- 1Shenyang Geological Survey Center of China Geological Survey, Shenyang, China
- 2Northeast Geological S&T Innovation Center of China Geological Survey, Shenyang, China
- 3Geological Research Center of Eastern Continental Edge, China Geological Survey, Shenyang, China
- 4Liaoning Geological and Mineral Survey Institute Co., Ltd., Shenyang, China
Introduction: The Jiao-Liao-Ji Belt (JLJB), situated between the Longgang Block and Nangrim Block, hosts extensive Paleoproterozoic metasedimentary-volcanic sequences of the Liaohe Group. There has always been controversy over the stratigraphic relationships between the various formations of the Liaohe Group and the tectonic evolution of the JLJB.
Methods: Field investigations, petrology, zircon U-Pb dating, and whole-rock geochemistry.
Results: The mafic rocks from the Lieryu Formation comprises gabbro and amphibolites. Zircon U-Pb geochronology yields crystallization ages of ca. 2052 ± 15 Ma, 2089 ± 55 Ma, 2053 ± 28 Ma, and 2,190 ± 13 Ma, which reconstruct the geochronological framework of Lieryu mafic magmatism to 2,190–1995 Ma. The average SiO2 content of samples ranges from 48.11 wt% to 50.47 wt%, which indicates mafic rocks. The K2O content of samples varies from 0.81 wt% to 1.44 wt% respectively, indicating calc-alkaline to high-K calc-alkaline series. The value of Mg# ranges from 45.93 to 57.10.
Discussion: The chronology of volcanic rocks cross different formations of the Liaohe Group exhibiting no systematic stratigraphic progression but rather interfingering relationships. Combined with field observations of mélange associated with boron deposits within the Lieryu Formation, it is believed that the Liaohe Group is not coherent stratigraphic succession, but a disordered tectonic stratigraphic unit. The results of trace element show that the Paleoproterozoic mafic rocks originated from a transitional mantle source, and three of them (D1902, D1905 and D1911) are mainly influenced by metasomatism, while the D1914 influenced by dual fluid and melt in the source. The D1902, D1905 and D1914 are product of partial melting of amphibole-bearing spinel lherzolite, leading to residual hornblende and spinel in the source. The D1911 is from partial melting of garnet–spinel lherzolite with the 50:50 ratios of garnet and spinel and 6:1 ratio of clinopyroxene to garnet, resulting in garnet and spinel left in the source. During the ascent and emplacement, assimilation and contamination occurred between mafic magmas and continental crustal materials. Geochemical data from the Lieryu mafic rocks reveal that D1902 (amphibolite) and D1914 (gabbro) show the same characteristics with island arc basalt (IAB), indicating an island arc environment. D1905 (amphibolite) and D1911 (gabbro) show the same characteristics with mid-ocean ridge basalt (MORB), and were formed in a mid-ocean ridge environment. These evidences indicate an ancient ocean existed in the Paleoproterozoic. The JLJB represents a collisional orogen formed during the closure of the Paleoproterozoic oceanic basin.
1 Introduction
The North China Craton (NCC) is one of the important Archean cratons in the eastern part of the Eurasian Continent. In recent years, significant progresses have been achieved on Paleoproterozoic tectonic evolution of the NCC, suggesting the existence of three Paleoproterozoic orogenic belts (Zhao et al., 2005; 2012; Zhao and Zhai, 2013), among which the Jiao-Liao-Ji Belt (JLJB) is currently the only widely accepted Paleoproterozoic orogenic belt. This orogenic belt is widely distributed in the Liaodong region and is known as the Liao-Ji tectonic belt. It is a Paleoproterozoic orogenic belt that connects the Liaonan-Nangrim Block in southern Liaoning and the Longgang Block in northern Liaoning-southern Jilin Province (Zhao et al., 2005; 2012) (Figure 1a). As an important carrier for studying the Paleoproterozoic tectonic evolution, all the Pre-Cambrian magmatic-sedimentary events have been completely preserved in the JLJB. Many research progress have been obtained on its material composition (Liu et al., 2015), sedimentation (Luo et al., 2008; Li et al., 2015a; b; Zhang et al., 2018), magmatism (Li and Zhao, 2007; Meng et al., 2014; Chen et al., 2017a; 2017b; Xu et al., 2017; 2018a; 2018b; Zhao et al., 2019), metamorphism (Liu et al., 2017; 2019), tectonism (Li et al., 2005; Tian et al., 2017), and tectonic evolution (Bai, 1993; Zhao and Zhai, 2013; Xu and Liu, 2019). However, there are still two issues of debate regarding the study of the JLJB.
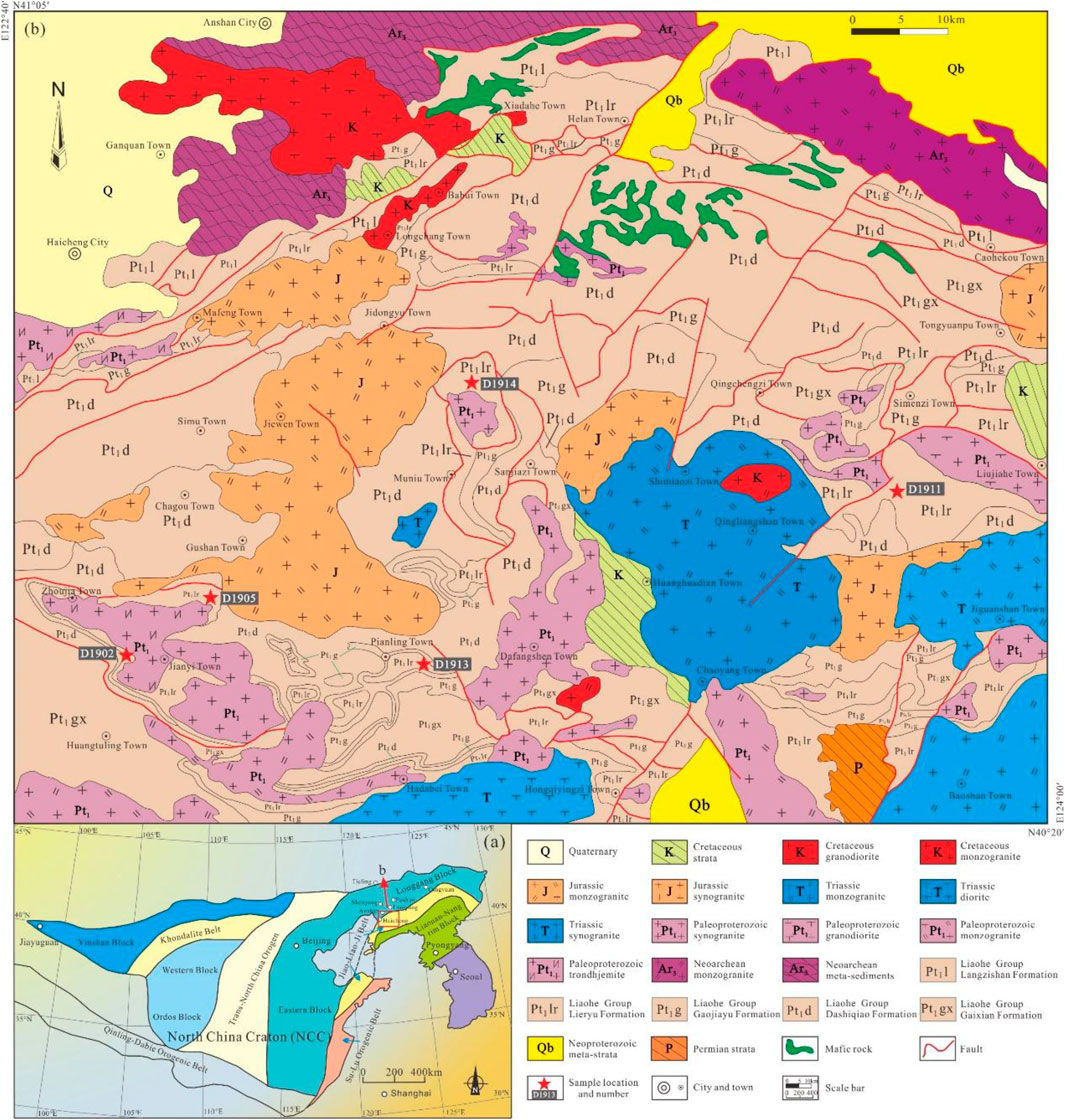
Figure 1. The tectonic location (a) (modified after Zhao et al., 2005); simplified geological map and sample location of the mafic rocks from the Lieryu Formation (b).
The first issue is whether the Lieryu Formation in the South Liaohe Group constitutes an ordered stratigraphic sequence. The Paleoproterozoic layered metamorphic sedimentary-volcanic rocks in this region are referred to as the North and South Liaohe Group in Liaoning, while in Jilin, they are known as the Laoling Group and Ji’an Group (Liu et al., 2015). There are different opinions among scholars regarding the relationship between these various rock formations within it. Early researchers posited that the Liaohe Group represents an ordered stratigraphic sequence, divided from bottom to top into the Langzishan, Lieryu, Gaojiayu, Dashiqiao, and Gaixian Formations, formed through successive depositional events during the Paleoproterozoic era (Bureau of Geology and Mineral Resource of Liaoning Province, 2014). However, recent regional geological surveys and in-depth studies on the Liaohe Group have led some scholars to propose alternative perspectives. Based on field observations and the geochronological characteristics of felsic volcanic rocks from the Lieryu Formation, the Liaohe Group is considered to be a disordered tectonic stratigraphic unit, with no clear conformable relationships among its formations (Li et al., 2015a; b; Liu et al., 2015; Chen et al., 2017a; 2018; 2020a; 2020b; 2021; 2024). In recent geological surveys, in addition to “bedded” amphibolite, marble, and granulite there is also a suite of mafic-ultramafic rocks associated with boron deposits have been observed in the Lieryu Formation from the Liaohe Group. These rocks include peridotite, gabbro, tourmaline granulite, and plagiogranite, occurring as a mélange, which further supports the possibility that the Lieryu Formation may not represent an ordered stratigraphic sequence.
The second issue is that there are still differences among scholars on the tectonic evolution model of the JLJB. The existing evolutionary model can be summarized as follows: (1) Continental rift opening-closure model: Scholars who hold this view believe that this belt was formed through the initial rifting and subsequent sedimentation of a continental rift, followed by recombination through subduction and collision (Zhang and Yang, 1988; Li et al., 2006; Zhao et al., 2005; 2011; 2012; Zhao and Zhai, 2013; Zhai and Santosh, 2011). (2) Subduction-accretion model: Some scholars, integrating modern plate tectonic theory and evidence from petrology, structure and paleogeothermal studies, propose that the belt represents a continent-arc collision zone formed through island arc accretion. Some researchers suggest that the orogenic belt is developed due to the closure of a back-arc basin (Bai, 1993; Li and Chen, 2014; Li et al., 2015a; Li et al., 2015b; Li et al., 2019a; Li et al., 2015b; Lu et al., 2006; Meng et al., 2014), with magmatic activity recording a complete tectonic cycle, including oceanic subduction, back-arc extension, back-arc basin closure, collisional orogeny, and post-collisional extension (Xu and Liu, 2019). The other researchers, supporting this model, believe that the orogenic belt formed through a complete Wilson cycle, encompassing Paleoproterozoic rifting, extension, ocean basin formation, subduction, and collision (Zhao et al., 2012; 2013; Zhai and Santosh, 2011; 2013; Li et al., 2012). (3) Continent-continent collision model: Based on the differing metamorphic grades and contrasting P-T-t evolution paths observed in the North Liaohe Group (Laoling Group) and South Liaohe Group (Ji’an Group), some scholars infer that these rock units originated from distinct continental blocks that collided and amalgamated during the Paleoproterozoic (Lu, 1996; He and Ye, 1998; Li et al., 2001).
The Lieryu Formation is characterized by extensive exposures of both felsic volcanic rocks and mafic rocks (including amphibolite, gabbro, and peridotite). Previous studies have mostly focused on the felsic volcanic rocks (Chen et al., 2017a; 2017b; Bi et al., 2018a; 2018b), but limited research on mafic rocks (Chen et al., 2020a; 2020b). Mafic magmas, originating from the subcontinental asthenosphere or lithospheric mantle under continental extension, record significant geological events and play a crucial role in reconstructing craton tectonic evolution (Mo, 2019). They can be used to study the characteristics of magma source, and the tectonic setting. So, the mafic rocks from the Lieryu Formation are the key to discuss the tectonic evolution of JLJB. So, the newly identified mafic rocks including amphibolite and gabbro were selected, petrographic, geochronological, and geochemical studies on these mafic rocks were conducted to determine the tectonic feature of the Lieryu Formation and discuss tectonic evolution of JLJB in this area.
