- 1Second School of Clinical Medicine, Zhongnan Hospital of Wuhan University, Wuhan, China
- 2State Key Laboratory of Cardiovascular Disease, National Center for Cardiovascular Diseases, Fuwai Hospital, Chinese Academy of Medical Sciences and Peking Union Medical College, Beijing, China
Background and Aim: Previous observational studies indicated that the serum albumin levels and circulating metabolites are associated with a high risk of venous thromboembolism (VTE). However, whether these observations reflect causality remained unclear. Hence, we conducted a two-sample Mendelian randomization (MR) analysis to evaluate the causal associations of serum albumin and circulating metabolites with the risk of VTE.
Methods and Results: Summary statistics of genetic instruments proxying serum albumin, total protein, and common circulating metabolites were extracted from genome-wide association studies in the European ancestry. Summary-level results of age- and sex-adjusted estimates for associations of the instruments with VTE were derived from the FinnGen consortium. We used the inverse-variance weighted (IVW) method as the primary analysis for univariable MR. Sensitivity analyses were performed to detect horizontal pleiotropy and outliers. Genetically proxied high-serum albumin and total protein levels were suggestive protective factor of VTE, with odds ratio (OR) = 0.69 (CI 0.54–0.89, p = 4.7 × 10−3) and 0.76 (CI 0.61–0.95, p = 0.015), respectively. Genetically proxied low-monounsaturated fatty acids and the ratio of monounsaturated fatty acid to total fatty acid are causally associated with increased risk of VTE, with ORs = 0.89 (CI 0.80–0.99, p = 0.031) and 0.85 (CI 0.78–0.94, p = 9.92 × 10−4), respectively. There is no indication of causal associations between other circulating metabolites and the risk of VTE.
Conclusions: Genetically liability to low-serum albumin and total protein levels, low proxied monounsaturated fatty acids (MUFAs) and the ratio of MUFAs to total fatty acids are associated with the higher risk of VTE.
Introduction
Venous thromboembolism (VTE), including deep vein thrombosis and pulmonary embolism, is the third most common vascular disease affecting 10 million cases every year and is now a major clinical burden (1, 2). The development of VTE is a complex process involving environmental and genetic factors (3). Previous efforts to prevent VTE mainly focus on the hospital-based factors such as cancer, history of surgery (4). Recent studies have shown that the circulating metabolites are also associated with the risk of VTE (5–8). Patients with hypoproteinemia are more prone to develop VTE. Recent observational cohort studies showed that low-serum albumin levels led to increased risk of VTE in the different populations (5, 6, 8). Metabolic syndrome (MS), characterized by changes in the metabolites including blood glucose, high-density lipoprotein cholesterol (HDL-C), and triglycerides levels, has been identified as a risk factor for various cardiovascular diseases including VTE in several studies (9). Besides, several publications have demonstrated that the use of lipid-lowering drugs decreases the risk of VTE (10–12). Hyperuricemia, hyper-homocysteinemia, and other metabolic dysregulations are also found in the patients with MS (13). However, the causal associations of the circulating metabolites with the risk of VTE are still unclear as conflicting results exist (7). Also, the evidence provided by the previous observational studies may be compromised by confounding factors or reverse causality. Mendelian randomization (MR), which is an epidemiological method exploiting genetic variants associated with exposures for appraising and strengthening causal inferences, diminishes the effect of confounding factors and reverse the causality.
Recent genome-wide association studies (GWASs) have identified the underlying genetic determinants of different circulating metabolites (14, 15). The MR method has been used by several studies for assessing the causal associations between low-serum albumin levels and the increased risk of heart failure and atrial fibrillation (16, 17). Genetic instruments associated with the lipid profile have also been used to examine the effects of lipid levels on several diseases (18, 19). Recent MR studies suggested that the several risk factors are causally associated with the risk of VTE, including taller height, obesity, and red blood cell traits (20–22). However, evidence about the causal associations between circulating metabolites and VTE risk are still lacking. Here, we performed two-sample MR analyses to investigate the relationships between several circulating metabolites [serum albumin, total protein levels, lipid profiles, branched-chain amino acids (BCAAs), etc.] and VTE.
Materials and Methods
Study Design
The schematic view of the study design and three assumptions of MR are presented in Figure 1. The genetic variants associated with the serum albumin and various circulating metabolites were used as genetic instruments (IVs) to examine the causal associations with the risk of VTE. There are three major assumptions that need to be satisfied to perform the MR analyses. First, these genetic variants are supposed to have a direct effect on VTE. Second, the genetic variants have no associations with any known confounding factors. At last, the effects of the genetic variants on VTE should only be mediated by the exposures. Genetic instruments associated with exposures are extracted from previously published GWASs (Table 1). The genetic associations between instrumental variables and the traits were all adjusted for age, sex, and study-specific covariates in the included GWASs. All the included studies had obtained ethical approvements from a relevant institutional review board, and all the subjects included had provided signed informed consent.
