- Division of Diet, Disease Prevention and Toxicology, National Food Institute, Technical University of Denmark, Kongens Lyngby, Denmark
Areola/nipple retention (NR) is an established biomarker for an anti-androgenic mode of action in rat toxicity studies. It is a mandatory measurement under several OECD test guidelines and is typically assessed in combination with anogenital distance (AGD). Both NR and AGD are considered retrospective biomarkers of insufficient androgen signaling during the masculinization programming window in male fetuses. However, there are still aspects concerning NR as a biomarker for endocrine disruption that remains to be clarified. For instance, can NR be regarded a permanent adverse effect? Is it a redundant measurement if AGD is assessed in the same study? Is NR equally sensitive and specific to anti-androgenic chemical substances as a shortening of male AGD? In this review we discuss these and other aspects concerning the use of NR as a biomarker in toxicity studies. We have collected available literature from rat toxicity studies that have reported on NR and synthesized the data in order to draw a clearer picture about the sensitivity and specificity of NR as an effect biomarker for an anti-androgenic mode of action, including comparisons to AGD measurements. We carefully conclude that NR and AGD in rats for the most part display similar sensitivity and specificity, but that there are clear exceptions which support the continued assessment of both endpoints in relevant reproductive toxicity studies. Available literature also support the view that NR in infant male rats signifies a high risk for permanent nipples in adulthood. Finally, the literature suggests that the mechanisms of action leading from a chemical stressor event to either NR or short AGD in male offspring are overlapping with respect to canonical androgen signaling, yet differ with respect to other mechanisms of action.
Introduction
Over the past few decades we have witnessed an increase in male reproductive disorders such as cryptorchidism and hypospadias in new-born boys, or infertility and testis cancers in young men (Diamanti-Kandarakis et al., 2009; Skakkebaek et al., 2016). These reproductive disorders have several known genetic factors, but these cannot alone account for the growing incidence rates (Skakkebaek et al., 2001). Thus, environmental factors must also be involved and developmental exposure to endocrine disrupting chemicals (EDCs) have been suggested to play a significant role in causing reproductive disorders (Diamanti-Kandarakis et al., 2009).
With respect to male reproductive disorders, chemical substances with anti-androgenic properties such as many phthalates and pesticides are of particular concern (Gray 2001; Schwartz et al., 2019a). This is because androgen signaling plays a central role in masculinizing the male fetus and thus blocking androgen action perturbs these virilization events. This failure to become fully masculinized can manifest in different ways. One classical morphometric biomarker of fetal masculinization is the anogenital distance (AGD). Under normal circumstances AGD is approximately twice as long in males as in females and this measure can therefore be used to determine the sex of various animal species, including cats and rodents (Hotchkiss and Vandenbergh 2005), but decreased AGD can also be used as a biomarker indicating that the male fetus is generally under-masculinized (Schwartz et al., 2019a).
Another morphometric biomarker of fetal androgen action is areola/nipple retention, in this review simply referred to as nipple retention (NR). In contrast to humans where both males and females have nipples, common laboratory strains of Rattus norwegicus and Mus musculus are sexually dimorphic in the number of nipples. Whereas female rats e.g. Wistar and Sprague Dawley normally have 12 nipples (six pairs), the males normally have none (Imperato-McGinley et al., 1986). In common laboratory mice strains the ratio is 10 nipples (five pairs) in females to zero in males (Mayer et al., 2008). Assessment of NR in male rat pups involves the detection of relatively small, dark spots located along the “milk lines” in positions corresponding to where the female nipples are located. In fact, when visually assessed in neonatal offspring–optimally around postnatal day 12/13 – they are only observed as pigmented patches (areolas) rather than actual nipples. If observed under a dissecting microscope the areolae with nipple buds are visible in both infant female and treated male rats. In mice and rats, the nipple anlagen in males regress in response to androgen signaling during development (Kratochwil 1977). Low levels of androgen signaling during this developmental window–as in female fetuses–will allow for the formation of nipples also in the male offspring. NR can thus be used as a retrospective biomarker of fetal androgen action in rats, where the presence of nipples in males signifies insufficient masculinization similarly to a reduced AGD.
In this review, we provide an overview of how various chemical classes can cause NR in male rat offspring and discuss the differences between NR and AGD as biomarkers for anti-androgenicity. We review the current use of NR in regulatory toxicology (focusing solely on rat studies) and provide guidance on how to measure NR to minimize error and bias. Finally, through an extensive literature review of relevant toxicological studies in rats covering numerous chemicals, we address three crucial questions that remain to be answered in order to ensure the optimal use of NR assessment in a regulatory context: 1) Do different chemical classes have different modes of action when affecting NR and if so, how does this relate to their effects on AGD? 2) When is NR a transient effect and when is it permanent? 3) How many retained nipples can you expect in control groups? Our intention is to provide a solid reference source to ensure high quality data when assessing NR and thereby improve chemical risk assessment.
Androgen-dependent Masculinization in Mammals and the Case of Species-specific Nipple Retention
The development of the male phenotype starts with genetic determination of gonadal sex and subsequent differentiation of the testes in XY fetuses (Svingen and Koopman 2013). Once formed, the fetal Leydig cells initiate steroidogenesis which is required for synthesis of testosterone by the fetal testes. In turn, testosterone can be converted to the more potent androgen dihydrotestosterone (DHT) by the enzyme 5α-reductase in some target tissues (Scott et al., 2009). Both testosterone and DHT bind the androgen receptor (AR) to activate androgen-regulated gene transcription and direct development of male accessory sex organs and the general masculinization of the fetus (Jost 1954; MacLeod et al., 2010; Matsushita et al., 2018; Sharpe 2020). It should also be noted, that although rodents seem to rely solely on androgens synthesized by the fetus, in humans there is a “backdoor pathway” supply of androgens from the placenta (Sharpe 2020).
With respect to testosterone versus DHT, it is worth noting that tissues in close proximity to the developing fetal testis, such as the Wolffian ducts, are exposed to high concentrations of androgens and thus conversion to DHT is not required for masculinization. Conversely, tissues more distant to the testes such as the urogenital sinus (from which the prostate and other urogenital structures differentiate), the perineum, and the nipple anlagen are exposed to lower concentrations of testosterone and thus require DHT conversion for masculinization. Because of this spatial gradient of androgens during development, it is likely that peripheral sites (prostate, perineum and nipple anlagen) are more sensitive to chemicals that act on the AR, as opposed to Wolffian duct derivatives. For example, prenatal exposure to the selective AR antagonist flutamide inhibits prostate differentiation at much lower dose levels than what is required to inhibit seminal vesicle differentiation. Conversely, exposure to phthalates (by reducing testosterone production in the testis) can have a greater effect on Wolffian duct derivate than on more distant tissues such as the prostate (Mylchreest et al., 1999).
In common rats, DHT induces regression of the nipple anlagen (Kratochwil and Schwartz 1976; Kratochwil 1977; Imperato-McGinley et al., 1986). Consequently, male rats do not have nipples. The female rats, who are not exposed to high levels of androgens during the critical stages of development, usually end up having six pairs of nipples, 12 in total. These androgen mediated effects take place during a short window of fetal development (embryonic day 15.5–18.5 in rats), often referred to as the masculinization programming window (MPW) (Welsh et al., 2008; van den Driesche et al., 2012, 2017; Sharpe 2020), and depicted in Figure 1A. Androgen action during the MPW is believed to programme the male reproductive organs in both humans and rats (Welsh et al., 2008; MacLeod et al., 2010), but compared to the rodents the human MPW occurs earlier during fetal life, more precisely in the first trimester between gestation weeks 8–14 (Welsh et al., 2008; Dean and Sharpe 2013). In mammals, disruption of androgen action during the MPW perturbs normal development of the androgen dependent tissues and may manifest as reproductive disorders (Welsh et al., 2008; Sharpe and Skakkebaek 2008; van den Driesche et al., 2017), for instance NR, hypospadias and short AGD (Figure 1B). Although the androgen signaling pathway can be disrupted in different ways, from reduced testosterone synthesis to blocking of AR binding, we refer to compounds that interfere with any step of the androgen signaling pathway as anti-androgenic compounds.
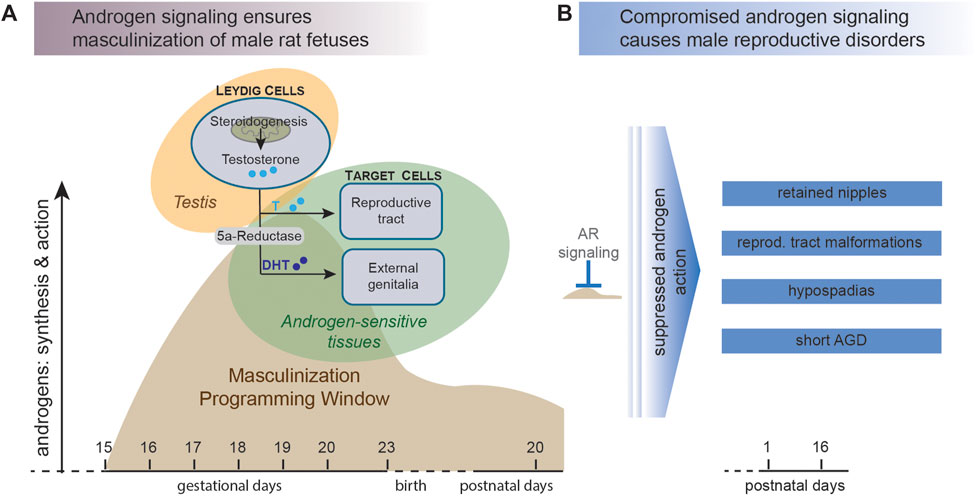
FIGURE 1. Disrupted androgen action during the male masculinization programming window (MPW) can lead to reproductive disorders in male offspring. (A) In mammals, the male fetus is acquiring male characteristics in response to androgen signaling. Testosterone is synthesized by Leydig cells in the fetal testis and then excreted into the circulation. Here, testosterone can be converted to the more potent ligand dihydrotestosterone (DHT), which activates androgen receptor (AR) in cells of the presumptive external genitalia and prompts differentiation into male reproductive tissues. The main masculinization events take place during a specific stage of fetal life denoted the masculinization programming window (MPW), which in rats is around days 15–19 of gestation (adapted from (Welsh et al., 2008). (B) Failure to initiate the masculinization differentiation events during the MPW, for instance by exposure to anti-androgenic chemicals, can lead to undervirilization of the male offspring and various reproductive disorders.
Perturbed androgen signaling during the MPW can result in NR in male rat offspring (Kratochwil 1977; Carruthers and Foster 2005) and is–similarly to AGD–considered a general biomarker of incomplete masculinization (Schwartz et al., 2019a). NR is associated with adverse effects such as hypospadias and cryptorchidism, decreased penile length and reduced seminal vesicle weight in rats (Bowman et al., 2003; Christiansen et al., 2008). Since this sexually dimorphic regression of the male nipples is occurring in rats, but not in humans, NR is not a useful biomarker in human epidemiological studies. That said, the phenomenon of nipple regression is naturally taking place in humans, albeit in both sexes (see section 8). Nevertheless, even though assessment of NR is not directly transferable to the human situation, it is a clear readout of insufficient androgen signaling during the MPW in rats. And since disrupted androgen signaling is directly relevant for adverse male reproductive health effects in humans, NR is a relevant biomarker which can be used to identify anti-androgenic chemicals and therefore an important endpoint to assess in rat toxicological studies that aim to predict endocrine disruption and adverse effects on human health.
Nipple Retention in Rat Toxicity Studies
A Compilation of Literature Reporting on NR in Rat Toxicity Studies
To provide an overview of available open-literature data pertaining to NR in toxicity studies, we carried out a search in PubMed (https://pubmed.ncbi.nlm.nih.gov/), as well as reassessing our extensive study compilations from our previous review on AGD (Schwartz et al., 2019a). Our new tables contain all of the information relevant for NR, including occurrence in control animals/groups and sensitivity of the endpoints by elucidating Lowest Observed Adverse Effect Level (LOAEL) of individual studies. Our literature searches were updated using the search strings “nipple AND retention” and “anogenital AND distance” (published until 10.02.21). We included studies if they 1) contained data on NR 2) were performed in rats and 3) reported information on single substance exposures (i.e. not studies on chemical mixtures). The full set of studies is listed in Supplementary Table S1.
We assessed the quality and strength of the results of all studies using a simple weight of evidence (WoE) approach. This included both positive and negative results so that we could draw an overall conclusion for each chemical (Table 1). The level of evidence was denoted “strong,” “moderate,” “weak” or “inconclusive” based on the following criteria. Strong: several studies indicating clear and coherent evidence in the absence of conflicts; Moderate: one or more studies showing coherent evidence; Weak: one or more studies showing clear trend or indication of evidence but not enough available data; Inconclusive: studies showing conflicting results or methodological limitations hindering evidence assessment.
How Is NR Affected by Different Chemicals?
Based on our literature review we identified 147 relevant reproductive toxicity studies in rats that have reported on the effects of various chemicals on NR in male offspring, as well as co-reporting of AGD (Supplementary Table S1). The following discussion focuses on the results from Table 1, which shows our WoE assessment of effects of each of the listed chemicals. We discuss the various chemical substances with focus on putative or suggested modes of action and how this relates to the observed adverse outcomes. An additional question is whether specific chemical classes cause different effect patterns on NR and AGD. Our aim was to examine if we, based on the collected information, could gain more insight into how chemicals induce these effects and use the information to elaborate more robust modes of action or Adverse Outcome Pathway frameworks (Villeneuve et al., 2014) to be used for chemical safety evaluations.