2 Geological setting and sample descriptions
2.1 Geological setting
The Liaodong region is part of NCC and has experienced all geological events from the Archean, Proterozoic, Paleozoic, Mesozoic to Cenozoic. It mainly exhibits Archean crystalline basement, Paleoproterozoic orogenic belt, and Triassic-Cretaceous intrusive rocks formed during the destruction of the NCC. The Precambrian basement is composed of the Archean Liaonan–Nangrim Block in the southeast and the Longgang Block in the northwest, and the Paleoproterozoic JLJB in the middle (Figure 1A; Zhang, 1988). Extensive occurrences of tonalite-trondhjemite- granodiorite rocks (TTGs) (Li et al., 2019a; b, 2020; Peng et al., 2015) and mafic volcanic rock (Li and Wei, 2017) exhibit in the Longgang Block, including approximately 3.8 Ga rocks and various ≥3.0 Ga zircons exposed in the Anshan area (Wan et al., 2005). Archean granitoid rocks have been observed in the Liaonan-Nangrim Block (Zhao et al., 2006).
The Paleoproterozoic JLJB, located between these two blocks, is characterized by large-scale distribution of meta-volcano-sedimentary successions and granitic to mafic intrusions (Xu et al., 2018a; 2018b; Xu and Liu, 2019; Liu et al., 2015; Chen et al., 2017a; 2018; 2020a; 2020b; 2021; 2024). Based on the degree of metamorphism and rock composition, along the Gaixian-Ximucheng-Taziling-Aiyang, the meta-sedimentary and volcanic rocks are divided into the South Liaohe Group and Ji’an Group on the south side, and the North Liaohe Group and Laoling Group on the north side, which have undergone low greenschist to amphibolite facies metamorphism (Bai, 1993; Li and Zhao, 2007; Liu et al., 2015; Liu et al., 2017; 2019). There are also Paleoproterozoic granitic rocks and metamorphic mafic intrusive rocks collectively known as “Liaoji granite” within the JLJB. The granitic rocks include striated or gneiss granite, porphyritic garnet monzogranite, and alkaline syenite (Li and Zhao, 2007; Liu et al., 2015; Chen et al., 2021).
The lithology of the Lieryu Formation is mainly composed of metamorphic rhyolite-decite interbedded with basalt, granulite, albite granulite, magnetite granulite, biotite granulite interbedded with amphibolite, and boron-bearing serpentine marble (Bureau of Geology and Mineral Resource of Liaoning Province, 2014). In recent years, according to 1:50,000 regional geological surveys and special studies in the Liaodong area, a rock complex related to boron deposits has been identified in the Lieryu Formation of the South Liaohe Group. The rock types include gabbro, peridotite, marble, and plagioclase granite, whose occurrence shows in the form of “blocks”. This study focuses on the amphibolite and gabbro, identified from the Lieryu Formation. Geochronology and geochemistry were conducted on these sample to provide a basis for the structural properties of the JLJB.
2.2 Petrological characteristics of mafic rocks
In this study, 5 chronological samples and 24 geochemical samples of mafic rocks were collected from the Lieryu Formation for analysis, respectively. The sampling locations are shown in Figure 1b.
Sample D1902 is an amphibolite, collected from the Lieryu Formation located 5 km west of Jianyi Town (Figure 1b), intruded by later Paleoproterozoic granite (Figure 2a). It exhibits a crystalloblastic texture and a massive structure. The mainly mineral components include plagioclase (∼30%), hornblende (∼65%), and sphene (∼5%), with obvious sericitization. Hornblende is subhedral and plate-columnar-shaped, displaying a yellow-green to green interference color, with typical cleavage of amphibole. Its grain size ranges from 0.2 to 1 mm. Plagioclase shows subhedral to anhedral columnar-shaped, exhibiting polysynthetic twin with slight sericitization, with grain size ranging from 0.2 to 0.5 mm. Sphene is anhedral granular texture, featuring a medium to high protrusion with advanced white interference colors, with grain size of 0.1–0.5 mm (Figures 2b,c). The accessory mineral is magnetite, with grain size of 0.05–0.1 mm, comprising approximately 1%.
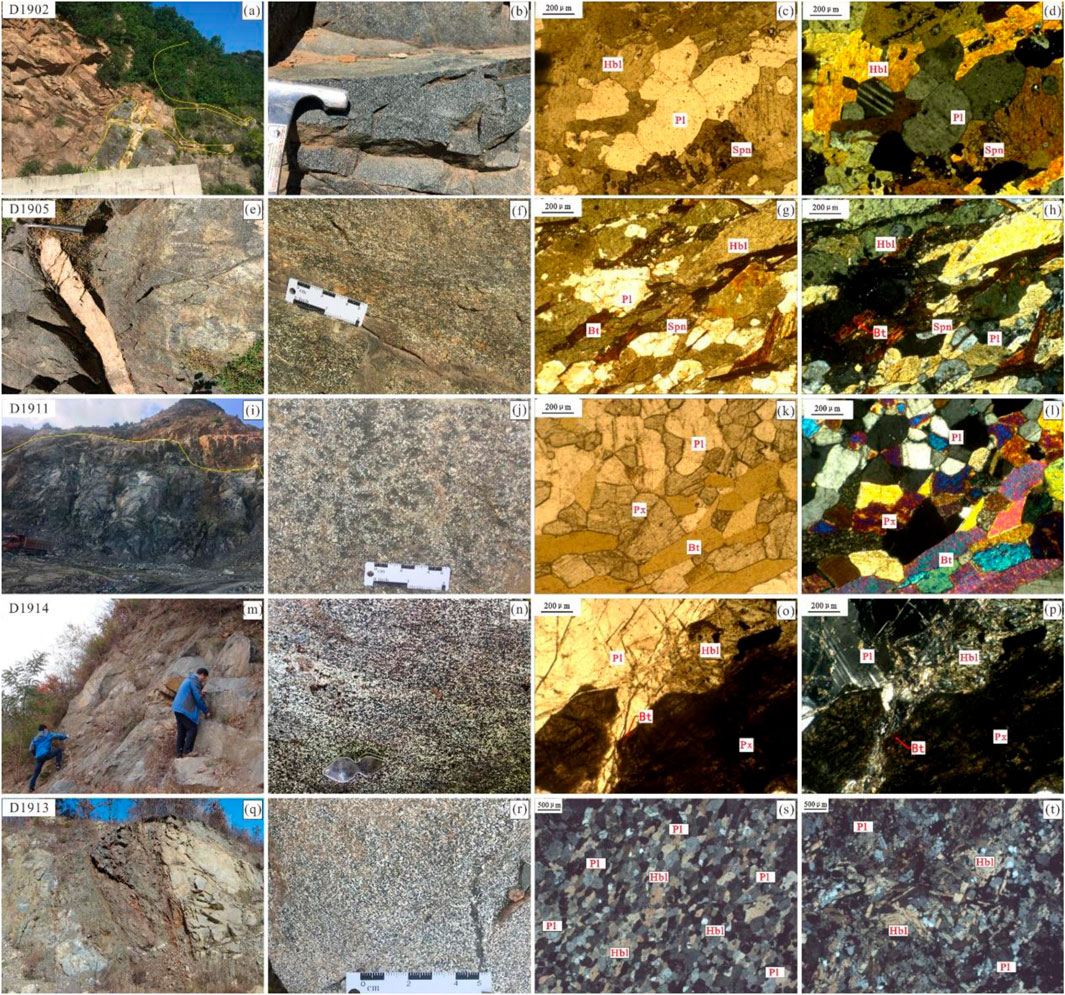
Figure 2. Occurrence and micro-pictures of mafic rocks from the Lieryu Formation. (a–d) D1902 amphibolite; (a) amphibolite xenolith in the granite; (b) fresh surface of the amphibolite; (c,d) microscopic characteristics of amphibolite; (e–h) D1905 amphibolite; (e) amphibolite is intruded by granite dike; (F) specimen of diabase; (g,h) microscopic characteristics of amphibolite; (i–l) D1911 gabbro; (I) gabbro is intruded by granite; (j) spherical weathering of gabbro; (k,l) microscopic characteristics of gabbro; (m–p) D1914 gabbro; (m) field occurrence of gabbro; (n) coarse-grained gabbro; (o,p) microscopic characteristics of gabbro; (q–t) D1913 amphibolite; (q) field occurrence of amphibolite; (r) spherical weathering of amphibolite; (s,t) microscopic characteristics of amphibolite; Pl: plagioclase; Px: pyroxene; Hbl: hornblende; Spn: sphene; Bt: biotite.
Sample D1905, amphibolite, sourced from the Lieryu Formation, 7 km south of Gushan Town (Figure 1b). It is intruded by later felsic dike (Figure 2e). It is composed of minerals such as hornblende (∼60%), plagioclase (∼30%) biotite (∼6%) and sphene (∼3%) exhibiting crystalloblastic texture with foliated structure (Figure 2F). Hornblende appears subhedral and plate-columnar-shaped, yellow-green to green interference color, oriented along its long axis with a foliated structure and a particle size of 0.2–1 mm. Plagioclase is subhedral to anhedral columnar-shaped, showing polysynthetic twin with slight sericitization, with a grain size of 0.2–0.5 mm. Biotite, euhedral, brown in color, and is oriented in a foliated plane, ranging from 0.1 to 0.5 mm in diameter. Sphene shows anhedral granular texture, exhibiting a medium to high protrusion and advanced white interference colors, ranging from 0.1 to 0.5 mm in diameter. The magnetite, as accessory mineral, exhibits a particle size of 0.05–0.1 mm and a content of about 1% (Figures 2g,h).
Sample D1911 is a gabbro collected from the Nuanhe Boron Mine, located 12 km west of Liujiahe Town (Figure 1b). It exhibits a crystalblastic texture and a massive structure (Figures 2i,j). The mineral components are pyroxene (50%), plagioclase (40%), and biotite (10%), with sericite as the alteration mineral. The colorless to light green pyroxene is subhedral and plate-columnar-shaped, with typical cleavage of pyroxene, forming dark layers with biotite, and its grain size ranges from 0.2 to 2 mm. Subhedral and plate-columnar-shaped plagioclase shows polysynthetic twin with slight sericitization, often forms light-colored bands, with a diameter ranging from 0.2 to 1 mm. The euhedral biotite is light yellow to yellow in color, and forms dark layers with pyroxene, with a grain size of 0.2–2 mm (Figures 2k,l). Accessory minerals include small amounts of magnetite and others.
Sample D1913, amphibolite, is collected from the Lieryu Formation, 5 km east of Pianling Town (Figure 1b), showing a crystalloblastic texture and a massive structure (Figures 2q,r). The components include hornblende (50%), plagioclase (40%), epidote (7%), and quartz (3%), with notable sericitization and epidotization. Hornblende is subhedral and columnar, exhibiting a green to yellow-green color, and has a diameter of 0.2–1.6 mm. Plagioclase is also subhedral, showing polysynthetic twin with slight sericitization, with a grain size of 0.2–1.2 mm. Epidote is anhedral and granular, exhibiting positive high protrusion and abnormal interference colors, with a grain size of 0.1–0.4 mm. Quartz, with a grain size of 0.2–2.0 mm, is anhedral and granular (Figures 2s,t).
Sample D1914, hornblende gabbro, collected from 10 km southeast of Jidongyu Town (Figure 1b), exhibits a gabbroic texture and a massive structure (Figures 2m,n). The rock is composed of plagioclase (45%), pyroxene (50%), hornblende (4%), and biotite (1%), with alteration minerals including chlorite, sericite, and calcite. The plagioclase, subhedral columnar crystals ranging from 2 to 5 mm in diameter, shows polysynthetic twin and zoning, with slight sericitization along fractures. The light yellow to green pyroxene is subhedral and columnar, with slight carbonation and a dominant grain size of 1–2 mm. Hornblende is subhedral, exhibiting typical amphibole cleavage, and shows chloritization and carbonation with a few areas exhibiting reaction rims at the edges of pyroxene, and has a grain size of 0.5–2 mm. Biotite is euhedral, brown in color, with a grain size of 0.2–1 mm (Figures 2o,p). Accessory minerals include small amounts of magnetite and ilmenite.
3 Analytical methods
3.1 Sample preparation
After collection from the field, samples for geochronological analysis were cleaned, crushed, and ground. Zircon crystals were subsequently separated from these samples using conventional heavy liquid and magnetic techniques at Langfang Yuneng Mineral Separation Co., Ltd. in Langfang, China. The isolated zircons were carefully hand-picked under a binocular microscope. To investigate their internal structures, the selected zircons were embedded in epoxy resin, polished, and then imaged with a scanning electron microscope equipped with cathodoluminescence (CL) at Beijing Gaonian Navigation Technology Limited Company in Beijing, China. CL images of four samples were obtained using a CAMECA SX51 microprobe (manufactured by CAMECA in France), operating at 50 kV and 15 nA.
