- 1State Key Laboratory of Shale Oil and Gas Enrichment Mechanisms and Effective Development, Beijing, China
- 2School of Geoscience and Technology, Southwest Petroleum University, Chengdu, China
- 3Exploration Business Department, PetroChina Southwest Oil and Gasfield Company, Chengdu, China
In recent years, various acidizing tests have been conducted to obtain information about the industrial gas flows from acidizing tests in vertical wells JS1 and YH1 in eastern Sichuan Province, China. The results indicated that the Mao-1 Member of the Maokou Formation in southeastern Sichuan consists of good source rocks and a reservoir with high gas content. In this study, through investigations of outcrop sections, core drilling observations, thin-section identification, SEM, XRD, and TOC measurements, combined with nitrogen adsorption and logging analysis, we systematically studied the characteristics of tight argillaceous limestone reservoirs in the Mao-1 Member of southeastern Sichuan and summarized the main controlling factors for their formation. In the study area, the reservoir lithologies of the Mao-1 Member were composed of micrite limestone, bioclastic micrite limestone, and argillaceous limestone. Argillaceous limestone exhibited the highest content of clay minerals and TOC, while bioclastic micrite limestone showed relatively lower values. Six types of reservoir spaces were identified: intergranular pores, solution pores, organic pores, bioclastic-related fractures, clay mineral interlayer fractures, and macroscopic fractures. Argillaceous limestone also exhibited relatively high porosity in the Mao-1a subsegment. The reservoir pores are mainly divided into micro-pores and medium-pores, with diameters of less than 4 nm. The reservoir is primarily distributed vertically in the Mao-1a sub-member and the upper part of the Mao-1c sub-member. The formation of the tight carbonate reservoir is primarily influenced by diagenetic processes, including rock type, mineral composition, pore type, organic matter content, clay mineral transformation, dissolution, and cementation. Our analysis demonstrated that argillaceous limestone with high TOC content and good porosity is the best reservoir rock type in the study area.
1 Introduction
Nodular limestone is also known as limestone-marl alternation (Miiller and Fabrieius, 1977; Moller and Vingan, 1988). Nodular limestones have been widely recorded in shallow marine basin sediments of the Phanerozoic era (e.g., Davaud and Lombard, 1975; Courtinat, 1993; Holmden et al., 1998; Samtleben et al., 2000; Westphal and Munnecke, 2003; Chacrone et al., 2004; Rey et al., 2004). Previous studies have primarily focused on investigating their origin (Wendler et al., 2016; Westphal and Munnecke, 1997; Reinhardt et al., 2000; Westphal et al., 2000; Westphal et al., 2008). In recent years, with the further exploration of unconventional reservoirs (Fan et al., 2020a; Li et al., 2024), it has been proposed that the argillaceous limestone in nodular limestone can serve not only as a source rock for hydrocarbons but also has storage capacity. Considering the numerous fractures discovered in the argillaceous limestone, these fractures can serve both as channels for hydrocarbon migration and as storage spaces for oil and natural gas (Li et al., 2025a).
Natural gas exploration of the Permian Maokou Formation in the Sichuan Basin, China, using conventional methods began in the 1950s and continues to this day. The beach facies and weathered crust paleo-karst reservoirs in members 2–3 of the Maokou Formation form a target stratum containing a group of medium-sized gas fields (Chen, 2007; Jiang et al., 2012; Cao et al., 2022; Hao et al., 2020). Among these, the “eyeball-eyelid” structure (“nodular limestone”) in the Mao-1 Member is composed of marine carbonate source rocks that have been systematically studied (Huang et al., 2016; Liang et al., 2008; Hu et al., 2021; Huang and Lü, 2011). Recent studies have confirmed that the natural gas in the Mao-1 Member is primarily sourced from its own source rock (Li et al., 2021; Bao et al., 2024; Zhai et al., 2024). These findings suggest the existence of a new type of unconventional exploration field. The carbonate source rock gas reservoir (source-reservoir integration) in the Mao-1 Member holds great potential for natural gas exploration (Hu et al., 2018; Ma et al., 2010). In the past 2 years, significant gas volumes have been detected during tests in wells JS1, YH1, TT1, JH1, and DS1HF in the Mao-1 Member in Eastern and Southern Sichuan. Specifically, industrial gas flows of 20 × 104 m3, 12.5 × 104 m3, and 22.6 × 104 m3 have been obtained from wells TT1, JH1, and DS1HF, respectively (Li et al., 2021; Lei et al., 2020; Li et al., 2012). This outcome proves that the Mao-1 Member of the Maokou Formation in eastern Sichuan contains a substantial amount of high-quality source rock and is also a productive gas-bearing reservoir. According to the literature, the Mao-1 Member is composed of nodular limestone strata with an “eyeball-eyelid” structure and is associated with a gentle slope sedimentary environment (Hao et al., 2020; Liu et al., 2019). Its primary reservoir spaces consist of shrinkage pores and talc fractures formed by the transformation of sepiolite into talc (Li et al., 2021). Some studies suggest that the formation of nodular limestone results from the sequential enhancement of sedimentation and diagenesis (Yuan et al., 2020). It has also been reported that the Milankovitch cycle caused the original sedimentary variations in the eyeball-eyelid limestone layers. In contrast, variations in terrigenous input, organic matter content, and paleoproductivity are considered crucial to its formation (Lei, 2021). It has also been confirmed that the Mao-1 Member exhibits self-generation and self-storage characteristics. Moreover, a unique carbonate reservoir exists between a shale reservoir and a fractured reservoir (Hao et al., 2020). Although considered a carbonate source rock, its pore characteristics differ from those of a shale reservoir (Hu et al., 2019). Previous studies have focused on the origin of talc in reservoir spaces (Li et al., 2021). However, the reservoir characteristics and principal controlling factors have been inadequately studied, limiting the exploration and development of the Mao-1 Member. To address this issue, this study focuses on the marly limestone of the Mao-1 Member in the Maokou Formation of the eastern Sichuan Basin. This study combines outcrop investigation, core drilling, thin-section identification, SEM, XRD, TOC analysis, liquid nitrogen adsorption, and logging analysis to thoroughly investigate and characterize the features of tight carbonate reservoirs. Additionally, the key controlling factors of reservoir development are analyzed in detail.
2 Geological background
The Yunnan movement at the end of the Carboniferous period caused the uplift of the Sichuan Basin’s basement as a whole, which was subjected to levelling and filling (Taylor and Hayes, 2013). During the deposition of the Liangshan Formation, a large-scale transgression occurred, and the sedimentary environment was changed from terrestrial to marine. As a result, a set of clastic coastal deposits was formed in the Liangshan Formation (Yang et al., 2021; Su et al., 2020; Yang et al., 2023). During the deposition of the Qixia Formation, the sedimentary environment was transformed into a carbonate platform (Xie et al., 2022). During the sedimentary period of the Maokou Formation, based on the platform facies of the Qixia Formation sedimentary environment, a sedimentary process of rapid transgression and slow regression took place. The overall sedimentary environment exhibits a gentle carbonate slope, carbonate slope, and carbonate basin (Hao et al., 2020; Zeng et al., 2023). From southwest to northeast, the sedimentary environment of the Sichuan Basin possesses shallow, gentle slope facies, medium gentle slope facies, deep gentle slope facies, and shallow shelf facies (Figure 1a), which are characterised as being high in the southwest and low in the northeast.
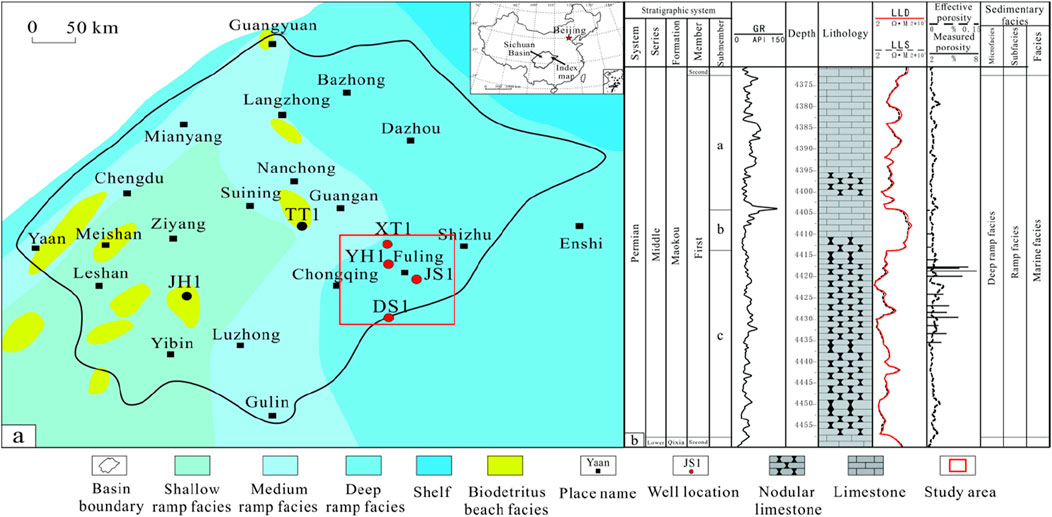
Figure 1. (a) Plane graph of the Mao-1 member, Maokou Formation, Sichuan Basin (Zhao, 2021). (b) Comprehensive well log of Well XT1.