Compounds With a Clear Dose Related Effect on Both NR and AGD
Flutamide, vinclozolin and procymidone all have AR antagonistic activity, and all three show very marked anti-androgenic effects in vivo. Flutamide is a drug used for various medical treatments, not least prostate cancers (Miyata et al., 2002). In vitro, flutamide antagonizes the AR by preventing receptor binding of both DHT and testosterone (Simard et al., 1986). With regard to NR, studies report a clear dose-response relationship between exposure concentrations and the number of nipples (for all 14 identified flutamide references, see Table 1). Three robust studies report on a full dose-response relationship of flutamide, and the retention of 12 nipples in male offspring at doses between 16 and 50 mg/kg bw/day administered during the MPW (McIntyre et al., 2001; Hass et al., 2007; Schreiber et al., 2020). At around 2 weeks of age, these male offspring were practically indistinguishable from their female littermates as regards the presence of nipples. Another study on flutamide exposure in the same dose range (40 mg/kg bw/day) reported only six retained nipples in males (You et al., 1998). It is unclear why only six retained nipples were observed in this study, but regardless there is no doubt that flutamide exposure results in clear effects on NR in rats.
Exposure to the fungicides vinclozolin and procymidone also give rise to dose-dependent NR in male rat offspring (see Table 1). Both chemicals display AR antagonism in vitro (Kelce et al., 1994; Nellemann et al., 2003; Kleinstreuer et al., 2017; Scholze et al., 2020) and give strong effects on NR in vivo. Vinclozolin induces NR with a clear dose-response relationship and, like flutamide, can induce retention of all 12 nipples in prenatally exposed males. This response is achieved at doses around 200 mg/kg bw/day (Wolf et al., 2004; Hass et al., 2007), whereas statistically significant effects on NR start occurring from around 5–10 mg/kg bw/day (Ostby et al., 1999a; Hass et al., 2007). Likewise, procymidone causes a clear dose-response for NR (Hass et al., 2007, 2012) and induces retention of all 12 nipples at a dose of 150 mg/kg (Hass et al., 2007).
Flutamide, vinclozolin and procymidone also induce shorter AGD in male rat offspring and in several studies male AGD decreased to (almost) resemble female AGD (Gray et al., 1994; You et al., 1998; Hellwig et al., 2000; McIntyre et al., 2001; Wolf et al., 2004; Hass et al., 2007). As discussed in more detail in section 5, NR and shortening of AGD in male offspring start manifesting at similar dose levels.
Certain phthalates can induce marked anti-androgenic effects in vivo (Table 1). However, phthalates are not as potent in inducing NR as potent AR antagonists and the 5-alpha reductase inhibitors. Instead, phthalates are typically considered to act as anti-androgens by reducing fetal testosterone levels (or by inhibiting testosterone biosynthesis) as this effect is measurable in vivo. Thus, it can be potentially illuminating to scrutinize the effect patterns of NR and AGD relative to mechanisms of action and to see if specific modalities are more potent at inducing adverse outcomes than others.
A thorough analysis of the available literature (Table 1 and Supplementary Table S1) reveals that a large percentage of the male pups present with NR within the single studies, but individual male pups rarely have a high number of retained nipples. There are exceptions, however, with diethylhexyl phthalate (DEHP) and dibutyl phthalate (DBP) inducing a high number of retained nipples at high exposure doses. A few studies on DEHP report up to 8–10 retained nipples in individual pups at doses of 750–1000 mg/kg DEHP (Wolf et al., 1999; Moore et al., 2001), even though the majority of DEHP studies find a much lower mean number of retained nipples (Jarfelt et al., 2005; Howdeshell et al., 2007; Gray et al., 2009). A high dose of 500 mg/kg DBP has been reported to induce up to 10 retained nipples in a single study (Barlow et al., 2004), but as with DEHP, the majority of studies report between 1 and 5 retained nipples even at high doses (Wolf et al., 1999; Howdeshell et al., 2007; Martino-Andrade et al., 2009). This effect pattern–low number of retained nipples in individual pups–is also evident for other anti-androgenic phthalates such as benzyl butyl phthalate (BBP) (Hotchkiss et al., 2004; Tyl et al., 2004), di-isononyl phthalate (DiNP) (Boberg et al., 2011), di-isobutyl phthalate (DiBP) (Saillenfait et al., 2008), Dicyclohexyl phthalate (DCHP) (Yamasaki et al., 2009), di-isoheptyl phthalate (DiHP) (McKee et al., 2006) and di-n-hexyl phthalate (DnHP) (Saillenfait et al., 2009). However, overall the phthalates may appear to cause less marked effects on both NR and AGD than the above mentioned chemicals, because the doses eliciting a very potent anti-androgenic response cannot be administered due to maternal toxicity. An additional explanation for the less marked effects of phthalates on AGD and nipple retention is that the phthalates disrupt androgen-regulated male sexual differentiation without interacting directly with the AR, as does e.g. flutamide (Mylchreest et al., 1999).
The above-mentioned phthalates typically induce shorter male AGD in the range of 6–34% reductions compared to control at exposure concentrations similar to those inducing NR. In other words, anti-androgenic phthalates seem to elicit effects on NR and AGD at comparable doses (Table 1).
Pyrifluquinazon (PFQ), a new active ingredient insecticide, has been tested in one study investigating its effects both in vitro and in vivo (Gray et al., 2019). PFQ showed weak AR antagonistic effects in vitro but surprisingly this compound elicited a very potent NR response in vivo with maximal NR around 9–10. This response was seen at a dose of 100 mg/day, a dose which caused a non-significant reduced maternal weight gain during dosing. PFQ had a dose-dependent but less marked effect on AGD which was maximally decreased by 33%.
Linuron is a well-characterized anti-androgenic herbicide that can antagonize the AR in vitro, but also inhibit the synthesis of androgens such as testosterone and androstenedione (Scholze et al., 2020). The performed in vivo studies show decreased testosterone levels indicating that this compound could also affect steroidogenesis in vivo. The maximally observed number of nipples in linuron exposed male offspring was 3.7 at 50 mg/kg (McIntyre et al., 2000). The effect on NR was much weaker than for PFQ (Gray et al., 2019), whereas the maximum effect on AGD (31% shorter) was comparable to PFQ. The dose levels of linuron that caused the maximum effects on NR and AGD also caused a marked degree of maternal and developmental toxicity, which prevented higher doses from being tested.
Dichlorodiphenyldichloroethylene (p,p’-DDE) is the main metabolite of the insecticide DDT and is an AR antagonist that inhibits AR-dependent gene expression in vitro (Kelce et al., 1995, 1997). This insecticide metabolite has been examined in several in vivo rat studies, with somewhat conflicting results. DDE induced a maximum NR around 4.8 (Loeffler and Peterson 1999), whereas a relatively small maximum effect on AGD of 14% was reported in one of the studies (You et al., 1998).
Together, the results from these AR antagonists indicate that in vitro knowledge alone is not sufficient to predict the in vivo potency on NR and AGD. This can probably be explained by the different toxicokinetic properties of these AR antagonists in vivo; important data that could increase the predictive power of in vitro data in the future.
Finasteride Has Shallow Dose Response Curves With Effects on Both NR and AGD
Finasteride, like flutamide, is an anti-androgenic drug used for prostate cancer treatment (FDA 2011). Rather than antagonizing the AR, however, finasteride works by inhibiting the enzyme 5α-reductase, thereby blocking the conversion of testosterone to DHT (Clark et al., 1990). Since DHT is the main androgen responsible for inducing regression of nipples in male laboratory rats, it is not surprising that finasteride exposure can induce NR. In fact, finasteride has been shown to be very potent in vivo, at very low doses of only 0.01 mg/kg bw/day (Bowman et al., 2003; Christiansen et al., 2009) resulting in statistically significant NR, while doses from 0.1 and up to 100 mg/kg result in 8–12 nipples in male offspring (Bowman et al., 2003; Christiansen et al., 2009; Martínez et al., 2011). Finasteride also has a potent effect on male AGD in rats, but notably, maximal decreases were 38% (Clark et al., 1990) and thus not as marked as the 50% observed for the AR-antagonists flutamide, vinclozolin and procymidone (Gray et al., 1994; Ostby et al., 1999b; Hellwig et al., 2000; McIntyre et al., 2001; Schreiber et al., 2020). It is also noteworthy that finasteride is one of the few identified compounds that can induce 10–12 nipples and shorten AGD to almost female length in male rats without causing other signs of toxicity. Only flutamide, vinclozolin, procymidone and finasteride seem to follow this pattern of complete demasculinization with almost no signs of concomitantly reduced body weights, increased mortality or effects on other target organs such as liver and kidney.
Compounds With Clear Effects on NR and No, or Minor, Effects on AGD
Azole fungicides have a somewhat perplexing effect pattern on NR versus AGD. Azole pesticides such as prochloraz, tebuconazole and epoxiconazole all seem to cause a consistent (yet relatively small) increase in NR, but without a significant effect on male AGD. Many azoles seemingly perturb endocrine signaling by disrupting steroidogenesis by inhibiting CYP enzyme activities and can alter steroid hormone concentrations both in vitro and in vivo as reviewed by (Dreisig et al., 2013).
Prochloraz can inhibit steroidogenesis and antagonize the AR in vitro (Vinggaard et al., 2005, 2006). Effects on steroidogenesis are also evident in vivo with testosterone output by fetal testis being reduced (Blystone et al., 2007). In utero exposure to prochloraz can affect the development of several androgen-sensitive tissues (Vinggaard et al., 2002, 2005; Laier et al., 2006), but it is unclear if these effects are a direct consequence of reduced testosterone levels, antagonistic effects on the AR, or a combination of the two. With the exception of one study reporting shorter male AGD and increased NR at 50 and 150 mg/kg doses (Laier et al., 2006), most studies on prochloraz find no effect on AGD following in utero exposure at dose between 25 and 150 mg/kg, yet significant effects on NR (Noriega et al., 2005; Vinggaard et al., 2005; Christiansen et al., 2009; Melching-Kollmuss et al., 2017). This effect pattern is puzzling when viewed against other compounds with similar in vitro activities and may be the result of a mechanisms of effect yet to be appreciated or complex in vivo toxicokinetics.
Tebuconazole is another azole fungicide that induce NR without a significant effect on male AGD. In utero exposure to tebuconazole induces a maximum average effect of 1.6–3.1 retained nipples at 50–100 mg/kg bw/day (Taxvig et al., 2007; Hass et al., 2012), but without a significant shorter AGD. To complicate the matter further, some studies on tebuconazole indicate longer female AGD among littermates exposed to the same doses (Taxvig et al., 2007; Hass et al., 2012).
Epoxiconazole is a third azole fungicide showing an effect pattern where male pups display increased NR but without an effect on AGD. NR in developmentally exposed male pups show a dose-dependent effect pattern and a maximal number of nipples of around 3.4 at doses of 50 mg/kg bw/day and without an effect on male AGD (Taxvig et al., 2007; Hass et al., 2012). As was the case with tebuconazole, epoxiconazole can also induce longer female AGD (Taxvig et al., 2007; Hass et al., 2012). The mode of action for this effect on female AGD is unknown, but could indicate an androgenic mode of action based on our knowledge about hormone-dependent sexual differentiation.
The mild analgesic paracetamol has been suggested to have endocrine disrupting properties (Kristensen et al., 2016). Only one study has investigated the effects on NR (Axelstad et al., 2014). Here, exposure to 360 mg/kg paracetamol induced 30% NR in male offspring but did not affect AGD. By contrast, one study in rats and one study in mice have found that 150 mg/kg paracetamol induced 10–15% shorter AGD in exposed males, but unfortunately NR was not assessed in these studies (Kristensen et al., 2011; Holm et al., 2015), which prevents further comparisons.
Nitrotriazolone (NTO) is a constituent of explosive formulations that, with respect to reproductive toxicity, may fall into the same category as the three above-mentioned azole fungicides. One study has investigated the reproductive toxicity of NTO and find no effects on male AGD, yet small effects on NR at doses from 144 to 3600 mg/L administered in the drinking water (Lent et al., 2016). The mode of action for NTO is unclear as it does not induce marked androgenic or estrogenic effects in the Hershberger and utero trophic assay at doses from 250 to 1000 mg/kg (Quinn et al., 2014).
Compounds With No/minor Effects on NR but Effects on AGD
Bisphenol A (BPA) and butyl paraben are both compounds that are considered to be mainly estrogenic in their ED mode of action (Routledge et al., 1998; Kim et al., 2001; Matthews et al., 2001; Byford et al., 2002), albeit they also display anti-androgenic properties in vitro (Chen et al., 2007; Rosenmai et al., 2014). Since NR is considered to be caused by an anti-androgenic mode of action, there are not many studies that have assessed NR in rodent toxicity studies with estrogenic chemicals. One study reports a small effect on NR (mean of 0.4 nipples) following exposure to 50 mg/kg BPA (Christiansen et al., 2014). By contrast, four other studies found no effect on NR (Tyl et al., 2002; Howdeshell et al., 2008; Ferguson et al., 2011) even at doses as high as 300–500 mg/kg (Tyl et al., 2002; Delclos et al., 2014), which was also the case with the structural analogue bisphenol C (BPC), with no effects on either NR or AGD at doses of 100 and 200 mg/kg (Gray et al., 2019).
