- 1School of Public Health, Capital Medical University, Beijing, China
- 2Feihe Research Institute,Heilongjiang Feihe Dairy Co., Ltd., Beijing, China
- 3Children’s Hospital of Nanjing Medical University, Nanjing, China
- 4National Institute for Nutrition and Health, Chinese Center for Disease Control and Prevention, Beijing, China
- 5Department of Rehabilitation Medicine, The Second Affiliated Hospital of Jiaxing University, The Second Hospital of Jiaxing, Jiaxing, China
- 6School of Medicine and Nursing, Huzhou University, Huzhou, Zhejiang, China
Introduction: The diversity of dairy products and the increasing consumption levels have led to a growing interest in goat and sheep milk, which are rich in essential nutrients and functional components. The study aims to explore the nutritional composition, growth performance, digestibility, and serum metabolic differences of milk powders from cow, goat, and sheep using LC–MS/MS-based metabolomics in rat models.
Methods: Sixty male Sprague-Dawley rats were fed with whole cow, goat, and sheep milk powder samples, and their feces and urine were analyzed for fat and protein content. LC/MS analysis was conducted using a Dionex UltiMate 3000 UHPLC system coupled with a Thermo Q EXACTIVE mass spectrometer, with data processed using Wekemo Bioincloud for quality control, normalization, comparisons with the KEGG database, statistical analyses, and selection of differential metabolites.
Results: The sheep milk powder showed highest protein and fat content level, while cow and goat milk powders separately demonstrated higher lactose and carbohydrate levels. Each milk powder had a unique mineral profile, with sheep milk powder containing the highest calcium content. All groups exhibited consistent growth in body weight and high rates of protein and fat digestibility. Metabolomics analysis revealed distinct metabolic profiles, with goat milk powder linked to steroid hormone biosynthesis and sheep milk powder associated with hormone regulation and bile acid pathways.
Conclusion: This study offers valuable insights into the metabolic implications of different milk powder sources, informing dietary choices and facilitating the development of targeted public health strategies to optimize nutritional intake and promote overall well-being.
Introduction
People’s choice of dairy products has become more diverse with changes in consumption levels. Compared with the year 2011 level, dairy consumption is expected to increase by 58% by 2050 (1). Therefore, the scale of the dairy industry and different types of dairy product will gradually expand. Goat milk production represents 2.1% of global milk production (2). Additionally, 95% of the world’s goat population is located in Asia, Africa, and Latin America. It is worth noting that Asia accounts for approximately 60% of the total goat population. China’s goat milk production increased from 54,000 tons in 1969 to 223,134 tons in 2018, with an impressive yearly average growth rate of 3.31% (3). Shanxi Province in China is home to the largest storage facility for goat milk (4). Goat milk contains relatively less lactose and fat but is rich in calcium, antimicrobial factors, antioxidants, and other functional components essential to human health (5). Similar to human breast milk, goat milk contains a high level of antimicrobial enzymes, such as lysozyme, which may enhance infants’ immunity against numerous infections (6). Due to its similarities with human breast milk, goat milk has been recognized as an important substitute for human breast milk (7). Sheep milk and products made from sheep milk have gained popularity in many populations and areas, especially among people with cow milk allergies. In ancient times, milk was primarily collected from small farms with a limited number of animals and consumed locally. However, with increasing urbanization and demand for dairy products in the early 1900s, the dairy industry underwent industrialization in more developed countries (8). Comparatively, sheep milk production in many countries still occurs on a small scale and is considered less significant in terms of volume. Nevertheless, sheep and goat milk have likely been used by humans for a longer time than cow milk, as sheep and goats were domesticated earlier than cattle (9).
Metabolomics has been employed in the investigation of dairy products through using an untargeted metabolomics approach by a gas chromatography coupled with mass spectrometry (GC–MS) to study the metabolite profiles of sheep’s and goat’s milk (10). Milk from cow, sheep, and goat in the ‘Alto Casertano’ region of Italy exhibits varying protein and amino acid profiles, with sheep milk having the highest total protein content level and goat milk containing unique amino acids such as taurine (10). In previous studies, researchers have utilized non-targeted Liquid Chromatography Mass Spectrometry (LC/MS) metabolomics approaches to characterize the profile of milk metabolites and identify differences between human milk and milk from cows, horses, and goats (11). However, those existing studies on dairy product metabolomics primarily focused on analyzing initial materials, lacking insights into in vivo metabolism. Thus, the current study aims to bridge this gap by providing a comprehensive understanding of the nutritional composition, growth performance, digestibility, and serum metabolic differences by using LC–MS/MS-based metabolomics analysis of rat models on milk powder diets from cow, goat, and sheep in order to explore potential metabolic pathways.
Materials and methods
Milk powder
The initial powdered materials obtained from the milk include full-cream cow milk powder sourced from New Zealand and the Netherlands, full-cream goat milk powder from New Zealand, the Netherlands, and Spain, and full-cream sheep milk powder from New Zealand, Italy, Spain, and Romania. These materials undergo a series of processes including filtration, purification, and sterilization to eliminate microorganisms, concentration to enhance richness, and spray drying to transform the liquid into a powder form.
Testing of the nutritional components of milk powder samples
Sample was weighed 100 mg of milk powder samples and added them to 0.6 mL of water. After 15 min of sonication, 15 rounds of 30-s sonication and 1 min of off-sonication were performed in a Bioruptor sonication device (Diagenode) for homogenization, before proceeding with the nutritional component analysis. The basic nutrients were determined in accordance with the Chinese National Standard such as protein by Kjeldahl method (GB 5009.5–2016), fat by Gas chromatography method (GB 5009.168–2016), lactose by High Performance Liquid chromatography (HPLC) method (GB 5413.5–2010), moisture by direct drying method (GB 5009.3–2010), docosahexaenoic acid (DHA), linoleic acid, linolenic acid, and other fatty acids by acetyl chloride-methanol methyl esterification method (GB 5413.27–2010),amino acids by the Amino acid automatic analyzer (A300, Amino acid Analyzer, MembraPure GmbH) (GB 5413.27–2010), calcium, iron, zinc, sodium, potassium, magnesium, copper, manganese by atomic absorption method (GB 5413.21–2010), vitamin A by HPLC method (GB 5009.82–2016),vitamin B1 by HPLC method (GB 5009.84–2016), vitamin B2 by HPLC method (GB 5009.85–2016), and folic acid by HPLC method (GB 5413.16–2010).
The formulation of milk powder feed
To equalize the protein levels among the three types of initial milk powder, whey protein powder corresponding to each type was used for supplementation based on the measured protein content in each type of initial milk powder. The milk powder solution was prepared at a concentration of 40% (wet basis), indicating that the milk powder comprised 40% of the total solution weight. Subsequently, the fat content in the milk powder and the added whey protein powder were adjusted to a consistent level by using full-cream cow milk powder from New Zealand and the Netherlands, full-cream goat milk powder from New Zealand, the Netherlands, and Spain, and full-cream sheep milk powder from New Zealand, Italy, Spain, and Romania, respectively, in the three types of milk powder solutions, the powder formulation should be presented in Supplementary Table S1. The solutions were then spray-dried to obtain powdered forms, which were subsequently physically compressed into rod-shaped forms (Nantong Teluofei Feed Technology Co., Ltd., Nantong, China). The resulting feeds underwent sterilization by using Co60 radiation at a dosage of 25 kGy.