3.2 Zircon LA-ICP-MS U-Pb isotope dating
LA-ICP-MS U-Pb isotope analyses were conducted using an Agilent 7500a quadrupole ICP-MS (Agilent Technologies Co., Ltd) equipped with an UP-193 solid-state laser (193 nm, New Wave Research Inc., Shanghai). The laser spot size was set to 32 μm for most analyses, with a laser energy density of 10 J/cm2 and a repetition rate of 8 Hz. The laser sampling procedure included a 30-s blank, followed by 30 s of sampling ablation, and a 2-min sample chamber flushing after ablation. The ablated material was transported to the ICP-MS by a high-purity helium gas stream with a flow rate of 1.15 L/min. The entire laser path was purged with argon (600 mL/min) to maintain energy stability. Counting times were 20 ms for isotopes 204Pb, 206Pb, 207Pb, and 208Pb; 15 ms for 232Th and 238U; 20 ms for 49Ti; and 6 ms for other elements. NIST 610 glass served as an external standard, while Si was used as an internal standard for zircon analyses. U-Pb isotope fractionation effects were corrected using zircon 91,500 as an external standard (Wiedenbeck et al., 1995). Glitter software was used to calculate isotopic ratios and element concentrations of zircons (Ludwig, 2003). Concordia ages and diagrams were generated using Isoplot/Ex (3.0) (Ludwig, 2003). Common lead was corrected using the LA-ICP-MS Common Lead Correction (version 3.15), following the method of Andersen (2002). The analytical data are presented on U-Pb concordia diagrams with 1σ errors. Mean ages are reported as weighted means at 95% confidence intervals. The analyses of 5 samples were performed at the Key Laboratory of Mineral Resources Evaluation in Northeast Asia, Ministry of Natural Resources. The data are summarized in Table 1.
3.3 Major- and trace-element analyses
The major, trace, and rare earth element (REE) analyses results for 24 samples were obtained from the Northeast China Supervision and Inspection Center of Mineral Resources, Ministry of Natural Resources (Shenyang, China). After petrographic examination and the altered rock surfaces were removed, the samples were crushed and ground to 74 μm using an agate mill. X-ray fluorescence spectrometry (XRF) method was used for the major element contents of the whole rock, with analytical precisions exceeding 2%. X Series II ICP-MS was used for the trace element and REE contents testing. A total of 0.1 g of the sample was placed in a digestion tank, with 1 mL of concentrated nitric acid and 1 mL of hydrofluoric acid added. After placed in an oven, the digestion tank was heated up to 180° for 10–12 h. After removal, it was heated at 120° on an electric heating plate in an open environment. Until only 2–3 mL of the digestion solution was left, it was heated up to 240°. After redissolving, 0.5% dilute nitric acid was added to the mark for measurement. Twelve times measurements were conducted on samples, with analytical precisions exceeding 5% for elements with contents of >10 μg/g, exceeding 8% for elements with contents of <10 μg/g, and 10% for the transition metals. The results are listed in Supplementary Table S1.
4 Analytical results
4.1 Zircon U-Pb geochronology
Twelve isotopic analyses were conducted on zircons from sample D1902. They are mostly euhedral rhombohedral with diameters of 100–140 μm and length-width ratios of 1.5:1 to 2:1, generally black, but unclear internal texture (Figure 3). Light edges with different widths occurred around the most zircons, which may be the proliferative edges formed by recrystallization during metamorphism. The Th/U ratios are ranging from 0.75 to 1.96, which indicates a magmatic origin (Hoskin and Ireland, 2000; Belousova et al., 2002). Twelve analyses yield an upper intercept age of 2052 ± 15 Ma (n = 12, MSWD = 0.02), which is consistent with the 207Pb/206Pb weighted average age of 2056 ± 11 Ma (n = 4, MSWD = 0.82) from 4 zircons on the concordant line (Figure 4a). Thus, this result represents the magmatic crystallization age.
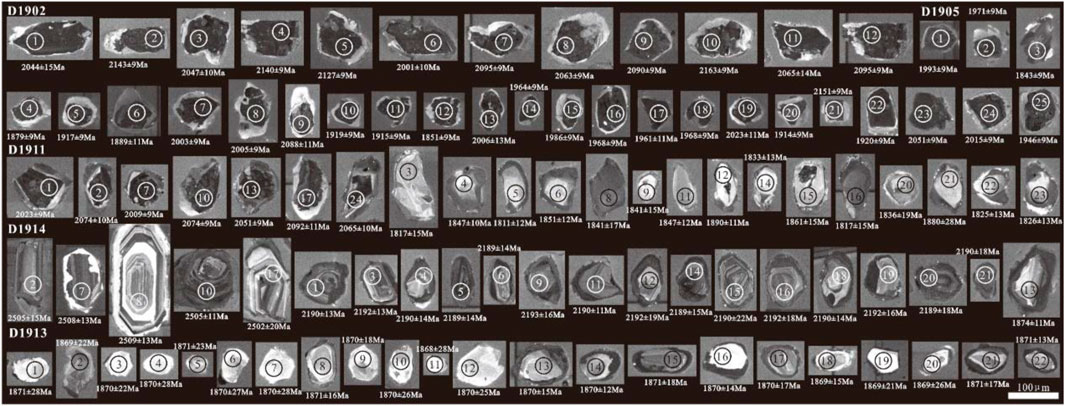
Figure 3. Cathodoluminescence (CL) images of the selected zircons from the mafic rocks in the Lieryu Formation. The circles on zircons represent analyzed spots.
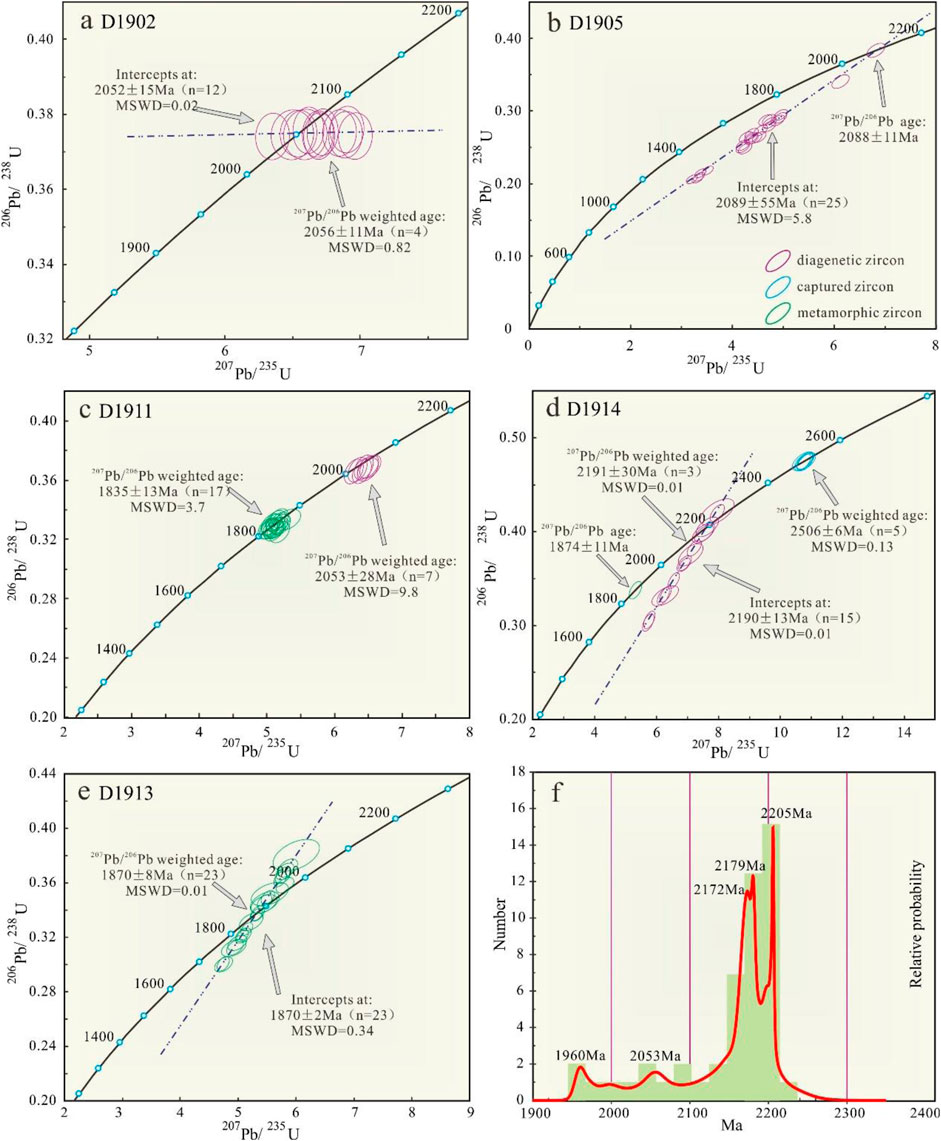
Figure 4. Concordia diagrams for zircons analysed from mafic rocks (a–e) and summary of geochronological data of volcanic rocks (f) in the Lieryu Formation.
Twenty-five zircons have been chosen from D1905. In cathodoluminescence (CL) imaging, they are smaller in size with diameters of 40–70 μm and length-width ratios of 1:1 to 2:1, generally black mostly, without clear internal texture (Figure 3). Light secondary outgrowth rims with different widths occurred around all the zircons, which may be the product of recrystallization during metamorphism (Figure 3). Combining with high Th/U ratios (0.48–3.38), indicating zircons in this sample are magmatic-derived zircon (Hoskin and Ireland, 2000; Belousova et al., 2002). Twenty-five analyses yield an upper intercept age of 2089 ± 55 Ma (n = 25, MSWD = 5.8), which is consistence with the 207Pb/206Pb age (2088 ± 11 Ma) in the concordant line (Figure 4B). Thus, this age is interpreted as the time of magmatic emplacement.
Zircons from sample D1911 can be divided into two groups according to the CL images (Figure 3). The shape of first group zircons is sub-angular with grain size ranging from 80 μm to 110 μm and length/width ratios of 1:1–2.5:1. The black cores and gray rims are present in these grains with no obvious zoning. The ratios of Th/U range from 0.59 to 1.65, indicating a magmatic origin (Hoskin and Ireland, 2000; Belousova et al., 2002). Seven analysis were obtained from seven zircons, yielding a concordant 207Pb/206Pb age of 2053 ± 28 Ma (n = 7, MSWD = 9.8) (Figure 4d). This result is interpreted as the age of magma crystallization. Zircons from the second group is sub-rounded with size ranging from 50 μm to 120 μm and length/width ratios from 1:1–2.5:1. The white cores with unzoned internal texture (Figure 3) and low ratio of Th/U (0.05–0.10), indicating a metamorphic zircon (Hoskin and Ireland, 2000; Belousova et al., 2002). Seventeen analysis were obtained from seventeen zircons, yielding a concordant 207Pb/206Pb age of 1835 ± 13 Ma (n = 17, MSWD = 3.7) (Figure 4D). This result is interpreted as the time of later metamorphism.
In cathodoluminescence (CL) imaging, zircons from sample D1914 can be divided into three groups. The zircons of first group show large grain size with the diameter of 110–210 μm and length/width ratios of 2:1–4:1. The zoning cores with clear internal texture and white to gray unzoned rims are present in these grains. The ratios of Th/U range from 0.55 to 0.93, indicating a magmatic origin (Hoskin and Ireland, 2000; Belousova et al., 2002). Five analyses were obtained from five zircons, yielding a concordant 207Pb/206Pb age of 2,506 ± 6 Ma (n = 5, MSWD = 0.13). This age is interpreted as the captured zircons from the surrounding Archean rocks. The size of zircons from the other two groups is smaller with the diameter of 60–110 μm and length/width ratios of 1:1–2:1. The internal texture of zircons from the second group is clear with obvious oscillatory zoning. Combining with high Th/U ratios (0.43–0.83), indicating magmatic-derived zircon (Hoskin and Ireland, 2000; Belousova et al., 2002). Fifteen analyses yield an upper intercept 207Pb/206Pb age of 2,190 ± 13 Ma (n = 15, MSWD = 0.01), which is consistent with the 207Pb/206Pb weighted average age of 2,191 ± 30 Ma (n = 3, MSWD = 0.01) from 3 zircons on the concordant line (Figure 4a). This age is interpreted as the time of magmatic emplacement. The only one zircon (data 13) belongs to the third group. It exhibits white core, unzoned internal texture (Figure 3) and low ratio of Th/U (0.03), which indicates a metamorphic zircon (Hoskin and Ireland, 2000; Belousova et al., 2002). This zircon shows a concordant 207Pb/206Pb age of 1874 ± 11 Ma (Figure 4a) and is interpreted as a later metamorphism.
Zircons from sample D1913 are anhedral subrounded ranging in size from 30 to 65 μm with length/width ratios from 1:1–2:1. The white unzoned cores show unclear internal texture in these grains (Figure 3). With the Th/U ratios ranging from 0.01 to 0.09, these zircons were considered to be a metamorphic origin [43‒46]. A total of twenty-three U-Pb isotopic analyses were conducted on zircons, yielding an upper intercept 207Pb/206Pb age of 1870 ± 2 Ma (n = 23, MSWD = 0.34), which is consistent with the weighted average 207Pb/206Pb age of 1870 ± 8 Ma (n = 23, MSWD = 0.01) (Figure 4C). This result is interpreted as the metamorphic age.