Only one study has investigated if butyl paraben can induce NR and found no effects after exposure to doses of 10–500 mg/kg (Boberg et al., 2016). Interestingly, studies with BPA and butyl paraben have both shown small, but significant, effects on AGD (Christiansen et al., 2014; Boberg et al., 2016), which could suggest that AGD, and not NR, may be regulated by an androgen-estrogen balance, perhaps similarly to what is the case for the genital tubercle which is sensitive to estrogen-androgen balance (Yucel et al., 2003; Yang et al., 2010; Mattiske and Pask 2021). Indeed, studies have shown that the perineal tissues express the estrogen receptor (ER) (Dionne et al., 1979; Schwartz et al., 2019b) and one study shows that polymorphisms in the gene encoding the ER are associated with short AGD in human boys (Sathyanarayana et al., 2012). This might suggest that AGD is a more sensitive endpoint than NR for chemicals with an estrogenic mode of action. However, the much more potent diethylstilbestrol (DES) has been shown to induce some effects on both NR and AGD (Johansson et al., 2021). In this latter study, rats exposed to DES at doses ranging from 0.003 to 0.0012 mg/kg resulted in a mean of 0.4–0.5 retained nipples. Only in the lowest dose group was a small, but statistically significant, effect observed on the male AGD. Thus, further investigations are needed to fully understand the effects of estrogenic compounds on NR and AGD, as well as to explore the possibility of the “estrogenic compounds” also inhibiting androgen production.
Compounds With Miscellaneous Effects on NR
We identified 10 additional chemicals that display endocrine modes of action in vitro and induce NR in rat offspring. The pattern of effects of these compounds on NR and AGD in developmental toxicity studies were rather variable. It is also worth noting that, for most of them, only one study is available, which complicates the WoE evaluation. For instance, the three pesticides fludioxonil, cyprodinil and dimethomorph have all been shown to have AR antagonistic activity in vitro; cyprodinil also showed AR agonistic activity at low concentrations (Orton et al., 2011). When tested in vivo, cyprodinil and fludioxonil had no effect on NR at doses between 20 and 180 mg/kg (Scholze et al., 2020). Dimethomorph was tested twice in the same study at doses of 6.7, 20, 60 and 180 mg/kg, with data showing conclusive effects on NR at 180 mg/kg (Scholze et al., 2020).
Ketoconazole is another azole fungicide where both AGD and NR have been assessed in developmental rat toxicity studies. In one study, developmental exposure to 12–50 mg/kg ketoconazole did not induce any effects on AGD or NR (Wolf et al., 1999). In contrast, a second study using doses between 3 and 12 mg/kg observed statistically significant reduced AGD (∼2% at all doses) and increased NR (mean for NR ranging from 0.3 to 1 nipple) in male offspring (Johansson et al., 2021). With the effects in the second study being very small, albeit statistically significant, some caution should be made when interpreting data, especially across studies. However, these clear discrepancies between exposure concentrations and anti-androgenic effects warrant further investigation.
The fungicide mancozeb induced a small, but significant effect on NR at a dose of 25 mg/kg (Hass et al., 2012). The same was seen for perfluorohexane sulfonate (PFHxS) (Ramhøj et al., 2018). The polychlorinated biphenyl (PCB) 126 was tested at a maximal dose of 1 μg/kg, but the NR results were not reported (Rice 1999). One study investigating the effects of the insecticide fenitrothion at doses of ∼1–8 mg/kg finds no effects on NR (Okahashi et al., 2005), while another using doses of 5–25 mg/kg finds 4.1 nipples in male offspring at 25 mg/kg (Turner et al., 2002). It should be noted, however, that in the latter study 20–25 mg/kg also induced maternal toxicity and pup mortality. Similarly, 1.2 nipples were observed in males exposed to 62.5 mg/kg of the cholesterol lowering drug simvastatin, but this was based on only few litters and this dose induced high pup mortality (Beverly et al., 2019).
Compounds That Have Only Been Investigated once, Where No Effects Were Found on NR or AGD
Eighteen chemicals had only been investigated in one study, and here no effects were found on NR and AGD. These will not be discussed further here, but can be found in Table 1 and in Supplementary Table S1.
Modes of Action for Nipple Retention
It is notable that several of the compounds blocking DHT-mediated AR signaling induce a higher number of retained nipples than for instance compounds only reducing testosterone concentrations. These potent anti-androgenic chemicals are finasteride, which blocks the conversion of testosterone to DHT by inhibiting 5-α-reductase, and the three AR antagonists: flutamide, vinclozolin and procymidone. Phthalates, on the other hand, which are known to decrease testosterone levels in vivo, tend to cause NR with a smaller effect size (i.e. lower number of nipples per affected male). This alone could suggest that chemicals directly affecting the synthesis of DHT or ligand-activation of the AR have a greater impact on nipple regression than the synthesis of testosterone. Therefore, although testosterone is an essential precursor for DHT production, it seems reasonable to surmise that it is the local concentration of DHT and its ability to activate AR that determines the overall effect on NR in male offspring. The same cause-effect relationship is seen in other tissues, for instance the prostate. Here, testosterone can only partly compensate for the absence of DHT in driving differentiation of the prostate, whereas the absence of both testosterone and DHT completely abolishes prostate differentiation (Imperato-McGinley et al., 1992). However, not all data supports this hypothesis, as some AR antagonists such as linuron and DDE induce a low number of nipples (You et al., 1998; Loeffler and Peterson 1999; McIntyre et al., 2000; Hotchkiss et al., 2004). This is also the case for fludioxonil, cyprodinil and dimethomorph, albeit the cause-and-effect relationship is much less clear for these chemicals as they have only been tested in vivo in one study (Scholze et al., 2020).
Phthalates with anti-androgenic properties are often considered to act by inhibiting steroidogenesis which results in reduced fetal testosterone levels in vivo. However, the effects caused by phthalate exposure are probably much more complex. In vitro, many phthalates appear to cause reduction in testosterone concentrations by activating aromatase with subsequent up-concentration of estrogens, in essence shifting the testosterone/estrogen balance (Lee et al., 2019). Notably, the endocrine disrupting effects of phthalates are not readily predicable from the in vitro effects (Lee et al., 2019), which may be due to any number of reasons, including absorption, distribution, metabolism and excretion (ADME), but also the fact that phthalates can cause other adverse effects in cells and tissues at doses where endocrine disruption is observed in vivo. For example, phthalates can induce apoptosis of germ cells in mice and rats (Borch et al., 2005; Zhang et al., 2014) and, perhaps more importantly, disrupt Leydig cell integrity in fetal rodent testes (Borch et al., 2005, 2006; Howdeshell et al., 2007; Hannas et al., 2011; Di Lorenzo et al., 2020). Even though the exact mechanism by which phthalates reduce testosterone concentrations in vivo remains unclear, the effect of phthalates on NR is most likely mediated by sub-optimal concentrations of testosterone and not by AR antagonism. This may be why most phthalate exposures result in few retained nipples in individual male pups, and only result in some effect on male AGD. In other words, and as discussed previously, reduced testosterone levels in tissues peripheral to the testes may be partially compensated for by local conversion into sufficient levels of DHT. The observation that NR seems to be more responsive to the DHT-AR signaling axis than AGD, however, is more difficult to explain, even if the AGD should be more sensitive to the testosterone-estrogen balance than NR. Regardless, these data suggest that even significantly reduced concentrations of circulating testosterone during the MPW would be enough for the nipples to regress, as it would still provide enough substrate to produce nearly adequate concentrations of DHT in target tissues such as the nipple anlagen. As can be seen in Table 2, the majority of phthalate studies report equal sensitivity of AGD and NR.
Based on the available literature pertaining to NR in reproductive toxicity studies in rats, and our knowledge about normal reproductive development, it is feasible to elaborate a putative AOP network for NR. This causal pathway relies on the fact that early rodent studies have unraveled the essential role for the DHT-AR signaling axis in preventing nipples from developing in male rats and mice, as well as the fact that available toxicity studies suggest strongest undervirilization effects (NR) with chemical substances that are either potent AR antagonists or prevent the synthesis of DHT. Inhibition of testosterone synthesis itself can also result in NR, but not to the same degree as through the aforementioned mechanisms. Figure 2 depicts a putative adverse outcome pathway (AOP) network for NR in male rat/mouse offspring that we are confident holds the potential to be elaborated into quantitative AOPs in the not so distant future.
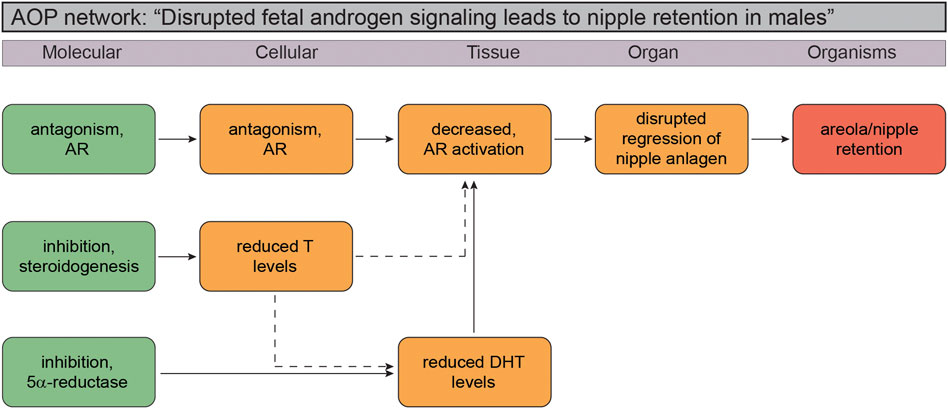
FIGURE 2. Putative Adverse Outcome Pathway (AOP) network for disrupted androgen signaling leading to nipple retention in male rodent offspring. The small AOP network suggest the molecular initiating event (MIE) can be any of the three vital stages of the androgen signaling axis: testosterone biosynthesis (“inhibition, steroidogenesis”), failure to convert testosterone to dihydrotestosterone (DHT; “inhibition, 5
It is still not possible to paint a clear picture of a cause-effect relationship from a molecular initiating event to NR, or a “one-size fits all” AOP. Most problematic are incorporation of effect patterns seen with for instance azoles, of which many are known to be potent CYP enzyme inhibitors and thus capable of disrupting steroidogenesis and testosterone production. The challenge is that many of these chemicals also display other mechanisms of action, for example antagonizing the AR. Without even considering the complex issue of ADME of chemicals in vivo relative to in vitro test assays, multiple mechanisms by single chemicals make it extremely difficult to elaborate naïve cause-effect pathways in complex biological systems. This also extends to the phthalates, where a mechanism of action is assumed rather than confirmed, which makes interpretations of effect data problematic.
Sensitivity of NR Assessment Compared to That of AGD Measurements
Table 2 summarizes existing toxicological studies that have assessed both AGD and NR in rat toxicity studies. 53 studies had sufficient data to allow for comparison of the lowest dose at which a statistically significant effect was found for each of the two endpoints. For each chemical, studies reporting effects on AGD at a lower dose than NR are listed under the “AGD” column, whereas those reporting an effect on NR at a lower dose than AGD are listed under the “NR” column. Studies where effects were seen at the same dose are grouped under the “Equal” column. Thus, the lowest adverse effect level (LOAEL) was used to determine the sensitivity of NR versus AGD assessment in developmental toxicity studies. As an added note, direct comparisons between the endpoints NR/areolae and AGD is challenged by the fact that the former is more subjective in nature than the latter. We nevertheless find the comparison relevant, and the value will increase in the future with more standardized protocols for NR/areola measurements.
Overall, studies found effects on NR at lower doses than effects on AGD in ∼38% of cases (20/53 studies), AGD was most sensitive in ∼15% of cases (8/53 studies), whereas the two effect endpoints were affected at the same doses in ∼47% of cases (25/53 studies). Taken together, the table shows a varied picture with somewhat limited concordance for each substance. However, as expected, it does identify compounds that generally affect only one of the two endpoints. By reasoning, the endpoint that these compounds affect is more sensitive than the endpoint they do not affect. This includes the azole fungicides tebuconazole and prochloraz which predominantly affect NR, and BPA and butyl paraben which predominantly affect AGD (Table 2). Furthermore, it appears plausible that the four potent anti-androgenic compounds that either antagonize AR (flutamide, procymidone and vinclozolin) or prevent the synthesis of DHT (finasteride) in most cases affect NR and AGD at the same doses. This is the case for flutamide and procymidone whereas the data on vinclozolin and finasteride is more inconclusive. For vinclozolin, some of the disparities can be due to dose choice in the studies, where large spacing between doses makes LOAEL estimations less accurate (see underlying data in Supplementary Table S1). Some of the studies investigating the effects of finasteride use doubtful methods to assess AGD and NR or inadequate statistical analysis. For the majority of the remaining compounds the number of studies for each compound is too limited to draw well-founded conclusions.
Taken together, both NR and AGD can provide the critical endocrine mediated effect and give improved understanding of the underlying mechanism. As such, we would like to stress the importance of performing both assessments in order to obtain the most complete information from every study.
Areola/nipple Retention - A Permanent or Transient Effect in Rats?
There is an ongoing debate whether or not NR measured in 2-week old rat offspring is a transient or a permanent effect. Some rat toxicity studies have reported on NR at PND12/13, but with retained nipples no longer visible a week later (Melching-Kollmuss et al., 2017). However, a sizeable number of other studies suggest NR to be permanent. This distinction between a transient and a permanent effect is important from a regulatory perspective, since only a permanent effect will be categorized as a malformation according to an OECD guidance document (OECD 2008).
Of the 147 studies included in this review, 65 have assessed NR later than PND 11–18. Out of these 65 studies, 14 studies did not see NR in infant males and were thus not included in our overall evaluation of whether NR is a permanent or a transient effect. For this analyses, the remaining 51 studies were included and are listed in Table 3.