Animals and rearing conditions
The study has been approved by the Animal Ethics Committee of China Center for Disease Control and Prevention Ethics Committee (EAWE-2018-015), ensuring compliance with ethical guidelines for animal research. Sixty Sprague–Dawley rats (3–4 weeks old; male) were purchased from the Vital River Laboratory Animal Technology Co. Ltd. (Beijing, China). The rats were housed in individual metabolic cages. They were housed in an animal room maintained under standard conditions. The rats were provided with a maintenance feed and water ad libitum. The room conditions included a 12-h/12-h light/dark cycle, controlled temperature at 22°C, and access restrictions. The animals were kept under specific pathogen-free conditions, with daily temperature fluctuations being around 3°C, relative humidity ranging from 40 to 70%, airflow velocity of approximately 0.18 m/s, and a room air pressure gradient of 20–50 Pa. After 3 days of acclimation, animals were randomly divided into three groups, with 20 rats for each based on their body weights. Group names are as follows: C: whole cow milk group; G: whole goat milk group; S: whole sheep milk group. After 1 week of dietary feeding, each group was provided with the corresponding milk powder feed and ad libitum access to water, and the mice were anesthetized with 10% chloral hydrate (0.1 mL/10 g body weight). Blood samples were collected into EDTA-containing tubes by being drawn from the abdominal aorta, and were then centrifuged at 1,600 g for 20 min at 4°C. Collected plasma was aliquoted and snap-frozen by using liquid nitrogen.
Collection and processing of fecal and urine samples in rat digestibility study
On the 4th day, a 24-h fasting period was commenced, with free access for rats to water. This was followed by a digestive experimental phase involving feeding from the morning of the 5th day until the morning of the 10th day. On the morning of the 5th day, the body weight of the rats was measured (in grams), and approximately 14 g of feed was provided. Feces and urine samples were collected in the morning and afternoon. From the morning of the 6th day to the morning of the 9th day, the weight of 5 mL centrifuge tubes (in grams), rat body weight (in grams), remaining feed weight (in grams), and the amount of feed replenished (approximately 14 g) were recorded to accurately measure the weight of feces and urine. Feces and urine samples were collected in the morning and afternoon of each day. On the morning of the 10th day, a 24-h period of fasting with unrestricted water access was implemented. Feces and urine were collected in the morning and afternoon of the 10th day, as well as in the morning of the 11th day. The urine samples were collected in centrifuge tubes and fixed with 10% hydrochloric acid, and feces were collected in 5 mL centrifuge tubes and fixed with 10% hydrochloric acid.
Detection of metabolites related to fat and protein metabolism
The determination of fat content was carried out according to the method specified in GB5009.6–2016 “Determination of Fat in Foods.” Approximately 0.6 g of thoroughly mixed freeze-dried fecal samples and 10 g of urine samples were weighed. The fecal samples were then placed in a steam dish with approximately 20 g of quartz sand, and dried in an oven at 100 ± 5°C for 30 min. The dried samples were then finely ground and transferred into a filter paper tube. Following this, the filter paper tube was placed in the Soxhlet extractor’s extraction tube, connected to a dried receiving bottle, and subjected to extraction with petroleum ether for approximately 8 h. After the extraction was completed, the remaining solvent in the receiving bottle was evaporated on a water bath, dried in an oven at 100 ± 5°C for 1 h, and weighed until a constant weight was achieved.
Protein content was determined according to the method specified in GB5009.5–2016 “Determination of Protein in Foods.” Approximately 0.6 g of thoroughly mixed freeze-dried fecal samples and 10 g of urine (centrifuged at 6000 r/min) were weighed and transferred into a dried 100 mL Kjeldahl flask. Subsequently, 0.4 g of copper sulfate, 6 g of potassium sulfate, and 20 mL of sulfuric acid were added to the flask. The mixture was then heated until carbonization was complete, followed by the addition of water and subsequent transferred into a 100 mL volumetric flask for further processing. The process included the preparation of reagent blank tests, setting up of the Kjeldahl distillation apparatus, and subsequent titration of the distillate with standard sulfuric acid solution until the endpoint color turned to gray-blue.
The combined daily volume of urine samples from each animal group was recorded. A certain volume of urine was aliquoted for protein and fat content determination, and the total protein and total fat content in the urine were calculated. The combined feces from each animal group were dried daily, weighed, and the total weight was recorded. A portion of the feces was aliquoted for protein and fat content determination, and the total protein and total fat content in the feces were calculated. The apparent protein/fat digestibility rate was calculated as (food nitrogen/fat – fecal nitrogen/fat) divided by food nitrogen/fat, multiplied by 100%. The true protein/fat digestibility rate was calculated as [food nitrogen/fat – (fecal nitrogen/fat – metabolic fecal nitrogen/fat)] divided by food nitrogen/fat, multiplied by 100%. The net protein utilization rate was calculated as [food nitrogen – (fecal nitrogen – fecal metabolic nitrogen) – (urinary nitrogen – urinary metabolic nitrogen)] divided by food nitrogen, multiplied by 100%.
The biological value of proteins was calculated as [food nitrogen – (fecal nitrogen – nitrogen from fecal metabolism) – (urine nitrogen – endogenous urinary nitrogen)] divided by [food nitrogen – (fecal nitrogen – nitrogen from fecal metabolism)] multiplied by 100%. The net protein utilization rate (NPU), defined as the product of biological value and true digestibility rate, represented the efficiency of protein utilization in the body. Where fecal nitrogen and fecal fat were the nitrogen and fat content in the feces of rats from the group fed with nitrogen-free feed, a nitrogen-free diet formulation has been provided in Supplementary Table S2.
Pretreatment
Serum samples were prepared by mixing 20 μL of serum with 200 μL of protein precipitant methanol-acetonitrile (V: V = 1:1) thoroughly, centrifuged at 10,000 rpm for 10 min, and 5 μL of serum were taken for LC–MS/MS analysis.
Liquid chromatography-mass spectrometry analysis (LC/MS)
All LC/MS experiments were carried out by using a Dionex UltiMate 3,000 UHPLC (Thermo Scientific, MA) system equipped with a binary solvent delivery manager and a sample manager, coupled with a Thermo Q EXACTIVE operating in positive (ESI+) and negative (ESI−) electrospray ionization mode (Thermo Fisher Scientific, Sunnyvale, CA, United States). The liquid chromatography was conducted by using an Acquity BEH C18 column (50 mm × 2.1 mm, 1.7 μm particle size; Waters, Milford, MA, United States). Separation was achieved with solvent A [2 mmoL/L ammonium formate and 0.1% (v/v) formic acid] and solvent B (acetonitrile) with the following gradient at a cow rate of 0.25 mL/min: 0 min (5% B), 0–1 min (5% B), 1–5 min (5–60% B), 5–8 min (60–100% B), 8–11 min (100% B), 11–14 min (100–60% B), 14–15 min (60–5% B), and 15–18 min (5% B). The injection volume was 5 μL and the analysis time was 18 min. The mass spectrometric data were collected by using a Thermo Q EXACTIVE mass spectrometer. The mass spectrometer parameters in this experiment were as follows: the voltage was 2.8 kV; the sheath gas: 35 Arb; the auxiliary gas: 10 Arb; the capillary temperature: 350°C; the s-lens RF: 50. The resolution of the first full scan (Full scan) was 70,000; AGC target: 1e6; Maximum TT: 100 ms; the scan range: 70–1,050 m/z. The resolution of the secondary data dependency scan (Full MS/dd-MS2) was 35,000; AGC target: 1e5; Maximum TT:50 ms; NCE:20, 40, 60.
Quality control
To ensure the consistency of the analysis process, it was reported that the quality control (QC) sample was initially prepared using 10 μL of serum from each sample. It was also noted that during the analysis, water samples and QC samples were injected once for every 10 samples to monitor sample preparation and instrument stability.