The study area of this work is located in the southeastern part of Sichuan Province. The whole Mao-1 member has a deep, gentle slope sedimentary environment. The lithology is mainly composed of dark-grey, a grey-black, thin-thick layer of organic-containing argillaceous limestone (due to obvious external structural characteristics, referred to as an “eyelid”) and a grey-to-dark-grey medium-thick–thick layer of bioclastic micrite limestone (“eyeball”). The existence of this type of limestone constitutes a typical eyeball-like structure in the vertical direction (Figure 2a; Fan et al., 2023). According to the rock structure and the logging curve characteristics, the Mao-1 member can be divided into three sub-members, namely, a, b and c from the top to the bottom, as shown in Figure 1b. The rock type of the Mao-1a member is mainly composed of dark-grey micrite limestone, whereas grey nodular limestone can be detected at the bottom. The characteristics of the interface curve of Mao-1a and Mao-1b are obvious, while the gamma and resistivity curves exhibit significant increasing trends. The lithology type of Mao-1b sub-member is mainly composed of dark-grey micrite limestone. Mao-1b, Mao-1a, and Mao-1c exhibit obvious differences in their electrical measurement curves, with the resistivity of Mao-1b being significantly higher than those of Mao-1a and Mao-1c. The rock type of Mao-1c is mainly composed of dark-grey nodular limestone. When the Mao-1c sub-member transits to the Qixia Formation, the logging curve is characterised by a decrease in the gamma value and an increase in the resistivity.
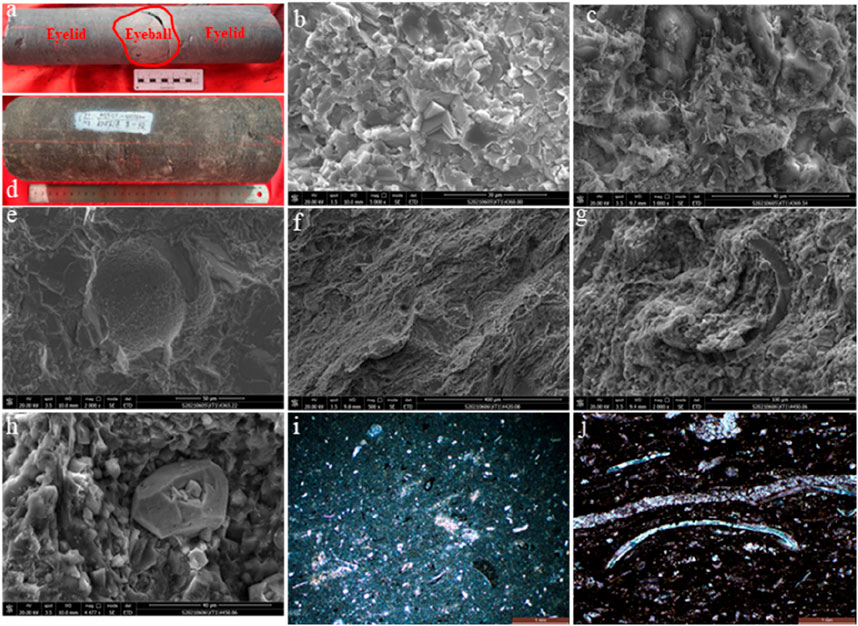
Figure 2. Rock images of the first member of the Maokou Formation in southeastern Sichuan. (a) Well XT1, 4373.57m, micritic limestone. (b) Well XT1, 4360 m, micrite limestone, calcite grain near embedded contact, see the self-shaped crystal calcite grain. (c) Well XT1, 469.54m, micritic limestone, flaky magnesium silicate mineral distribution. (d) Well XT1, 4449–4450m, nodular limestone, dark gray part is eyelid, light colour part is eyeball. (e) Well XT1, 4365.22m, micritic limestone, bioclastic. (f) Well XT1, 4420.08 m, bioclastic argillaceous limestone, argillaceous mineral lamellar distribution, directionality, in which calcareous and siliceous particles are dispersed. (g) Well XT1, 4450.06m, siliceous thin shell-like bioclasts. (h) Well XT1, 4450.06 m. Pyrite grains. (i) Well XT1, 4435.75 m, micrite limestone, see biological debris. (j) Well XT1, 4431.55 m, eyelid limestone, bioclastic development, mostly filled with calcite.
The Mao-2 and Mao-3 members of the Maokou Formation can be described as regressive system tracts. The lithology of the Mao-2 member is mainly composed of interbedded, bright, crystal bioclastic limestone, micritic bioclastic limestone, and bioclastic micritic limestone with a low shale content. The whole of the Mao-3 member is grey, thick massive limestone. A thick granular limestone is interbedded in the middle and upper parts with thin micrite limestone. In the lower part, grey bioclastic micrite limestone, and argillaceous limestone have been developed (Hu et al., 2019; Su et al., 2020; Tian et al., 2021).
3 Samples and experimental methods
The samples for this work were primarily collected from Well XT1 in the eastern Sichuan Basin. Samples of different rock types were obtained and sequentially subjected to observations with thin sections. Various measurements were conducted including SEM imaging, whole-rock XRD and TOC analyses, as well as nitrogen adsorption tests.
A total of 82 samples were prepared into thin sections for a detailed observation of their lithological characteristics. SEM observations were conducted using a FEI Quanta 250 FEG field emission scanning electron microscope. Twenty-five regular samples of 2 cm × 2 cm were prepared, and their surfaces were initially polished with sandpaper. Subsequently, some samples were further polished using an argon ion polishing machine and coated with a gold layer. After the treatment, SEM observations were carried out under a scanning voltage of 10 kV and a working distance of approximately 9 mm.
A total of 82 samples were analysed carrying out whole-rock XRD and TOC content experiments. The XRD whole-rock analysis can semi-quantitatively analyse the mineral composition of rocks. In this work, the X’pert PRO X-ray diffractometer produced by the Dutch PANalytical company was used to test the sample powder that was ground to 200 mesh. The sample powder was initially dried to a constant weight at 110°C. Then, it was evenly spread on a microscope slide after cooling, and subsequently subjected to XRD analysis. The determination of THE TOC content was performed using a Leco CS-400 carbon and sulfur analyser. The test samples were first treated with acid (a concentration of 50% hydrochloric acid) to remove inorganic carbon contaminants from the samples; then, the measurement was carried out at temperatures exceeding 800°C. During this process, the organic carbon in the samples was oxidized under high-temperature and oxygen-rich conditions, and the generated carbon dioxide was quantitatively detected by the analyser. Finally, the total organic carbon content in the samples was calculated using the external standard method (He et al., 2025; Li et al., 2025b).
Four samples were subjected to liquid nitrogen adsorption experiments to study the pore structure. A Micromeritics ASAP2460 surface area analyser was used to conduct the liquid nitrogen adsorption experiments. Oil-containing samples were required prior to the de-oiling treatment; the representative samples were ground and sieved, and 2 g of particles with sizes ranging from 20 to 40 mesh were selected and degassed under vacuum at 100°C for 10 h. Oil-containing samples were automatically de-oiled for an extended period using a Soxhlet extractor with dichloromethane until the extractant was clear. The samples were crushed using a jaw crusher and ball mill, and a vibrating sieve machine was used to select particles with sizes between 20 and 40 mesh, taking more than 10 g. The sieved particle samples were then loaded into sample tubes, placed in the degassing position of the analyser, and degassed at 105°C for 10 h. After degassing, the mass of the samples was measured using a balance with a precision of one ten-thousandth. The thermostat bath was filled with a cooling medium (liquid nitrogen for N2) to the required level; the test control software was activated to perform the test and analyse the results (using multi-point BET, BJH, and DFT methods to calculate the specific surface area and pore size distribution).
4 Results
4.1 Petrological characteristics of reservoir
The existence of micrite limestone is not common in the Mao-1 member and is mainly distributed in the middle and upper sections of the Mao-1b member. Its colour is grey and dark-grey on the whole, with calcite contents of 80%–90% (Figure 2a) and very low dolomite contents. In addition, a small amount of authigenic pyrite can be detected on the surface of these rocks. Under SEM imaging, near-embedded contacts of calcite grains, self-shaped calcite grains, and a distribution of flaky magnesium silicate minerals can be seen (Figures 2b, c).
The presence of bioclastic micrite limestone (Figure 2d) is common in the Mao-1 member of the Maokou Formation. It is mainly distributed at the bottom of Mao-1a and in Mao-1c, and is often interbedded with bioclastic argillaceous limestone. The rock colour is grey and brown-grey. The content of bioclasts is 20%–30%, the biological particles are poorly sorted and rounded, gastropods are well preserved, while ostracods and bivalves are half-shaped to broken (Figures 2e, i).
The existence of argillaceous limestone (Figure 2d) is common and is mainly developed in Mao-1a. Most of it is interbedded with dark-grey bioclastic micritic limestone. Biological debris is also common. In addition to bivalves, there are often small amounts of gastropods and ostracods mixed with deep and shallow-water organisms. The bivalves are filled with argillaceous and micritic calcite (Figures 2g, j). The SEM analysis showed that the argillaceous minerals are layered and directional, whereas calcareous and siliceous particles are dispersed and distributed. The presence of bioclasts can be also seen. The outer layer of bioclasts is a siliceous shell and pyrite grains (Figures 2f–h).
4.2 Types of reservoir spaces
According to the observations of thin sections and SEM images of different rock types in the Mao-1 member combined with existing data, it can be inferred that the reservoir spaces of the Mao-1 member are mainly formed by intergranular pores, dissolution pores, organic matter pores, fractures associated with bioclasts, and clay mineral interlayer fractures. Among them, clay mineral interlayer fractures and organic matter pores play a leading role (Liu et al., 2024).
The intergranular pores are mostly developed between the mineral grains and are primary pores. In the study area, the intercrystalline pores of Mao-1 members are mainly distributed between the calcite grains (Figures 3a–d). The intercrystalline pores between the clay minerals are mostly flaky, their pore widths range from tens to hundreds of nanometres, and the extension lengths are 1–15 μm. However, the connectivity of both is poor. This kind of pore is considered an important type of reservoir space in the carbonate reservoir of the Maokou Formation in southeastern Sichuan.