As evident from Table 3, the majority of studies support the view that NR is generally a permanent effect, with 41 out of 51 studies (∼80%) reporting permanent nipples in mature male rats. Only 10 out of the 51 studies (∼20%) found no effect at the later time point (Supplementary Table S2). Notably, several studies deal with the same chemical, but there are still 15 different substances representing various mechanisms of action. Again, the potent anti-androgens flutamide, vinclozolin and procymidone generally show permanent effects (17 of 19 studies). One study on flutamide finds transient NR but uses a short, and late (GD18), exposure window (Foster and Harris 2005), which suggests that the androgen-dependent regression of the nipples is almost completed before GD18 in rats and not that the effect is transient. In the single study of vinclozolin that does not find permanent NR it is not specified if the male offspring were shaved before assessment (Flick et al., 2017). If they were not, this would likely have impaired the ability to assess permanent nipples, as shaving the abdomen is usually necessary in order to visually observe NR in mature rats.
Even for the phthalates (where the frequency of male pups presenting with NR is relatively high but the number of retained nipples are relatively low in each individual pup) the vast majority of studies report on permanent nipples. This includes BBP (two of two studies), DBP/DiBP (seven of nine studies), DEHP (four of four studies), DiNP (one of one study) and DnHP (one of one study). With regard to the DBP studies reporting transient NR, it is notable that permanent nipples were observed after exposure on GD16 and GD17, but not after exposure on either GD15-16 or GD17-18 (Carruthers and Foster 2005). Thus, as previously mentioned for flutamide, this lack of “permanent effect” may come down to exposure period. After exposure on GD15-16, around 3% of the males were found to have permanent nipples, albeit not statistically significant, suggesting that the main window of sensitivity for phthalate-induced permanent NR is GD16-17. For most reproductive toxicity studies, however, the exposure period spans the critical window for when nipples should normally regress in males.
There are several other compounds with anti-androgenic properties that induce permanent nipples in rats following in utero exposure. This includes linuron (Wolf et al., 1999; McIntyre et al., 2001; Hotchkiss et al., 2004), DDE (Wolf et al., 1999), and prochloraz (Noriega et al., 2005). Prochloraz has also been reported to cause only transient NR (Melching-Kollmuss et al., 2017). However, the study reporting transient NR has limited sensitivity as the males were seemingly not shaved before assessment. The AR antagonist fenitrothion did not induce permanent nipples (Turner et al., 2002). The 5α-reductase inhibitor finasteride has induced both permanent and transient NR (Clark et al., 1990; Imperato-McGinley et al., 1992; Bowman et al., 2003; Martínez et al., 2011). Notably, three out of six finasteride studies do not find permanent nipples, but different exposure periods and dose levels were used in these three in vivo studies which makes comparisons difficult (Clark et al., 1990).
Taken together, the majority of studies support that NR is a permanent effect. The data also highlights that the validity of NR data is dependent on proper measurement and recording, which will be discussed in more detail in the following sections.
NR in Regulatory Toxicology and Recommendations for Proper Measurements in Rodent Studies
NR in International Test Guidelines
The ectopic expression of nipples/areolae in infant male offspring is included as a mandatory endpoint in several OECD test guidelines (TGs) for development and reproductive toxicity. NR is assessed in all offspring (male and female) at PND 12–14 under the two screening studies for reproductive toxicity (TG 421 and TG 422) (OECD 2016a, b), as well the extended one-generation study (TG 443) (OECD 2018). NR is, however, not included in the test guideline for the current two-generation study (TG 416) (OECD 2001), but requested by EU member states as a default endpoint when the two-generation study is conducted instead of the OECD TG 443 study to address endocrine evaluations (EFSA, 2020). NR can be used in chemical risk assessment to set the NOAEL as stated in the OECD guidance document 151, which guides the interpretation of TG 443 (OECD 2013): “A statistically significant change in nipple retention should be evaluated similarly to an effect on AGD as both endpoints indicate an adverse effect of exposure and should be considered in setting a NOAEL.”
Assessment of permanent nipples in mature males is not specifically mandated in any OECD TG, but only suggested indirectly in that abnormalities should be recorded at necropsy. It is our distinct impression that permanent nipples are rarely, if ever, recorded in TGs studies. Although we are not advocating that adult NR should be mandated in all studies, we hope that our discussion can promote the recording of NR in mature males when relevant in future studies and thereby help us improve on our knowledge pertaining to NR for the sake of improving its utility as a continued in vivo biomarker.
Practical Considerations for Assessing NR in Rats
Assessment of NR in male rat pups can be highly subjective since it involves the detection of relatively small, dark spots located along the “milk lines” in positions corresponding to where the female nipples are located (Figure 4). As previously mentioned, at early developmental stages they are only pigmented patches (areolas) rather than actual nipples. Consequently, the number of nipples that are recorded–including in control males–may be influenced by factors such as age of the pups at assessment, the quality of the light source, and the experience of the assessor.
When examining NR, the areolas/nipples could be reported either as a qualitative (binominal) yes/no answer or quantitatively by counting the number of nipples. In OECD GD 151 (OECD 2013) it is recommended to provide the quantitative count as a qualitative assessment gives a low statistical power. A qualitative assessment may therefore be rather insensitive, particularly when the incidence of retained nipples in control animals is high.
The presence of nipples/areolas must be assessed at correct developmental stages. Areolas are only visible on the skin and therefore not identifiable as the pups start to grow fur. On the other hand, assessing nipples/areolas at early time points, for instance at PND4, is not feasible since the nipples are not developed enough to be identified (OECD 2015). As a result, OECD TGs stipulate that NR should be assessed in males when nipples are visible in their female littermates; that is, at PND12-14 depending on the rat strain. When assessing more mature pups for permanent nipples, it is important to shave the abdomen of the animals as fur makes it difficult, if not impossible, to properly detect the presence of nipples at this age, especially if they present as areolas rather than fully developed nipples. Importantly, all pups within the same study should be evaluated on the same days to account for differences in maturation.
In addition, actual “criteria” for a positive score may differ between labs and assessors, a fact that can make direct comparisons between studies problematic. This is also why, nipples/areolas should ideally be assessed by the same assessor for each study, and this person(s) should be blinded to exposure group. Swapping between assessors or knowing the exposure group of the investigated offspring could inadvertently skew the data. For CROs performing guideline studies for developmental and reproductive toxicity these criteria may be challenging or even impossible to adhere to, due to logistical considerations. When this is the case, we recommend that the performing laboratories arrange training sessions for the technical personnel, in order to aligning their assessments as much as possible. The same issue extends to AGD measurements, where it is critical that measurement are performed the same way for each animal to avoid “technical variations” that could obscure subtle changes between study groups.
NR in Control Animals
It would be easy to assume that male control animals always have 0 (zero) nipples. It is, however, not that simple. Retained areolas/nipples are sometimes observed in control male rats and assessment of NR in control animals (as well as knowledge of the number of nipples one can expect to find in control animals) is imperative for assay validity and accurate interpretation of NR data from toxicological studies. In other words, the establishment of a reliable number of areolas/nipples in control animals is important for the detection of substance-induced effects.
An example of how this can be an issue can be gleaned from a large generation study on oxybenzone published by NTP in US (https://tools.niehs.nih.gov/cebs3/views/?action=main.dataReview&bin_id=14485). Here, close to nine hundred male rats were assessed for NR at PND 13. Despite the large number of animals, no nipples were seemingly found in any of the pups from either control or exposed groups. This finding is surprising, since some degree of NR is expected even in control males. This result–zero areolas/nipples in control males–brings into question whether the assessment of NR was performed in accordance with good practices or if “good practices” for measuring NR in infant male rats is in itself sufficiently standardized. To further investigate the “expected background NR rate,” we analyzed an extensive database with NR data from our own animal experiments performed between 2003 and 2019. Based on 35 control groups (Table 4), we find that the control values are 0 in only three out of 35 studies (8.6%), suggesting that control values of 0% are uncommon. It should be noted that these studies were mainly performed with Wistar rats, but in several recent studies with Sprague-Dawley rats. Comparison of the control values for these two rat strains commonly used in toxicological tests shows quite similar values for both mean number of NR and for % pups with NR (Table 4).
To further substantiate our concern, we also examined all of the reported control levels in the studies shown in Supplementary Table S1, giving a total of 80 male control groups. Out of the 80 control groups, 54% report a control value of 0% (Figure 3A). Again, we find this to be surprisingly high based on personal experience. The picture becomes somewhat different when control values are reported as a number rather than as percentage (Figure 3B). In this case, 20% of 43 studies show zero nipples in male controls. This difference could be coincidental, but might also indicate that personnel trained to count NR are superior at detecting NR than those who only score a yes/no response.
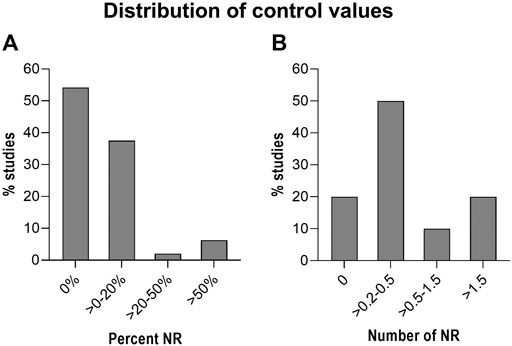
FIGURE 3. Distribution of Nipple retention (NR) in control rats/groups. Control rats/groups from the 80 studies in Supplementary Table S1. (A): Results shown as percent NR in the control groups. (B): Results shown as number of nipples in the control groups.
Studies reporting very high control levels may also raise concern as this could mask any treatment related effects. A total of 48 studies reported the control values as % positives (i.e. as a yes/no answer). The majority of these studies (∼92%) report values below 20% (Figure 3A). Only three out of the 48 studies report control values above 50% (Fussell et al., 2015; Flick et al., 2017; Melching-Kollmuss et al., 2017). These three studies on prochloraz, vinclozolin and flutamide are from the same industry group and report control values of 65–67%, which are unusually high for control groups. This calls into question the sensitivity of the testing. In such cases we recommend to scrutinize the criteria used for scoring positives or for counting areolas/nipples. For instance, the prochloraz study reports an increase in NR at PND 12 and the authors argued that “the historical background incidence of retained nipples/areola is high at the observation time point PND 12 and is observed in percentages up to 70% in untreated controls, raising some doubts on the treatment relationship of this finding” (Melching-Kollmuss et al., 2017). Notably, a similar argument is not used in the studies on flutamide and vinclozolin, where a similarly and unusually high control values are reported (Fussell et al., 2015; Flick et al., 2017).
Overall, given both the published data (Figure 3) and the calculations based on our own raw data (Table 4), a control value around 10–20% or a nipple count ranging from larger than zero to around 0.5 appears to be most common for control males. This raises concern for the validity of larger studies reporting a control value of 0% or 0.0; especially in cases where this is seen in several studies and where no exposure-related effects are found. In such cases, we recommend to perform a positive control study focusing on doses inducing low, but significant NR/areolas. A more comprehensive overview of historical control data could be achieved by including unpublished data from CRO/Industry for this endpoint, routinely collected in OECD TG 443, 421 and 422 studies.
Supernumerary Nipples in Humans–Any Relevance to Endocrine Disruption?
Just like other mammals, humans possess a rudimentary mammary ridge, or “milk lines,” running along the front torso from the arm pits (axilla) to the upper medial side of the thighs (Figure 4). They first appear between gestational week 4 and 5, but will regress in a caudal to cranial direction later in development (Leung and Robson 1989). Humans typically have two nipples, regardless of sex, that are located at the fourth rib and will form the pectoral breasts. In some individuals, regression of the nipple anlagen is not complete which may results in supernumerary (ectopic) nipples, or polythelia. A single extra nipple is most common, but multiple extra nipples may also occur. The “third nipple” is most frequently located along the mammary ridges and often present between the pectoral nipple and the naval (umbilicus); albeit, excess nipples can appear in other places such as face, neck, vulva and thighs (Leung and Robson 1989; Brown and Schwartz 2003). Supernumerary nipples are surprisingly common and occur in 0.2–6% of the population, with prevalence varying greatly amongst geographic regions and ethnicities (Mimouni et al., 1983; Leung and Robson 1989; Brown and Schwartz 2003). The etiology of supernumerary nipples, however, remains poorly characterized.
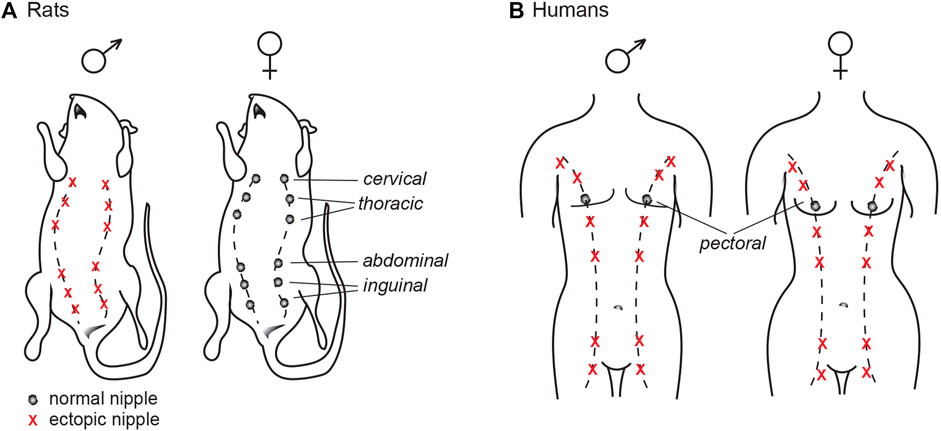
FIGURE 4. The mammalian milk line, or mammary ridge. (A) In common laboratory rats (such as Wistar and Sprague Dawley), nipples will only develop in the female offspring, as androgen signaling during development prompts the nipple anlagen to regress in males. (B) Humans have auxiliary mammary ridges, but only the two central pectoral nipples will develop. Contrary to rats, both sexes have the same number of nipples.