Data processing
Raw data analysis was performed by using Wekemo Bioincloud, a free online platform for data analysis1. Raw data was processed using Proteowizard software (v3.0.8789) for quality assessment and quality control to detect outliers and remove metabolites or samples that exceeded threefold standard error during data preprocessing. Metabolomics workflow was based on the R (v3.1.3) language MetaboAnalystR package (12). Each metabolite was then compared with the KEGG database br08001 (13) to determine its percentage content in each biological role. Statistical analyses, including compound summaries and assessments of structural differences, were performed. Chemometric analysis of serum metabolites was conducted for all groups, utilizing principal component analysis (PCA), Partial Least Squares Discriminant Analysis (PLS-DA) (14), and Orthogonal Partial Least Squares Discriminant Analysis (OPLS-DA) (15). Differential serum metabolites between the groups were identified based on the variable importance in projection (VIP > 1) from OPLS-DA and the false discovery rate (FDR) from t-tests (FDR < 0.05). For the selection of differential metabolites, machine learning techniques such as random forest were employed, and only the results with FDR < 0.05 were shown. To provide intuitive insights into relationships, functional patterns, and metabolic pathways, correlation analyses, over-representation analysis (ORA) of pathways (15), topology analysis of pathways, and metabolic pathway maps, along with the topological structure of metabolites within the enriched pathways with pathway impact >0.1 and FDR < 0.05, were deemed key differential pathways between groups.
Statistical analysis
The data was presented as the mean + standard deviation (SD) and was analyzed by one-way analysis of variance (one-way ANOVA). If significant (p < 0.05) differences were found by the ANOVA test, the t-test was used to determine pairwise differences between means. All statistical analyses were carried out by using SPSS (IBM SPSS 23.0, SPSS Inc.).
Results
Nutritional components of cow milk powder, goat milk powder, and sheep milk powder
The macronutrient analysis revealed sheep milk powder as the richest one in protein and fat, cow milk powder as the highest one in lactose, and goat milk powder was leading in carbohydrates (Table 1).
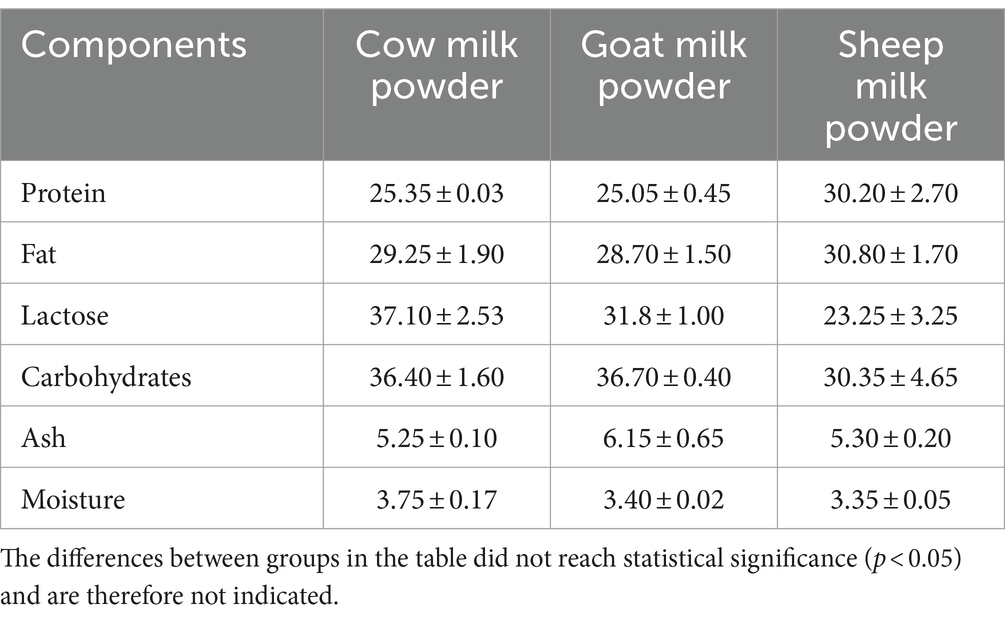
Table 1. Moisture and ash content of cow milk powder, goat milk powder, and sheep milk powder (g/100 g).
Fatty acid of cow milk powder, goat milk powder, and sheep milk powder
The fatty acid analysis of cow, goat, and sheep milk powders (Tables 2, 3) showed significant differences. Goat milk powder had the highest total fatty acid content (30.54 g/100 g), followed by sheep milk powder (29.60 g/100 g), and cow milk powder (27.03 g/100 g). Sheep milk powder exhibited the highest levels of monounsaturated and polyunsaturated fatty acids, Ω-3, arachidonic acid, and α-linolenic acid among the three types. Cow milk powder had the lowest content of saturated, monounsaturated, and polyunsaturated fatty acids.
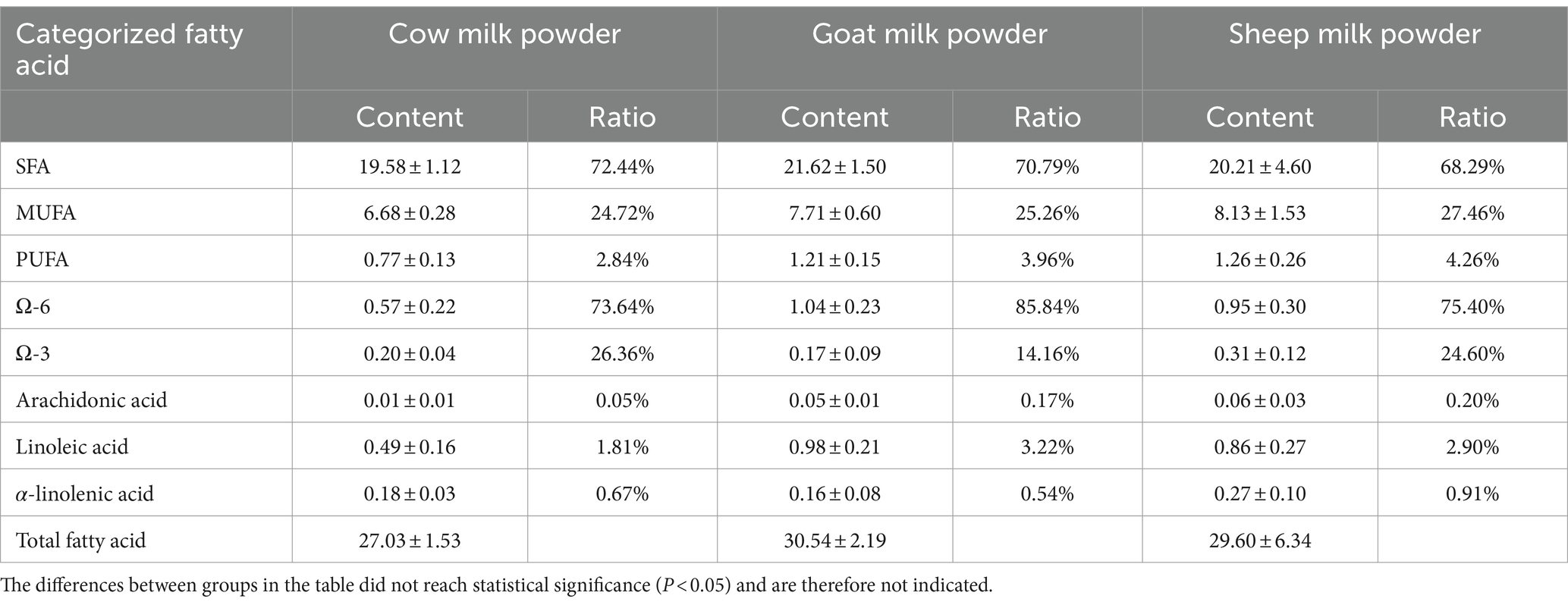
Table 3. The categorized fatty acid content (g/100 g) and its proportion relative to the total fatty acids in three types of powdered milk.
Protein nutritional composition analysis of three types of powdered milk
Sheep milk powder showed the highest total amino acid content (25.07 g/100 g) among cow, goat, and sheep milk powders (Tables 4, 5). It also had the highest proportions of individual amino acids to total proteins, reaching 83.0%. Cow and goat milk powders exhibited notable levels of specific amino acids like glutamic acid, leucine, and lysine, but overall, sheep milk powder outperformed in amino acid composition.