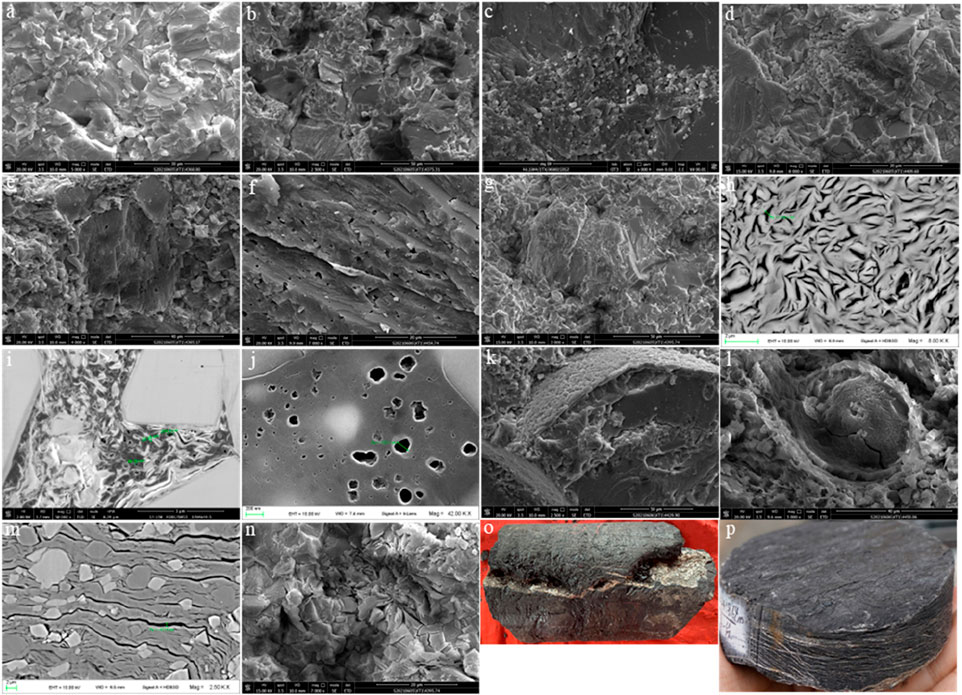
Figure 3. Rock images of the first member of the Maokou Formation in southeastern Sichuan (a) XT1 well, 4360 m, calcite intercrystalline pore. (b) XT1 well, 4375.31 m, calcite intercrystalline pore. (c) XT1 well, 4461.64m, calcite intercrystalline pore. (d) XT1 well, 4409.6 m. Intergranular pores and grain edge fractures. (e) XT1 well, 4385.17 m, calcite intragranular dissolved pore. (f) XT1 well, 4454.74 m, calcite intracrystalline dissolution pores. (g) XT1 well, 4395.74 m, calcite intragranular dissolved pore. (h) XT1 well, 4431.5m, flaky talc aggregates developed interlayer fractures. (i) YH1 well, a small amount of pores are developed in organic matter. (j) TY1 well, 2834.06m, organic pore. (k) XT1 well, 4429.90 m, bioclastic grain edge fracture, intragranular fracture. (l) XT1 well, 4450.06m, intragranular fractures. (m) XT1 well, 4420 m, lamellar talc aggregate development interlayer fracture. (n) XT1 well, 4395.74m, clay interlamellar fracture. (o) XT1 well, 4410.34–4410.50 m, eyeball limestone (calcite half filling seam). (p) XT1 well, 4420.85m, eyelid limestone, well-developed foliation.
The dissolution pores in the study area are mostly developed in calcite crystals due to calcite dissolution in the deposit. Calcite is strongly corroded, often residual and harbour-like, forming irregular dissolution pores. The dissolution pores are developed in groups. The pore size distribution of the dissolution pores is wide, ranging from tens of nanometres to tens of microns (Figures 3e–h).
After the pyrolysis of organic matter, the residual organic matter is mostly filled and broken in the intergranular pores of minerals, inducing the formation of organic hydrocarbon generation pores. Organic matter pores are mostly developed in bioclastic micrite and bioclastic argillaceous limestones with high organic matter content. Organic matter pores are relatively isolated and honeycomb-like with pore sizes ranging generally from tens to hundreds of nanometres and the connectivity is poor (Figures 3i, j).
Bioclasts are well developed in the Mao-1 member. The existence of Intercrustal and pericrustal fractures at the edges of bioclast fossil shells are considered common types of pores and fractures in carbonate rocks. The original fabric of individual large shells is mostly preserved, and the fractures are caused by an extrusion process when the shell is closely arranged with the shell or shell edge. In these regions, fractures are formed between the shell and the clay minerals (Figure 3k). It is interesting to notice that a number of the unstable shells retain some of the original fabric, while the remaining shells develop calcite grains, showing fractures in the shell. This shell part has a certain stress inheritance (Figure 3l).
When the buried depth of the formation is increasing, the ground temperature and the gradual alkalinisation of formation water are also increased. As a result, the clay minerals become dehydrated and a precipitation of a large amount of interlayer water is induced. Then, micro-fractures are formed between the layers. Considering the SEM observations of the samples from the southeastern Sichuan area, the existence of many interlayer pores and fractures developed between the layered and flaky talc aggregates in the samples can be seen, which are linear or triangular. The widths of the fractures are generally 0.5–8 μm, and the connectivity is good (Figures 3m, n).
Fractures are also developed in the core samples from the Mao-1 member. Nonetheless, most of them are half-filled with calcite (Figures 3o, p).
4.3 Reservoir distribution characteristics
The Mao-1 members in southeastern Sichuan exhibit stable and continuous formation characteristics. The reservoir is characterized by high Gamma Ray (GR) values and low resistivity values. Vertically, the Mao-1 member reservoir is mainly developed at the bottom of the Mao-1a sub-member and the top of the Mao-1c sub-member, the Mao-1b sub-member reservoir is hardly developed at all (Figure 4). The reservoir lithology is mostly argillaceous limestone, followed by bioclastic micrite limestone. Horizontally, the reservoir is mainly produced in the deep, gentle slope, argillaceous limestone microfacies and the deep, gentle slope, bioclastic micrite microfacies.
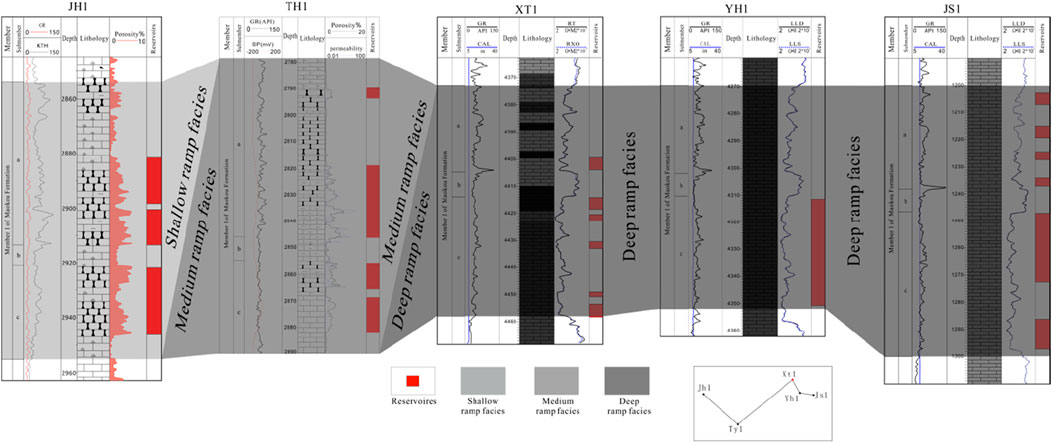
Figure 4. Sedimentary reservoir correlation diagram of the JH1 well, TY1 well, XT1 well, YH1 well, JS1 well, 1-YH1 well, and JS1 well.
4.4 Mineral composition characteristics of reservoir
The XRD results (Table 1) show that the mineral composition of the Mao-1 member of Maokou Formation in the study area is mainly calcite. In contrast, dolomite, quartz, clay minerals, siderite, pyrite, orthoclase, and plagioclase can be also seen. The clay minerals mainly include talc and a talc-mixed layer. The calcite mineral content is 12%–100%, with a mean of 82.69%. The dolomite content, the content of quartz, the content of talc, and the content of clay minerals lie between 0% and 79.5%, 0% and 86.5%, 0% and 22.4%, and 0%–19%, an average content of 6.81%,6.76%, 1.55%, and 1.34%, respectively. The clay minerals are mainly composed of a mixed layer and talc. The content of plagioclase can be found between 0% and 4.1%, with an average content of 0.12%. The content of orthoclase, siderite, and pyrite minerals is 0%–8.2%, 0%–6.5%, and 0%–4.6% with an average content of 0.2%, 0.26%, and 0.28%, respectively. In general, calcite and dolomite are the main minerals in the rock of the Mao-1 member, whereas the content of brittle minerals is high. The content of calcite in the micritic limestone is 60%–99%, the content of quartz is 0%–20%, and the content of clay minerals is very small at <3%. The calcite content in bioclastic micrite limestone is 60%–95%, the content of quartz is 1%–23%, and clay minerals is 1%–10%. The calcite content in argillaceous limestone is 55%–85%, the content of quartz is 0%–11%, and clay minerals is 10%–27% (Figure 5). Vertically, the content of calcite of Mao-1a is 60%–90%, the content of quartz is 0%–30%, and the content of clay minerals is 0%–20%. Additionally, the content of calcite of Mao-1b is 60%–90%, the content of quartz is 0%–10%, and the content of clay minerals is 3%–20%. The calcite content of Mao-1c is 60%–99%, quartz is 0%–30%, and clay minerals is 0%–20% (Figure 6).