4.2 Major and trace element geochemistry
The average SiO2 content of the amphibolite (D1902) is 50.47 wt%, while the other three mafic rocks show a lower average SiO2 content (48.11–48.80 wt%). The Al2O3 content is moderate (12.63–14.14 wt%). The average total alkali content (K2O + Na2O) is moderate, ranging from 2.77 to 4.69 wt%. On the TAS diagram (Figure 5a), all the samples belong to the gabbro, and on the R1-R2 diagram (Figure 5b), they fall within the olivine-gabbro (olivine basalt) and gabbro norite area. The K2O content of samples is variable, with average values from 0.81 wt% to 1.44 wt% respectively. On the SiO2-K2O diagram, the samples belong to the calc-alkaline to high-K calc-alkaline series (Figure 5c), and Ta/Yb vs. Ce/Yb ratios allow an allocation to the calc-alkaline series (Figure 5d). The average Fe2O3 content of the samples is 1.59–3.20 wt%, with a high average FeO content of 9.13–11.43 wt%, an average MgO content of 6.79–8.04 wt%, an average MnO content of 0.18–0.23 wt%. Mg# ranges from 45.93 to 57.10, which is a little lower than that of primary basaltic rocks (Mg# = 70, (Dupuy and Dostal, 1984). The average TiO2 content of the amphibolite (D1905) is high (2.58 wt%) relatively, while the other three mafic rocks show a lower average TiO2 content ranging from 1.02 wt% to 1.13 wt%. The amphibolite (D1905) exhibits a relatively low average CaO content (8.97 wt%) and high P2O5 content (0.26 wt%), while the other three mafic rocks show a relatively high CaO content (10.02–10.42 wt%) and very low P2O5 content (0.07–0.12 wt%).
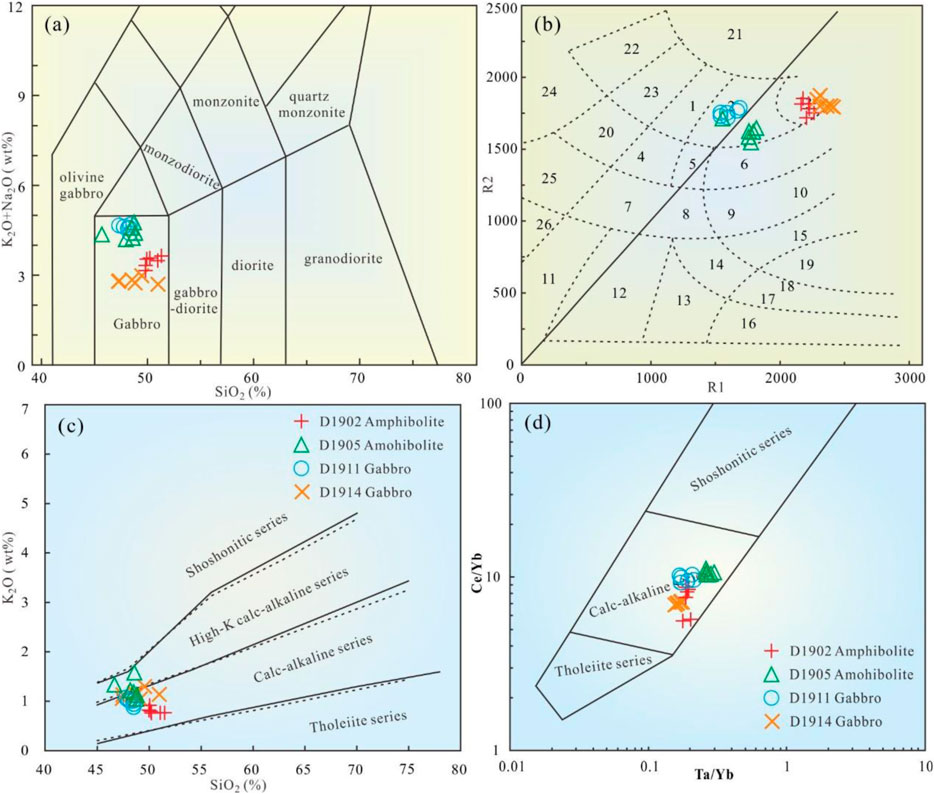
Figure 5. SiO2 vs. total alkali (Na2O + K2O) (a), after (Middlemost, 1994), R1 vs. R2 (b), after (De la Roche et al., 1980), SiO2-K2O (c), after (Peccerillo and Taylor, 1976) and Ta/Yb vs. Ce/Yb (d), after (Pearce, 1982) diagrams for mafic rocks from Lieryu Formation. (b) 1—alkaline gabbro (alkaline basalt); 2—olivine gabbro (olivine basalt); 3—gabbro norite (tholeiite); 4—syenite gabbro (trachyte basalt); 5—monzonite gabbro (andesite coarse basalt); 6—gabbro (basalt); 7—trachyandesite (syenite); 8—monzonite (andesite); 9—monzodiorite (trachyte); 10—diorite (andesite); 11—nepheline syenite (trachyte phonolite); 12—syenite (trachyte); 13—quartz syenite (quartz trachyte); 14—quartz monzonite (quartz andesite); 15—tonalite (dacite); 16—alkaline granite (alkaline rhyolite); 17—syenogranite (rhyolite); 18—monzogranite (dacite rhyolite); 19—granodiorite (rhyolite dacite); 20—essenite-aegirine gabbro; 21—peridotite (picrite); 22—nepheline (picrite nepheline); 23—qilieyan (basanite); 24—neonite (nepheline); 25—essenite; 26—nepheline syenite (phonolite).
These mafic rocks show different characteristics of rare earth elements (REEs) and trace elements. The amphibolite (D1902) and the two gabbro (D1911, D1914) show low contents of total REEs, with average contents of 54.02 μg/g, 59.18 μg/g and 51.28 μg/g, respectively. They exhibit similar characteristics to enriched mid-ocean ridge basalt (E-MORB) on the chondrite-normalized rare earth element (REE) diagram, with a right-skewed smooth curve (Figure 6a), indicating an enrichment of light rare earth elements (LREEs) and a flat distribution pattern of heavy rare earth elements (HREEs). The differentiation between LREEs and HREEs is low, with an average (La/Yb)N value of 2.08–4.43 and a slight positive europium anomaly (Eu/Eu* = 1.03–1.21) (Figure 6a). The other amphibolite (D1905) shows same trend with E-MORB, but with a high average REEs contents, which is 137.70 μg/g (Sun and McDonough, 1989). On the chondrite-normalized REE diagram, the samples show a right-skewed curve (Figure 6a), indicating a slight differentiation between them, with average (La/Yb)N values of 3.31. This amphibolite shows a slight negative europium anomaly (Eu/Eu* = 0.92–0.97) (Figure 6a).
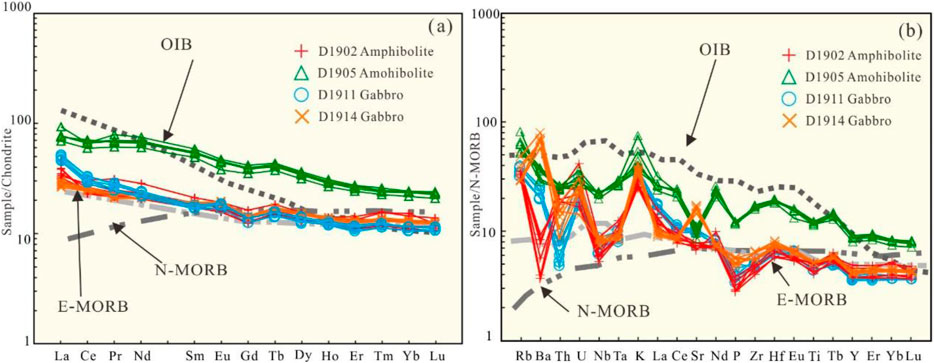
Figure 6. Chondrite-normalized rare earth element patterns (a) and primitive mantle-normalized trace element spider diagram (b) for the mafic rocks from Lieryu Formation. (The values of chondrite and primitive mantle are from (Sun and McDonough, 1989).
In the primitive mantle-normalized trace element spider diagram, these mafic rocks demonstrate different characteristics. The amphibolite (D1902) exhibits significantly varying incompatible elements, with an enrichment of large ion lithophile elements (LILEs) (Rb, K, etc.), depletion of high field strength elements (HFSEs) (Nb, Ta, Zr, Ti, etc.), and slight negative anomalies of Sr and P, as well as positive anomalies of Th and U (Figure 6b). The average contents of Cr, Co and Ni are 52.0 μg/g, 41.3 μg/g and 19.3 μg/g respectively. The other amphibolite (D1905) shows relatively high overall elemental content, with an enrichment of LILEs (Rb, Ba, K, etc.), and strong negative anomalies of Sr and P, as well as slight positive anomalies of U, Zr and Hf (Figure 6b). The average contents of Cr, Co and Ni are 132 μg/g, 33.1 μg/g and 49.1 μg/g respectively, which is slightly higher than N-MORB. The two gabbro (D1911, D1914) have a roughly same pattern, with an enrichment of LILEs (Rb, Ba, K, etc.), depletion of HFSEs (Th, Nb, Ta, Ce, Zr, Ti, etc.), and negative anomalies of P. The gabbro shows positive anomalies of Sr, with the D1911 being a slight positive anomaly and the D1914 being a strong positive anomaly (Figure 6b). They have different contents of Cr, Co and Ni, with the contents of Cr (with average contents of 22.0 μg/g and 189.0 μg/g), Co (with average contents of 43.5 μg/g and 46.7 μg/g), and Ni (with average contents of 38.0 μg/g and 68.4 μg/g).
5 Discussion
5.1 The limitations of geochronology on the relationships between the formations of the Liaohe group
Since the initial investigations of the Liaohe Group in the late 1930s, this Precambrian sequence has been conventionally interpreted as an ordered stratigraphic column comprising, from bottom to top: the Langzishan, Lieryu, Gaojiayu, Dashiqiao, and Gaixian Formations (Liaoning Bureau of Geology and Mineral Resources, 2017). These units were historically perceived to exhibit an orderly stratigraphic succession from bottom to top in the typical sections of the Liaohe Group. However, recent investigations have challenged this traditional stratigraphic framework. Based on lithological assemblages and zircon geochronological data, Li et al. (2014) proposed that the Langzishan Formation correlates temporally with the Gaixian Formation of the southern Liaohe Group, suggesting its uppermost position within the sequence. The age of the amphibolite obtained from the Langzishan Formation also indicates that the Langzishan Formation is no longer suitable to be placed beneath the Lieryu Formation (Chen et al., 2018). The preservation of original stratigraphic relationships remains questionable given the complex Paleoproterozoic tectonic evolution that affected the region (Zhao et al., 2012; Liu et al., 2015). Field investigations by Chen et al. (2017a) along the “typical sections” revealed that most metamorphic rocks from the Liaohe Group exhibit characteristics of contact and dynamic metamorphism, with various lithologies occurring in interlayered patterns. Crucially, the boundaries between formations predominantly manifest as ductile shear zones rather than conformable contacts (Chen et al., 2017a). Furthermore, the coeval presence of low-grade and high-grade metamorphic sequences within both the Gaojiayu and Gaixian Formations (Liu et al., 2015) provides additional evidence against the preservation of an intact stratigraphic succession. These findings collectively suggest that the Liaohe Group is no longer orderly strata, but represent a structural rock assemblage resulting from polyphase metamorphic and deformation events.
Chronostratigraphic studies reveal significant variations in the formation ages of different lithostratigraphic units within the Liaohe Group (Table 2). The mafic volcanic rocks from the Langzishan Formation yield a crystallization age of 1952 ± 38 Ma (Chen et al., 2018), while detrital zircon analyses constrain their depositional age to post-2078 Ma (Luo et al., 2004). In contrast, the Lieryu Formation exhibits the most extensive volcanic exposures, though previous investigations predominantly focused on felsic rocks. These studies reveal a long formation time of the felsic rocks ranging from 2,229 to 1959 Ma (Li and Chen, 2014; Li et al., 2015a; Li et al., 2015b; Li et al., 2019a; Chen et al., 2016; 2017a; Meng et al., 2017; Bi et al., 2018b; Liu et al., 2018; Xu et al., 2019). Amphibolite geochronology from this formation constrains its mafic magmatism to 2,159–1995 Ma (Chen et al., 2020a; Gao et al., 2017; Meng et al., 2017). The new U-Pb zircon ages of 2052 ± 15 Ma, 2089 ± 55 Ma, 2053 ± 28 Ma, and 2,190 ± 13 Ma from mafic rocks in this study refine the geochronological framework of Lieryu mafic magmatism to 2,190–1995 Ma. Therefore, the age of the Lieryu Formation ranges from 2,229 to 1959 Ma under the limitation of the geochronological results of felsic volcanic rocks and mafic rocks. The volcanic rocks from the Gaojiayu Formation exhibit an age range of 2,184–1928 Ma (Chen et al., 2017b; Bi et al., 2018a; Dong et al., 2019), whereas the Dashiqiao Formation volcanics were emplaced between 2,171 and 2054 Ma (Chen et al., 2017b; Xu et al., 2018a; b; Dong et al., 2019). From the above, the overlapping age among these formations (2078–1952 Ma for Langzishan, 2,229–1959 Ma for Lieryu, 2,184–1928 Ma for Gaojiayu, and 2054–2,171 Ma for Dashiqiao) did not show a continuous sedimentary strata but a disordered relationships, which reveals that the relationship between the various formation of the Liaohe Group is not a conformable stratigraphic succession. Combined with a set of boron-associated mélange assemblage (peridotite, gabbro, tourmaline granulite, and plagiogranite) distinguished from the Lieryu Formation, it also indicates that the Liaohe Group is not an ordered stratum.