There are familial cases of supernumerary nipples displaying a pattern of autosomal dominant transmission with variable penetrance, but most cases appear to be sporadic. Thus, although genetics is suspected to play a dominant role in the etiology of excess nipples in humans, the responsible genes remain elusive. Interestingly, supernumerary nipples have been associated with numerous diseases, most prominently urogenital disorders (Méhes et al., 1987; Leung and Robson 1989; Grotto et al., 2001; Brown and Schwartz 2003). This supports the view that genetics is a major factor, but also allows for the possibility of environmental influences. Whether or not endocrine disruption play a significant role, however, cannot be determined as there is almost a complete lack of epidemiological studies looking at associations between excess nipples and either altered hormone profiles or exposure to EDCs. Since males and females express the same number of nipples in humans, the influence of androgens would necessarily be different than what is the case in rats. However, this does not exclude the possibility that hormone disruption could play some part. At least sex hormones are major regulators of nipple/breast development at puberty, a developmental stage when diffuse areolas/moles in humans can develop into nipples and breast tissues (Mimouni et al., 1983; Leung and Robson 1989). Nevertheless, until we have data available, a link between endocrine disruption and supernumerary nipples in humans remains speculative; but it would certainly be an interesting issue to explore in future studies.
Conclusions and Perspectives
The retention of areolas/nipples in male rodent offspring after in utero exposure to chemicals is a sign of perturbed androgen action during critical stages of the “masculinization programming window.” As adverse outcomes that can be observed in intact animals (as currently required to categorize a chemical substance as an EDC from a regulatory point of view), both NR and AGD are essentially markers for the same mode of action: anti-androgenic. In this regard, measuring both endpoints could be considered redundant. However, as our current synthesis of available data has shown, there are differences in specificity and sensitivity between the two biomarkers. In addition, these differences may stem largely from the fact that the molecular pathways governing development of the perineal region and the nipple anlagen in rats are different. Both processes are highly sensitive to androgen action, but there are data also strongly suggesting that AGD is influenced by additional mechanisms/modalities outside the linear, and canonical, testosterone-DHT-AR signaling pathway in a way that nipple regression is not.
To summarize, we want to highlight the following five points to stress why we think NR is of continued value as a biomarker of anti-androgenicity, as well as some points that should be of focus in the future.
- NR is a sensitive endpoint for anti-androgenic effects in developmental/reproductive rodent toxicity studies and is relevant for human risk assessment purposes
- In most cases, NR and AGD reveals similar effect data as pertaining to endocrine disruption through anti-androgenic mode of action, but in some cases NR is more sensitive than AGD. The assessment of both NR and AGD in guideline studies is therefore still recommended
- We recommend an international standardization to reduce the lab-to-lab variability in the outcome of NR assessments
- NR may be more specific than AGD with regard to anti-androgenic mode of action (specific to the DHT-AR signaling axis), with AGD seemingly also more vulnerable to perturbations outside the canonical DHT-AR signaling axis; e.g. substances with estrogenic action
- Most data indicate that NR is a permanent effect
Author Contributions
CS, SC, UH, and TS contributed to conception and design of review. NL, CS, UH, and SC organized the database. NL and LR made literature searches. CS and SC wrote the first draft of the manuscript. CS, TS, UH, SC, MA, and LR wrote sections of the manuscript. All authors contributed to manuscript revision, read, and approved the submitted version.
Funding
This work was funded by grants from: - The Danish Veterinary and Food Administration (DVFA), Project “Feminix” -The Nordic Working Group on Chemicals, Environment and Health (NKE) sub-group Nord UTTE (project 2020-027 and 2021-020). None of the funding bodies have had any role in the submitted publication.
Conflict of Interest
The authors declare that the research was conducted in the absence of any commercial or financial relationships that could be construed as a potential conflict of interest.
Publisher’s Note
All claims expressed in this article are solely those of the authors and do not necessarily represent those of their affiliated organizations, or those of the publisher, the editors and the reviewers. Any product that may be evaluated in this article, or claim that may be made by its manufacturer, is not guaranteed or endorsed by the publisher.
Acknowledgments
We thank Camilla Nielsen for assistance with PubMed literature searches. We also want to extend a great thanks to our magnificent group of laboratory technicians that for many years have assisted with our rat toxicity studies.
Supplementary Material
The Supplementary Material for this article can be found online at: https://www.frontiersin.org/articles/10.3389/ftox.2021.730752/full#supplementary-material
References
Andrade, A. J. M., Grande, S. W., Talsness, C. E., Grote, K., Golombiewski, A., Sterner-Kock, A., et al. (2006). A Dose-Response Study Following In Utero and Lactational Exposure to Di-(2-ethylhexyl) Phthalate (DEHP): Effects on Androgenic Status, Developmental Landmarks and Testicular Histology in Male Offspring Rats. Toxicology 225, 64–74. doi:10.1016/j.tox.2006.05.007
Axelstad, M., Boberg, J., Hougaard, K. S., Christiansen, S., Jacobsen, P. R., Mandrup, K. R., et al. (2011). Effects of Pre- and Postnatal Exposure to the UV-Filter Octyl Methoxycinnamate (OMC) on the Reproductive, Auditory and Neurological Development of Rat Offspring. Toxicol. Appl. Pharmacol. 250, 278–290. doi:10.1016/j.taap.2010.10.031
Axelstad, M., Christiansen, S., Boberg, J., Scholze, M., Jacobsen, P. R., Isling, L. K., et al. (2014). Mixtures of Endocrine-Disrupting Contaminants Induce Adverse Developmental Effects in Preweaning Rats. Reproduction 147, 489–501. doi:10.1530/REP-13-0447
Barlow, N. J., McIntyre, B. S., and Foster, P. M. D. (2004). Male Reproductive Tract Lesions at 6, 12, and 18 Months of Age Following In Utero Exposure to Di(n-Butyl) Phthalate. Toxicol. Pathol. 32, 79–90. doi:10.1080/01926230490265894
Beverly, B. E. J., Furr, J. R., Lambright, C. S., Wilson, V. S., McIntyre, B. S., Foster, P. M. D., et al. (2019). In Utero Exposure to Simvastatin Reduces Postnatal Survival and Permanently Alters Reproductive Tract Development in the Crl:CD(SD) Male Rat. Toxicol. Appl. Pharmacol. 365, 112–123. doi:10.1016/j.taap.2019.01.001
Blystone, C. R., Lambright, C. S., Howdeshell, K. L., Furr, J., Sternberg, R. M., Butterworth, B. C., et al. (2007). Sensitivity of Fetal Rat Testicular Steroidogenesis to Maternal Prochloraz Exposure and the Underlying Mechanism of Inhibition. Toxicol. Sci. 97, 512–519. doi:10.1093/toxsci/kfm055
Boberg, J., Axelstad, M., Svingen, T., Mandrup, K., Christiansen, S., Vinggaard, A. M., et al. (2016). Multiple Endocrine Disrupting Effects in Rats Perinatally Exposed to Butylparaben. Toxicol. Sci. 152, 244–256. doi:10.1093/toxsci/kfw079
Boberg, J., Christiansen, S., Axelstad, M., Kledal, T. S., Vinggaard, A. M., Dalgaard, M., et al. (2011). Reproductive and Behavioral Effects of Diisononyl Phthalate (DINP) in Perinatally Exposed Rats. Reprod. Toxicol. 31, 200–209. doi:10.1016/j.reprotox.2010.11.001
Borch, J., Dalgaard, M., and Ladefoged, O. (2005). Early Testicular Effects in Rats Perinatally Exposed to DEHP in Combination with DEHA?apoptosis Assessment and Immunohistochemical Studies. Reprod. Toxicol. 19, 517–525. doi:10.1016/j.reprotox.2004.11.004
Borch, J., Metzdorff, S. B., Vinggaard, A. M., Brokken, L., and Dalgaard, M. (2006). Mechanisms Underlying the Anti-androgenic Effects of Diethylhexyl Phthalate in Fetal Rat Testis. Toxicology 223, 144–155. doi:10.1016/j.tox.2006.03.015
Bowman, C. J., Barlow, N. J., Turner, K. J., Wallace, D. G., and Foster, P. M. D. (2003). Effects of In Utero Exposure to Finasteride on Androgen-dependent Reproductive Development in the Male Rat. Toxicol. Sci. 74, 393–406. doi:10.1093/toxsci/kfg128
Byford, J. R., Shaw, L. E., Drew, M. G. B., Pope, G. S., Sauer, M. J., and Darbre, P. D. (2002). Oestrogenic Activity of Parabens in MCF7 Human Breast Cancer Cells. J. Steroid Biochem. Mol. Biol. 80, 49–60. doi:10.1016/S0960-0760(01)00174-1
Carruthers, C. M., and Foster, P. M. D. (2005). Critical Window of Male Reproductive Tract Development in Rats Following Gestational Exposure to Di-n-butyl Phthalate. Birth Defect Res. B 74, 277–285. doi:10.1002/bdrb.20050
Casanova, M., You, L., Gaido, K. W., Archibeque-Engle, S., Janszen, D. B., and Heck, H. A. (1999). Developmental Effects of Dietary Phytoestrogens in Sprague-Dawley Rats and Interactions of Genistein and Daidzein with Rat Estrogen Receptors Alpha and Beta In Vitro. Toxicol. Sci. 51, 236–244. doi:10.1093/toxsci/51.2.236
Chen, J., Ahn, K. C., Gee, N. A., Gee, S. J., Hammock, B. D., and Lasley, B. L. (2007). Antiandrogenic Properties of Parabens and Other Phenolic Containing Small Molecules in Personal Care Products. Toxicol. Appl. Pharmacol. 221, 278–284. doi:10.1016/j.taap.2007.03.015
Christiansen, S., Axelstad, M., Boberg, J., Vinggaard, A. M., Pedersen, G. A., and Hass, U. (2014). Low-dose Effects of Bisphenol a on Early Sexual Development in Male and Female Rats. Reproduction 147, 477–487. doi:10.1530/REP-13-0377
Christiansen, S., Boberg, J., Axelstad, M., Dalgaard, M., Vinggaard, A. M., Metzdorff, S. B., et al. (2010). Low-dose Perinatal Exposure to Di(2-ethylhexyl) Phthalate Induces Anti-androgenic Effects in Male Rats. Reprod. Toxicol. 30, 313–321. doi:10.1016/j.reprotox.2010.04.005
Christiansen, S., Scholze, M., Axelstad, M., Boberg, J., Kortenkamp, A., and Hass, U. (2008). Combined Exposure to Anti-androgens Causes Markedly Increased Frequencies of Hypospadias in the Rat. Int. J. Androl. 31, 241–248. doi:10.1111/j.1365-2605.2008.00866.x
Christiansen, S., Scholze, M., Dalgaard, M., Vinggaard, A. M., Axelstad, M., Kortenkamp, A., et al. (2009). Synergistic Disruption of External Male Sex Organ Development by a Mixture of Four Antiandrogens. Environ. Health Perspect. 117, 1839–1846. doi:10.1289/ehp.0900689
Clark, R. L., Anderson, C. A., Prahalada, S., Robertson, R. T., Lochry, E. A., Leonard, Y. M., et al. (1993). Critical Developmental Periods for Effects on Male Rat Genitalia Induced by Finasteride, a 5α-Reductase Inhibitor. Toxicol. Appl. Pharmacol. 119, 34–40. doi:10.1006/taap.1993.1041
Clark, R. L., Antonello, J. M., Grossman, S. J., Wise, L. D., Anderson, C., Bagdon, W. J., et al. (1990). External Genitalia Abnormalities in Male Rats Exposed In Utero to Finasteride, a 5α-Reductase Inhibitor. Teratology 42, 91–100. doi:10.1002/tera.1420420111
Clewell, R. A., Thomas, A., Willson, G., Creasy, D. M., and Andersen, M. E. (2013). A Dose Response Study to Assess Effects after Dietary Administration of Diisononyl Phthalate (DINP) in Gestation and Lactation on Male Rat Sexual Development. Reprod. Toxicol. 35, 70–80. doi:10.1016/j.reprotox.2012.07.008