Figure 5. XRD mineral percentage bar chart of different lithofacies. (a) Micrite limestone; (b) Bioclastic micrite limestone; (c) Argillaceous limestone.
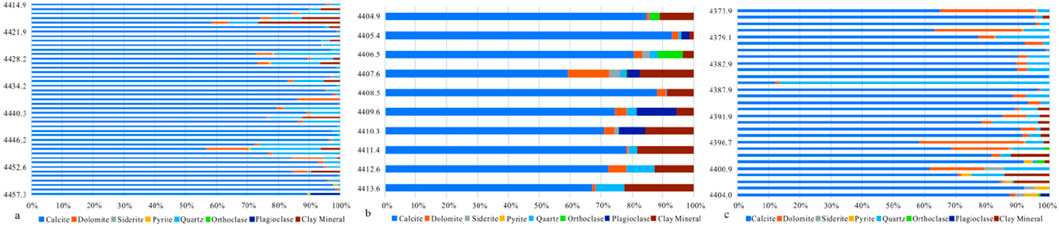
Figure 6. X-ray diffraction mineral percentage bar chart of different sub-members. (a) Mao-1a member; (b) Mao-1b member; (c) Mao-1c member.
4.5 Organic matter abundance and evolutionary stages
The range of vitrinite reflectance (Ro) values for the organic matter in the Mao-1 member, is between 1.57% and 1.60% (Table 2), indicating that the organic matter is primarily in the high maturity stage of thermal evolution. There is little difference in the degree of thermal evolution among different rock types.
According to the TOC test results of 82 samples from the Maokou Formation (Table 1), the extracted TOC values ranged from 0.11% to 1.63%, with an average of 0.51% (Figure 7a). The TOC content of argillaceous limestone was the highest, ranging from 0.26% to 1.36%, with an average value of 0.64% (Figure 7b). The samples from the Mao-1a section were the most enriched in TOC, ranging from 0.09% to 1.66%, with an average value of 0.61% (Figure 7c).
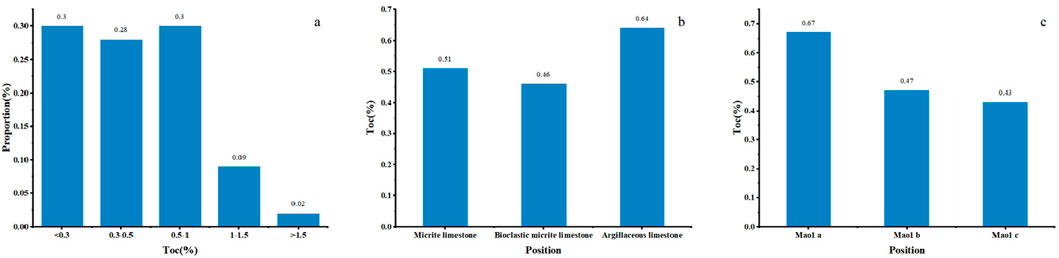
Figure 7. (a) Histogram of organic carbon distribution frequency. (b) Histogram of organic carbon content in different reservoir lithologies. (c) Histogram of organic carbon content in each sub-member of the Mao-1 member.
4.6 Reservoir physical property characteristics
The porosity distribution ranges for Micrite limestone, Bioclastic micrite limestone, and Argillaceous limestone are from 0.17% to 3.62%, 0.31%–3.01%, and 0.35%–3.78%, respectively (Figure 8a). The primary physical properties of argillaceous limestone are significantly better than those of micrite and bioclastic micrite limestones. Vertically, the porosity ranges for Mao-1a, Mao-1b, and Mao-1c sub-member are from 0.19% to 3.78%, 0.38%–1.45%, and 0.17%–3.62%, respectively, with the Mao-1a sub-member reservoir having the best physical properties (Figure 8b).
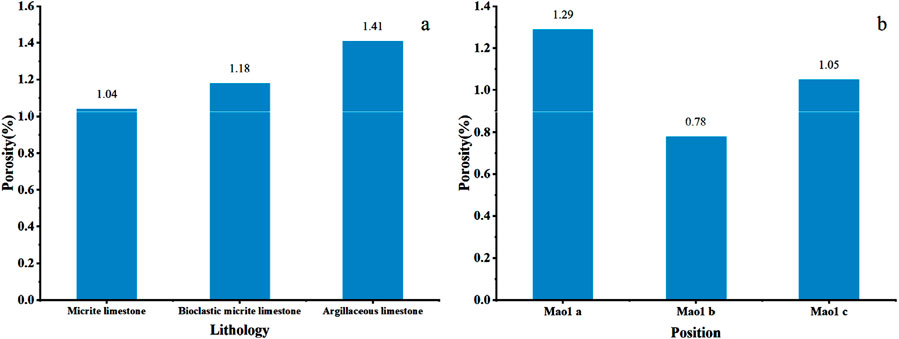
Figure 8. Physical property analysis histogram. (a) Histogram of porosity distribution in different reservoir lithologies. (b) Histogram of porosity distribution at different layers.
4.7 Pore structure of reservoir
The pore types and characteristics can be directly observed with SEM imaging. However, local and individual carbonate rock samples can be only observed, while the overall pore characteristics of a sample cannot be quantitatively assessed (Loucks et al., 2009). Therefore, in this work, four argillaceous limestone samples were selected in the reservoir section for nitrogen adsorption experiments to quantitatively observe the overall pore characteristics of the samples (Table 3). The results showed that the N2 adsorption-desorption curve of the sample is similar to the type-IV isothermal curve proposed by IUPAC (International Union of Applied Chemistry), and the whole system is transformed into a capillary condensation system of porous adsorbent (Figure 9). The adsorption capacity rapidly increased under lower relative pressure, and the curve became convex, indicating a strong interaction between the adsorbate and the surface, with the overall deviation occurring on the y-axis. No evident plateau was observed at the highest point of relative pressure, and the adsorption curve’s upward trend was obvious (Figures 9a–d). These results indicate that either there are still larger macropores in the sample, which are not filled with nitrogen, or the interaction between the adsorbate molecules is strong. The adsorption process may be continued to form a multi-molecular layer, with a continuous rise in the adsorption isotherm. In the middle section of relative pressure (P/P0), hysteresis occurs between the adsorption-desorption curves, leading to the manifestation of a hysteresis loop. According to the industry standard GBT21650.2-2008, the hysteresis loop is between H3 and H4. This result indicates that the pores in the sample have mainly a slit-like morphology, with the pores composed of flaky clay and rigid particles. An obvious inflexion point can be also seen on the desorption curve, which is caused by the difference between the capillary condensation and the evaporation of thin-necked bottle-like pores (Wei et al., 2013). According to IUPAC (1994), the shale pores can be divided into three categories: micropores (<2 nm), mesopores (2–50 nm), and macropores (>50 nm). Considering the peaks in the pore size distribution maps (Figures 9a1–d1, a2–d2) induced by adsorption and desorption branches, it can be seen that the pores in the sample are mainly micropores and mesopores <4 nm. In summary, the pore morphology of carbonate reservoirs in the Mao-1 member is mainly lamellar clay (mainly talc) interlayer fractures, slit-like pores composed of rigid particles, and fine-necked bottle-like pores. The pores are primarily micropores and mesopores <4 nm.
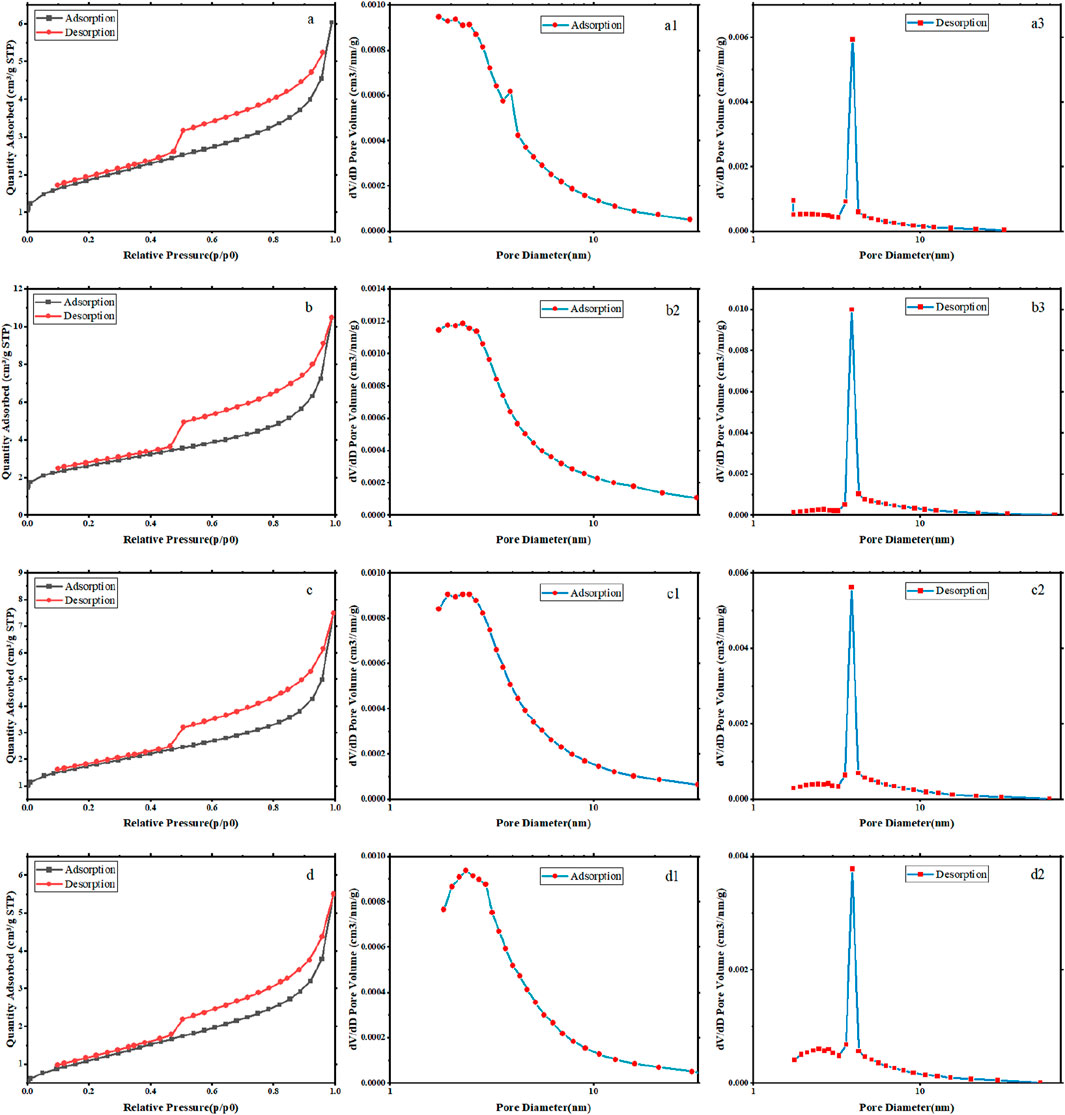
Figure 9. (a-d) Nitrogen adsorption-desorption isotherms of four argillaceous limestone samples; (a1-d1) Pore size distribution diagrams obtained from nitrogen adsorption of the samples; (a2-d2) Pore size distribution diagrams obtained from nitrogen desorption of the samples.