5.2 Source and genesis of the mafic rocks
The mafic rocks of the Lieryu Formation in Liaodong have undergone low- to medium-grade metamorphism, such as epidotization, and chloritization. Under changing conditions, strong active elements tend to migrate, whereas REEs and HFSEs remain relatively stable. Consequently, these elements can be utilized to reconstruct and investigate the magmatic series, genesis, and source characteristics of these mafic rocks (Rollinson, 1993; Kerrich et al., 1999).
Typically, mafic rocks originate from either the lithospheric mantle or the asthenospheric mantle, with partial melting of lithospheric mantle rocks resulting in negative europium (Eu) anomalies (Sklyarov et al., 2003). These mafic rocks exhibit enrichment in LREEs and LILEs, along with depletion of HREEs and HFSEs such as Nb, Ta, Ti, Zr Ce and Th. They also show either positive europium anomaly (Eu/Eu* = 1.03–1.21) or a slight negative europium anomaly (Eu/Eu* = 0.92–0.97). In the pattern of the REEs and trace elements, the amphibolite (D1902) and two gabbro (D1911, D1914) are similar to E-MORB suggesting a source from the asthenospheric mantle, while the other amphibolite (D1905) resembles OIB (Figure 7a) indicating a complicated mantle source (Sklyarov et al., 2003). On the Nb/Th-Zr/Nb figure,the amphibolite (1905) and the gabbro (1911) are positioned near the primitive mantle (PM) (Fugure 7a), the amphibolite (1902) is close to the enrichment mantle (EM), the gabbro (1914) lies between the PM and EM (Fugure 7a). In the La/Yb-Th/Ta diagram, these mafic rocks distribute in the middle of PM and EM (Figure 7b). These features suggest that these mafic rocks are originated from the transitional mantle.
The nature of the original magma in the source can be studied by content and ratio of trace elements, due to their similar distribution coefficients and is difficult to fractionate during partial melting or fractional crystallization (McKenzie and O'Nions, 1991). Three mafic rocks (D1902, D1905, D1914) show similar patterns of flat REEs, with a lower LREE/HREE ratio (2.80–3.66, 3.58–3.73 and 2.86–2.96 respectively), indicating that there may be no residual garnet in the source (Figures 7c,d). They are located at the Amphibole-bearing spinel lherzolite melting curve on the La/Yb-Yb diagram (Figure 7c), indicating the presence of residual amphibole and spinel in the magma source. But there is difference on the melting degree, with 3%–4% melting degree of the amphibolite (D1905) and 10%–20% melting degree of the amphibolite (D1902) and gabbro (D1914) (Figure 7c). The other gabbro (D1911) shows a pattern of relatively enrichment in LREEs, flat HREEs, high ratios of LREE/HREE (3.94–4.19), which indicates that garnet may be the residual mineral in the source. On the La/Yb-Yb diagram, the gabbro is located at the Garnet spinel lherzolite melting curve with the garnet to spinel ratio being 50:50 (Figure 7c), indicating the presence of residual garnet and spinel in the magma source.
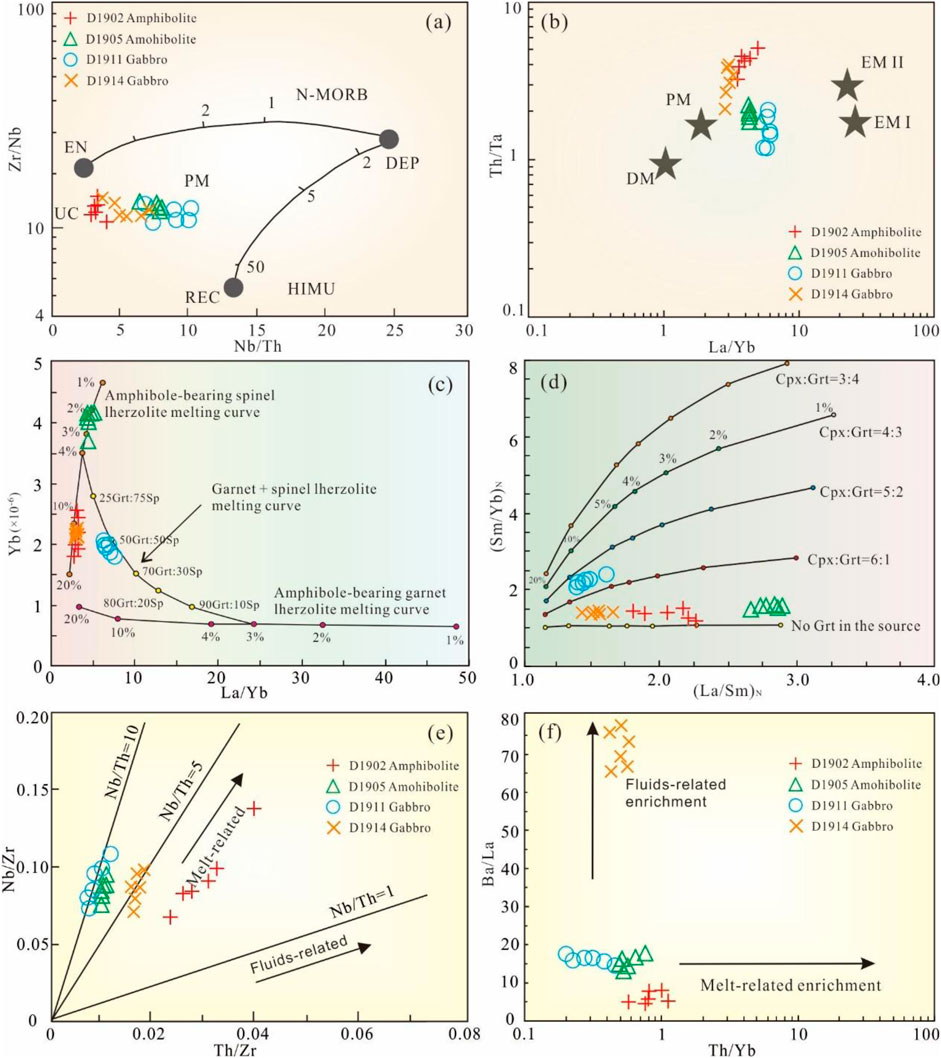
Figure 7. Source characteristics of the mafic rocks from the Lieryu Formation (a). After Condie et al., 2002, DEP-depleted mantle, EN-enriched mantle, N-MORB-normal mid-ocean ridge basalt, PM-primitive mantle, REC-recycled plate, UC-upper crust; (b) After Zhang et al., 2012; DM-depleted mantle, PM-primitive mantle, EM Ⅰ-enriched mantle Ⅰ, EM Ⅱ- enriched mantle Ⅱ; (c,d) After McKenzie and O'Nions, 1991, Grt- garnet, SP-spine, Cpx-clinopyroxene; (e,f) After Woodhead et al., 2001.
In contrast to the partial melting of spinel lherzolite, the partial melting of various proportions of clinopyroxene and garnet exhibits distinct characteristics in the La/Sm and Sm/Yb ratios. Notably, the Sm/Yb ratio remains unaffected by the decrease in La/Sm (Aldanmaz et al., 2000). These mafic rocks show the similar characteristics on the (La/Sm)N-(Sm/Yb)N diagram (Figure 7d). Consistent with the above conclusion, there is no garnet in the magma source of the three mafic rocks (D1902, D1905, D1914). They are the melting product of amphibole-bearing spinel lherzolite, showing different partial melting degrees of about 1%–2%, 3%–4%, and around 10%, respectively (Figure 7d). The other gabbro is product of partial melting of garnet–spinel lherzolite with a ratio of clinopyroxene to garnet of 6:1 in the 5%–10% melting degree (Figure 7d; Jourdan et al., 2007). The amphibolite (D1905) shows negative Sr anomaly (Figure 6b), indicating the possible residual plagioclase in the source. And the positive Sr anomaly of the gabbro (D1914) indicates no residual plagioclase in its magma source (Figure 6b).
These mafic rocks exhibit similar characteristics to of island arc basalt (IAB): enrichments in LREEs and LILEs such as Rb, Ba, and K, as well as depletions in HFSEs such as Nb, Ta, Zr, Hf, Ti, and P. It may be related to the subduction fluids or the involvement of island arc components, indicating that metasomatic processes occurred in the source (Sun and Nesbitt, 1978). Thus, is the metasomatism caused by melts or fluids? When the metasomatism occurred in the source is caused by melts, the magma generally exhibits high Na2O, P2O5, and TiO2 contents (Sajona et al.,2000), or obvious negative Ce anomaly (Plank and Langmuir, 1998). The D1902 (amphibolite), D1905 (amphibolite) and D1911 (gabbro) exhibit high Na2O content and negative Ce anomalies (average Ce/Ce* = 0.87, 0.91 and 0.87 respectively), indicating a melt related metasomatism in the source, which is consistent with the trends shown in the Th/Zr-Nb/Zr and Th/Yb-Ba/La diagram (Figures 7e, f). The D1914 (gabbro) exhibits a low Na2O, P2O5, and TiO2 contents, with no Ce anomaly (average Ce/Ce* = 1.00), indicating the source has undergone fluid metasomatism, which is consistent with the trends shown in the Th/Yb-Ba/La diagram (Figure 7f). But, the characteristics of Th/Zr and Nb/Zr ratio indicate that it also has undergone melt metasomatism (Figure 7e). The higher Cr, Co and Ni content than N-MORB in D1914 also suggests a melt related metasomatism in the source. So the D1914 (gabbro) has undergone dual metasomatism of fluid and melt, while the others mafic rocks are mainly influenced by melt metasomatism.
Generally, during the process of ascent and emplacement, mantle-derived magma may experience assimilation and contamination with crustal materials. Geochemical data for these mafic rocks indicate moderate to high levels of potassium and alkali content (Figure 5c), low Mg# values (Mg# = 45.93–57.61), enrichment in LILEs such as Rb, Ba, and K, and depletion in HFSEs like Th, Nb, Ta, and Zr. These characteristics suggest that the magma has likely been contaminated by crustal materials (Wu et al., 2007). These mafic rocks have a lower Nb/Ta ratios (averaging 11.04, 14.04, 14.00, and 16.03) and Zr/Hf ratios (averaging 26.19, 31.80, 28.19, and 28.64) than the continental crust in eastern China (Nb/Ta = 15.38, Zr/Hf = 35.56, according to Chi and Yan, 2007), as well as the mid-ocean ridge basalts (MORB) and the primitive mantle (Nb/Ta = 17.7, Zr/Hf = 36.1, Sun and McDonough, 1989). These differences suggest that crustal contamination has influenced the mafic magmas. The La/Sm ratio is frequently used to assess the degree of crustal contamination (Zhang et al., 2019). A higher La/Sm ratio generally signifies a stronger influence of crustal components, whereas a lower ratio may indicate a more pristine mantle source (Zhang et al., 2019). The average La/Sm ratios of these mafic rocks are 16.37, 15.92, 18.24, and 13.88, respectively, reflecting the assimilation and contamination of continental crustal composition during magma ascent. The contamination level is highest in D1911 (gabbro), followed by D1902 (amphibolite) and D1905 (amphibolite), with the lowest contamination found in D1914 (gabbro).
In conclusion, the Paleoproterozoic mafic rocks originated from a transitional mantle source, of which the D1902 (amphibolite), D1905 (amphibolite) and D1911 (gabbro) are mainly influenced by melt metasomatism, while the D1914 (gabbro) has undergone dual metasomatism of fluid and melt in the source. The D1902 (amphibolite), D1905 (amphibolite) and D1914 (gabbro) are product of 2%–3% or 10%–20% partial melting of amphibole-bearing spinel lherzolite, leading to residual hornblende and spinel in the source. The D1911 (gabbro) is product of 3%–4% partial melting of garnet–spinel lherzolite with the 50:50 ratios of garnet and spinel and 6:1 ratio of clinopyroxene to garnet, resulting in garnet and spinel in the source. During the ascent and emplacement, assimilation and contamination occurred between mafic magmas and continental crustal materials.
