with vascular stiffness with aging. PLoS One 15, e0236834. doi:10.1371/journal.pone.0236834
Shimoyama, Y., Mitsuda, Y., Tsuruta, Y., Hamajima, N., and Niwa, T. (2014). Polymorphism of Nrf2, an antioxidative gene, is associated with blood pressure and cardiovascular mortality in hemodialysis patients. Int. J. Med. Sci. 11, 726–731. doi:10.7150/ijms.8590
Sioutas, C., Delfino, R. J., and Singh, M. (2005). Exposure assessment for atmospheric ultrafine particles (UFPs) and implications in epidemiologic research. Environ. Health Perspect. 113, 947–955. doi:10.1289/ehp.7939
Sugamata, M., Ihara, T., Takano, H., Oshio, S., and Takeda, K. (2006). Maternal diesel exhaust exposure damages newborn murine brains. J. Health Sci. 52, 82–84. doi:10.1248/jhs.52.82
Suzuki, T., Hidaka, T., Kumagai, Y., and Yamamoto, M. (2020). Environmental pollutants and the immune response. Nat. Immunol. 21, 1486–1495. doi:10.1038/s41590-020-0802-6
Suzuki, T., Shibata, T., Takaya, K., Shiraishi, K., Kohno, T., Kunitoh, H., et al. (2013). Regulatory nexus of synthesis and degradation deciphers cellular Nrf2 expression levels. Mol. Cel Biol 33, 2402–2412. doi:10.1128/mcb.00065-13
Symanski, E., Davila, M., McHugh, M. K., Waller, D. K., Zhang, X., and Lai, D. (2014). Maternal exposure to fine particulate pollution during narrow gestational periods and newborn health in Harris County, Texas. Matern. Child. Health J. 18, 2003–2012. doi:10.1007/s10995-014-1446-7
Tachibana, K., Takayanagi, K., Akimoto, A., Ueda, K., Shinkai, Y., Umezawa, M., et al. (2015). Prenatal diesel exhaust exposure disrupts the DNA methylation profile in the brain of mouse offspring. J. Toxicol. Sci. 40, 1–11. doi:10.2131/jts.40.1
Takahashi, Y., Mizuo, K., Shinkai, Y., Oshio, S., and Takeda, K. (2010). Prenatal exposure to titanium dioxide nanoparticles increases dopamine levels in the prefrontal cortex and neostriatum of mice. J. Toxicol. Sci. 35, 749–756. doi:10.2131/jts.35.749
Takeda, K., Suzuki, K.-i., Ishihara, A., Kubo-Irie, M., Fujimoto, R., Tabata, M., et al. (2009). Nanoparticles transferred from pregnant mice to their offspring can damage the genital and cranial nerve systems. J. Health Sci. 55, 95–102. doi:10.1248/jhs.55.95
Takizawa, H., Ohtoshi, T., Kawasaki, S., Kohyama, T., Desaki, M., Kasama, T., et al. (1999). Diesel exhaust particles induce NF-kappa B activation in human bronchial epithelial cells in vitro: importance in cytokine transcription. J. Immunol. 162, 4705–4711.
Takizawa, H. (2004). Diesel exhaust particles and their effect on induced cytokine expression in human bronchial epithelial cells. Curr. Opin. Allergy Clin. Immunol. 4, 355–359. doi:10.1097/00130832-200410000-00005
Umezawa, M., Onoda, A., and Takeda, K. (2014). “Findings regarding the hazard assessment of nanoparticles and their effects on the next generation in PM2.,” in 5-role of oxidative stress in health effects & prevention strategy. Editors Y. J. Li, M. Umezawa, H. Takizawa, K. Takeda, and T. Kawada (New York: Nova Science Publishers), 159–175.