Colbert, N. K. W., Pelletier, N. C., Cote, J. M., Concannon, J. B., Jurdak, N. A., Minott, S. B., et al. (2005). Perinatal Exposure to Low Levels of the Environmental Antiandrogen Vinclozolin Alters Sex-Differentiated Social Play and Sexual Behaviors in the Rat. Environ. Health Perspect. 113, 700–707. doi:10.1289/ehp.7509
Dean, A., and Sharpe, R. M. (2013). Anogenital Distance or Digit Length Ratio as Measures of Fetal Androgen Exposure: Relationship to Male Reproductive Development and its Disorders. J. Clin. Endocrinol. Metab. 98, 2230–2238. doi:10.1210/jc.2012-4057
Delclos, K. B., Camacho, L., Lewis, S. M., Vanlandingham, M. M., Latendresse, J. R., Olson, G. R., et al. (2014). Toxicity Evaluation of Bisphenol A Administered by Gavage to Sprague Dawley Rats from Gestation Day 6 Through Postnatal Day 90. Toxicol. Sci. 139, 174–197. doi:10.1093/toxsci/kfu022
Di Lorenzo, M., Winge, S. B., Svingen, T., De Falco, M., and Boberg, J. (2020). Intrauterine Exposure to Diethylhexyl Phthalate Disrupts Gap Junctions in the Fetal Rat Testis. Curr. Res. Toxicol. 1, 5–11. doi:10.1016/j.crtox.2020.02.002
Diamanti-Kandarakis, E., Bourguignon, J.-P., Giudice, L. C., Hauser, R., Prins, G. S., Soto, A. M., et al. (2009). Endocrine-Disrupting Chemicals: An Endocrine Society Scientific Statement. Endocr. Rev. 30, 293–342. doi:10.1210/er.2009-0002
Dionne, F. T., Lesage, R. L., Dubé, J. Y., and Tremblay, R. R. (1979). Estrogen Binding Proteins in Rat Skeletal and Perineal Muscles: In Vitro and In Vivo Studies. J. Steroid Biochem. 11, 1073–1080. doi:10.1016/0022-4731(79)90156-0
Dreisig, K., Taxvig, C., Kjærstad, M., Nellemann, C., Hass, U., and Vinggaard, A. M. (2013). Predictive Value of Cell Assays for Developmental Toxicity and Embryotoxicity of Conazole Fungicides. ALTEX 30, 319–330. doi:10.14573/altex.2013.3.319
EFSA (2020). Outcome of the Pesticides Peer Review Meeting on General Recurring Issues in Mammalian Toxicology. EFSA Support, 17, 1–26. doi:10.2903/sp.efsa.2019.EN-1837
FDA (2011). Center for Drug Evaluation and Research. Approval package for application number NDA 20-788/S-020/S-021/S-023. Available at: https://www.accessdata.fda.gov/drugsatfda_docs/nda/2012/020788Orig1s020.pdf
Ferguson, S. A., Law, C. D., and Abshire, J. S. (2011). Developmental Treatment with Bisphenol a or Ethinyl Estradiol Causes Few Alterations on Early Preweaning Measures. Toxicol. Sci. 124, 149–160. doi:10.1093/toxsci/kfr201
Flick, B., Schneider, S., Melching-Kollmuss, S., Fussell, K. C., Gröters, S., Buesen, R., et al. (2017). Investigations of Putative Reproductive Toxicity of Low-Dose Exposures to Vinclozolin in Wistar Rats. Arch. Toxicol. 91, 1941–1956. doi:10.1007/s00204-016-1811-y
Foster, P. M. D., and Harris, M. W. (2005). Changes in Androgen-Mediated Reproductive Development in Male Rat Offspring Following Exposure to a Single Oral Dose of Flutamide at Different Gestational Ages. Toxicol. Sci. 85, 1024–1032. doi:10.1093/toxsci/kfi159
Fussell, K. C., Schneider, S., Buesen, R., Groeters, S., Strauss, V., Melching-Kollmuss, S., et al. (2015). Investigations of Putative Reproductive Toxicity of Low-Dose Exposures to Flutamide in Wistar Rats. Arch. Toxicol. 89, 2385–2402. doi:10.1007/s00204-015-1622-6
Gray, L. E., Ostby, J. S., and Kelce, W. R. (1994). Developmental Effects of an Environmental Antiandrogen: The Fungicide Vinclozolin Alters Sex Differentiation of the Male Rat. Toxicol. Appl. Pharmacol. 129, 46–52. doi:10.1006/taap.1994.1227
Gray, L. E., Barlow, N. J., Howdeshell, K. L., Ostby, J. S., Furr, J. R., and Gray, C. L. (2009). Transgenerational Effects of Di (2-Ethylhexyl) Phthalate in the Male CRL:CD(SD) Rat: Added Value of Assessing Multiple Offspring Per Litter. Toxicol. Sci. 110, 411–425. doi:10.1093/toxsci/kfp109
Gray, L. E. (2001). Effects of Environmental Antiandrogens on Reproductive Development in Experimental Animals. Hum. Reprod. Update 7, 248–264. doi:10.1093/humupd/7.3.248
Gray, L. E., Furr, J. R., Conley, J. M., Lambright, C. S., Evans, N., Cardon, M. C., et al. (2019). A Conflicted Tale of Two Novel AR Antagonists In Vitro and In Vivo: Pyrifluquinazon Versus Bisphenol C. Toxicol. Sci. 168, 632–643. doi:10.1093/toxsci/kfz010
Gray, L. E., Ostby, J., Furr, J., Price, M., Veeramachaneni, D. N. R., and Parks, L. (2000). Perinatal Exposure to the Phthalates DEHP, BBP, and DINP, but Not DEP, DMP, or DOTP, Alters Sexual Differentiation of the Male Rat. Toxicol. Sci. 58, 350–365. doi:10.1093/toxsci/58.2.350
Gray, L. E., Ostby, J., Monosson, E., and Kelce, W. R. (1999b). Environmental Antiandrogens: Low Doses of the Fungicide Vinclozolin Alter Sexual Differentiation of the Male Rat. Toxicol. Ind. Health 15, 48–64. doi:10.1191/074823399678846646
Grotto, I., Browner‐Elhanan, K., Mimouni, D., Varsano, I., Avner Cohen, H., and Mimouni, M. (2001). Occurrence of Supernumerary Nipples in Children with Kidney and Urinary Tract Malformations. Pediatr. Dermatol. 18, 291–294. doi:10.1046/j.1525-1470.2001.01930.x
Hannas, B. R., Lambright, C. S., Furr, J., Howdeshell, K. L., Wilson, V. S., and Gray, L. E. (2011). Dose-Response Assessment of Fetal Testosterone Production and Gene Expression Levels in Rat Testes Following InUtero Exposure to Diethylhexyl Phthalate, Diisobutyl Phthalate, Diisoheptyl Phthalate, and Diisononyl Phthalate. Toxicol. Sci. 123, 206–216. doi:10.1093/toxsci/kfr146
Hass, U., Boberg, J., Christiansen, S., Jacobsen, P. R., Vinggaard, A. M., Taxvig, C., et al. (2012). Adverse Effects on Sexual Development in Rat Offspring after Low Dose Exposure to a Mixture of Endocrine Disrupting Pesticides. Reprod. Toxicol. 34, 261–274. doi:10.1016/j.reprotox.2012.05.090
Hass, U., Scholze, M., Christiansen, S., Dalgaard, M., Vinggaard, A. M., Axelstad, M., et al. (2007). Combined Exposure to Anti-androgens Exacerbates Disruption of Sexual Differentiation in the Rat. Environ. Health Perspect. 115, 122–128. doi:10.1289/ehp.9360
Hellwig, J., Van Ravenzwaay, B., Mayer, M., and Gembardt, C. (2000). Pre- and Postnatal Oral Toxicity of Vinclozolin in Wistar and Long-Evans Rats. Regul. Toxicol. Pharmacol. 32, 42–50. doi:10.1006/rtph.2000.1400
Holm, J. B., Chalmey, C., Modick, H., Jensen, L. S., Dierkes, G., Weiss, T., et al. (2015). Aniline Is Rapidly Converted into Paracetamol Impairing Male Reproductive Development. Toxicol. Sci. 148, 288–298. doi:10.1093/toxsci/kfv179
Hotchkiss, A. K., and Vandenbergh, J. G. (2005). The Anogenital Distance index of Mice (Mus musculus Domesticus): An Analysis. Contemp. Top. Lab. Anim. Sci. 44, 46–48.
Hotchkiss, A. K., Parks-Saldutti, L. G., Ostby, J. S., Lambright, C., Furr, J., Vandenbergh, J. G., et al. (2004). A Mixture of the “Antiandrogens” Linuron and Butyl Benzyl Phthalate Alters Sexual Differentiation of the Male Rat in a Cumulative Fashion1. Biol. Reprod. 71, 1852–1861. doi:10.1095/biolreprod.104.031674
Howdeshell, K. L., Furr, J., Lambright, C. R., Rider, C. V., Wilson, V. S., and Gray, L. E. (2007). Cumulative Effects of Dibutyl Phthalate and Diethylhexyl Phthalate on Male Rat Reproductive Tract Development: Altered Fetal Steroid Hormones and Genes. Toxicol. Sci. 99, 190–202. doi:10.1093/toxsci/kfm069
Howdeshell, K. L., Furr, J., Lambright, C. R., Wilson, V. S., Ryan, B. C., and Gray, L. E. (2008). Gestational and Lactational Exposure to Ethinyl Estradiol, But Not Bisphenol a, Decreases Androgen-dependent Reproductive Organ Weights and Epididymal Sperm Abundance in the Male Long Evans Hooded Rat. Toxicol. Sci. 102, 371–382. doi:10.1093/toxsci/kfm306
Imperato-McGinley, J., Binienda, Z., Gedney, J., and Vaughan, E. D. (1986). Nipple Differentiation in Fetal Male Rats Treated with an Inhibitor of the Enzyme 5α-Reductase: Definition of a Selective Role for Dihydrotestosterone*. Endocrinology 118, 132–137. doi:10.1210/endo-118-1-132
Imperato-McGinley, J., Sanchez, R. S., Spencer, J. R., Yee, B., and Vaughan, E. D. (1992). Comparison of the Effects of the 5 Alpha-Reductase Inhibitor Finasteride and the Antiandrogen Flutamide on Prostate and Genital Differentiation: Dose-Response Studies. Endocrinology 131, 1149–1156. doi:10.1210/endo.131.3.1324152
Jarfelt, K., Dalgaard, M., Hass, U., Borch, J., Jacobsen, H., and Ladefoged, O. (2005). Antiandrogenic Effects in Male Rats Perinatally Exposed to a Mixture of Di(2-ethylhexyl) Phthalate and Di(2-ethylhexyl) Adipate. Reprod. Toxicol. 19, 505–515. doi:10.1016/j.reprotox.2004.11.005
Johansson, H. K. L., Christiansen, S., Draskau, M. K., Svingen, T., and Boberg, J. (2021). Classical Toxicity Endpoints in Female Rats Are Insensitive to the Human Endocrine Disruptors Diethylstilbestrol and Ketoconazole. Reprod. Toxicol. 101, 9–17. doi:10.1016/j.reprotox.2021.01.003
Jost, A. (1954). Hormonal Factors in the Development of the Fetus. Cold Spring Harbor Symposia Quantitative Biol. 19, 167–181. doi:10.1101/sqb.1954.019.01.023
Kelce, W. R., Lambright, C. R., Gray, L. E., and Roberts, K. P. (1997). Vinclozolin Andp,p′-DDE Alter Androgen-dependent Gene Expression:In Vivo Confirmation of an Androgen Receptor-Mediated Mechanism. Toxicol. Appl. Pharmacol. 142, 192–200. doi:10.1006/taap.1996.7966
Kelce, W. R., Monosson, E., Gamcsik, M. P., Laws, S. C., and Gray, L. E. (1994). Environmental Hormone Disruptors: Evidence that Vinclozolin Developmental Toxicity Is Mediated by Antiandrogenic Metabolites. Toxicol. Appl. Pharmacol. 126, 276–285. doi:10.1006/taap.1994.1117
Kelce, W. R., Stone, C. R., Laws, S. C., Gray, L. E., Kemppainen, J. A., and Wilson, E. M. (1995). Persistent DDT Metabolite P,p'-DDE Is a Potent Androgen Receptor Antagonist. Nature 375, 581–585. doi:10.1038/375581a0
Kim, H. S., Han, S.-Y., Yoo, S. D., Lee, B. M., and Park, K. L. (2001). Potential Estrogenic Effects of Bisphenol-A Estimated by In Vitro and In Vivo Combination Assays. J. Toxicol. Sci. 26, 111–118. doi:10.2131/jts.26.111
Kim, T. S., Jung, K. K., Kim, S. S., Kang, I. H., Baek, J. H., Nam, H.-S., et al. (2010). Effects of In Utero Exposure to Di(n-butyl) Phthalate on Development of Male Reproductive Tracts in Sprague-Dawley Rats. J. Toxicol. Environ. Health A 73, 1544–1559. doi:10.1080/15287394.2010.511579
Kleinstreuer, N. C., Ceger, P., Watt, E. D., Martin, M., Houck, K., Browne, P., et al. (2017). Development and Validation of a Computational Model for Androgen Receptor Activity. Chem. Res. Toxicol. 30, 946–964. doi:10.1021/acs.chemrestox.6b00347
Kratochwil, K. (1977). Development and Loss of Androgen Responsiveness in the Embryonic Rudiment of the Mouse Mammary Gland. Develop. Biol. 61, 358–365. doi:10.1016/0012-1606(77)90305-0
Kratochwil, K., and Schwartz, P. (1976). Tissue Interaction in Androgen Response of Embryonic Mammary Rudiment of Mouse: Identification of Target Tissue for Testosterone. Proc. Natl. Acad. Sci. 73, 4041–4044. doi:10.1073/pnas.73.11.4041
Kristensen, D. M., Hass, U., Lesné, L., Lottrup, G., Jacobsen, P. R., Desdoits-Lethimonier, C., et al. (2011). Intrauterine Exposure to Mild Analgesics Is a Risk Factor for Development of Male Reproductive Disorders in Human and Rat. Hum. Reprod. 26, 235–244. doi:10.1093/humrep/deq323
Kristensen, D. M., Mazaud-Guittot, S., Gaudriault, P., Lesné, L., Serrano, T., Main, K. M., et al. (2016). Analgesic Use - Prevalence, Biomonitoring and Endocrine and Reproductive Effects. Nat. Rev. Endocrinol. 12, 381–393. doi:10.1038/nrendo.2016.55
Laier, P., Metzdorff, S. B., Borch, J., Hagen, M. L., Hass, U., Christiansen, S., et al. (2006). Mechanisms of Action Underlying the Antiandrogenic Effects of the Fungicide Prochloraz. Toxicol. Appl. Pharmacol. 213, 160–171. doi:10.1016/j.taap.2005.10.013
Lawson, G., and Luderer, U. (2004). Gestational and Lactational Exposure to Heptachlor Does Not Alter Reproductive System Development in Rats. Vet. Hum. Toxicol. 46, 113–118.