5.3 Tectonic significance of mafic rocks
TiO2 contents vary in mafic rocks formed in different tectonic environments (Wilson, 1989). Ocean island basalt (OIB) typically has the highest TiO2 content, reaching 2.63 wt%. Island arc basalt (IAB), on the other hand, has the lowest TiO2 content, approximately 0.98 wt%, whereas MORB contains about 1.5 wt% TiO2 (Wilson, 1989). Within-plate basalts (WPB) display higher TiO2 contents, ranging from 2.23 wt% to 2.9 wt% (Wilson, 1989). Regarding trace element abundances, IAB has significantly lower Nb and Ta contents, ranging from 1.7 to 2.7 μg/g and 0.1–0.18 μg/g, respectively. In comparison, WPB generally have elevated Nb and Ta concentrations, ranging from 13 to 84 μg/g and 0.73–5.9 μg/g, respectively (Peccerillo and Taylor, 1976). Characteristics of element ratios in basaltic rocks from various tectonic settings reveal that WPB and MORB are enriched in TiO2 and HFSEs, and show special element ratios as following: Nb/La >0.8, Ti/Y > 350, Ti/V > 30, Hf/Ta <5, La/Ta <15, Th/Ta <3, and Ta/Yb < 0.1 (Peccerillo and Taylor, 1976; Condie, 1989). In contrast, IAB from active continental margins shows similar trends.
D1902 (amphibolite) and D1914 (gabbro) show a similar low TiO2 content (average of 1.03 wt% and 1.13 wt%), which likes the continental arc basalts (CAB). But, the content of Nb and Ta is a little higher than CAB. The average trace element ratios of Nb/La (average of 0.52 and 0.87), Hf/Ta (average of 4.93 and 6.48), La/Ta (average of 21.46 and 18.35), Ti/Y (average of 328 and 344), Ti/V (average of 23.7 and 23.0), Th/Ta (average of 4.23 and 3.18), Zr/Y (average of 2.82 and 3.54), Ta/Yb = (average of 0.19 and 0.17) indicate that it is different from WPB and MORB but similar with CAB (Peccerillo and Taylor, 1976; Condie, 1989). These two mafic rocks also exhibit characteristics of island arc volcanic rocks in the La/Nb- Nb/Th diagram (Figure 8a). Furthermore, in the Nb/Yb-Th/Yb diagram, Hf/3-Th-Nb/16 and Hf/3-Th-Ta triangular diagram, these two mafic rocks fall within the continental arc and CAB area (Figures 8b–d). These characteristics suggest that D1902 (amphibolite) and D1914 (gabbro) were formed in an island arc environment.
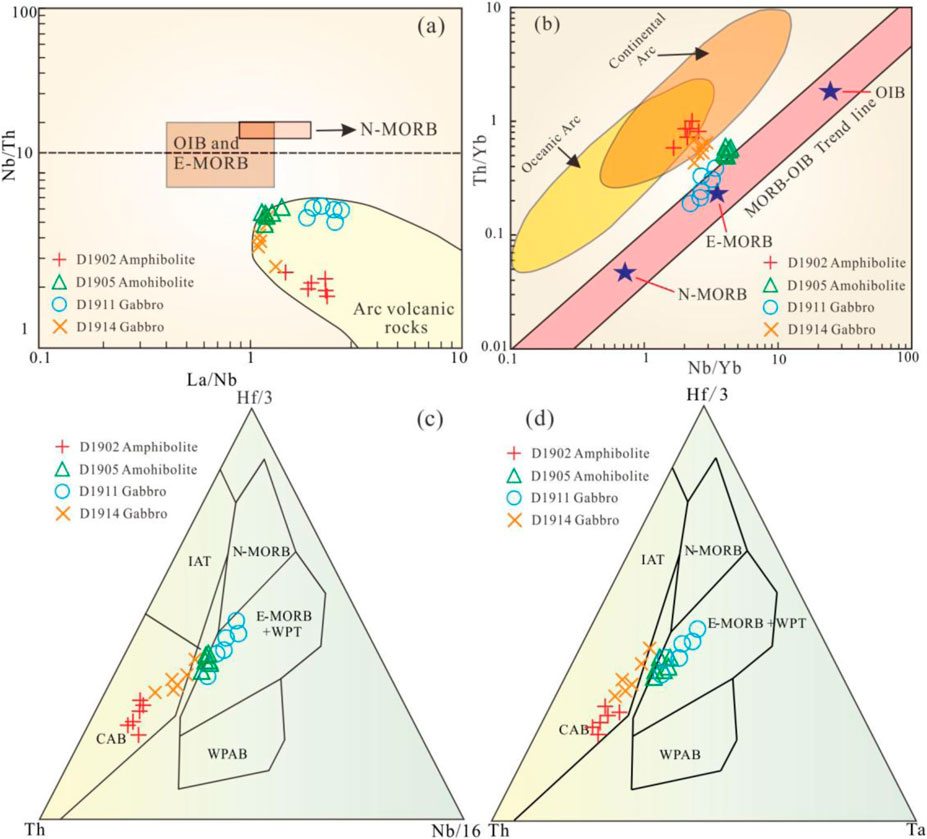
Figure 8. Identification diagram of tectonic setting for the mafic rocks from the Lieryu Formation. (a) La/Nb versus Nb/Th diagram (Zhang et al., 2012); (b) Nb/Yb versus Th/Yb diagram (after Pearce, 1996); (c) Hf/3 versus Th versus Nb/16; (d) Hf/3 versus Th versus Th/Yb diagram (after Pearce, 1982).
The content of Nb (average = 15.8 μg/g) Ta (average = 1.13 μg/g) TiO2 (average = 2.58 wt%) in D1905 (amphibolite) indicate a WPB setting. This may be attributed to the melt contamination, resulting in high contents. The ratios of Zr/Y (average = 4.75) and Ta/Yb (averages = 0.28) suggest a CAB setting, which is consistent with the La/Nb-Nb/Th diagram (Figure 8a). But the other average ratios of trace element Nb/La (average = 0.86), Hf/Ta (average = 4.84), La/Ta (average = 14.67), Ti/Y (average = 390), Ti/V (average = 40.3) and Th/Ta (average = 1.89) show the same characteristics with MORB. In the Hf/3-Th-Nb/16 and Hf/3-Th-Ta triangular diagram, this falls within the E-MORB area (Figures 8c,d). On the Nb/Yb-Th/Yb diagram, this sample distribute near the E-MORB (Figure 8b). These characteristics suggest D1905 (amphibolite) was formed in a mid-ocean ridge environment.
In D1911 (gabbro), the low content of TiO2 (average = 1.02 wt%), Nb (average = 5.17 μg/g), Ta (average = 0.37 μg/g) and the average trace element ratios of Nb/La (average = 0.45), Hf/Ta (average = 5.44), La/Ta (average = 31.26) suggest an island arc setting (Figure 8a). But, the other average trace element ratios of Ti/Y = 359, Ti/V = 30.9, Th/Ta = 1.56, Zr/Y = 2.84 and Ta/Yb = 0.19 suggest a MORB feature, which is consistent with the results in tectonic setting patterns (Figures 8b–d). So, D1911 (gabbro) was formed in a mid-ocean ridge environment.
The tectonic nature of the Jiao-Liao-Ji Belt (JLJB) has been a subject of ongoing debate. A group of researchers advocates a continental rift model based on the presence of bimodal volcanic rocks within the Liaohe Group and geochemical signatures of Paleoproterozoic granitoids. These studies propose that the JLJB originated through continental rifting, subsequent sedimentary deposition, and eventual tectonic reworking via subduction and collision (Zhang, 1988; Chen et al., 2003; Hao et al., 2004; Luo et al., 2004; Li et al., 2005; 2006; Lu et al., 2006; Zhang et al., 2010; Jiang, 2014; Zhai and Santosh, 2011; Zhao et al., 2005; 2011; 2012; Zhao and Zhai, 2013; Hu et al., 2014). In contrast, other researchers distinguish the northern and southern Liaohe Group as two distinct continental margins based on contrasting P-T-t paths and metamorphic grades. These studies interpret the JLJB as an amalgamation zone formed by collision between two independent terranes (Lu, 1996; He and Ye, 1998; Li et al., 2001; Li and Chen, 2014; Lu et al., 2006; Meng et al., 2014). Alternatively, some researchers propose a third arc-continent collisional model for the JLJB, supported by integrated petrological, structural, and paleogeothermal evidence. The formation of the JLJB is related to northward subduction of the Nangrim Block in the south (Bai, 1993; Zhao et al., 2005; 2012; Zhai and Santosh, 2011; 2013; Zhao and Zhai, 2013; Li et al., 2012; Li and Chen, 2014; Liu et al., 2015; Li et al., 2015a; b, 2019; Xu et al., 2018a; 2018b; Xu and Liu, 2019; Xu and Liu, 2019; Chen et al., 2017a; 2018; 2020a; 2020b; 2021; 2024). Our new geochemical data from the Lieryu mafic rocks reveal distinct arc and mid-ocean ridge settings, providing critical evidence for the existence of a Paleoproterozoic oceanic basin that underwent subduction-accretion processes. These findings collectively support the interpretation that the JLJB represents a collisional orogen formed during the closure of a Paleoproterozoic oceanic basin.
6 Conclusion
(1) Zircon U-Pb ages (2052 ± 15 Ma, 2089 ± 55 Ma, 2053 ± 28 Ma, 2,190 ± 13 Ma) have been obtained from mafic rocks in Lieryu Formation, which constrained the mafic magmatism to 2,190–1995 Ma. Combined with the geochronological results of felsic volcanic rocks, the formation age of the Lieryu Formation is limited to 2,229–1959 Ma. The overlapping age among Langzishan (2078–1952 Ma), Lieryu (2,229–1959 Ma), Gaojiayu (2,184–1928 Ma) and Dashiqiao (2054–2,171 Ma) formations reveals a disordered stratum.
(2) Geochemical characteristics reveal that the mafic rocks belong to the calc-alkaline series and originated from transitional mantle. The D1902 (amphibolite), D1905 (amphibolite) and D1911 (gabbro) are mainly influenced by melt metasomatism, while the D1914 (gabbro) has undergone dual metasomatism of fluid and melt in the source. The D1902 (amphibolite), D1905 (amphibolite) and D1914 (gabbro) are product of partial melting of amphibole-bearing spinel lherzolite, and the D1911 (gabbro) is product of partial melting of garnet–spinel lherzolite. During the ascent and emplacement, assimilation and contamination occurred between mafic magmas and continental crustal materials.
(3) Characteristics of major and trace elements suggest that D1902 (amphibolite) and D1914 (gabbro) were formed in an island arc environment, and the D1905 (amphibolite) and D1911 (gabbro) were formed in a mid-ocean ridge environment. This indicates the existence of ancient oceans in the Paleoproterozoic and the JLJB was formed during the subduction and extinction process of the Paleoproterozoic ocean.
Data availability statement
The original contributions presented in the study are included in the article/Supplementary Material, further inquiries can be directed to the corresponding author.
Author contributions
JC: Writing – original draft, Formal Analysis, Project administration, Visualization, Data curation, Methodology, Validation, Resources, Investigation, Supervision, Software, Funding acquisition, Conceptualization, Writing – review and editing. LL: Writing – review and editing, Validation, Resources, Investigation, Methodology. BL: Methodology, Validation, Writing – review and editing, Investigation. YT: Methodology, Validation, Investigation, Writing – review and editing. CZ: Methodology, Writing – review and editing, Investigation, Validation. YaZ: Writing – review and editing, Investigation, Validation, Methodology. YZ: Methodology, Writing – review and editing, Investigation, Validation.
Funding
The author(s) declare that financial support was received for the research and/or publication of this article. This research was financially supported by the China Geological Survey (Grants DD20242927 and DD20190042) and the National Natural Science Foundation of China (Grant Nos 42302221 and U2244213).
Conflict of interest
Author YT was employed by Liaoning Geological and Mineral Survey Institute Co., Ltd.
The remaining authors declare that the research was conducted in the absence of any commercial or financial relationships that could be construed as a potential conflict of interest.
Generative AI statement
The author(s) declare that no Generative AI was used in the creation of this manuscript.
Publisher’s note
All claims expressed in this article are solely those of the authors and do not necessarily represent those of their affiliated organizations, or those of the publisher, the editors and the reviewers. Any product that may be evaluated in this article, or claim that may be made by its manufacturer, is not guaranteed or endorsed by the publisher.
Supplementary material
The Supplementary Material for this article can be found online at: https://www.frontiersin.org/articles/10.3389/feart.2025.1592749/full#supplementary-material
References
Aldanmaz, E., Pearce, J. A., Thirlwall, M. F., and Mitchell, J. G. (2000). Petrogenetic evolution of late Cenozoic, post-collision volcanism in western Anatolia, Turkey. J. Volcanol. Geotherm. Res. 102, 67–95. doi:10.1016/s0377-0273(00)00182-7
Andersen, T. (2002). Correction of common lead in U-Pb analyses that do not report 204Pb. Chem. Geol. 192, 59–79. doi:10.1016/s0009-2541(02)00195-x
Bai, J. (1993). The precambrian Geology and Pb-Zn mineralization in the northern margin of north China platform. Beijing: Geological Publishing House, 1–136.