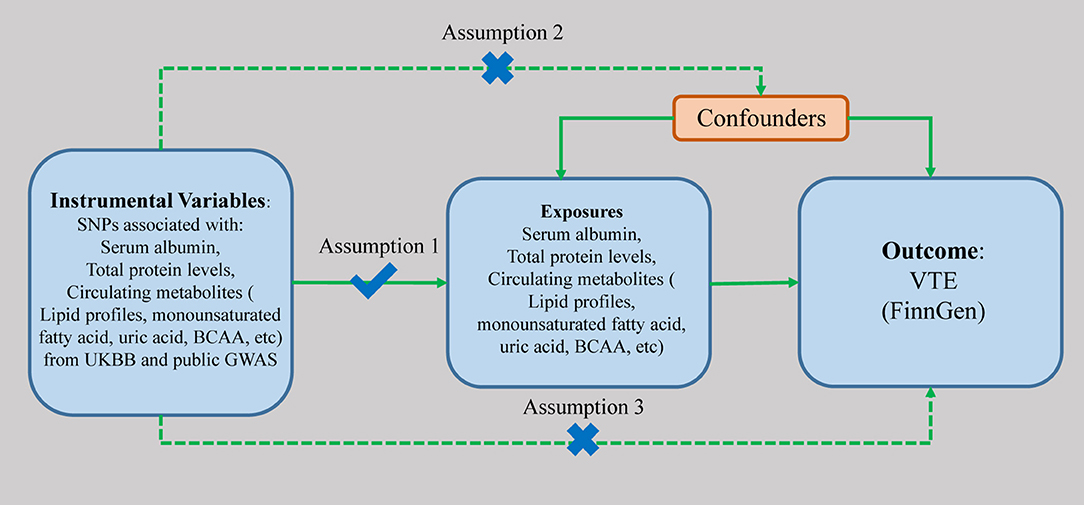
Figure 1. The scheme of study design. SNP, single nucleotide polymorphism; UKBB, UK BioBank; BCAA, branched-chain amino acid; GWAS, genome-wide association study; VTE, venous thromboembolism.
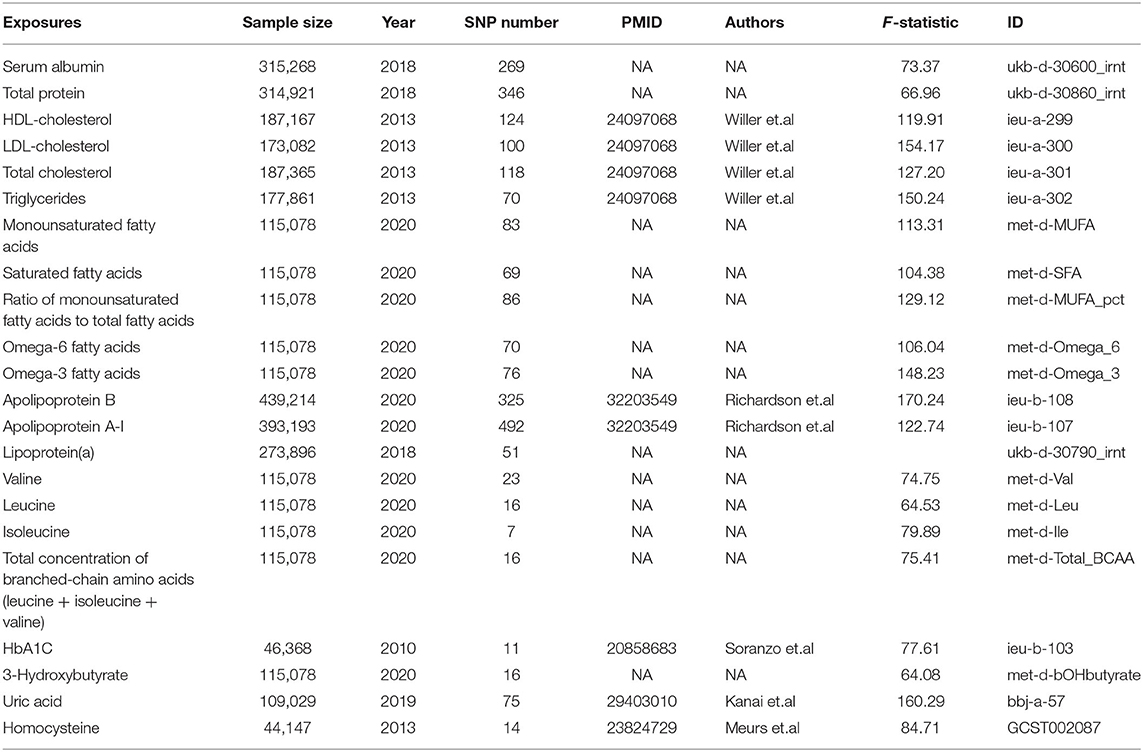
Table 1. Information of the data sources for exposures included in this Mendelian randomization study.