5 Discussion
The development of carbonate reservoirs in the Mao-1 member is affected by many factors, with lithofacies, TOC, and diagenesis playing major roles.
5.1 Effect of rock type on reservoir development
From the above-mentioned analysis, it can be inferred that the Mao-1 member reservoir is mainly developed in the deep, gentle slope, bioclastic micritic limestone, and argillaceous limestone at the bottom of the Mao-1a sub-member and top of the Mao-1c sub-member. This effect can be explained by considering that the bioclastic and argillaceous contents in the micritic limestone are low, resulting in the development of fewer inter-layer pores and organic pores in clay minerals. Meanwhile, the bioclastic contents in the bioclastic micritic limestone and argillaceous limestone and the content of clay minerals are relatively high, which facilitates the development of pores.
5.2 Organic matter content has a positive effect on reservoir performance
Organic matter pores are formed by the existence of residual organic matter in the intergranular pores of minerals, which take the form of fillings and fragments after the pyrolysis of organic matter. As can be seen from Figure 7, the TOC content has a good positive correlation with porosity (Figure 10a), specific surface area (Figure 10c) and total pore volume (Figure 10b). This outcome shows that the organic matter content has a good effect on the pore structure of the reservoir. However, the organic matter content is regarded as one of the key factors determining the reservoir performance of shale gas reservoirs. High-quality reservoirs are often found in shale development sections with high organic matter contents (Chen, 2007). For the tight marl reservoir in the Mao-1 member, due to its low overall organic matter content (average TOC = 0.51%), the impact of organic matter pores on pore characteristics is positive but weak. This result reflects the fact that TOC content is one of the main factors affecting the reservoir in the Mao-1 member.
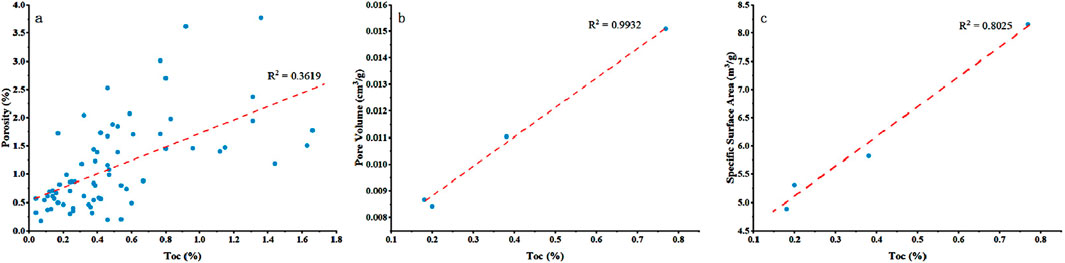
Figure 10. (a) Scatter plot of TOC versus Porosity. (b) Scatter plot of TOC versus Surface Area. (c) Scatter plot of TOC versus Pore Volume.
5.3 Diagenesis
The constructive diagenesis process that took place during the sedimentary period of the Mao-1 member mainly comprised the transformation of clay minerals and dolomitization. The cementation of calcite primarily caused destructive diagenesis.
As can be seen from the SEM results, a large number of talc shrinkage pores (fractures) are developed in Mao-1 (Figures 6h, m). It can be also observed that clay minerals are positively correlated with porosity (Figure 11b). According to the literature, sepiolite [Mg8Si12O30(OH)4·4H2O] will be transformed into talc [Mg3Si4O10(OH)2] under the combined influence of temperature and maturity Ro (Chen et al., 1985; Cai et al., 2019). In the laboratory, the structural transformation temperature of sepiolite is 310°C–330 °C (Yang and Xu, 1986). When the maturity Ro value reaches 1.2% (Yang and Xu, 1986), sepiolite will be almost completely converted into talc. Nonetheless, this procedure may not necessarily occur in nature. Yang and Xu, (1986) argued that sepiolite can be converted to talc when the burial temperature reaches 120 °C. The measurement of three samples (Table 2) showed that the Ro value of rock maturity in the study area was 1.57%–1.6%, and the geothermal temperature at the end of the early Cretaceous was up to 140°C (Li et al., 2021), which offer suitable conditions for the conversion of sepiolite to talc. With the progress of talcisation, mineral crystals will be transformed from the trioctahedral chain of sepiolite to the fibrous flakes of talc. Thus, a large number of diagenetic shrinkage fractures will be formed. At the same time, the size of the talc crystals will become smaller than that of sepiolite. Several primary pores, such as intergranular pores, will be also formed during the transformation process. These diagenetic shrinkage pores and fractures are considered important reservoir spaces for the tight reservoirs in the Mao-1 member of the Maokou Formation.
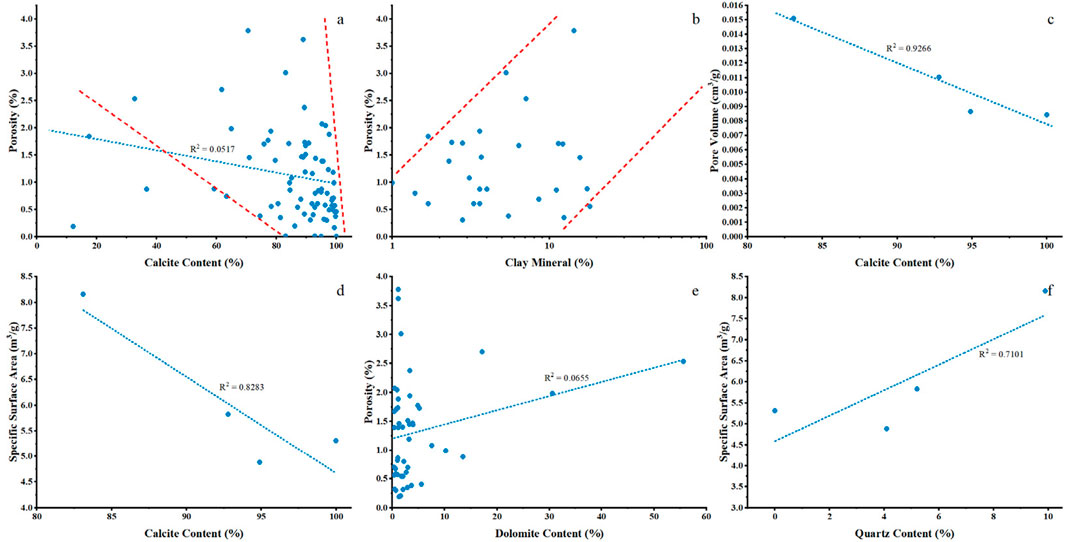
Figure 11. (a) Scatter plot of calcite versus porosity. (b) Scatter plot of clay minerals versus porosity. (c) Scatter plot of calcite versus pore volume. (d) Scatter plot of calcite versus surface area. (e) Scatter plot of dolomite versus porosity. (f) Scatter plot of quartz versus surface area.
Dolomitization is commonly developed along with the transformation of clay minerals. It is currently believed that the genetic mechanism of dolomite in the Permian strata of the Maokou Formation is associated with a thermal convection process driven by abnormal ground temperatures related to Emei ground fissure movement in a buried environment (Dong et al., 2020). Dolomite has a higher density than calcite. During the process of dolomitization, the precipitation volume of dolomite is less than the dissolution volume of calcite (Liu et al., 2021), and the porosity of the rock increases. The diagram in Figure 11e shows that there is a positive correlation between the dolomite content and porosity, indicating that dolomitization improves the Mao-1 reservoir in the study area. Another mineral, calcite, has a high content and is negatively correlated with porosity, specific surface area, and pore volume. This result also demonstrates that limestone dolomitization has a positive impact on reservoir improvement.