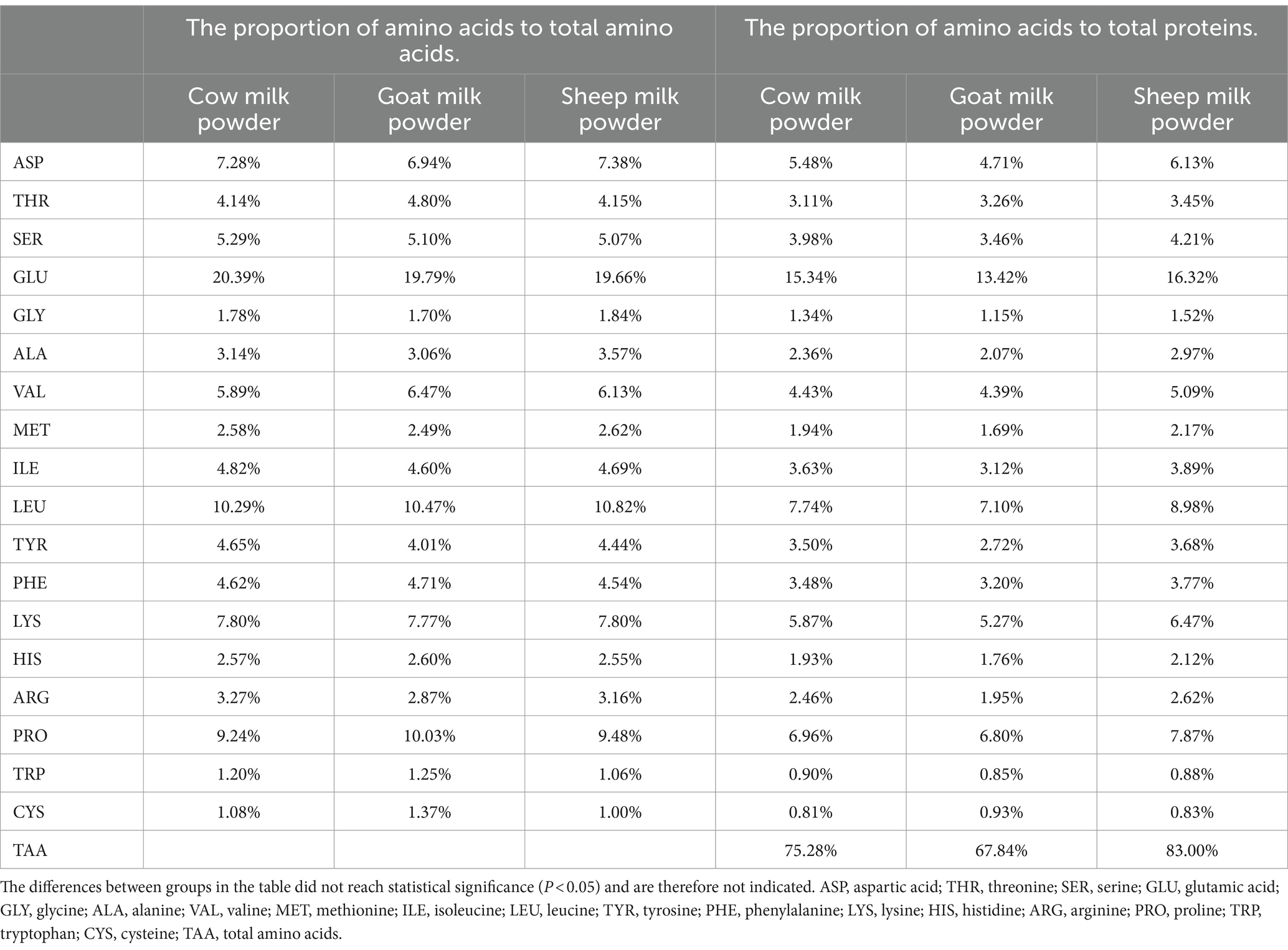
Table 5. The proportion of amino acids to total amino acids and total proteins in three types of powdered milk.
Analysis of the vitamin composition of three types of powdered milk
Sheep milk powder contained the highest levels of vitamin A, vitamin B1, and vitamin B2 among cow, goat, and sheep milk powders (Table 6). Cow milk powder had the highest folate content, while goat milk powder showed the lowest levels of vitamin B1 and B2.
Mineral element analysis of three types of powdered milk
Sheep milk powder showed the highest calcium content among cow, goat, and sheep milk powders, while goat milk powder exceled in potassium and magnesium levels (Table 7). Cow milk powder generally exhibited lower mineral levels compared to the other types, except for sodium and zinc, where it surpassed both goat and sheep milk powders.
The growth status of rats fed three types of powdered milk
The changes in rat body weight after 7 days of feeding with three different types of milk powder were depicted in Figures 1A,B. There were minimal differences in food intake among the rat groups fed with the three types of milk powder, however, a consistent trend was observed across all three milk powder groups, with rat body weight showing an increasing tendency.
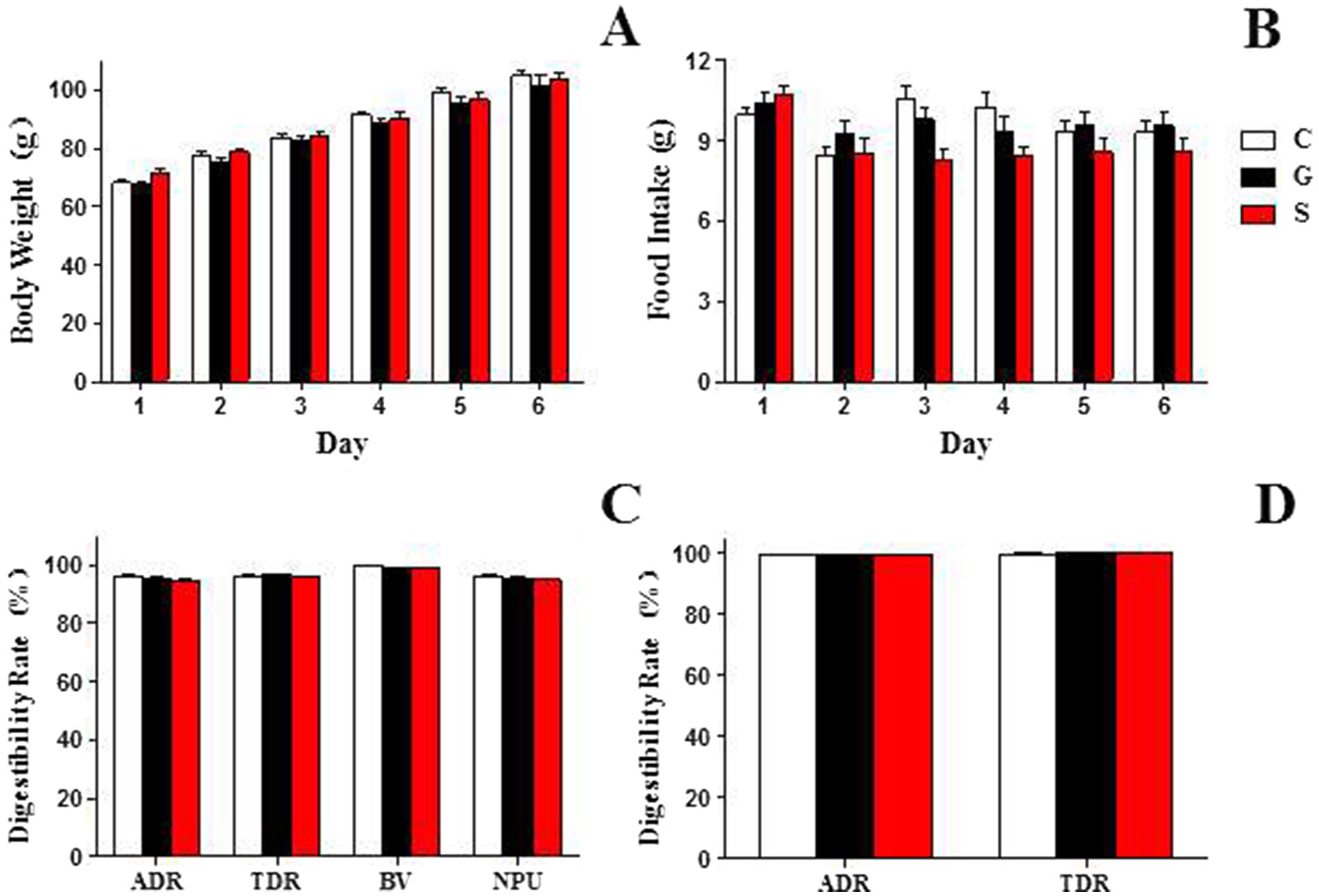
Figure 1. The growth status and digestion and absorption profiles of rats fed three types of powdered milk feed. (A) The body weight changes among rat groups consuming the three types of powdered milk feed. (B) The food intake among rat groups fed with the three types of powdered milk feed. (C) The digestibility and absorption rates of protein from the three different types of powdered milk feed. (D) The digestibility rates of fat from the three different types of powdered milk feed. ADR, apparent digestibility rate; TDR, true digestibility rate; BV, biological value; NPU, net protein utilization rate. C, Cow milk powder group (n = 20); G, Goat milk powder group (n = 20); S, Sheep milk powder group (n = 20). The differences between groups in the table did not reach statistical significance (p < 0.05) and are therefore not indicated.