Data Source and SNP Selection
Summary level data of the serum albumin and total protein levels were obtained from the UK BioBank cohort (https://www.ukbiobank.ac.uk/). Summary level data of HDL, low-density lipoproteins (LDL), total cholesterol, and triglycerides levels were obtained from the Global Lipids Genetics Consortium (GLGC) (15). Detailed information of data source for the instrumental variables associated with other exposures is presented in Table 1. Instrumental strength has been characterized by mean F-statistic with the approximation method described by Bowden et al. to test for weak instruments (23) (Table 1, Supplementary Table 2).
Single-nucleotide polymorphisms (SNPs) were identified as associated with the exposures with p-values at the genome-wide significance level (p < 5 × 10–8). SNPs with R2 > 0.01 and within 5,000 kb distance were identified as in linkage disequilibrium and were excluded from the study. For serum albumin and total protein levels, 111 and 146 SNPs were extracted and used as instrumental variables, respectively. The number of used SNPs and details of included SNPs are shown in Table 1 and Supplementary Table 2. Associations of these SNPs with VTE were studied in summary level results including 6,913 patients and 1,69,986 controls from the FinnGen consortium (release 4, https://r4.finngen.fi/).
Statistical Methods
The inverse-variance weighted (IVW) method was used as the main method to assess the causal associations between the exposures and VTE, supported by the multiple sensitivity analyses including MR-Egger, weighted median, and MR-PRESSO (Mendelian Randomization Pleiotropy RESidual Sum and Outlier) methods. MR-Egger method can identify potential pleiotropy (p for intercept < 0.05) and give corrected estimates (24). MR-PRESSO method was used for detecting and correcting for the potential outliers (25). The weighted median method can give consistent causal estimates even when half the weight in the MR analysis came from invalid instrumental variables (26). We used leave-one-out analysis to evaluate the stability of these genetic variants by excluding one individual SNP each time.
All statistical results are two-sided, and a p < 0.0022 (0.05/23 adjusted with Bonferroni method) was considered statistically significant, p between 0.05 and 0.0022 were considered suggestive significant. The statistical analyses were performed with R (version 4.0.2), TwoSampleMR (0.5.5), MR (0.5.0), and MR-PRESSO packages (27, 28).
Results
The associations with VTE per one SD increase in the genetically predicted serum albumin and total protein levels are shown in Figure 2. Genetically proxied serum albumin and total protein levels were inversely correlated to VTE, with odds ratios (ORs) equal to 0.69 (CI 0.54–0.89, p = 4.7 × 10−3) and 0.76 (CI 0.61–0.95, p = 0.015) per one SD increase by using IVW method. MR-PRESSO detected no outliers in the analyses and gave causal estimates consistent with results from IVW (Serum albumin: OR 0.83, 95% CI 0.73–0.94, p = 5.6 × 10−3; Total protein: OR 0.87, 95% CI 0.78–0.97, p = 0.016). No horizontal pleiotropy was identified with MR-egger method (Serum albumin: p = 0.23; Total protein: p = 0.66). We further replicated the MR analyses with VTE data from UK BioBank. Associations of both the serum albumin and total protein levels with VTE remained in the same direction and reached p < 0.05 (data not shown).
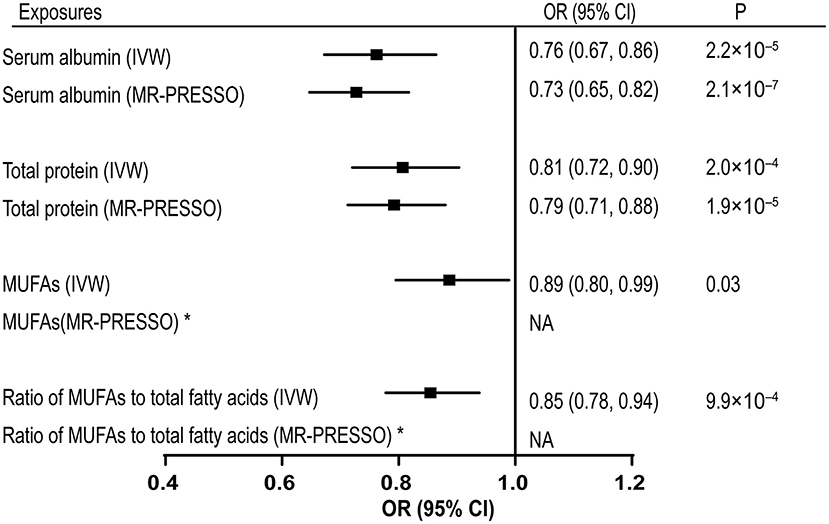
Figure 2. Associations of genetically predicted serum albumin, total protein, MUFAs levels, and ratio of MUFAs to total fatty acids with VTE. MUFAs, monounsaturated fatty acids; VTE, venous thromboembolism; IVW, inverse-variance weighted; MR-PRESSO, Mendelian Randomization Pleiotropy RESidual Sum and Outlier; OR, odds ratio; CI, confidence interval. *No outliers detected. NA, no adjusted estimates is available.