Belousova, E., Griffin, W., O’Reilly, S. Y., and Fisher, N. (2002). Igneous zircon: trace element composition as an indicator of source rock type. Contributions Mineralogy Petrology 143, 602–622. doi:10.1007/s00410-002-0364-7
Bi, J. H., Ge, W. C., Xing, D. H., Yang, H., Dong, Y., Tian, D. X., et al. (2018b). Palaeoproterozoic meta-rhyolite and meta-dacite of the Liaohe group, jiao-liao-ji belt, north China craton: petrogenesis and implications for tectonic setting. Precambrian Res. 314, 306–324. doi:10.1016/j.precamres.2018.06.007
Bi, J. H., Xing, D. H., Ge, W. C., Yang, H., and Wang, Z. H. (2018a). Age and tectonic setting of meta-acid volcanic rocks from the North Liaohe Group in the Liaodong area: Paleoproterozoic intracontinental rift or active continental margin? Earth Sci. Front. 25 (3), 295–308.
Bureau of Geology and Mineral Resources of Liaoning Province (2014). Regional Geology of liaoning Province. Beijing: Geological Publishing House, 595–763. (in Chinese).
Chen, B., Li, Z., Wang, J. L., Yang, F., Liu, C., and Zhao, Y. (2016). Liaodong Peninsula ∼2.2 Ga magmatic event and its geological significance. J. Jilin Univ. Earth Sci. Ed. 46 (2), 303–320.
Chen, J., Tian, Y., Gao, Z., Li, B., Zhao, C., Li, W., et al. (2024). Geochronology and geochemistry of Paleoproterozoic mafic rocks in northern Liaoning and their geological significance. Minerals 14, 717. doi:10.3390/min14070717
Chen, J. S., An, M. G., Duan, M., Li, W., and Liu, Y. (2017b). The age of the Dashiqiao Formation in the Liaohe Group: constraints from LA-ICP-MS zircon U-Pb dating of acidic volcanic rocks. Geol. Resour. 26 (2), 105–110.
Chen, J. S., Jiang, Z. Q., Li, W. W., Li, B., Liu, M., Yang, F., et al. (2018). The formation ages of the Langzishan and Lieryu formations in Lianshanguan area, Benxi, Liaoning province, and its geological significance. Geol. Bull. China 37, 1693–1703.
Chen, J. S., Li, W. W., Xing, D. H., Yang, Z. Z., Tian, D. X., Zhang, L. D., et al. (2020b). Zircon U-Pb geochronology of volcanic rocks from Gaojiayu Formation, Liaohe group, liaoning province and its geological significance. Earth Sci. 45, 3934–3949.
Chen, J. S., Tian, D. X., Xing, D. H., Li, B., Liu, M., Yang, F., et al. (2020a). Zircon U-Pb geochronology and its geological significance of the basic volcanic rocks from the Li’eryu Formation, Liaohe Group in Kuandian area. Earth Sci. 45, 3282–3294.
Chen, J. S., Xing, D. H., Liu, M., Li, B., Yang, H., Tian, D. X., et al. (2017a). Zircon U–Pb chronology and geological significance of felsic volcanic rocks in the Liaohe Group from the Liaoyang area, Liaoning Province. Acta Petrol. Sin. 33, 2792–2810.
Chen, J. S., Yang, Z. Z., Tian, D. X., Xing, D. H., Zhang, L. D., Yang, F., et al. (2021). Geochronological framework of Paleoproterozoic intrusive rocks and its constraints on tectonic evolution of the Liao-Ji Belt, Sino-Korean Craton. J. Earth Sci. 32, 8–24. doi:10.1007/s12583-020-1367-x
Chen, R. D., Li, X. D., and Zhang, F. S. (2003). Several problems about the Paleoproterozoic geology of eastern Liaodong. Geol. China 30 (2), 207–213.
Chi, Q. H., and Yan, M. C. (2007). Handbook of elemental abundance for applied geochemistry. Beijing: Geological Publishing House, 1–148. (in Chinese).
Condie, K. C. (1989). Geochemical changes in baslts and andesites across the Archean-Proterozoic boundary: identification and significance. Lithos 23, 1–18. doi:10.1016/0024-4937(89)90020-0
Condie, K. C., Frey, B. A., and Kerrich, R. (2002). The 1.75 Ga Iron King Volcanics in West Central Arizona: a remnant of an accreted oceanic plateau derived from a mantle plume with a deep depleted component. Lithos 64, 49–62. doi:10.1016/s0024-4937(02)00158-5
De la Roche, H., Leterrier, J., Grandclaude, P., and Marchal, M. (1980). A classification of volcanic and plutonic rocks using R1R2-diagram and major-element analyses—its relationships with current nomenclature. Chem. Geol. 29, 183–210. doi:10.1016/0009-2541(80)90020-0
Dong, Y., Bi, J. H., Xing, D. H., Liu, F. L., Tian, Z. H., Cai, J., et al. (2019). Geochronology and geochemistry of Liaohe group and liaoji granitoid in the jiao-liao-ji belt, north China craton: implications for petrogenesis and tectonic evolution. Precambrian Res. 332, 105399. doi:10.1016/j.precamres.2019.105399
Dupuy, C., and Dostal, J. (1984). Trace element geochemistry of some continental tholeiites. Earth Planet. Sci. Lett. 67, 61–69. doi:10.1016/0012-821x(84)90038-4
Gao, B. S., Dong, Y. S., Li, F. Q., Wang, P. S., Gan, Y. C., Chen, M. S., et al. (2017). Petrogenesis of the Lieryu Formation of the South Liaohe Group in the Huanghuadian area, Liaodong Peninsula. Acta Petrologica Sinica 33 (9), 2725–2742 (in Chinese).
Hao, D. F., Li, S. Z., Zhao, G. C., Sun, M., Han, Z. Z., and Zhao, G. T. (2004). Origin and its constraint to tectonic evolution of Paleoproterozoic granitoids in the eastern Liaoning and Jilin province, North China. Acta Petrol. Sin. 20 (6), 1409–1416.
He, G. P., and Ye, H. W. (1998). Two types of early Proterozoic metamorphism in the eastern Liaoning to southern Jilin and their tectonic implication. Acta Petrol. Sin. 14, 152–162.
Hoskin, P. W. O., and Ireland, T. R. (2000). Rare earth element chemistry of zircon and its use as a provenance indicator. Geology 28, 627–630. doi:10.1130/0091-7613(2000)028<0627:reecoz>2.3.co;2
Hu, G. Y., Li, Y. H., Fan, R. L., Wang, T. H., Fan, C. F., and Wang, Y. B. (2014). The forming age of the borate deposits in Kuandian area, eastern Liaoning Province: constraints from the SHRIMP U-Pb data and boron isotopic composition. Acta Geol. Sin. 88 (10), 1932–1943.
Jiang, C. C. (2014). Continental margin fold belt of Paleoproterozoic in Eastern Liaoning-Jilin—the formation and evolution of Kuandian-Caohe fold belt. Geol. Rev. 60 (3), 576–579. (in Chinese).
Jourdan, F., Bertrand, H., Schaerer, U., Blichert-Toft, J., Feraud, G., and Kampunzu, A. B. (2007). Major and trace element and Sr, Nd, Hf, and Pb isotope compositions of the Karoo large igneous province, Botswana-Zimbabwe: lithosphere vs mantle plume contribution. J. Petrology 48, 1043–1077. doi:10.1093/petrology/egm010
Kerrich, R., Polat, A., Wyman, D., and Hollings, P. (1999). Trace element systematics of Mg- to Fe-tholeiitic basalt suites of the Superior Province: implications for Archean mantle reservoirs and greenstone belt genesis. Lithos 46, 163–187. doi:10.1016/s0024-4937(98)00059-0
Li, S. Z., Han, Z. Z., Liu, Y. J., Zhao, G. C., Sun, M., and Wang, P. C. (2001). Regional metamorphism of the Liaohe group: implications for continental dynamics. Geol. Rev. 47, 9–18.
Li, S. Z., and Zhao, G. C. (2007). SHRIMP U-Pb zircon geochronology of the liaoji granitoids: constraints on the evolution of the paleoproterozoic jiao-liao-ji belt in the eastern block of the North China craton. Precambrian Res. 158, 1–16. doi:10.1016/j.precamres.2007.04.001
Li, S. Z., Zhao, G. C., Santosh, M., Liu, X., Dai, L. M., Suo, Y. H., et al. (2012). Paleoproterozoic structural evolution of the southern segment of the jiao-liao-ji belt, north China craton. Precambrian Res. 200–203, 59–73. doi:10.1016/j.precamres.2012.01.007
Li, S. Z., Zhao, G. C., Sun, M., Han, Z. Z., Liu, Y. J., Wang, Y. B., et al. (2005). Deformation history of the paleoproterozoic Liaohe assemblage in the eastern block of the North China craton. J. Asian Earth Sci. 24, 659–674. doi:10.1016/j.jseaes.2003.11.008
Li, S. Z., Zhao, G. C., Sun, M., Liu, Y. J., Han, Z. Z., and Wilde, S. A. (2006). Are the South and North Liaohe groups of north China craton different exotic terranes? Nd isotope constraints. Gondwana Res. 9, 198–208. doi:10.1016/j.gr.2005.06.011
Li, Z., and Chen, B. (2014). Geochronology and geochemistry of the paleoproterozoic metabasalts from the jiao-liao-ji belt, north China craton: implications for petrogenesis and tectonic setting. Precambrian Res. 255, 653–667. doi:10.1016/j.precamres.2014.07.003
Li, Z., Chen, B., Liu, J. W., Wei, C. J., Yan, X., and Gong, M. Y. (2015a). Zircon U-Pb ages and their implications for the South Liaohe group in the Liaodong peninsula, northeast China. Acta Petrol. Sin. 31 (6), 1589–1605.
Li, Z., Chen, B., Wei, C. J., Yan, X., Fu, B., and Gong, M. Y. (2015b). Provenance and tectonic setting of the Paleoproterozoic metasedimentary rocks from the Liaohe Group, Jiao-Liao-Ji Belt, North China Craton: insights from detrital zircon U-Pb geochronology, whole-rock Sm-Nd isotopes, and geochemistry. J. Asian Earth Sci. 111, 711–732. doi:10.1016/j.jseaes.2015.06.003
Li, Z., Chen, B., and Yan, X.(2018). The Liaohe Group: an insight into the Paleoproterozoic tectonic evolution of the Jiao–Liao–Ji Belt, North China Craton. Precambrian Resserach. doi:10.1016/j.precamres.2018.01.009
Li, Z., Chen, B., and Yan, X. (2019a). The Liaohe group: an insight into the paleoproterozoic tectonic evolution of the jiao-liao-ji belt, north China craton. Precambrian Res. 326, 174–195. doi:10.1016/j.precamres.2018.01.009
Li, Z., Meng, E., Wang, C. Y., and Li, Y. G. (2019b). Early precambrian tectono-thermal events in southern Jilin province, China: implications for the evolution of neoarchean to paleoproterozoic crust in the northeastern north China craton. Mineralogy Petrology 113, 185–205. doi:10.1007/s00710-018-0649-9
Li, Z., and Wei, C. J. (2017). Two types of Neoarchean basalts from Qingyuan greenstone belt, North China Craton: petrogenesis and tectonic implications. Precambrian Res. 292, 175–193. doi:10.1016/j.precamres.2017.01.014
Li, Z., Wei, C. J., Chen, B., Fu, B., and Gong, M. Y. (2020). Late neoarchean reworking of the mesoarchean crustal remnant in northern liaoning, north China craton: a U-Pb-Hf-O-Nd perspective. Gondwana Res. 80, 350–369. doi:10.1016/j.gr.2019.10.020
Liu, F. L., Liu, P. H., Wang, F., Liu, C. H., and Cai, J. (2015). Progresses and overviews of voluminous meta-sedimentary series within the Paleoproterozoic Jiao-Liao-Ji orogenic/mobile belt, North China Craton. Acta Petrol. Sin. 31, 2816–2846.
Liu, J., Zhang, J., Liu, Z. H., Yang, F., Wang, Y., Tan, H. Y., et al. (2018). Geochemical and geochronological study on the Paleoproterozoic rock assemblage of the Xiuyan region: new constraints on an integrated rift-and-collision tectonic process involving the evolution of the Jiao-Liao-Ji Belt, North China Craton. Precambrian Res. 310, 179–197. doi:10.1016/j.precamres.2018.03.005
Liu, P. H., Cai, J., and Zou, L. (2017). Metamorphic P-T-t path and its geological implication of the Sanjiazi garnet amphibolites from the northern Liaodong Peninsula, Jiao-Liao-Ji Belt: constraints on phase equilibria and zircon U-Pb dating. Acta Petrol. Sin. 33, 2649–2674.
Liu, P. H., Liu, F. L., Tian, Z. H., Yang, H., Cai, J., and Shi, Y. (2019). Petrological and geochronological evidence for paleoproterozoic granulite-facies metamorphism of the South Liaohe group in the jiao-liao-ji belt, north China craton. Precambrian Res. 332, 105406. doi:10.1016/j.precamres.2019.105406
Lu, L. Z. (1996). The Precambrian metamorphic geology and tectonic evolution of the Jiao-Liao massif. J. Changchun Univ. Earth Sci. 26, 25–32.