The digestion and absorption profiles of three types of powdered milk
This study investigated the digestibility and absorption rates of three types of milk powder proteins (Figure 1C): goat, sheep, and cow milk powders. Digestibility rates (%) for goat, sheep, and cow milk powders were 95.39 ± 0.44, 94.80 ± 0.15, and 95.19 ± 1.11, respectively. True digestibility (%) values were 96.54 ± 0.38, 95.99 ± 0.27, and 96.34 ± 0.82, respectively. Biological values (%) were 98.93 ± 0.37, 98.95 ± 0.30, and 99.86 ± 0.18, and net utilization rates (%) were 95.50 ± 0.71, 94.98 ± 0.47, and 96.20 ± 0.92 for goat, sheep, and cow milk powders, respectively. For milk powder fat (Figure 1D), digestibility rates (%) were 99.52 ± 0.06, 99.61 ± 0.10, and 99.39 ± 0.15, and true digestibility (%) values were 99.96 ± 0.17, 100.07 ± 0.08, and 99.83 ± 0.19 for goat, sheep, and cow milk powders, respectively.
Metabolite content analysis of three types of powdered milk group
Metabolite analysis of three types of powdered milk showed minimal differences in overall metabolic composition based on PCA (Figure 2A). Further analysis by using PLS-DA and O-PLS-DA (16) revealed distinct metabolite profiles among cow, sheep, and goat milk powders (Figures 2B,C). Cow milk powder was notably higher in lipids, sheep milk powder in organic acids, and goat milk powder in steroids. Peptide and nucleic acid contents were relatively consistent across all groups, while vitamins, cofactors, hormones, and transmitters showed minimal variation or negligible presence (Figures 2D,E).
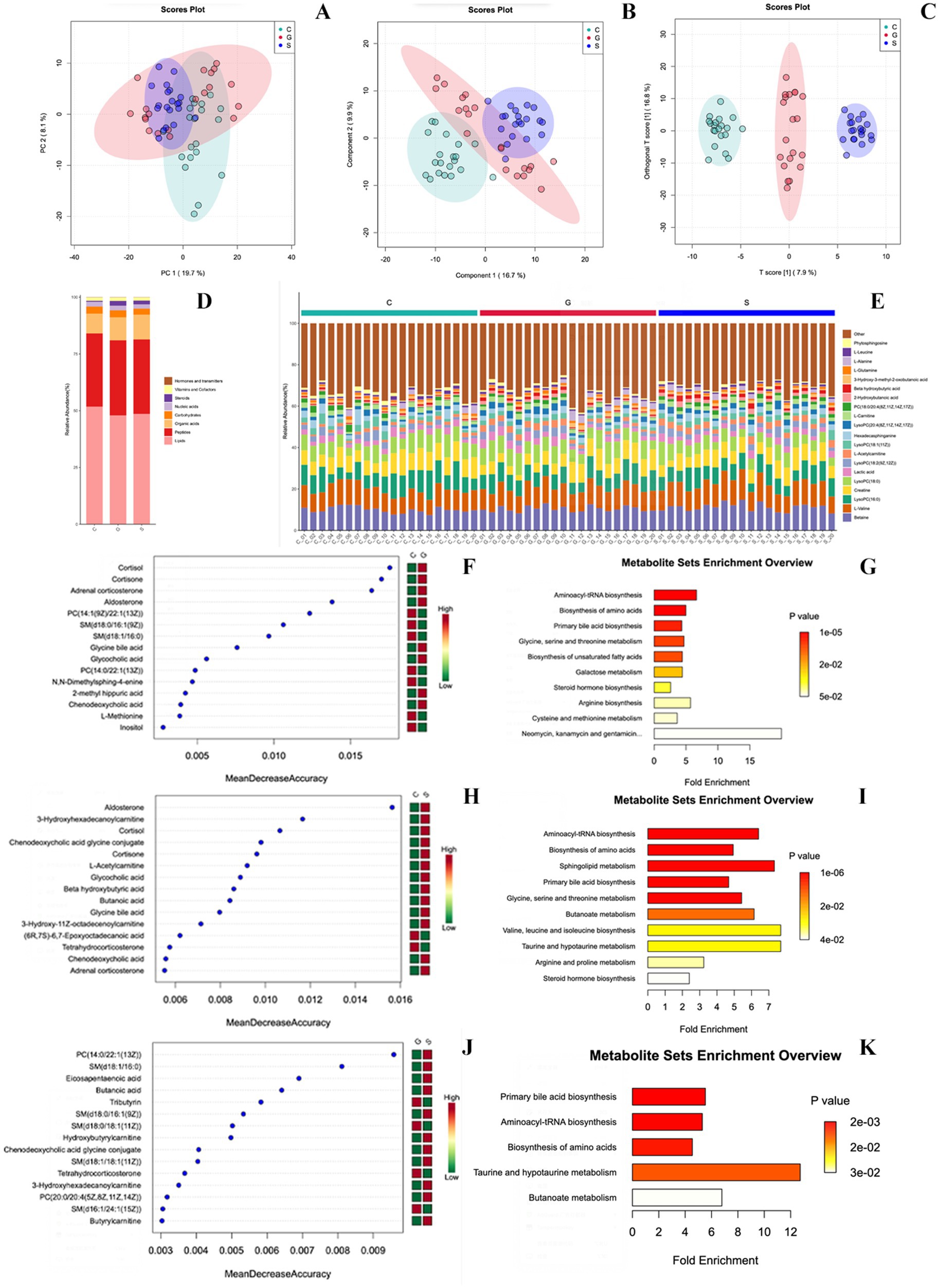
Figure 2. Metabolomics analysis of three powdered milk groups. (A) PCA of metabolites. (B) PLS-DA of metabolites. (C) O-PLS-DA of metabolites. (D) Biomolecule categories among groups (Lipids, Peptides, Organic acids, Carbohydrates, Nucleic acids, Steroids, Vitamins, Cofactors, Hormones, and transmitters). (E) Different metabolites per sample. (F) Random forest algorithm for differential metabolites (Cow vs. Goat). (G) Over-Representation Analysis (ORA) based on KEGG pathways (Cow vs. Goat). (H) Random forest algorithm for differential metabolites (Cow vs. Sheep). (I) ORA based on KEGG pathways (Cow vs. Sheep). (J) Random forest algorithm for differential metabolites (Goat vs. Sheep). (K) ORA based on KEGG pathways (Goat vs. Sheep). C, Cow milk powder (n = 20); G, Goat milk powder (n = 20); S, Sheep milk powder (n = 20).