Genetically proxied monounsaturated fatty acids (MUFAs) and the ratio of monounsaturated fatty acids to total fatty acids were causally associated with the risk of VTE by using the IVW method, with ORs of 0.89 (CI 0.80–0.99, p = 0.031) and 0.85 (CI 0.78–0.94, p = 9.92 × 10−4), respectively (Supplementary Table 1). No outliers were detected in the SNPs associated with these two exposures. However, the MR-egger method detected significant pleiotropic effects on the SNPs used for the ratio of monounsaturated fatty acids to total fatty acids, indicating potential confounding effect (intercept = 0.010, p = 0.028). The estimates provided by MR-egger supported a causal association between the ratio of monounsaturated fatty acids to total fatty acids and VTE risk (OR = 0.75, CI 0.65–0.87, p = 2.19 × 10−4) (Supplementary Table 1). No causal association was identified between other circulating metabolites and the risk of VTE (Supplementary Table 1). Scatter plots for the associations of included 22 exposures with VTE are shown in Supplementary Figures 1–22. Leave-one-out plots (serum albumin, total protein, MUFAs, ratio of MUFAs to the total fatty acids) were shown in Supplementary Figures 23–26.
Discussion
This MR study demonstrated that the genetically proxied serum albumin, total protein levels, MUFAs, and the ratio of MUFAs to the total fatty acids are inversely associated with the risk of VTE. There is no indication of causal effects of other circulating metabolites, including the measurements in the lipid profiles, BCAA, glucose, uric acid, homocysteine, carnitine, and three-hydroxybutyrate on the risk of VTE.
Serum Albumin and Total Protein With VTE
Several observational cohort studies indicated that a lower level of serum albumin level can increase the risk of VTE in the different individuals (8, 29). The study based on the two population cohorts: the Atherosclerosis Risk in Communities (ARIC) Study and the Cardiovascular Health Study (CHS) suggested that the low-serum albumin level is the modest marker of increased risk of VTE with the adjusted hazard ratio (HR) per SD lower albumin equal to 1.18 (95% CI = 1.08, 1.31) in the ARIC and 1.10 (95% CI = 0.94, 1.29) in CHS, after adjustment with other VTE-associated risk factors (29). Another long-term cohort, which enrolled 2,176 male individuals in the Caucasian ancestries with an average follow-up of 24.8 years, also showed that one SD lower serum albumin was associated with a higher risk of VTE, with HR = 1.23 (95% CI 1.02–1.47), and this association was independent of CRP levels (8). In addition, the cancer patients and individuals with nephrotic syndromes were also shown to be more likely to develop VTE (5). Our study, for the first time, by using MR method, demonstrated that the higher levels of serum albumin and total protein levels show a causal protective effect on the risk of VTE based on GWASs from the relatively large populations. Of note, our results are less susceptible to biases including the confounders and reverse causality. This is of particular importance in disease conditions considering the dramatic changes in circulating metabolites in the human microenvironment. Therefore, our data put forward another layer of evidence supporting the causal associations of the serum albumin and total protein levels with the risk of VTE.
Metabolic Syndrome-Associated Metabolites With VTE
Lipid Profiles and VTE
Previous observational studies provided conflicting evidence about the associations of several traits in lipid profiles and the risk factors of VTE. For example, one study demonstrated that Lp(a) levels above 30 mg/dl were more than twice likely to be found in the patients with VTE as compared with the controls (30). An observational cohort study enrolling 27,081 healthy women with an average follow-up of 11.4 years showed no causal relationships between any lipid profile trait and VTE. Subgroup analyses in individuals taking hormonal replacement therapy suggested that extreme tertile of HDL-C and apo-AI levels were associated with an increased risk of unprovoked VTE (9). However, our MR analyses showed no causal associations between any of the lipids with VTE. The conflicting results might be because of the biases led by limited sample size or uncharacterized confounding factors in the observational studies. Of note, our results are supported by the Prevention of Renal and Vascular Endstage Disease (PREVEND) study, which is based on a large cohort including all the habitant aged 28–75 years living in Groningen, the Netherland (31). The PREVEND study showed that the apolipoproteins were not associated with a higher risk of VTE in both the univariable and multivariable analyses. Although the classical lipoproteins: TC, non-HDL, LDL, TG, and TC/HDL ratio were showed to increase the risk of VTE in the univariable analyses, the significance was absent after the multivariable adjustment with age and sex (31).