Dissolution is also prevalent in the Mao section of carbonate rocks in the study area, and is mainly evident as intracrystalline dissolution pores of brittle minerals such as calcite (Figures 6c, g). These dissolution pores can be used as reservoir spaces but also as fluid channels, which are conducive to the transformation of reservoirs.
In addition to clay mineral transformation, dolomitization, and dissolution, which play a constructive role in the reservoir, on the contrary, cementation (especially calcite mud crystal cementation) plays a destructive role in the reservoir. The microscopic photographs and SEM observations of the reservoir section of the Maokou Formation in the study area demonstrate that the cementation type of the reservoir section of the Maokou Formation in the study area primarily consists of calcite mud crystal and mud cementations in the eyelid tuff section with high mud content. The impact of different mineral components on the reservoir in Figure 11 reveals a negative correlation among pore volume, specific surface area, pore structure parameters, and calcite content and a positive correlation with clay mineral content (Figures 11a–d). This finding indicates that the mud crystal cementation of calcite exerts a destructive effect on the reservoir.
5.4 Petroleum geology significance
Overall, the analysis of thin sections, physical properties, XRD, nitrogen adsorption, SEM, TOC, and logging indicates that the reservoirs are mostly developed in the lower part of Mao-1a and the upper part of Mao-1c. In contrast, the reservoirs in Mao-1b are largely undeveloped. The reservoir lithofacies are mostly composed of argillaceous limestone and bioclastic micritic limestone microfacies with a high shale content. The reservoir pores mainly possess micropores and mesopores, such as organic matter pores and clay mineral shrinkage pores. The nitrogen adsorption experiments showed that the pore sizes are mostly <4 nm, mainly being micropores. Hu et al. (2007) argued that as the pore size increases, the gas stored in the pore changes from an adsorbed state to a free state. The existence of a larger pore volume leads to a higher free gas content. In addition, a larger total volume of micropores induces a bigger specific surface area (Zhong et al., 2002; Xu et al., 2009). Taking into account that the spacing between the pore walls and adsorbate is very small, the ability to adsorb gas is stronger than that of wider pores. Therefore, the force between the pore surfaces and the adsorbate is stronger than that of larger pores (Lozano Castello et al., 2002). Hence, in this kind of ultra-low-porosity and low-permeability reservoir, the presence of nano-scale pores significantly improves natural gas enrichment; however, at the same time, it complicates the overall model.
In recent years, the exploration of dense carbonate reservoirs is constantly increasing (Farouk et al., 2024a; Farouk et al., 2024b; Farouk et al., 2024c; Farouk et al., 2024d). For example, the Dalan Formation in the Permian System of the Persian Gulf, the Apollonia Formation in the western desert of Egypt, and the S1 formation in the Indus Basin on the Pakistani landmass have all achieved gas discoveries in dense limestone reservoirs (Kidambi and Kumar, 2016; Gomaa et al., 2019; Durrani et al., 2020). Afşar et al. (2014) studied the dense carbonate reservoirs in the Bristol Channel and found that argillaceous limestone exhibits a higher non-interlayer fracture percentage than micrite limestone. This result suggests that argillaceous limestone reservoirs have better reservoir properties compared to micrite limestone. These cases prove that argillaceous limestone reservoirs have good exploration prospects in the field of unconventional reservoirs. This work provides a detailed analysis of the characteristics of argillaceous limestone reservoirs and proposes the main controlling factors affecting their development, which can be compared with similar dense carbonate reservoirs globally.
6 Conclusion
In this work, a systematic analysis was performed using SEM imaging, XRD and TOC analysis, as well as studying nitrogen adsorption and other experimental data to comprehensively analyse the reservoir characteristics of Mao-1 members in southeastern Sichuan, elucidating the primary controlling factors and filling in the gaps in this area. From our analysis, the following can be drawn.
1. The reservoir rock types of Mao-1 members in southeastern Sichuan are mainly composed of micritic limestone, bioclastic micritic limestone, and argillaceous limestone. The mineral composition is primarily calcite. Clay minerals are primarily talc.
2. The TOC content in argillaceous limestone is relatively high, and it is relatively enriched in the Mao-1a sub-member. The range of vitrinite reflectance (Ro) values for the organic matter in the Mao-1 member, is between 1.57% and 1.60%, indicating that the organic matter is primarily in the high maturity stage of thermal evolution.
3. There are six main types of reservoir spaces in the Mao-1 member: intergranular pores, dissolution pores, organic matter pores, fractures related to bioclastic, interlayer fractures of clay minerals, and macroscopic fractures. The porosity of argillaceous limestone is relatively high. The overall characteristics are of a low-porosity and low-permeability tight limestone reservoir.
4. The pores of the carbonate reservoir of the Mao-1 member are mainly composed of micropores and mesopores with a diameter of about 4 nm. Their morphology is mainly slit-like pores comprising flaky clay and rigid particles.
5. The reservoir formation in the Mao-1 member is mainly affected by the lithofacies, organic matter content, clay mineral transformation, dolomitization, dissolution, and other diagenesis. The transformation of clay minerals can create a large number of shrinkage pores, which is key to the quality of the reservoir; clay minerals also adsorb a significant amount of organic matter, promoting the formation of organic matter pores and enhancing the storage performance. Dissolution processes provide additional storage space, further modifying the reservoir, while micritic cementation of calcite has a destructive effect on the reservoir. Clay minerals and argillaceous limestone with higher TOC content are the main high-quality reservoirs.
Data availability statement
The original contributions presented in the study are included in the article/supplementary material, further inquiries can be directed to the corresponding author.
Author contributions
RY: Conceptualization, Investigation, Writing - review and editing, Validation, Writing - original draft. XY: Conceptualization, Investigation, Writing - original draft, Data curation, Validation, Writing - review and editing. YZ: Conceptualization, Writing - original draft, Data curation, Investigation. YD: Investigation, Validation, Writing - original draft. LQ: Investigation, Writing - original draft. YL: Data curation, Investigation, Writing - original draft. XF: Conceptualization, Investigation, Writing - original draft.
Funding
The author(s) declare that financial support was received for the research and/or publication of this article. Open funding of State Key Laboratory of Shale Oil and Gas Enrichment Mechanisms and Effective Development (G5800-20-ZS-KFGY010).
Conflict of interest
Authors YZ, YD, and LQ were employed by PetroChina Southwest Oil and Gasfield Company.
The remaining authors declare that the research was conducted in the absence of any commercial or financial relationships that could be construed as a potential conflict of interest.
Publisher’s note
All claims expressed in this article are solely those of the authors and do not necessarily represent those of their affiliated organizations, or those of the publisher, the editors and the reviewers. Any product that may be evaluated in this article, or claim that may be made by its manufacturer, is not guaranteed or endorsed by the publisher.
Abbreviations
Mao-1 member, The Mao-1 member of the Maokou Formation; TOC, Total organic carbon content; SEM, Scanning electron microscopy; XRD, X-ray diffraction of total rock.
References
Afşar, F., Westphal, H., and Philipp, S. L. (2014). How facies and diagenesis affect fracturing of limestone beds and reservoir permeability in limestone–marl alternations. Mar. Pet. Geol. 57, 418–432. doi:10.1016/j.marpetgeo.2014.05.024
Bao, H. Y., Zhao, S., Wang, B. J., Guo, Z. F., Liu, H. T., Zhang, L., et al. (2024). Exploration breakthrough and main controlling factors for shale gas enrichment in the Permian Maokou Formation in Hongxing area in eastern Sichuan Basin. China Pet. Explor. 29 (1), 65–75. doi:10.3969/j.issn.1672-7703.2024.01.005
Cai, Z. X., Li, J., Chen, H. R., Cong, F. Y., Wu, N., Wang, L. J., et al. (2019). Genesis of Mg-phyllosilicate occurrences in the middle permian marine successions of south China. Appl. Clay Sci. 181, 105242. doi:10.1016/j.clay.2019.105242
Cao, H., Chen, Y. G., Chen, C., Gao, Z. L., Shan, S. J., Li, T. J., et al. (2022). Controlling factors of karst reservoir in Maokou Formation, northeastern Sichuan Basin. Nat. Gas Explor. Dev. 45 (3), 1–10. doi:10.12055/gaskk.issn.1673-3177.2022.03.001
Chacrone, C., Hamoumi, N., and Attou, A. (2004). Climatic and tectonic control of Ordovician sedimentation in the western and central High Atlas (Morocco). J. Afr. Earth Sci. 39 (3), 329–336. doi:10.1016/j.jafrearsci.2004.07.035
Chen, Y. J., Wang, P. Y., and Ren, L. F. (1985). Transformation of sepiolite into talc during diagenesis. Chin. Sci. Bull. 36 (4), 284–287.
Chen, Z. Q. (2007). Exploration for natural gas in middle permian Maokou Formation of Sichuan Basin. China Pet. Explor. 05, 1–11. doi:10.3969/j.issn.1672-7703.2007.05.001
Courtinat, B. (1993). The significance of palynofacies fluctuations in the greenhorn formation (Cenomanian-Turonian) of the western interior basin, USA. U. S. A Mar. Micropaleontolgy 21 (1), 249–257. doi:10.1016/0377-8398(93)90017-R
Davaud, E., and Lombard, A. (1975). Statistical approach to the problem of alternating beds of limestone and marl (Upper Oxfordian of the French Jura). Eclogae Geol. Helv. 68 (3), 491–509.