Metabolite identification
The study indicates that the percentage composition of metabolites in serum samples from three types of powdered milk has been identified and quantified. This analysis is visually represented by using stacked bar charts, facilitating direct comparisons of metabolic differences between groups. Figures 2F–K highlights the top 20 metabolites by abundance, grouping the remaining under ‘Others’. The random forest algorithm was applied to identify differential metabolites between different three types of powdered milk groups (Figures 2F,H,J). Over-Representation Analysis (ORA) based on KEGG pathways elucidated key biological pathways influencing metabolic processes in the serum of rats under different formula-fed regimens (Figures 2G,I,K).
Comparative analysis between cow milk powder and goat milk powder groups (Figures 2F,G) revealed significant differences, particularly in metabolites related to steroid hormone biosynthesis (e.g., cortisol and aldosterone) and bile acid metabolism. Similar comparisons between cow milk powder and sheep milk powder groups (Figures 2H,I) highlighted associations with hormonal regulation and pathways such as amino acid and lipid metabolism. Comparing sheep milk powder and goat milk powder groups (Figures 2J,K) uncovered significant associations with various metabolic pathways, including lipid metabolism and amino acid synthesis, influenced by specific metabolites like primary bile acids and fatty acids.
Discussion
The study provides a comprehensive comparative analysis of the nutritional composition, growth performance, digestibility, and metabolomic profiles of milk powder diets from cows, goats, and sheep in rat models. The comprehensive comparative analysis of milk powders from sheep, goats, and cows reveals significant variations in both macronutrient and micronutrient compositions. Sheep milk powder stands out with the highest protein content and lowest lactose content among the three types, while goat milk powder shows the highest carbohydrate content. Additionally, sheep milk powder demonstrates superior levels of monounsaturated and polyunsaturated fatty acids, including essential fatty acids such as Ω-3, arachidonic acid, and α-linolenic acid, distinguishing it from the other types. Sheep milk powder also exhibits the highest total amino acid content and proportion to total proteins, with notable elevations in specific amino acids like glutamic acid, leucine, proline, lysine, aspartic acid, valine, and serine, highlighting its nutritional superiority.
Previous studies have demonstrated that sheep colostrum typically contains higher protein levels compared to other domesticated animal milks, including water buffalo (17, 18), camel (19), cattle (20), goat (21), horse (22), and donkey colostrum (23). Previous research has also observed that sheep milk had a higher total fat content compared to cow (24) or goat milk (25). Cow’s milk generally has a higher lactose content compared to goat and sheep milk, which makes it harder to digest (26). Sheep milk powder stood out with the lowest carbohydrate content, for those needing to control carbohydrate intake, sheep milk powder may be a preferable option. Sheep milk powder contains the highest levels of monounsaturated fatty acids, polyunsaturated fatty acids, omega-3 fatty acids, arachidonic acid, and alpha-linolenic acid among the three types of powdered milk. These findings are consistent with previous research (24), indicating that sheep milk tends to exhibit a higher proportion of omega-3 polyunsaturated fatty acids (PUFAs) and PUFA bio hydrogenation intermediates (PUFA-BHIs), such as conjugated linoleic acid (CLA), trans-mono-unsaturated fatty acids (t-MUFAs), vaccenic acid (VA), rumenic acid (RA), and branched-chain fatty acids (BCFAs), compared to cow milk. This difference is attributed to a less extensive bio hydrogenation process in sheep milk (24). This study confirms that sheep milk powder exhibits a markedly higher total amino acid content and proportion relative to total proteins compared to both cow and goat milk powders.
This finding is consistent with previous research (27) and is underscored by notable increases in specific amino acids, namely glutamic acid, leucine, proline, lysine, aspartic acid, valine, and serine. Furthermore, the amino acid profiles derived from milks power of cows, sheep, and goats display distinct characteristics, serving as hallmark indicators of these respective species.
The analysis of three types of powdered milk unveils significant disparities in both vitamin and mineral compositions, with sheep milk powder exhibiting the highest levels of vitamin A, B1, and B2, along with superior calcium content, while cow milk powder excels in folate content and demonstrates higher sodium and zinc levels, and goat milk powder presents the lowest levels of vitamin B1 and B2, as well as distinctive potassium and magnesium levels. The variation in the concentration of different components found in milk depends on mammalian species, genetic, physiological, nutritional factors, and environmental conditions, concentrations of different vitamin and mineral components vary depending on mammalian species, genetics, physiological factors, nutritional requirements, and environmental factors (28).
The study investigates the growth performance and digestion kinetics of rats fed with three types of powdered milk. Despite minimal differences in food intake, all three milk powder groups exhibited a consistent trend of increased body weight over 7 days. Assessment of protein and fat digestibility revealed high apparent and true digestibility rates across goat, sheep, and cow milk powder groups, with slight variations evaluated, demonstrating high apparent and true digestibility rates with slight variations among the groups. This study also found that the protein digestibility revealed high rates across goat, sheep, and cow milk powder groups, surpassing previous researches (29–31). The mean digestibility of goat milk protein (94.0%) was similar to that of cow milk protein isolate and skimmed milk protein (95.1 and 95.5%) (32, 33). Additionally, it compared reasonably well (94.0%) with the digestibility of goat milk protein concentrate in rats (34). The high digestibility of three type of milk protein renders it a crucial animal-sourced foodstuff for populations predominantly adhering to vegetarian diets (35) and in countries where meat consumption frequency is low, mitigating the risk of inadequate quality protein intake (36). From both nutritional and economic perspectives, fat is one of the most critical components of milk. This research has also shown that the true digestibility rate for fat content in the three types of milk powders is approximately 99%. The study indicates that milk fat intake is often associated with a higher risk of cardiovascular disease (CVD) due to its significant saturated fat content. Yet, it is important to understand that saturated fatty acids (SFAs) are not a monolithic group; they have structural differences that can lead to varying impacts on biological processes (37). Therefore, future research should pay attention to the impact of the structure of milk fatty acids on health.
In spite of the worldwide consumption of bovine milk, small ruminant milk, such as goat’s and sheep’s milk, is gaining a great deal of attention, especially in the Mediterranean region (38). This study analyzed serum metabolites in rats fed with cow, goat, and sheep milk powders, identifying key differences through random forest analysis and pathway enrichment. Comparing the cow, goat, and sheep milk powder groups revealed distinct metabolic signatures in vivo. In comparison to the cow milk powder group, metabolites in the serum of rats fed goat milk powder, such as cortisol, cortisone, adrenal corticosterone, and aldosterone, which are essential for steroid hormone biosynthesis, highlight the significance of this pathway in the synthesis of crucial adrenal cortex hormones. Simultaneously, glycine bile acid and glycocholic acid play a crucial role in the metabolism of glycine, serine, and threonine, connecting to primary bile acid biosynthesis through the generation and metabolism of bile acids. Metabolites in the serum of rats fed sheep milk powder, including hormonal regulators such as aldosterone, cortisol, and tetrahydrocorticosterone, as well as bile acids like chenodeoxycholic acid glycine conjugate, actively engage in bile acid pathways while being modulated by taurine and hypotaurine metabolism. Due to their close phylogenetic relationship, the goat milk powder-fed group and the sheep milk powder-fed group exhibit fewer enriched metabolic pathways in vivo compared to the milk powder-fed group derived from species such as cows. These pathways mainly involve lipid metabolism and its regulation by specific metabolites, notably within butanoate metabolism and primary bile acid biosynthesis. Within butanoate metabolism, metabolites such as eicosapentaenoic acid, butanoic acid, and tributyrin serve as regulators, impacting the metabolism of short-chain fatty acids. Steroid hormones play an essential role in regulating water and salt balance, metabolism, and stress response, as well as in initiating and maintaining sexual differentiation and reproduction (39). Bile acids facilitate the absorption of lipids in the gut but are also involved in maintaining cholesterol homeostasis, releasing bile, excreting toxic substances, and controlling energy metabolism. The synthesis of bile acids, including cholic acid and chenodeoxycholic acid, is a complex process involving at least 17 enzymes and metabolite transport proteins. Disorders of bile acid synthesis can affect individuals in various ways, ranging from cholestatic liver disease to neuropsychiatric symptoms and spastic paraplegia (40). Butanoate metabolism is the metabolic fate of several short-chain fatty acids or short-chain alcohols typically produced by intestinal fermentation. These molecules are eventually converted into ketone bodies, short-chain lipids, citrate cycle precursors, glycolysis precursors, or glutamine precursors (41). Overall, this study provides comprehensive insights into the metabolic profiles and pathway associations influenced by different formula-fed regimens in rat serum samples.