Likewise, we did not see causation of the saturated fatty acid, omega-3 fatty acid, and omega-6 fatty acid with VTE, though multiple previous studies suggested a protective effect of long-chained n-3 polyunsaturated fatty acids (n-3 PUFAs) with the risk of VTE (32–35). This might be because these studies were focusing on hospitalized patients, therefore confounding factors such as immobilization and liver function deficiency might play additional roles in promoting VTE. However, we saw a suggestive protective effect of MUFAs and the ratio of MUFAs to total fatty acid level. The roles of MUFAs in inhibiting pro-inflammatory gene expression and anti-oxidation have been widely studied (36). Further studies are required to investigate whether the anti-inflammatory effects from MUFAs can exert a beneficial effect on preventing VTE. Our study is a complement to a recent MR analysis, showing specific protective effect of MUFA on the risk of VTE without affecting the risk of other major cardiovascular disorders (37).
HbA1C, Uric Acid, Homocysteine, and VTE
Our MR study also suggested that the genetically proxied HbA1C is not associated with high-VTE risk. These data are in line with previous findings from the population-based cohorts (38–40). Venous thromboembolism In Northern Sweden (VEINS) cohort study suggested that not only fasting plasma glucose (FPG), oral glucose tolerance test [2-h post-load plasma glucose (2HPG)] but clinically also diagnosed diabetes have no association with increased risk of VTE after multiple confounder adjustment (age, sex, body mass index, cancer at inclusion, education level, smoking, and hypertension) (39). The Tromsø study obtained similar results suggesting no relationships between high HbA1C levels and increased risk of VTE (38). Besides, though diabetes can increase the risk of VTE by using various models to adjust for the effects from confounders, elevated HbA1C levels in individuals without diabetes are not related to a higher risk of VTE (41).
Several cohort studies showed that serum uric acid (SUA) level is an independent risk factor of VTE (42–44). Though our MR data does not show a significant association between high level with VTE, the p-value is around the border of suggestive significance (p = 0.078) and suggested a trend toward increasing VTE risk. Likewise, there is no indication of elevated homocysteine in increasing the VTE risk, which suggested that the association seen between homocysteine and VTE might be due to confounders (45).
BCAA, 3-Hydroxybutyrate, and Other Metabolites With VTE
A previous pilot study applied nuclear magnetic resonance spectroscopy (NMR) and mass spectrometry (MS) method to measure the levels of BCAA, 3-hydroxybutyrate in plasma, serum, thrombus, and vein wall in human samples and suggested higher levels of BCAA and 3- hydroxybutyrate in individuals with VTE (46). However, the causations cannot be inferred and the associations between these metabolites and VTE are still elusive. Our data confirmed that there is no causal association between the levels of BCAA and 3-hydroxybutyrate with VTE.
Limitations
There are several limitations with this study. First, we assumed that the correlations of different exposures with VTE are linear in the MR analysis. Second, we are unable to perform population stratification. The conclusions might be compromised if there are differences in allele frequency in different populations. Third, the results are based on European ancestry. Future studies on mixed populations or other populations are needed to extend our conclusion.
Conclusion
In Summary, our MR study demonstrated the causal effects of genetically proxied lower serum, total protein levels, MUFAs, and the ratio of MUFAs to total fatty acids on the risk of VTE. Targeting these factors might be a potential strategy to prevent VTE.
Data Availability Statement
Publicly available datasets were analyzed in this study. This data can be found at: https://r4.finngen.fi/.
Author Contributions
JM and ZL conceptualized and designed the study, analyzed the data, and wrote the manuscript. All authors read and approved the final manuscript.
Conflict of Interest
The authors declare that the research was conducted in the absence of any commercial or financial relationships that could be construed as a potential conflict of interest.
Publisher's Note
All claims expressed in this article are solely those of the authors and do not necessarily represent those of their affiliated organizations, or those of the publisher, the editors and the reviewers. Any product that may be evaluated in this article, or claim that may be made by its manufacturer, is not guaranteed or endorsed by the publisher.
Acknowledgments
The authors thank all participants and investigators for the contributions of UK BioBank, FinnGen, and other GWAS data.
Supplementary Material
The Supplementary Material for this article can be found online at: https://www.frontiersin.org/articles/10.3389/fnut.2021.712600/full#supplementary-material
Supplementary Figures 1–22. Scatter plot shows individual causal estimates from each genetic variant associated with each exposure on the x-axis and VTE on the y-axis.