Dong, Y. X., Chen, H. D., Wang, J. Y., Hou, M. C., Xu, S. L., Zhu, P., et al. (2020). Thermal convection dolomitization induced by the emeishan large igneous Province. Mar. Pet. Geol. 116, 104308. doi:10.1016/j.marpetgeo.2020.104308
Durrani, M. Z. A., Talib, M., Ali, A., Sarosh, B., and Naseem, N. (2020). Characterization and probabilistic estimation of tight carbonate reservoir properties using quantitative geophysical approach:a case study from a mature gas field in the Middle Indus Basin of Pakistan. J. Pet. Explor. Prod. Technol. 10 (7), 2785–2804. doi:10.1007/s13202-020-00942-0
Fan, C. H., Li, H., Qin, Q. R., He, S., and Zhong, C. (2020a). Geological conditions and exploration potential of shale gas reservoir in Wufeng and Longmaxi Formation of southeastern Sichuan Basin, China. J. Petrol. Sci. Eng. 191, 107138. doi:10.1016/j.petrol.2020.107138
Fan, J. P., Song, J. M., Liu, S. G., Jiang, Q. C., Li, Z. W., Yang, Di, et al. (2023). Paleotemperature evolution and its driving mechanism during the formation of limestone-marl alternations in first member of Middle Permian Maokou Formation in Sichuan Basin. Shiyou shiyan dizhi 45 (4), 726–738. doi:10.11781/sysydz202304726
Farouk, S., Fagelnour, M., Zaky, A. S., Arafat, M., Salama, A., Al-Kahtany, K., et al. (2024b). Petroleum system evaluation: hydrocarbon potential and basin dynamics in abu darag sub-basin, northern Gulf of suez (Egypt). Minerals 14 (11), 1154. doi:10.3390/min14111154
Farouk, S., Saada, S. A., Fagelnour, M., Tawfik, A. Y., Arafat, M., and El-Kahtany, K. (2024d). Geochemical evaluation and basin modelling of the cretaceous succession in the azhar oil field, west beni suef basin, Egypt. Geol. J. 59 (7), 1949–1967. doi:10.1002/gj.4979
Farouk, S., Saada, S. A., Fagelnour, M. S., and Arafat, M. (2024a). Petrophysical and gas chromatographic analysis integration for hydrocarbon identifications in cretaceous reservoirs, azhar field, beni suef basin, Egypt. Egypt. J. Petroleum 33 (1), 7. doi:10.62593/2090-2468.1018
Farouk, S., Sen, S., Ahmed, F., Qteishat, A., Al-Kahtany, K., Moreno, H. M., et al. (2024c). Assessment of the upper cretaceous abu roash carbonate source rocks from the beni suef field, western desert, Egypt. J. Afr. Earth Sci. 215, 105272. doi:10.1016/j.jafrearsci.2024.105272
Gomaa, S., Attia, A., and Abdelhady, A. (2019). Well testing analysis of unconventional gas reservoirs: real case study of tight gas carbonate reservoir, Apollonia Formation, Western Desert, Egypt. Int. J. Petroleum Petrochem. Eng. 5 (1), 1–5. doi:10.20431/2454-7980.0501002
Hao, Y., Yao, Q. Y., Tian, H., Gu, M. F., She, M., and Wang, Y. (2020). Sedimentary characteristics and reservoir-controlling factors of the permian Maokou Formation in Sichuan Basin. Mar. Orig. Pet. Geol. 25 (3), 202–209. doi:10.3969/j.issn.1672-9854.2020.03.002
He, S., Tan, W. C., Li, H., Wang, Y., Niu, P. F., and Qin, Q. R. (2025). Mineralogical and lithofacies controls on gas storage mechanisms in organic-rich marine shales. Energy Fuel. 39 (8), 3846–3858. doi:10.1021/acs.energyfuels.4c05685
Holmden, C., Creaser, R. A., Muehlenbachs, K., Leslie, S. A., and Bergström, S. M. (1998). Isotopic evidence for geochemical decoupling between ancient epeiric seas and bordering oceans: implications for secular curves. Geology. 26 (6), 567–570. doi:10.1130/0091-7613(1998)026<0567:iefgdb>2.3.co;2
Hu, A., Pan, Y. S., Tang, J. P., Li, C. Q., and Dong, Z. X. (2007). Experiment research of adsorption and NMR on the noulding coal. Clean. Coal Technol. 13 (3), 37–40. doi:10.13226/j.issn.1006-6772.2007.03.003
Hu, A. P., Pan, L. Y., Hao, Y., Shen, A. J., and Gu, M. F. (2018). Origin, characteristics and distribution of dolostone reservoir in Qixia Formation and Maokou Formation, Sichuan Basin, China. Mar. Orig. Pet. Geol. 23 (2), 39–52. doi:10.3969/j.issn.1672-9854.2018.02.006
Hu, D. F., Wang, L. J., Huang, R. C., Duan, J. B., Xu, Z. X., and Pan, L. (2019). Characteristics and main controlling factors of the Middle Permian Maokou dolomite reservoirs in the eastern Sichuan Basin. Nat. Gas. Ind. 39 (6), 13–21. doi:10.3787/j.issn.1000-0976.2019.06.002
Hu, D. F., Wang, L. J., Zhang, H. R., Duan, J. B., Xia, W. Q., Liu, Z. J., et al. (2021). Discovery of carbonate source rock gas reservoir and its petroleum geological implications: a case study of the gas reservoir in the first Member of Middle Permian Maokou Formation in the Fuling area, Sichuan Basin. Nat. Gas. Ind. B 8 (1), 13–23. doi:10.1016/j.ngib.2020.07.001
Huang, J. Z., and Lü, Z. G. (2011). How to judge carbonate rock as source rock: a case of Sichuan Basin. Mar. Orig. Pet. Geol. 16 (3), 8–14. doi:10.3787/j.issn.1000-0976.2020.07.003
Huang, S. P., Jiang, Q. C., Wang, Z. C., Su, W., Feng, Q. F., and Feng, Z. (2016). Differences between the middle permian Qixia and Maokou source rocks in the Sichuan Basin. Nat. Gas. Ind. 36 (12), 26–34. doi:10.3787/j.issn.1000-0976.2016.12.004
Jiang, Q. C., Hu, S. Y., Wang, Z. C., Chi, Y. L., Yang, Y., Lu, W. H., et al. (2012). Paleokarst landform of the weathering crust of middle permian Maokou Formation in Sichuan Basin and selection of exploration regions. Acta Pet. Sin. 33 (6), 949–960. doi:10.7623/syxb201206005
Kidambi, T., and Kumar, G. S. (2016). Mechanical earth modeling for a vertical well drilled in a naturally fractured tight carbonate gas reservoir in the Persian Gulf. J. Pet. Sci. Eng. 141, 38–51. doi:10.1016/j.petrol.2016.01.003
Lei, H. (2021). Enesis and reservoir microscopic characteristics of eyelid-eyeball-shaped limestone in the 1st member of Maokou Formation, Sichuan Basin. China Univ. Geosci. (Beijing).
Lei, L., Zhang, L. S., and Xiong, W. (2020). Acid fracturing technology of Maokou Formation in well- dashi- 1HF, southeastern chongqing. Reserv. Eval. Dev. 10 (5), 84–90. doi:10.13809/j.cnki.cn32-1825/te.2020.05.012
Li, H., Duan, H. T., Qin, Q. R., Zhao, T. B., Fan, C. H., Luo, J., et al. (2025a). Characteristics and distribution of tectonic fracture networks in low permeability conglomerate reservoirs. Sci. Rep. 15, 5914. doi:10.1038/s41598-025-90458-6
Li, H., He, S., Radwand, A. E., Xie, J. T., and Qin, Q. R. (2024). Quantitative analysis of pore complexity in lacustrine organic-rich shale and comparison to marine shale: insights from experimental tests and fractal theory. Energy Fuel. 38 (17), 16171–16188. doi:10.1021/acs.energyfuels.4c03095
Li, H. J., Xie, X. N., Huang, J. H., Chen, H., and Lin, Z. L. (2012). Main factors controlling the formation of excellent marine source rocks in Permian Maokou formation of northwest Sichuan, China. Earth Science-Journal China Univ. Geosciences 37 (1), 171–180. doi:10.3799/dqkx.2012.017
Li, R., Su, C. P., Shi, G. S., Jia, H. F., Li, S. H., and Yu, Y. (2021). The genesis of nodular limestone reservoirs of the first period of Maokou Formation of Permian in southern Sichuan Basin. Nat. Gas. Geosci. 32 (6), 806–815. doi:10.11764/j.issn.1672-1926.2021.02.005
Li, J., Zhang, Q., Jiang, W., Li, H., Li, L., Xue, T. F., et al. (2025b). Lithological controls on pore structure and their ımplications for deep shale gas reservoir quality in the Longmaxi Formation, Luzhou area, Southern Sichuan Basin, China. Energy Fuel. 39 (3), 1541–1558. doi:10.1021/acs.energyfuels.4c05247
Liang, D. G., Guo, T. L., Chen, J. P., Bian, L., and Zhao, Z. (2008). Some progresses on studies of hydrocarbon generation and accumulation in marine sedimentary regions, southern China (Part 1): distribution of four suits of regional marine source rocks. Mar. Qrigin Pet. Geol. 13 (2), 1–16. doi:10.3969/j.issn.1672-9854.2011.03.002
Liu, J., He, X., and Xue, F. (2024). The influence of natural fractures of multi-feature combination on seepage behavior in shale reservoirs. J. Min. Strata Control Eng. 6 (1), 013437. doi:10.13532/j.jmsce.cn10-1638/td.20240018.001
Liu, J., Xia, W., Li, J. J., Zhang, X., Li, R., and Wang, L. (2019). Analysis of reservoir characteristics of the first member of Maokou Formation in southeastern Sichuan Basin. Bull. Sci. Technol. 35 (7), 26–32. doi:10.13774/j.cnki.kjtb.2019.07.006