Conclusion
In summary, this study reveals significant disparities in nutritional composition and metabolic responses among cow, goat, and sheep milk powders. Sheep milk powder demonstrates a superior nutritional profile, with elevated levels of protein, essential fatty acids, amino acids, vitamins, and minerals. All three milk powders exhibit high rates of protein and fat digestibility, highlighting their efficacy as quality nutrition sources for diverse dietary needs. Furthermore, distinct metabolic signatures were identified in rats administered these milk powder formulations, emphasizing the crucial roles of steroid hormone biosynthesis and bile acid metabolism in eliciting physiological responses. Notably, despite their close evolutionary relationship, goat and sheep milk powders display unique metabolic enrichments, especially in lipid metabolism pathways. This study offers valuable insights into the metabolic implications of different milk powder sources, informing dietary choices and facilitating the development of targeted public health strategies to optimize nutritional intake and promote overall well-being.
Data availability statement
The raw data supporting the conclusions of this article will be made available by the authors, without undue reservation.
Ethics statement
The animal study was reviewed and approved by Animal Ethics Committee of China Center for Disease Control and Prevention Ethics Committee. The study was conducted in accordance with the local legislation and institutional requirements.
Author contributions
CY: Writing – original draft. JP: Writing – original draft. SP: Writing – review & editing. SH: Visualization, Writing – review & editing. ML: Data curation, Writing – review & editing. XZ: Writing – review & editing. LS: Writing – review & editing. XR: Project administration, Writing – review & editing. ZW: Project administration, Writing – review & editing.
Funding
The author(s) declare that no financial support was received for the research, authorship, and/or publication of this article.
Acknowledgments
Authors would like to thank all the teachers in the Department of Nutrition and Food Hygiene, School of Public Health, Capital Medical University, for their help in this study.
Conflict of interest
JP, SP, XZ, and LS are employed by Feihe Research Institute, Heilongjiang Feihe Dairy Co., Ltd.
The remaining authors declare that the research was conducted in the absence of any commercial or financial relationships that could be construed as a potential conflict of interest.
Publisher’s note
All claims expressed in this article are solely those of the authors and do not necessarily represent those of their affiliated organizations, or those of the publisher, the editors and the reviewers. Any product that may be evaluated in this article, or claim that may be made by its manufacturer, is not guaranteed or endorsed by the publisher.
Supplementary material
The Supplementary material for this article can be found online at: https://www.frontiersin.org/articles/10.3389/fnut.2024.1428938/full#supplementary-material
Footnotes
References
2. Tsakalidou, E. O. I. , Microbiota of goat’s milk and goat’s milk cheese; Proceedings of the First Asia Dairy Goat Conference; Kuala Lumpur, Malaysia. (2012).
3. The milk production of goats in China. Available at: https://knoema.com/data/china+agriculture-indicators-production+goats+milk (Accessed 22 September 2020).
4. Liang, JB, and Paengkoum, P. Current status, challenges and the way forward for dairy goat production in Asia – conference summary of dairy goats in Asia. Asian Australas J Anim Sci. (2019) 32:1233–43. doi: 10.5713/ajas.19.0272
5. Prajapati, DB, Kapadiya, DB, Jain, AK, Mehta, BM, Darji, VB, and Aparnathi, KD. Comparison of Surti goat milk with cow and buffalo milk for physicochemical characteristics, selected processing-related parameters and activity of selected enzymes. Vet World. (2017) 10:477–84. doi: 10.14202/vetworld.2017.477-484
6. Xu, M, Wang, Y, Dai, Z, Zhang, Y, Li, Y, and Wang, J. Comparison of growth and nutritional status in infants receiving goat milk-based formula and cow milk-based formula: a randomized, double-blind study. Food Nutr Res. (2015) 59:28613. doi: 10.3402/fnr.v59.28613
7. Tannock, GW, Lawley, B, Munro, K, Gowri Pathmanathan, S, Zhou, SJ, Makrides, M, et al. Comparison of the compositions of the stool microbiotas of infants fed goat milk formula, cow milk-based formula, or breast milk. Appl Environ Microbiol. (2013) 79:3040–8. doi: 10.1128/AEM.03910-12
8. Ranadheera, CS, Naumovski, N, and Ajlouni, S. Non-bovine milk products as emerging probiotic carriers: recent developments and innovations. Curr Opin Food Sci. (2018) 22:109–14. doi: 10.1016/j.cofs.2018.02.010