Supplementary Figures 23–26. Leave-one-out analysis of serum albumin, total protein, MUFAs, and ratios of MUFAs to the total fatty acid.
Supplementary Table 1. Associations of 22 exposures with venous thromboembolism (VTE) in Mendelian randomization analyses.
Supplementary Table 2. Single-nucleotide polymorphisms used for each exposure.
References
1. Heit JA. Epidemiology of venous thromboembolism. Nat Rev Cardiol. (2015) 12:464–74. doi: 10.1038/nrcardio.2015.83
2. Di Nisio M, van Es N, Buller HR. Deep vein thrombosis and pulmonary embolism. Lancet. (2016) 388:3060–73. doi: 10.1016/S0140-6736(16)30514-1
3. Rosendaal FR. Venous thrombosis: a multicausal disease. Lancet. (1999) 353:1167–73. doi: 10.1016/S0140-6736(98)10266-0
4. Goldhaber SZ. Risk factors for venous thromboembolism. J Am Coll Cardiol. (2010) 56:1–7. doi: 10.1016/j.jacc.2010.01.057
5. Konigsbrugge O, Posch F, Riedl J, Reitter EM, Zielinski C, Pabinger I, et al. Association between decreased serum albumin with risk of venous thromboembolism and mortality in cancer patients. Oncologist. (2016) 21:252–7. doi: 10.1634/theoncologist.2015-0284
6. Gyamlani G, Molnar MZ, Lu JL, Sumida K, Kalantar-Zadeh K, Kovesdy CP. Association of serum albumin level and venous thromboembolic events in a large cohort of patients with nephrotic syndrome. Nephrol Dial Transplant. (2017) 32:157–64. doi: 10.1093/ndt/gfw227
7. Morelli VM, Lijfering WM, Bos MHA, Rosendaal FR, Cannegieter SC. Lipid levels and risk of venous thrombosis: results from the MEGA-study. Eur J Epidemiol. (2017) 32:669–81. doi: 10.1007/s10654-017-0251-1
8. Kunutsor SK, Seidu S, Katechia DT, Laukkanen JA. Inverse association between serum albumin and future risk of venous thromboembolism: interrelationship with high sensitivity C-reactive protein. Ann Med. (2018) 50:240–8. doi: 10.1080/07853890.2018.1441537
9. Everett BM, Glynn RJ, Buring JE, Ridker PM. Lipid biomarkers, hormone therapy and the risk of venous thromboembolism in women. J Thromb Haemost. (2009) 7:588–96. doi: 10.1111/j.1538-7836.2009.03302.x
10. Glynn RJ, Danielson E, Fonseca FA, Genest J, Gotto AM Jr, Kastelein JJP, et al. A randomized trial of rosuvastatin in the prevention of venous thromboembolism. N Engl J Med. (2009) 360:1851–61. doi: 10.1056/NEJMoa0900241
11. Ramcharan AS, Van Stralen KJ, Snoep JD, Mantel-Teeuwisse AK, Rosendaal FR, Doggen CJ. HMG-CoA reductase inhibitors, other lipid-lowering medication, antiplatelet therapy, and the risk of venous thrombosis. J Thromb Haemost. (2009) 7:514–20. doi: 10.1111/j.1538-7836.2008.03235.x
12. Rahimi K, Bhala N, Kamphuisen P, Emberson J, Biere-Rafi S, Krane V, et al. Effect of statins on venous thromboembolic events: a meta-analysis of published and unpublished evidence from randomised controlled trials. PLoS Med. (2012) 9:e1001310. doi: 10.1371/journal.pmed.1001310
13. Jang MJ, Choi WI, Bang SM, Lee T, Kim YK, Ageno W, et al. Metabolic syndrome is associated with venous thromboembolism in the Korean population. Arterioscler Thromb Vasc Biol. (2009) 29:311–5. doi: 10.1161/ATVBAHA.109.184085
14. Soranzo N, Sanna S, Wheeler E, Gieger C, Radke D, Dupuis J, et al. Common variants at 10 genomic loci influence hemoglobin A(1)(C) levels via glycemic and nonglycemic pathways. Diabetes. (2010) 59:3229–39. doi: 10.2337/db10-0502
15. Willer CJ, Schmidt EM, Sengupta S, Peloso GM, Gustafsson S, Kanoni S, et al. Discovery and refinement of loci associated with lipid levels. Nat Genet. (2013) 45:1274–83. doi: 10.1038/ng.2797
16. Liao LZ, Zhang SZ, Li WD, Liu Y, Li JP, Zhuang XD, et al. Serum albumin and atrial fibrillation: insights from epidemiological and mendelian randomization studies. Eur J Epidemiol. (2020) 35:113–22. doi: 10.1007/s10654-019-00583-6
17. Zhuang XD, Zhang SZ, Liao LZ, Lin X, Zhou HM, Zhong XB, et al. Serum albumin and incident heart failure: insights from epidemiological and mendelian randomization studies. Circ Genom Precis Med. (2020) 13:e002989. doi: 10.1161/CIRCGEN.120.002989