Liu, W. D., Zhong, D. K., Yin, H., Sun, H., Liang, X., Li, R., et al. (2021). Development characteristics and main controlling factors of ultra-deep dolomite reservoirs of the Qixia Formation in the northwestern Sichuan Basin. J. China Univ. Min. and Technol. 50 (2), 342–362. doi:10.13247/j.cnki.jcumt.001203
Loucks, R. G., Reed, R. M., Ruppel, S. C., and Jarvie, D. M. (2009). Morphology, genesis, and distribution of nanometer-scale pores in siliceous mudstones of the mississippian barnett shale. J. Sediment. Res. 79 (12), 848–861. doi:10.2110/jsr.2009.092
Lozano Castello, D., Alcaniz Monge, J., Lillo, C., Cazorla Amoros, D., and Linares Solano, A. (2002). Advances in the study of methane storage in porous carbonaceous materials. Fuel 81 (14), 1777–1803. doi:10.1016/S0016-2361(02)00124-2
Ma, Y. S., Cai, X. Y., Zhao, P. R., Luo, Y., and Zhang, X. F. (2010). Distribution and further exploration of the large-medium sized gas fields in Sichuan Basin. Acta Pet. Sin. 31 (03), 347–354. doi:10.7623/syxb201003001
Miiller, J., and Fabrieius, F. (1977). Magnesian-Calcite nodules in the Ionian deep sea, an actualistic model for the formation of some nodular limestones Spec. Publs Int. Ass.Sediment. 1, 235–247. doi:10.1002/9781444304855.ch10
Moller, N. K., and Vingan, K. (1988). The genesis of nodular limestones in the Ordovician and Silurian of the Oslo Region (Norway). Sedimentology. 35 (3), 405–420. doi:10.1111/j.1365-3091.1988.tb00994.x
Reinhardt, E. G., Cavazza, W., Patterson, R. T., and Blenkinsop, J. (2000). Differential diagenesis of sedimentary components and the implication for strontium isotope analysis of carbonate rocks. Chem. Geol. 164 (3-4), 331–343. doi:10.1016/S0009-2541(99)00147-3
Rey, O., Simo, J. A., and Lorente, M. A. (2004). A record of long- and short-term environmental and climatic change during OAE3: La Luna Formation, Late Cretaceous (Santonian-early Campanian), Venezuela. Sediment. Geol. 170 (1-2), 85–105. doi:10.1016/j.sedgeo.2004.06.006
Samtleben, C., Munnecke, A., and Bickert, T. (2000). Development of facies and C/O-isotopes in transects through the Ludlow of Gotland: evidence for global and local influences on a shallow-marine environment. Facies. 43 (1), 1–38. doi:10.1007/BF02536983
Su, C. P., Tan, X. C., Wang, X. F., Li, F., Lu, F. F., Li, M. L., et al. (2020). Characteristics of eyeball-shaped limestone reservoir and its genesis of the middle permian Maokou Formation in east Sichuan Basin. Mar. Orig. Pet. Geol. 25 (1), 55–62. doi:10.3969/j.issn.1672-9854.2020.01.006
Taylor, B., and Hayes, D. E. (2013). Origin and History of the South China Sea Basin. The Tectonic and Geologic Evolution of Southeast Asian Seas and Islands: Part 2 98, 23–56. doi:10.1029/GM027p0023
Tian, X., Shi, J., Dong, J., Tan, W. C., Yin, C. H., Li, Q., et al. (2021). New understanding of sedimentary facies of the first Member of Maokou Formation based on the latest drilling in central Sichuan Basin. Nat. Gas. Geosci. 32 (11), 1646–1655. doi:10.11764/j.issn.1672-1926.2021.05.001
Wei, X. F., Liu, R. B., Zhang, T. S., and Liang, X. (2013). Micro-pores structure characteristics and development control factors of shale gas resetvoir: a caxe of longm-axi Formation in xx area of southern sichuan and northern guizhou. Nat. Gas. Geosci. 24 (05), 1048–1059. doi:10.11764/j.issn.1672-1926.2013.05.1048
Wendler, I., Wendler, J. E., and Clarke, L. J. (2016). Sea-level reconstruction for Turonian sediments from Tanzania based on integration of sedimentology, microfacies, geochemistry and micropaleontology. Palacogeogr. Palaeoclimatol. Palaeoecol. 441 (3), 528–564. doi:10.1016/j.palaeo.2015.08.013
Westphal, H., Head, M. J., and Munnecke, A. (2000). Differential diagenesis of rhythmic limestone alternations supported by palynological evidence. J. Sediment. Res. 70 (3), 715–725. doi:10.1306/2DC40932-0E47-11D7-8643000102C1865D
Westphal, H., and Munnecke, A. (1997). Mechanical compaction versus early cementation in fine-grained limestones: differentiation by the preservation of organic microfossils. Sediment. Geol. 112 (1-2), 33–42. doi:10.1016/S0037-0738(97)00033-X
Westphal, H., and Munnecke, A. (2003). Limestone-marl alternations: a warm-water phenomenon? Geology. 31 (3), 263–266. doi:10.1130/0091-7613(2003)031<0263:lmaaww>2.0.co;2
Westphal, H., Munnecke, A., and Brandano, M. (2008). Effects of diagenesis on the astrochronological approach of defining stratigraphic boundaries in calcareous rhythmites: the Tortonian GSSP. Lethaia. 41 (4), 461–476. doi:10.1111/j.1502-3931.2008.00101.x
Xie, S. Y., Wang, X. Z., Li, B., Jiang, H., Du, Y., Zhang, R., et al. (2022). Analysis of the diagenetic fluid of deep dolomitic “Leopard-Spot” limestones in the middle permian Qixia Formation in shuangyushi region in northwest Sichuan Basin, China. Front. Earth Sci. 10, 931834. doi:10.3389/feart.2022.931834
Xu, M. G., Ma, Z. H., Chen, J., and Xu, S. Q. (2009). Experimental study on influencing factors of adsorption properties of methane on coal. Mineral Eng. Res. 24 (2), 51–54.
Yang, S., Chen, A. Q., Zhang, X. H., Li, Q., Xu, S. L., Chen, C., et al. (2021). Paleogeographic transition of the permian chihsia-maokou period in the Sichuan Basin and indications for oil-gas exploration. Acta Sedimentol. Sin. 39 (6), 1466–1477. doi:10.14027/j.issn.1000-0550.2021.072
Yang, W. J., Tan, X. C., Tang, D. H., Zhang, Z. K., Hu, X., Li, M. L., et al. (2023). Sequence lithofacies paleogeography evolution of the middle permian Maokou Formation in the northwest margin of the Sichuan Basin. Front. Earth Sci. 11, 1301302. doi:10.3389/feart.2023.1301302
Yang, Z. Q., and Xu, J. W. (1986). The formation and post-sedimentary alternation of sepiolite in Pingle Depression. Bulletin 12, 31–51.
Yuan, Z., Chen, L., Mu, Z. C., Liao, L., Lai, Y. G., Li, K. L., et al. (2020). Genesis of nodular limestones from the lower triassic yinkeng Formation in chaohu region. J. Xi’an Univ. Sci. Technol. 40 (4), 672–681. doi:10.13800/j.cnki.xakjdxxb.2020.0415
Zeng, D. M., Xie, X. B., Huang, D., Yu, L. Z., and Zhang, Y. (2023). Sedimentary characteristics of the Maokou Formation of the permian in northern Sichuan Basin and its petroleum significance. J. Southwest Petroleum Univ. 45 (1), 1–12. doi:10.11885/j.issn.1674-5086.2020.12.24.04
Zhai, C. B., Lin, L. B., You, D. H., Liu, F. B., and Liu, S. Y. (2024). Sedimentary microfacies characteristics and organic matter enrichment pattern of the 1st member of the Middle Permian Maokou Formation, southwestern Sichuan Basin. Oil Gas Geol. 45 (2), 440–456. doi:10.11743/ogg20240210
Zhao, P. R. (2021). Reservoir characteristics and gas exploration potential of permian Mao- 1 member of Maokou Formation in jiaoshiba area. Reserv. Eval. Dev. 11 (5), 772–781. doi:10.13809/j.cnki.cn32-1825/te.2021.05.016
Keywords: reservoir space types, pore characteristics, unconventional reservoirs, south region of eastern Sichuan, Mao-1 member of Maokou formation
Citation: Yang R, Yang X, Zhang Y, Du Y, Qiao L, Lei Y and Fan X (2025) Reservoir characteristics and main controlling factors of argillaceous limestone in the Mao-1 Member, middle permian Maokou formation, southeastern Sichuan basin, southwest China. Front. Earth Sci. 13:1494518. doi: 10.3389/feart.2025.1494518
Received: 11 September 2024; Accepted: 17 March 2025;
Published: 07 April 2025.
Edited by:
Peng Tan, CNPC Engineering Technology R&D Company Limited, ChinaReviewed by:
Aleksandar Valjarević, University of Belgrade, SerbiaAndres Felipe Alonso Rodriguez, Great Colombia University, Colombia
Qilu Xu, China University of Petroleum, China
Jintong Liang, Chengdu University of Technology, China
Sherif Farouk, Egyptian Petroleum Research Institute, Egypt
Copyright © 2025 Yang, Yang, Zhang, Du, Qiao, Lei and Fan. This is an open-access article distributed under the terms of the Creative Commons Attribution License (CC BY). The use, distribution or reproduction in other forums is permitted, provided the original author(s) and the copyright owner(s) are credited and that the original publication in this journal is cited, in accordance with accepted academic practice. No use, distribution or reproduction is permitted which does not comply with these terms.
*Correspondence: Xiyan Yang, MTUxODE0Mjc3MDVAMTYzLmNvbQ==