Lee, H., Lee, J., Choi, K., and Kim, K.-T. (2019). Comparative Analysis of Endocrine Disrupting Effects of Major Phthalates in Employed Two Cell Lines (MVLN and H295R) and Embryonic Zebrafish Assay. Environ. Res. 172, 319–325. doi:10.1016/j.envres.2019.02.033
Lee, K.-Y., Shibutani, M., Takagi, H., Kato, N., Takigami, S., Uneyama, C., et al. (2004). Diverse Developmental Toxicity of Di-n-butyl Phthalate in Both Sexes of Rat Offspring After Maternal Exposure During the Period from Late Gestation through Lactation. Toxicology 203, 221–238. doi:10.1016/j.tox.2004.06.013
Lent, E. M., Crouse, L. C. B., Jackovitz, A. M., Carroll, E. E., and Johnson, M. S. (2016). An Extended One-Generation Reproductive Toxicity Test of 1,2,4-Triazol-5-One (NTO) in Rats. J. Toxicol. Environ. Health Part A 79, 1159–1178. doi:10.1080/15287394.2016.1219893
Leung, A. K. C., and Robson, W. L. M. (1989). Polythelia. Int. J. Dermatol. 28, 429–433. doi:10.1111/j.1365-4362.1989.tb02498.x
Loeffler, I. K., and Peterson, R. E. (1999). Interactive Effects of TCDD Andp,p′-DDE on Male Reproductive Tract Development Inin Uteroand Lactationally Exposed Rats. Toxicol. Appl. Pharmacol. 154, 28–39. doi:10.1006/taap.1998.8572
MacLeod, D. J., Sharpe, R. M., Welsh, M., Fisken, M., Scott, H. M., Hutchison, G. R., et al. (2010). Androgen Action in the Masculinization Programming Window and Development of Male Reproductive Organs. Int. J. Androl. 33, 279–287. doi:10.1111/j.1365-2605.2009.01005.x
Mandrup, K. R., Jacobsen, P. R., Isling, L. K., Axelstad, M., Dreisig, K., Hadrup, N., et al. (2013). Effects of Perinatal Ethinyl Estradiol Exposure in Male and Female Wistar Rats. Reprod. Toxicol. 42, 180–191. doi:10.1016/j.reprotox.2013.09.001
Martínez, A. G., Pardo, B., Gámez, R., Mas, R., Noa, M., Marrero, G., et al. (2011). Effects of In Utero Exposure to D-004, A Lipid Extract from Roystonea Regia Fruits, in the Male Rat: A Comparison with Finasteride. J. Med. Food 14, 1663–1669. doi:10.1089/jmf.2010.0279
Martino-Andrade, A. J., Morais, R. N., Botelho, G. G. K., Muller, G., Grande, S. W., Carpentieri, G. B., et al. (2009). Coadministration of Active Phthalates Results in Disruption of Foetal Testicular Function in Rats. Int. J. Androl. 32, 704–712. doi:10.1111/j.1365-2605.2008.00939.x
Matsushita, S., Suzuki, K., Murashima, A., Kajioka, D., Acebedo, A. R., Miyagawa, S., et al. (2018). Regulation of Masculinization: Androgen Signalling for External Genitalia Development. Nat. Rev. Urol. 15, 358–368. doi:10.1038/s41585-018-0008-y
Matsuura, I., Saitoh, T., Ashina, M., Wako, Y., Iwata, H., Toyota, N., et al. (2005a). Evaluation of a Two-Generation Reproduction Toxicity Study Adding Endopoints to Detect Endocrine Disrupting Activity Using Vinclozolin. J. Toxicol. Sci. 30, S163–S188. doi:10.2131/jts.30.S163
Matsuura, I., Saitoh, T., Tani, E., Wako, Y., Iwata, H., Toyota, N., et al. (2005b). Evaluation of a Two-Generation Reproduction Toxicity Study Adding Endopoints to Detect Endocrine Disrupting Activity Using Lindane. J. Toxicol. Sci. 30, S135–S161. doi:10.2131/jts.30.s135
Matthews, J. B., Twomey, K., and Zacharewski, T. R. (2001). In Vitro and In Vivo Interactions of Bisphenol A and its Metabolite, Bisphenol A Glucuronide, with Estrogen Receptors α and β. Chem. Res. Toxicol. 14, 149–157. doi:10.1021/tx0001833
Mattiske, D. M., and Pask, A. J. (2021). Endocrine Disrupting Chemicals in the Pathogenesis of Hypospadias; Developmental and Toxicological Perspectives. Curr. Res. Toxicol. 2, 179–191. doi:10.1016/j.crtox.2021.03.004
Mayer, J. A., Foley, J., De La Cruz, D., Chuong, C.-M., and Widelitz, R. (2008). Conversion of the Nipple to Hair-Bearing Epithelia by Lowering Bone Morphogenetic Protein Pathway Activity at the Dermal-Epidermal Interface. Am. J. Pathol. 173, 1339–1348. doi:10.2353/ajpath.2008.070920
McIntyre, B. S., Barlow, N. J., and Foster, P. M. D. (2001). Androgen-mediated Development in Male Rat Offspring Exposed to Flutamide In Utero: Permanence and Correlation of Early Postnatal Changes in Anogenital Distance and Nipple Retention with Malformations in Androgen-dependent Tissues. Toxicol. Sci. 62, 236–249. doi:10.1093/toxsci/62.2.236
McIntyre, B. S., Barlow, N. J., and Foster, P. M. D. (2002). Male Rats Exposed to Linuron In Utero Exhibit Permanent Changes in Anogenital Distance, Nipple Retention, and Epididymal Malformations that Result in Subsequent Testicular Atrophy. Toxicol. Sci. 65, 62–70. doi:10.1093/toxsci/65.1.62
McIntyre, B. S., Barlow, N. J., Wallace, D. G., Maness, S. C., Gaido, K. W., and Foster, P. M. D. (2000). Effects of In Utero Exposure to Linuron on Androgen-dependent Reproductive Development in the Male Crl:CD(SD)BR Rat. Toxicol. Appl. Pharmacol. 167, 87–99. doi:10.1006/taap.2000.8998
McIntyre, B. S., Vancutsem, P. M., Treinen, K. A., and Morrissey, R. E. (2003). Effects of Perinatal Loratadine Exposure on Male Rat Reproductive Organ Development. Reprod. Toxicol. 17, 691–697. doi:10.1016/S0890-6238(03)00108-4
McKee, R. H., Pavkov, K. L., Trimmer, G. W., Keller, L. H., and Stump, D. G. (2006). An Assessment of the Potential Developmental and Reproductive Toxicity of Di-isoheptyl Phthalate in Rodents. Reprod. Toxicol. 21, 241–252. doi:10.1016/j.reprotox.2005.09.002
Méhes, K., Szüle, E., Törzsök, F., and Meggyessy, V. (1987). Supernumerary Nipples and Urologic Malignancies. Cancer Genet. Cytogenet. 24, 185–188. doi:10.1016/0165-4608(87)90097-5
Melching-Kollmuss, S., Fussell, K. C., Schneider, S., Buesen, R., Groeters, S., Strauss, V., et al. (2017). Comparing Effect Levels of Regulatory Studies with Endpoints Derived in Targeted Anti-androgenic Studies: Example Prochloraz. Arch. Toxicol. 91, 143–162. doi:10.1007/s00204-016-1678-y
Mimouni, F., Merlob, P., and Reisner, S. H. (1983). Occurrence of Supernumerary Nipples in Newborns. Arch. Pediatr. Adolesc. Med. 137, 952–953. doi:10.1001/archpedi.1983.02140360016005
Miyata, K., Yabushita, S., Sukata, T., Sano, M., Yoshino, H., Nakanishi, T., et al. (2002). Effects of Perinatal Exposure to Flutamide on Sex Hormones and Androgen-dependent Organs in F1 Male Rats. J. Toxicol. Sci. 27, 19–33. doi:10.2131/jts.27.19
Moore, R. W., Rudy, T. A., Lin, T. M., Ko, K., and Peterson, R. E. (2001). Abnormalities of Sexual Development in Male Rats With In Utero and Lactational Exposure to the Antiandrogenic Plasticizer Di(2-ethylhexyl) Phthalate. Environ. Health Perspect. 109, 229–237. doi:10.1289/ehp.01109229
Mylchreest, E., Sar, M., Cattley, R. C., and Foster, P. M. D. (1999). Disruption of Androgen-Regulated Male Reproductive Development by Di(n-Butyl) Phthalate During Late Gestation in Rats Is Different from Flutamide. Toxicol. Appl. Pharmacol. 156, 81–95. doi:10.1006/taap.1999.8643
Mylchreest, E., Wallace, D. G., Cattley, R. C., and Foster, P. M. (2000). Dose-dependent Alterations in Androgen-Regulated Male Reproductive Development in Rats Exposed to Di(n-Butyl) Phthalate During Late Gestation. Toxicol. Sci. 55, 143–151. doi:10.1093/toxsci/55.1.143
Nakamura, N., Inselman, A. L., White, G. A., Chang, C.-W., Trbojevich, R. A., Sephr, E., et al. (2015). Effects of Maternal and Lactational Exposure to 2-Hydroxy-4-Methoxybenzone on Development and Reproductive Organs in Male and Female Rat Offspring. Birth Defects Res. B 104, 35–51. doi:10.1002/bdrb.21137
Nellemann, C., Dalgaard, M., Lam, H. R., and Vinggaard, A. M. (2003). The Combined Effects of Vinclozolin and Procymidone Do Not Deviate from Expected Additivity In Vitro and In Vivo. Toxicol. Sci. 71, 251–262. doi:10.1093/toxsci/71.2.251
Noriega, N. C., Ostby, J., Lambright, C., Wilson, V. S., and Gray, L. E. (2005). Late Gestational Exposure to the Fungicide Prochloraz Delays the Onset of Parturition and Causes Reproductive Malformations in Male but Not Female Rat Offspring1. Biol. Reprod. 72, 1324–1335. doi:10.1095/biolreprod.104.031385
OECD (2015). Feasibility Study for Minor Enhancements of TG 421/422 with ED Relevant Endpoints. Environment, Health and Safety Publications, Series on Testing and Assessment (No. 217). Paris: Organisation for Economic Cooperation and Development. Available at: https://www.oecd.org/officialdocuments/publicdisplaydocumentpdf/?cote=env/jm/mono.
OECD (2008). Guidance Document on Mammalian Reproductive Toxicity Testing and Assessment. OECD Series on Testing and Assessment No. 43. Paris: Organisation for Economic Cooperation and Development, 88. Available at: http://www.oecd.org/officialdocuments/publicdisplaydocumentpdf/?cote=env/jm/mono.
OECD (2013). Guidance Document Supporting OECD Test Guideline 443 on the Extended One Generation Reproductive Toxicity Study. Series on Testing and Assessment. ENV/JM/MONO(2013)10. Paris: OECD Publishing. Available at: http://www.oecd.org/officialdocuments/publicdisplaydocumentpdf/?cote=ENV/JM/MONO(2013)10&doclanguage=en.