Ungvári, I., Hadadi, É., Virág, V., Nagy, A., Kiss, A., Kalmár, Á., et al. (2012). Relationship between air pollution, NFE2L2 gene polymorphisms and childhood asthma in a Hungarian population. J. Community Genet. 3, 25–33. doi:10.1007/s12687-011-0075-8
Volk, H. E., Lurmann, F., Penfold, B., Hertz-Picciotto, I., and McConnell, R. (2013). Traffic-related air pollution, particulate matter, and autism. JAMA Psychiatry 70, 71–77. doi:10.1001/jamapsychiatry.2013.266
von Otter, M., Landgren, S., Nilsson, S., Celojevic, D., Bergström, P., Håkansson, A., et al. (2010). Association of Nrf2-encoding NFE2L2 haplotypes with Parkinson's disease. BMC Med. Genet. 11, 36. doi:10.1186/1471-2350-11-36
Wåhlin, P., Palmgren, F., Van Dingenen, R., and Raes, F. (2001). Pronounced decrease of ambient particle number emissions from diesel traffic in Denmark after reduction of the sulphur content in diesel fuel. Atmos. Environ. 35, 3549–3552. doi:10.1016/s1352-2310(01)00066-8
Wang, L., Zhang, J., Zheng, Y., Yang, J., Zhang, Q., and Zhu, X. (2010). Bioeffects of CdTe quantum dots on human umbilical vein endothelial cells. J. Nanosci. Nanotech. 10, 8591–8596. doi:10.1166/jnn.2010.2681
Wang, X., Chen, H., Liu, J., Ouyang, Y., Wang, D., Bao, W., et al. (2015). Association between the NF-E2 Related Factor 2 Gene Polymorphism and Oxidative Stress, Anti-oxidative Status, and Newly-Diagnosed Type 2 Diabetes Mellitus in a Chinese Population. Ijms 16, 16483–16496. doi:10.3390/ijms160716483
Xia, T., Korge, P., Weiss, J. N., Li, N., Venkatesen, M. I., Sioutas, C., et al. (2004). Quinones and aromatic chemical compounds in particulate matter induce mitochondrial dysfunction: implications for ultrafine particle toxicity. Environ. Health Perspect. 112, 1347–1358. doi:10.1289/ehp.7167
Xia, Z., Duan, X., Tao, S., Qiu, W., Liu, D., Wang, Y., et al. (2013). Pollution level, inhalation exposure and lung cancer risk of ambient atmospheric polycyclic aromatic hydrocarbons (PAHs) in Taiyuan, China. Environ. Pollut. 173, 150–156. doi:10.1016/j.envpol.2012.10.009
Xiao, G. G., Wang, M., Li, N., Loo, J. A., and Nel, A. E. (2003). Use of proteomics to demonstrate a hierarchical oxidative stress response to diesel exhaust particle chemicals in a macrophage cell line. J. Biol. Chem. 278, 50781–50790. doi:10.1074/jbc.m306423200
Yamamoto, T., Yoh, K., Kobayashi, A., Ishii, Y., Kure, S., Koyama, A., et al. (2004). Identification of polymorphisms in the promoter region of the human NRF2 gene. Biochem. Biophysical Res. Commun. 321, 72–79. doi:10.1016/j.bbrc.2004.06.112
Yokota, S., Moriya, N., Iwata, M., Umezawa, M., Oshio, S., and Takeda, K. (2013). Exposure to diesel exhaust during fetal period affects behavior and neurotransmitters in male offspring mice. J. Toxicol. Sci. 38, 13–23. doi:10.2131/jts.38.13
Yokota, S., Oshio, S., Moriya, N., and Takeda, K. (2016). Social isolation-induced territorial aggression in male offspring is enhanced by exposure to diesel exhaust during pregnancy. PLOS One 11, e0149737. doi:10.1371/journal.pone.0149737
Keywords: ultrafine and nano-sized particles, oxidative stress, antioxidants, fetal development, NRF2 SNP
Citation: Li Y-J, Takeda K, Yamamoto M and Kawada T (2021) Potential of NRF2 Pathway in Preventing Developmental and Reproductive Toxicity of Fine Particles. Front. Toxicology 3:710225. doi: 10.3389/ftox.2021.710225
Received: 15 May 2021; Accepted: 27 August 2021;
Published: 13 September 2021.
Edited by:
Rosaria Meccariello, University of Naples Parthenope, ItalyReviewed by:
Yasser Said El-Sayed, Damanhour University, EgyptMohamed M. Abdel-Daim, Suez Canal University, Egypt
Copyright © 2021 Li, Takeda, Yamamoto and Kawada. This is an open-access article distributed under the terms of the Creative Commons Attribution License (CC BY). The use, distribution or reproduction in other forums is permitted, provided the original author(s) and the copyright owner(s) are credited and that the original publication in this journal is cited, in accordance with accepted academic practice. No use, distribution or reproduction is permitted which does not comply with these terms.
*Correspondence: Ying-Ji Li, li-yingji@nms.ac.jp