18. Beeghly-Fadiel A, Khankari NK, Delahanty RJ, Shu XO, Lu Y, Schmidt MK, et al. A mendelian randomization analysis of circulating lipid traits and breast cancer risk. Int J Epidemiol. (2020) 49:1117–31. doi: 10.1093/ije/dyz242
19. Richardson TG, Sanderson E, Palmer TM, Ala-Korpela M, Ference BA, Davey Smith G, et al. Evaluating the relationship between circulating lipoprotein lipids and apolipoproteins with risk of coronary heart disease: a multivariable mendelian randomisation analysis. PLoS Med. (2020) 17:e1003062. doi: 10.1371/journal.pmed.1003062
20. Roetker NS, Armasu SM, Pankow JS, Lutsey PL, Tang W, Rosenberg MA, et al. Taller height as a risk factor for venous thromboembolism: a Mendelian randomization meta-analysis. J Thromb Haemost. (2017) 15:1334–1343. doi: 10.1111/jth.13719
21. Luo S, Au Yeung SL, Zuber V, Burgess S, Schooling CM. Impact of genetically predicted red blood cell traits on venous thromboembolism: multivariable mendelian randomization study using UK Biobank. J Am Heart Assoc. (2020) 9:e016771. doi: 10.1161/JAHA.120.016771
22. Yuan S, Bruzelius M, Xiong Y, Hakansson N, Akesson A, Larsson SC. Overall and abdominal obesity in relation to venous thromboembolism. J Thromb Haemost. (2021) 19:460–9. doi: 10.1111/jth.15168
23. Bowden J, Del Greco MF, Minelli C, Davey Smith G, Sheehan NA, Thompson JR. Assessing the suitability of summary data for two-sample mendelian randomization analyses using MR-Egger regression: the role of the I2 statistic. Int J Epidemiol. (2016) 45:1961–74. doi: 10.1093/ije/dyw220
24. Burgess S, Thompson SG. Erratum to: interpreting findings from mendelian randomization using the MR-Egger method. Eur J Epidemiol. (2017) 32:391–2. doi: 10.1007/s10654-017-0276-5
25. Verbanck M, Chen CY, Neale B, Do R. Detection of widespread horizontal pleiotropy in causal relationships inferred from mendelian randomization between complex traits and diseases. Nat Genet. (2018) 50:693–8. doi: 10.1038/s41588-018-0099-7
26. Bowden J, Davey Smith G, Haycock PC, Burgess S. Consistent estimation in mendelian randomization with some invalid instruments using a weighted median estimator. Genet Epidemiol. (2016) 40:304–14. doi: 10.1002/gepi.21965
27. Hemani G, Zheng J, Elsworth B, Wade KH, Haberland V, Baird D, et al. The MR-Base platform supports systematic causal inference across the human phenome. Elife. (2018) 7:e012. doi: 10.7554/eLife.34408.012
28. Verbanck M, Chen CY, Neale B, Do R. Publisher correction: detection of widespread horizontal pleiotropy in causal relationships inferred from mendelian randomization between complex traits and diseases. Nat Genet. (2018) 50:1196. doi: 10.1038/s41588-018-0164-2
29. Folsom AR, Lutsey PL, Heckbert SR, Cushman M. Serum albumin and risk of venous thromboembolism. Thromb Haemost. (2010) 104:100–4. doi: 10.1160/TH09-12-0856
30. von Depka M, Nowak-Gottl U, Eisert R, Dieterich C, Barthels M, Scharrer I, et al. Increased lipoprotein (a) levels as an independent risk factor for venous thromboembolism. Blood. (2000) 96:3364–8. doi: 10.1182/blood.V96.10.3364
31. van Schouwenburg IM, Mahmoodi BK, Gansevoort RT, Muntinghe FL, Dullaart RP, Kluin-Nelemans HC, et al. Lipid levels do not influence the risk of venous thromboembolism. Results of a population-based cohort study. Thromb Haemost. (2012) 108:923–9. doi: 10.1160/TH12-06-0426
32. Reiner MF, Stivala S, Limacher A, Bonetti NR, Mean M, Egloff M, et al. Omega-3 fatty acids predict recurrent venous thromboembolism or total mortality in elderly patients with acute venous thromboembolism. J Thromb Haemost. (2017) 15:47–56. doi: 10.1111/jth.13553
33. Isaksen T, Evensen LH, Braekkan SK, Hansen JB. Dietary intake of marine polyunsaturated n-3 fatty acids and risk of recurrent venous thromboembolism. Thromb Haemost. (2019) 119:2053–63. doi: 10.1055/s-0039-1697663