9. The variation of animals and plants under domestication. Br Foreign Med Chir Rev. (1868) 42:143–66.
10. Caboni, P, Murgia, A, Porcu, A, Manis, C, Ibba, I, Contu, M, et al. A metabolomics comparison between sheep's and goat's milk. Food Res Int. (2019) 119:869–75. doi: 10.1016/j.foodres.2018.10.071
11. Wu, R, Chen, J, Zhang, L, Wang, X, Yang, Y, and Ren, X. LC/MS-based metabolomics to evaluate the milk composition of human, horse, goat and cow from China. Eur Food Res Technol. (2021) 3:1–13. doi: 10.1007/s00217-020-03654-1
12. Chong, J, and Xia, J. MetaboAnalystR: an R package for flexible and reproducible analysis of metabolomics data. Bioinformatics. (2018) 34:4313–4. doi: 10.1093/bioinformatics/bty528
13. Kanehisa, M, and Goto, S. KEGG: Kyoto encyclopedia of genes and genomes. Nucleic Acids Res. (2000) 28:27–30. doi: 10.1093/nar/28.1.27
14. Lee, LC, Liong, CY, and Jemain, AA. Partial least squares-discriminant analysis (PLS-DA) for classification of high-dimensional (HD) data: a review of contemporary practice strategies and knowledge gaps. Analyst. (2018) 143:3526–39. doi: 10.1039/C8AN00599K
15. Boccard, J, and Rutledge, DN. A consensus orthogonal partial least squares discriminant analysis (OPLS-DA) strategy for multiblock omics data fusion. Anal Chim Acta. (2013) 769:30–9. doi: 10.1016/j.aca.2013.01.022
16. Westerhuis, JA, van Velzen, EJJ, Hoefsloot, HCJ, and Smilde, AK. Multivariate paired data analysis: multilevel PLSDA versus OPLSDA. Metabolomics. (2010) 6:119–28. doi: 10.1007/s11306-009-0185-z
17. Puppel, K, Gołębiewski, M, Grodkowski, G, Slósarz, J, Kunowska-Slósarz, M, Solarczyk, P, et al. Composition and factors affecting quality of bovine colostrum: A review. Animals. (2019) 9:1070. doi: 10.3390/ani9121070
18. Duan, H, Sun, Q, Chen, C, Wang, R, and Yan, W. A review: the effect of bovine colostrum on immunity in people of all ages. Nutrients. (2024) 16:2007. doi: 10.3390/nu16132007
19. Liu, C, Liu, LX, Yang, J, and Liu, YG. Exploration and analysis of the composition and mechanism of efficacy of camel milk. Food Biosci. (2023) 53:102564. doi: 10.1016/j.fbio.2023.102564
20. Arslan, A, Kaplan, M, Duman, H, Bayraktar, A, Ertürk, M, Henrick, BM, et al. Bovine colostrum and its potential for human health and nutrition. Front Nutr. (2021) 8:651721. doi: 10.3389/fnut.2021.651721
21. ALKaisy, QH, al-Saadi, JS, al-Rikabi, AKJ, Altemimi, AB, Hesarinejad, MA, and Abedelmaksoud, TG. Exploring the health benefits and functional properties of goat milk proteins. Food Sci Nutr. (2023) 11:5641–56. doi: 10.1002/fsn3.3531
22. Roy, D, Ye, A, Moughan, PJ, and Singh, H. Composition, structure, and digestive dynamics of Milk from different species-A review. Front Nutr. (2020) 7:577759. doi: 10.3389/fnut.2020.577759
23. Fox, P. F., Uniacke-Lowe, T., McSweeney, P. L. H., and O'Mahony, J. A., Dairy chemistry and biochemistry. Milk Proteins (2015): Springer International Publishing; Cham, Switzerland. 145–239
24. Moatsou, G, and Sakkas, L. Sheep milk components: focus on nutritional advantages and biofunctional potential. Small Rumin Res. (2019) 180:86–99. doi: 10.1016/j.smallrumres.2019.07.009
25. Chilliard, YGFF . Diet rumen biohydrogenation and nutritional quality of cow and goat milk fat. Eur J Lipid Sci Technol. (2007) 109:828–55. doi: 10.1002/ejlt.200700080
26. Park, YW, Juárez, M, Ramos, M, and Haenlein, GFW. Physico-chemical characteristics of goat and sheep milk. Small Rumin Res. (2007) 68:88–113. doi: 10.1016/j.smallrumres.2006.09.013
27. Landi, N, Ragucci, S, and Di Maro, A. Amino acid composition of Milk from cow, sheep and goat raised in Ailano and Valle Agricola, two localities of ‘alto Casertano’ (Campania region). Food Secur. (2021) 10:2431. doi: 10.3390/foods10102431
28. Pietrzak-Fiećko, R, and Kamelska-Sadowska, AM. The comparison of nutritional value of human Milk with other Mammals' Milk. Nutrients. (2020) 12:1404. doi: 10.3390/nu12051404
29. Kashyap, S, Shivakumar, N, Sejian, V, Deutz, NEP, Preston, T, Sreeman, S, et al. Goat milk protein digestibility in relation to intestinal function. Am J Clin Nutr. (2021) 113:845–53. doi: 10.1093/ajcn/nqaa400
30. Tagliazucchi, D, Martini, S, Shamsia, S, Helal, A, and Conte, A. Biological activities and peptidomic profile of in vitro-digested cow, camel, goat and sheep milk. Int Dairy J. (2018) 81:19–27. doi: 10.1016/j.idairyj.2018.01.014
31. Bhat, ZF, Morton, JD, el-Din, A, Bekhit, A, Kumar, S, and Bhat, HF. Processing technologies for improved digestibility of milk proteins. Trends Food Sci Technol. (2021) 118:1–16. doi: 10.1016/j.tifs.2021.09.017
32. Gaudichon, C, Bos, C, Morens, C, Petzke, KJ, Mariotti, F, Everwand, J, et al. Ileal losses of nitrogen and amino acids in humans and their importance to the assessment of amino acid requirements. Gastroenterology. (2002) 123:50–9. doi: 10.1053/gast.2002.34233
33. Gaudichon, C, Mahé, S, Benamouzig, R, Luengo, C, Fouillet, H, Daré, S, et al. Net postprandial utilization of [15N]-labeled milk protein nitrogen is influenced by diet composition in humans. J Nutr. (1999) 129:890–5. doi: 10.1093/jn/129.4.890
34. Tessier, R, Khodorova, N, Calvez, J, Tomé, D, and Gaudichon, C. Challenging in rats the use of 13C Spirulina as reference protein for the dual isotope method to determine amino acid bioavailability (P08-061-19). Curr Dev Nutr. (2019) 3:nzz044.P08-061-19. doi: 10.1093/cdn/nzz044.P08-061-19
35. Sobiecki, JG, Appleby, PN, Bradbury, KE, and Key, TJ. High compliance with dietary recommendations in a cohort of meat eaters, fish eaters, vegetarians, and vegans: results from the European prospective investigation into Cancer and nutrition–Oxford study. Nutr Res. (2016) 36:464–77. doi: 10.1016/j.nutres.2015.12.016
36. González, N, Marquès, M, Nadal, M, and Domingo, JL. Meat consumption: which are the current global risks? A review of recent (2010–2020) evidences. Food Res Int. (2020) 137:109341. doi: 10.1016/j.foodres.2020.109341
37. Gómez-Cortés, P, Juárez, M, and de la Fuente, MA. Milk fatty acids and potential health benefits: An updated vision. Trends Food Sci Technol. (2018) 81:1–9. doi: 10.1016/j.tifs.2018.08.014
38. Scintu, MF, and Piredda, G. Typicity and biodiversity of goat and sheep milk products. Small Rumin Res. (2007) 68:221–31. doi: 10.1016/j.smallrumres.2006.09.005
39. Schiffer, L, Barnard, L, Baranowski, ES, Gilligan, LC, Taylor, AE, Arlt, W, et al. Human steroid biosynthesis, metabolism and excretion are differentially reflected by serum and urine steroid metabolomes: a comprehensive review. J Steroid Biochem Mol Biol. (2019) 194:105439–9. doi: 10.1016/j.jsbmb.2019.105439
40. Varma, VR, Wang, Y, An, Y, Varma, S, Bilgel, M, Doshi, J, et al. Bile acid synthesis, modulation, and dementia: A metabolomic, transcriptomic, and pharmacoepidemiologic study. PLoS Med. (2021) 18:e1003615–5. doi: 10.1371/journal.pmed.1003615
Keywords: nutritional composition, growth performance, digestibility, metabolomics profiles, cow milk, goat milk, sheep milk, rat models
Citation: Yang C, Pan J, Pang S, Hu S, Liu M, Zhang X, Song L, Ren X and Wang Z (2024) Comparative analysis of the nutritional composition, digestibility, metabolomics profiles and growth influence of cow, goat and sheep milk powder diets in rat models. Front. Nutr. 11:1428938. doi: 10.3389/fnut.2024.1428938
Edited by:
Marija Takic, University of Belgrade, SerbiaReviewed by:
Lijun Chen, Beijing Sanyuan Foods Co., Ltd., ChinaJoshua Arimi, Meru University of Science and Technology, Kenya
Copyright © 2024 Yang, Pan, Pang, Hu, Liu, Zhang, Song, Ren and Wang. This is an open-access article distributed under the terms of the Creative Commons Attribution License (CC BY). The use, distribution or reproduction in other forums is permitted, provided the original author(s) and the copyright owner(s) are credited and that the original publication in this journal is cited, in accordance with accepted academic practice. No use, distribution or reproduction is permitted which does not comply with these terms.
*Correspondence: Xiangnan Ren, cmVueG5AbmluaC5jaGluYWNkYy5jbg==; Zhongli Wang, d2FuZ3pob25nbGlsb3ZlZEAxNjMuY29t
†These authors have contributed equally to this work