Lu, X. P., Wu, F. Y., Guo, J. H., Zhao, G. C., Sun, M., Wilde, S. A., et al. (2006). Zircon U-Pb geochronological constraints on the paleoproterozoic crustal evolution of the eastern block in the North China craton. Precambrian Res. 146, 138–164. doi:10.1016/j.precamres.2006.01.009
Ludwig, K. R. (2003). User’s manual for Isoplot 3.0: a geochronological toolkit for microsoft excel, 75. Berkeley: Berkeley Geochronology Center Special Publication.
Luo, Y., Sun, M., Zhao, G. C., Wilde, S. A., Li, S. Z., Han, Z. Z., et al. (2004). LA-ICP-MS U-Pb zircon ages of the Liaohe group in the eastern block of the North China craton: constraints on the evolution of the jiao-liao-ji belt. Precambrian Res. 134, 349–371. doi:10.1016/j.precamres.2004.07.002
Luo, Y., Sun, M., Zhao, G. C., Wilde, S. A., Li, S. Z., Han, Z. Z., et al. (2008). A comparison of U-Pb and Hf isotopic compositions of detrital zircons from the North and South Liaohe groups: constraints on the evolution of the jiao-liao-ji belt, north China craton. Precambrian Res. 163, 279–306. doi:10.1016/j.precamres.2008.01.002
McKenzie, D., and O’Nions, R. K. (1991). Partial melt distributions from inversion of rare earth element concentrations. J. Petrol. 32, 1021–1091. doi:10.1093/petrology/32.5.1021
Meng, E., Liu, F. L., Liu, P. H., Liu, C. H., Yang, H., Wang, F., et al. (2014). Petrogenesis and tectonic significance of Paleoproterozoic meta-mafic rocks from central Liaodong Peninsula, northeast China: evidence from zircon U-Pb dating and in situ Lu-Hf isotopes, and whole-rock geochemistry. Precambrian Res. 247, 92–109. doi:10.1016/j.precamres.2014.03.017
Meng, E., Wang, C. Y., Li, Y. G., Li, Z., Yang, H., Cai, J., et al. (2017). Zircon U-Pb-Hf isotopic and whole-rock geochemical studies of Paleoproterozoic metasedimentary rocks in the northern segment of the Jiao-Liao-Ji Belt, China: implications for provenance and regional tectonic evolution. Precambrian Res. 298, 472–489. doi:10.1016/j.precamres.2017.07.004
Middlemost, E. A. K. (1994). Naming materials in the magma/igneous rock system. Earth-Sci. Rev. 37, 215–224. doi:10.1016/0012-8252(94)90029-9
Pearce, J. A. (1982). “Trace elements characteristic of lavas from destructive plate boundaries,” in Andesites. Orogenic andesites and related rocks. Editor R. S. Thorpe (Chichester, UK: John Wiley & Sons), 525–548.
Pearce, J. A. (1996). “A user’s guide to basalt discrimination diagrams,” in Trace element geochemistry of volcanic rocks: applications for massive sulphide exploration. Short course notes. Editor D. A. Wyman (Ottawa, Canada: Geological Association of Canada: St. John’s, NL, Canada), 12, 79–113.
Peccerillo, A., and Taylor, S. R. (1976). Geochemistry of Eocene calc-alkaline volcanic rocks from the Kastamonu area, northern Turkey. Contrib. Mineral. Petrol. 58, 63–81. doi:10.1007/bf00384745
Peng, P., Wang, C., Wang, X. P., and Yang, S. Y. (2015). Qingyuan high-grade granite-greenstone terrain in the eastern north China craton: root of a neoarchaean arc. Tectonophysics 662, 7–21. doi:10.1016/j.tecto.2015.04.013
Plank, T., and Langmuir, C. H. (1998). The chemical composition of subducting sediment and its consequences for the crust and mantle. Chem. Geol. 145, 325–394. doi:10.1016/s0009-2541(97)00150-2
Sajona, F. G., Maury, R. C., Pubellier, M., Leterrier, J., Bellon, H., and Cotton, J. (2000). Magmatic source enrichment by slab-derived melts in a young post-collision setting, Central Mindanao (Philippines). Lithos 54, 173–206. doi:10.1016/s0024-4937(00)00019-0
Sklyarov, E. V., Gladkochub, D. P., Mazukabzov, A. M., Menshagin, Y. V., Watanabe, T., and Pisarevsky, S. A. (2003). Neoproterozoic mafic dike swarms of the Sharyzhalgai metamorphic massif, southern Siberian Craton. Precambrian Res. 122, 359–376. doi:10.1016/s0301-9268(02)00219-x
Sun, S. S., and McDonough, W. F. (1989). Chemical and isotopic systematics of oceanic basalts: implications for mantle composition and processes. Geol. Soc. Lond. Spec. Publ. 42, 313–345. doi:10.1144/gsl.sp.1989.042.01.19
Sun, S. S., and Nesbitt, R. W. (1978). Geochemical regularities and genetic significance of ophiolitic basalts. Geology 6, 689–693. doi:10.1130/0091-7613(1978)6<689:gragso>2.0.co;2
Tian, Z. H., Liu, F. L., Windley, B. F., Cai, J., Wang, W., Zhang, M., et al. (2017). Polyphase structural deformation of low-to medium-grade metamorphic rocks of the Liaohe group in the jiao-liao-ji orogenic belt, north China craton: correlations with tectonic evolution. Precambrian Res. 303, 641–659. doi:10.1016/j.precamres.2017.08.017
Wan, Y. S., Song, B., Yang, C., and Liu, D. Y. (2005). Zircon SHRIMP U-Pb geochronology of Archaean rocks from the Fushun-Qingyuan area, Liaoning province and its geological significance. Acta Geol. Sin. 79, 78–87.
Wiedenbeck, M., Allé, P., Corfu, F., Griffin, W. L., Meier, M., Oberli, F., et al. (1995). Three natural zircon standards for U-Th-Pb, Lu-Hf, trace element and REE analyses. Geostand. Newsl. 19, 1–23.
Wilson, M. (1989). Igneous petrogenesis: a global tectonic approach. London, UK: Unwin Hyman, 1–466.
Woodhead, J. D., Hergt, J. M., Davidson, J. P., and Eggins, S. M. (2001). Hafnium isotope evidence for conservative element mobility during subduction zone processes. Earth Planet. Sci. Lett. 192, 331–346. doi:10.1016/s0012-821x(01)00453-8
Wu, F. Y., Li, X. H., Yang, J. H., and Zheng, Y. F. (2007). Discussions on the petrogenesis of granites. Acta Petrol. Sin. 23, 1217–1238.
Xu, W., and Liu, F. L. (2019). Geochronological and geochemical insights into the tectonic evolution of the paleoproterozoic jiao-liao-ji belt, sino-Korean craton. Earth-Science Rev. 193, 162–198. doi:10.1016/j.earscirev.2019.04.019
Xu, W., Liu, F. L., and Liu, C. H. (2017). Petrogenesis and geochemical characteristics of the North Liaohe metabasic rocks, Jiao-Liao-Ji orogenic belt and their tectonic significance. Acta Petrol. Sin. 33 (9), 2743–2757.
Xu, W., Liu, F. L., Santosh, M., Liu, P. H., Tian, Z. H., and Dong, Y. S. (2018a). Constraints of mafic rocks on a paleoproterozoic back-arc in the jiao-liao-ji belt, north China craton. J. Asian Earth Sci. 166, 195–209. doi:10.1016/j.jseaes.2018.06.016
Xu, W., Liu, F. L., Tian, Z. H., Liu, L. S., Ji, L., and Dong, Y. S. (2018b). Source and petrogenesis of paleoproterozoic meta-mafic rocks intruding into the North Liaohe group: implications for back-arc extension prior to the formation of the jiao-liao-ji belt, north China craton. Precambrian Res. 307, 66–81. doi:10.1016/j.precamres.2018.01.011
Xu, W., Liu, F. L., Wang, F., Tian, Z. H., Liu, P. H., and Dong, Y. S. (2019). Paleoproterozoic tectonic evolution of the jiao-liao-ji belt, north China craton: geochemical and isotopic evidence from ca. 2.17 Ga felsic tuff. Geol. J. 54, 1–20. doi:10.1002/gj.3380
Zhai, M. G., and Santosh, M. (2011). The early Precambrian odyssey of the North China Craton: a synoptic overview. Gondwana Res. 20 (1), 6–25. doi:10.1016/j.gr.2011.02.005
Zhai, M. G., and Santosh, M. (2013). Metallogeny of the North China craton: link with secular changes in the evolving earth. Gondwana Res. 24 (1), 275–297. doi:10.1016/j.gr.2013.02.007
Zhang, Q. S. (1988). Early Proterozoic tectonic styles and associated mineral deposits of the North China platform. Precambrian Res. 39 (1-2), 1–29. doi:10.1016/0301-9268(88)90047-2
Zhang, Q. S., and Yang, Z. S. (1988). Early crust and mineral deposits of Liaodong peninsula. Beijing: China. Geological Publishing House, 1–575.
Zhang, W., Liu, F. L., Cai, J., Tian, Z. H., Liu, L. S., Ji, L., et al. (2018). Geochemistry, zircon U-Pb dating and tectonic implications of the palaeoproterozoic ji’an and laoling groups, northeastern jiao-liao-ji belt, north China craton. Precambrian Res. 314, 264–287. doi:10.1016/j.precamres.2018.06.010
Zhang, X. H., Yuan, L. L., Xue, F. H., and Zhang, Y. B. (2012). Contrasting Triassic ferroan granitoids from northwestern Liaoning, North China: magmatic monitor of Mesozoic decratonization and a craton–orogen boundary. Lithos 144-145, 12–23. doi:10.1016/j.lithos.2012.03.022
Zhang, Y. F., Liu, J. D., Xiao, R. G., Wang, S. Z., Wang, J., and Bao, D. J. (2010). The Hyalotourmalites of Houxianyu borate deposit in eastern Liaoning: zircon features and SHRIMP dating. Earth Science—Journal China Univ. Geosciences 35 (6), 985–999.
Zhang, Y. M., Pei, X. Z., Li, Z. C., Li, R. B., Liu, C. J., Pei, L., et al. (2019). Zircon U-Pb geochronology, geochemistry and its geological implication of the early Indosinian basic complex in the Qinghai Nanshan tectonic belt. Earth Sci. 44 (8), 2461–2477.
Zhao, G. C., Cao, L., Wilde, S., Sun, M., Choe, W., and Li, S. Z. (2006). Implications based on the first SHRIMP U–Pb zircon dating on Precambrian granitoid rocks in North Korea. Earth Planet. Sci. Lett. 251 (3-4), 365–379. doi:10.1016/j.epsl.2006.09.021
Zhao, G. C., Cawood, P. A., Li, S. Z., Wilde, S. A., Sun, M., Zhang, J., et al. (2012). Amalgamation of the North China craton: key issues and discussion. Precambrian Res. 222-223, 55–76. doi:10.1016/j.precamres.2012.09.016
Zhao, G. C., Li, S. Z., Sun, M., Wilde, S. A., Liu, J., and Wu, C. M. (2011). Assembly, accretion, and break-up of the Palaeo-Mesoproterozoic Columbia supercontinent: record in the North China Craton revisited. Int. Geol. Rev. 53 (11-12), 1331–1356. doi:10.1080/00206814.2010.527631
Zhao, G. C., Sun, M., Wilde, S. A., and Li, S. Z. (2005). Late archean to paleoproterozoic evolution of the North China craton: key issues revisited. Precambrian Res. 136 (3-4), 177–202. doi:10.1016/j.precamres.2004.10.002
Zhao, G. C., and Zhai, M. G. (2013). Lithotectonic elements of precambrian basement in the North China craton: review and tectonic implications. Gondwana Res. 23 (4), 1207–1240. doi:10.1016/j.gr.2012.08.016
Keywords: Paleoproterozoic mafic rocks, Lieryu formation, Zircon U-Pb chronology, geochemistry, feature of source, tectonic background
Citation: Chen J, Li L, Li B, Tian Y, Zhang C, Zang Y and Zhao Y (2025) Genesis and geological significance of the mafic rocks in the paleoproterozoic Lieryu formation, Liaoning. Front. Earth Sci. 13:1592749. doi: 10.3389/feart.2025.1592749
Received: 13 March 2025; Accepted: 30 April 2025;
Published: 19 May 2025.
Edited by:
Xiaoguang Liu, Shandong University of Science and Technology, ChinaCopyright © 2025 Chen, Li, Li, Tian, Zhang, Zang and Zhao. This is an open-access article distributed under the terms of the Creative Commons Attribution License (CC BY). The use, distribution or reproduction in other forums is permitted, provided the original author(s) and the copyright owner(s) are credited and that the original publication in this journal is cited, in accordance with accepted academic practice. No use, distribution or reproduction is permitted which does not comply with these terms.
*Correspondence: Linchuan Li, bGlsaW5jaHVhbkBtYWlsLmNncy5nb3YuY24=