OECD (2001). Test No. 416: Two-Generation Reproduction Toxicity, OECD Guidelines for the Testing of Chemicals. Paris: OECD Publishing. doi:10.1787/9789264070868-en
OECD (2016a). Test No. 421: Reproduction/Developmental Toxicity Screening Test, OECD Guidelines for the Testing of Chemicals, Section 4. Paris: OECD Publishing. doi:10.1787/9789264264380-en
OECD (2016b). Test No. 422: Combined Repeated Dose Toxicity Study with the Reproduction/Developmental Toxicity Screening Test, OECD Guidelines for the Testing of Chemicals, Section 4. Paris: OECD Publishing. doi:10.1787/9789264264403-en
OECD (2018). Test No. 443: Extended One-Generation Reproductive Toxicity Study, OECD Guidelines for the Testing of Chemicals. Paris: OECD Publishing. doi:10.1787/9789264185371-en
Okahashi, N., Sano, M., Miyata, K., Tamano, S., Higuchi, H., Kamita, Y., et al. (2005). Lack of Evidence for Endocrine Disrupting Effects in Rats Exposed to Fenitrothion In Utero and from Weaning to Maturation. Toxicology 206, 17–31. doi:10.1016/j.tox.2004.04.020
Orton, F., Rosivatz, E., Scholze, M., and Kortenkamp, A. (2011). Widely Used Pesticides with Previously Unknown Endocrine Activity Revealed as In Vitro Antiandrogens. Environ. Health Perspect. 119, 794–800. doi:10.1289/ehp.1002895
Ostby, J., Kelce, W. R., Lambright, C., Wolf, C. J., Mann, P., and Gray, L. E. (1999a). The Fungicide Procymidone Alters Sexual Differentiation in the Male Rat by Acting as an Androgen-Receptor Antagonist In Vivo and In Vitro. Toxicol. Ind. Health 15, 80–93. doi:10.1177/074823379901500108
Politano, V. T., Lewis, E. M., Hoberman, A. M., Diener, R. M., Api, A. M., and Patel, A. (2017). Oral 1-Generation Rat Reproduction Study of Isobornyl Acetate: An Evaluation Through Sexual Maturity in the F1 Generation. Int. J. Toxicol. 36, 252–259. doi:10.1177/1091581817708197
Quinn, M. J., Bannon, D. I., Jackovitz, A. M., Hanna, T. L., Shiflett, A. A., and Johnson, M. S. (2014). Assessment of 3-nitro-1,2,4-triazol-5-one as a Potential Endocrine Disrupting Chemical in Rats Using the Hershberger and Uterotrophic Bioassays. Int. J. Toxicol. 33, 367–372. doi:10.1177/1091581814548729
Ramhøj, L., Hass, U., Boberg, J., Scholze, M., Christiansen, S., Nielsen, F., et al. (2018). Perfluorohexane Sulfonate (PFHxS) and a Mixture of Endocrine Disrupters Reduce Thyroxine Levels and Cause Antiandrogenic Effects in Rats. Toxicol. Sci. 163, 579–591. doi:10.1093/toxsci/kfy055
Rice, D. C. (1999). Effect of Exposure to 3,3′,4,4′,5-Pentachlorobiphenyl (PCB 126) throughout Gestation and Lactation on Development and Spatial Delayed Alternation Performance in Rats. Neurotoxicology and Teratology 21, 59–69. doi:10.1016/s0892-0362(98)00031-2
Rosenmai, A. K., Dybdahl, M., Pedersen, M., Alice van Vugt-Lussenburg, B. M., Wedebye, E. B., Taxvig, C., et al. (2014). Are Structural Analogues to Bisphenol a Safe Alternatives? Toxicol. Sci. 139, 35–47. doi:10.1093/toxsci/kfu030
Routledge, E. J., Parker, J., Odum, J., Ashby, J., and Sumpter, J. P. (1998). Some Alkyl Hydroxy Benzoate Preservatives (Parabens) Are Estrogenic. Toxicol. Appl. Pharmacol. 153, 12–19. doi:10.1006/taap.1998.8544
Saillenfait, A.-M., Sabaté, J.-P., and Gallissot, F. (2008). Diisobutyl Phthalate Impairs the Androgen-dependent Reproductive Development of the Male Rat. Reprod. Toxicol. 26, 107–115. doi:10.1016/j.reprotox.2008.07.006
Saillenfait, A.-M., Sabaté, J.-P., and Gallissot, F. (2009). Effects of In Utero Exposure to Di-n-hexyl Phthalate on the Reproductive Development of the Male Rat. Reprod. Toxicol. 28, 468–476. doi:10.1016/j.reprotox.2009.06.013
Sathyanarayana, S., Swan, S. H., Farin, F. M., Wilkerson, H.-W., Bamshad, M., Grady, R., et al. (2012). A Pilot Study of the Association Between Genetic Polymorphisms Involved in Estrogen Signaling and Infant Male Genital Phenotypes. Asian J. Androl. 14, 766–772. doi:10.1038/aja.2012.27
Schneider, S., Kaufmann, W., Strauss, V., and van Ravenzwaay, B. (2011). Vinclozolin: A Feasibility and Sensitivity Study of the ILSI-HESI F1-Extended One-Generation Rat Reproduction Protocol. Regul. Toxicol. Pharmacol. 59, 91–100. doi:10.1016/j.yrtph.2010.09.010
Scholze, M., Taxvig, C., Kortenkamp, A., Boberg, J., Christiansen, S., Svingen, T., et al. (2020). Quantitative In Vitro to In Vivo Extrapolation (QIVIVE) for Predicting Reduced Anogenital Distance Produced by Anti-androgenic Pesticides in a Rodent Model for Male Reproductive Disorders. Environ. Health Perspect. 128, 117005. doi:10.1289/EHP6774
Schreiber, E., Garcia, T., González, N., Esplugas, R., Sharma, R. P., Torrente, M., et al. (2020). Maternal Exposure to Mixtures of Dienestrol, Linuron and Flutamide. Part I: Feminization Effects on Male Rat Offspring. Food Chem. Toxicol. 139, 111256. doi:10.1016/j.fct.2020.111256
Schwartz, C. L., Christiansen, S., Vinggaard, A. M., Axelstad, M., Hass, U., and Svingen, T. (2019a). Anogenital Distance as a Toxicological or Clinical Marker for Fetal Androgen Action and Risk for Reproductive Disorders. Arch. Toxicol. 93, 253–272. doi:10.1007/s00204-018-2350-5
Schwartz, C. L., Vinggaard, A. M., Christiansen, S., Darde, T. A., Chalmel, F., and Svingen, T. (2019b). Distinct Transcriptional Profiles of the Female, Male, and Finasteride-Induced Feminized Male Anogenital Region in Rat Fetuses. Toxicol. Sci. 169, 303–311. doi:10.1093/toxsci/kfz046
Scott, H. M., Mason, J. I., and Sharpe, R. M. (2009). Steroidogenesis in the Fetal Testis and its Susceptibility to Disruption by Exogenous Compounds. Endocr. Rev. 30, 883–925. doi:10.1210/er.2009-0016
Sharpe, R. M. (2020). Androgens and the Masculinization Programming Window: Human-Rodent Differences. Biochem. Soc. Trans. 48, 1725–1735. doi:10.1042/BST20200200
Sharpe, R. M., and Skakkebaek, N. E. (2008). Testicular Dysgenesis Syndrome: Mechanistic Insights and Potential New Downstream Effects. Fertil. Sterility 89, e33–e38. doi:10.1016/j.fertnstert.2007.12.026
Simard, J., Luthy, I., Guay, J., Bélanger, A., and Labrie, F. (1986). Characteristics of Interaction of the Antiandrogen Flutamide with the Androgen Receptor in Various Target Tissues. Mol. Cell Endocrinol. 44, 261–270. doi:10.1016/0303-7207(86)90132-2
Skakkebaek, N. E., Rajpert-De Meyts, E., Buck Louis, G. M., Toppari, J., Andersson, A.-M., Eisenberg, M. L., et al. (2016). Male Reproductive Disorders and Fertility Trends: Influences of Environment and Genetic Susceptibility. Physiol. Rev. 96, 55–97. doi:10.1152/physrev.00017.2015
Svingen, T., and Koopman, P. (2013). Building the Mammalian Testis: Origins, Differentiation, and Assembly of the Component Cell Populations. Genes Dev. 27, 2409–2426. doi:10.1101/gad.228080.113
Skakkebaek, N. E., Rajpert-De Meyts, E., and Main, K. M. (2001). Testicular Dysgenesis Syndrome: An Increasingly Common Developmental Disorder with Environmental Aspects. Hum. Repro. 16, 972–978. doi:10.1093/humrep/16.5.972
Taxvig, C., Hass, U., Axelstad, M., Dalgaard, M., Boberg, J., Andeasen, H. R., et al. (2007). Endocrine-disrupting Activities In Vivo of the Fungicides Tebuconazole and Epoxiconazole. Toxicol. Sci. 100, 464–473. doi:10.1093/toxsci/kfm227
Turner, K. J., Barlow, N. J., Struve, M. F., Wallace, D. G., Gaido, K. W., Dorman, D. C., et al. (2002). Effects of In Utero Exposure to the Organophosphate Insecticide Fenitrothion on Androgen-dependent Reproductive Development in the Crl:CD(SD)BR Rat. Toxicol. Sci. 68, 174–183. doi:10.1093/toxsci/68.1.174
Tyl, R. W., Myers, C. B. B., Marr, M. C. C., Thomas, B. F. F., Keimowitz, A. R. R., Brine, D. R. R., et al. (2002). Three-generation Reproductive Toxicity Study of Dietary Bisphenol A in CD Sprague-Dawley Rats. Toxicol. Sci. 68, 121–146. doi:10.1093/toxsci/68.1.121
Tyl, R. W., Myers, C. B., Marr, M. C., Fail, P. A., Seely, J. C., Brine, D. R., et al. (2004). Reproductive Toxicity Evaluation of Dietary Butyl Benzyl Phthalate (BBP) in Rats. Reprod. Toxicol. 18, 241–264. doi:10.1016/j.reprotox.2003.10.006
van den Driesche, S., Kilcoyne, K. R., Wagner, I., Rebourcet, D., Boyle, A., Mitchell, R., et al. (2017). Experimentally Induced Testicular Dysgenesis Syndrome Originates in the Masculinization Programming Window. JCI Insight 2, 1–20. doi:10.1172/jci.insight.91204
van den Driesche, S., Kolovos, P., Platts, S., Drake, A. J., and Sharpe, R. M. (2012). Inter-relationship Between Testicular Dysgenesis and Leydig Cell Function in the Masculinization Programming Window in the Rat. PLoS One 7, e30111. doi:10.1371/journal.pone.0030111
Villeneuve, D. L., Crump, D., Garcia-Reyero, N., Hecker, M., Hutchinson, T. H., LaLone, C. A., et al. (2014). Adverse Outcome Pathway (AOP) Development I: Strategies and Principles. Toxicol. Sci. 142, 312–320. doi:10.1093/toxsci/kfu199
Vinggaard, A. M., Christiansen, S., Laier, P., Poulsen, M. E., Breinholt, V., Jarfelt, K., et al. (2005). Perinatal Exposure to the Fungicide Prochloraz Feminizes the Male Rat Offspring. Toxicol. Sci. 85, 886–897. doi:10.1093/toxsci/kfi150
Vinggaard, A. M., Hass, U., Dalgaard, M., Andersen, H. R., Bonefeld-jorgensen, E., Christiansen, S., et al. (2006). Prochloraz: An Imidazole Fungicide with Multiple Mechanisms of Action. Int. J. Androl. 29, 186–192. doi:10.1111/j.1365-2605.2005.00604.x
Vinggaard, A. M., Nellemann, C., Dalgaard, M., Jørgensen, E. B., and Andersen, H. R. (2002). Antiandrogenic Effects In Vitro and In Vivo of the Fungicide Prochloraz. Toxicol. Sci. 69, 344–353. doi:10.1093/toxsci/69.2.344
Welsh, M., Saunders, P. T. K., Fisken, M., Scott, H. M., Hutchison, G. R., Smith, L. B., et al. (2008). Identification in Rats of a Programming Window for Reproductive Tract Masculinization, Disruption of Which Leads to Hypospadias and Cryptorchidism. J. Clin. Invest. 118, 1479–1490. doi:10.1172/JCI34241
Wolf, C. J., LeBlanc, G. A., and Gray, L. E. (2004). Interactive Effects of Vinclozolin and Testosterone Propionate on Pregnancy and Sexual Differentiation of the Male and Female SD Rat. Toxicol. Sci. 78, 135–143. doi:10.1093/toxsci/kfh018
Wolf, C. J., LeBlanc, G. A., Ostby, J. S., and Gray, L. E. (2000). Characterization of the Period of Sensitivity of Fetal Male Sexual Development to Vinclozolin. Toxicol. Sci. 55, 152–161. doi:10.1093/toxsci/55.1.152
Wolf, C., Lambright, C., Mann, P., Price, M., Cooper, R. L., Ostby, J., et al. (1999). Administration of Potentially Antiandrogenic Pesticides (Procymidone, Linuron, Iprodione, Chlozolinate, P,p′-DDE, and Ketoconazole) and Toxic Substances (Dibutyl- and Diethylhexyl Phthalate, PCB 169, and Ethane Dimethane Sulphonate) During Sexual Differentiation Produces Diverse Profiles of Reproductive Malformations in the Male Rat. Toxicol. Ind. Health 15, 94–118. doi:10.1177/074823379901500109
Yamasaki, K., Okuda, H., Takeuchi, T., and Minobe, Y. (2009). Effects of In Utero through Lactational Exposure to Dicyclohexyl Phthalate and P,p′-DDE in Sprague-Dawley Rats. Toxicol. Lett. 189, 14–20. doi:10.1016/j.toxlet.2009.04.023
Yang, J. H., Menshenina, J., Cunha, G. R., Place, N., and Baskin, L. S. (2010). Morphology of Mouse External Genitalia: Implications for a Role of Estrogen in Sexual Dimorphism of the Mouse Genital Tubercle. J. Urol. 184, 1604–1609. doi:10.1016/J.JURO.2010.03.079
You, L., Casanova, M., Archibeque-Engle, S., Sar, M., Fan, L.-Q., and Heck, H. D. A. (1998). Impaired Male Sexual Development in Perinatal Sprague-Dawley and Long-Evans Hooded Rats Exposed In Utero and Lactationally to P,p′-DDE. Toxicol. Sci. 45, 162–173. doi:10.1006/toxs.1998.2515
Yucel, S., Cavalcanti, A. G., Desouza, A., Wang, Z., and Baskin, L. S. (2003). The Effect of Oestrogen and Testosterone on the Urethral Seam of the Developing Male Mouse Genital Tubercle. BJU Int. 92, 1016–1021. doi:10.1111/j.1464-410X.2003.04511.x
Keywords: risk assessment, endocrine disruption, nipple retention, rats, anogenital distance, anti-androgen
Citation: Schwartz CL, Christiansen S, Hass U, Ramhøj L, Axelstad M, Löbl NM and Svingen T (2021) On the Use and Interpretation of Areola/Nipple Retention as a Biomarker for Anti-androgenic Effects in Rat Toxicity Studies. Front. Toxicology 3:730752. doi: 10.3389/ftox.2021.730752
Received: 25 June 2021; Accepted: 13 October 2021;
Published: 27 October 2021.
Edited by:
Rosaria Meccariello, University of Naples Parthenope, ItalyReviewed by:
Jennifer Anne Thompson, University of Calgary, CanadaAnderson J Martino-Andrade, Universidade Federal do Paraná, Brazil
Copyright © 2021 Schwartz, Christiansen, Hass, Ramhøj, Axelstad, Löbl and Svingen. This is an open-access article distributed under the terms of the Creative Commons Attribution License (CC BY). The use, distribution or reproduction in other forums is permitted, provided the original author(s) and the copyright owner(s) are credited and that the original publication in this journal is cited, in accordance with accepted academic practice. No use, distribution or reproduction is permitted which does not comply with these terms.
*Correspondence: Sofie Christiansen, c29jaHJAZm9vZC5kdHUuZGs=