34. Isaksen T, Evensen LH, Johnsen SH, Jacobsen BK, Hindberg K, Braekkan SK, et al. Dietary intake of marine n-3 polyunsaturated fatty acids and future risk of venous thromboembolism. Res Pract Thromb Haemost. (2019) 3:59–69. doi: 10.1002/rth2.12168
35. Zhang Y, Ding J, Guo H, Liang J, Li Y. Associations of fish and omega-3 fatty acids consumption with the risk of venous thromboembolism. A meta-analysis of prospective cohort studies. Front Nutr. (2020) 7:614784. doi: 10.3389/fnut.2020.614784
36. Herrera-Marcos LV, Lou-Bonafonte JM, Arnal C, Navarro MA, Osada J. Transcriptomics and the mediterranean diet: a systematic review. Nutrients. (2017) 9:472. doi: 10.3390/nu9050472
37. Mazidi M, Katsiki N, Shekoohi N, Banach M. Monounsaturated fatty acid levels may not affect cardiovascular events: results from a mendelian randomization analysis. Front Nutr. (2020) 7:123. doi: 10.3389/fnut.2020.00123
38. Lerstad G, Brodin EE, Enga KF, Jorde R, Schirmer H, Njolstad I, et al. Hyperglycemia, assessed according to HbA1c, and future risk of venous thromboembolism: the tromso study. J Thromb Haemost. (2014) 12:313–9. doi: 10.1111/jth.12498
39. Johansson M, Lind M, Jansson JH, Fharm E, Johansson L. Fasting plasma glucose, oral glucose tolerance test, and the risk of first-time venous thromboembolism. A report from the VEINS cohort study. Thromb Res. (2018) 165:86–94. doi: 10.1016/j.thromres.2018.03.015
40. Akirov A, Grossman A, Shochat T, Shimon I. Blood glucose on admission and mortality in patients with venous thromboembolism. J Diabetes Complications. (2017) 31:358–63. doi: 10.1016/j.jdiacomp.2016.06.019
41. Bell EJ, Selvin E, Lutsey PL, Nambi V, Cushman M, Folsom AR. Glycemia (hemoglobin A1c) and incident venous thromboembolism in the atherosclerosis risk in communities cohort study. Vasc Med. (2013) 18:245–50. doi: 10.1177/1358863X13506764
42. Kubota Y, McAdams-DeMarco M, Folsom AR. Serum uric acid, gout, and venous thromboembolism: the atherosclerosis risk in communities study. Thromb Res. (2016) 144:144–8. doi: 10.1016/j.thromres.2016.06.020
43. Yu M, Ling K, Teng Y, Li Q, Mei F, Li Y, et al. Serum uric acid is associated with increased risk of idiopathic venous thromboembolism in high HDL-C population: a case-control study. Exp Ther Med. (2016) 11:2314–20. doi: 10.3892/etm.2016.3228
44. De Lucchi L, Nardin C, Sponchiado A, Raggi D, Faggin E, Martini E, et al. Serum uric acid levels and the risk of recurrent venous thromboembolism. J Thromb Haemost. (2021) 19:194–201. doi: 10.1111/jth.15139
45. Marouf R, Mojiminiyi O, Qurtom M, Abdella N, Al Wazzan H, Al Humood S, et al. Plasma homocysteine and hematological factors in patients with venous thromboembolic diseases in Kuwait. Acta Haematol. (2007) 117:98–105. doi: 10.1159/000097384
Keywords: mendelian randomization analysis, venous thromboembolism, serum albumin, metabolic syndrome, monounsaturated fatty acid
Citation: Liu Z and Mi J (2021) Serum Albumin and Circulating Metabolites and Risk of Venous Thromboembolism: A Two-Sample Mendelian Randomization Study. Front. Nutr. 8:712600. doi: 10.3389/fnut.2021.712600
Received: 20 May 2021; Accepted: 06 October 2021;
Published: 11 November 2021.
Edited by:
Ani Manichaikul, University of Virginia, United StatesReviewed by:
Vittorio Calabrese, University of Catania, ItalyAgnieszka Micek, Jagiellonian University, Poland
Copyright © 2021 Liu and Mi. This is an open-access article distributed under the terms of the Creative Commons Attribution License (CC BY). The use, distribution or reproduction in other forums is permitted, provided the original author(s) and the copyright owner(s) are credited and that the original publication in this journal is cited, in accordance with accepted academic practice. No use, distribution or reproduction is permitted which does not comply with these terms.
*Correspondence: Jiarui Mi, amlhcnVpLm1pLmRyQGdtYWlsLmNvbQ==