- 1Department of Neonatology, Affiliated Women’s Hospital of Jiangnan University, Wuxi Maternity and Child Health Care Hospital, Wuxi, China
- 2Department of Gastroenterology, The First Affiliated Hospital of Soochow University, Suzhou, China
- 3Reproductive Medicine Centre, Affiliated Women’s Hospital of Jiangnan University, Wuxi, China
- 4Department of Child Health Care, Affiliated Women’s Hospital of Jiangnan University, Wuxi, China
Digestive diseases are becoming an increasingly serious health burden, creating an urgent need to develop more effective treatment strategies. Probiotics and postbiotics have been extensively studied for their potential to prevent and treat digestive diseases. Growing evidence suggests that programmed cell death, especially apoptosis, is a critical mechanism influencing the molecular and biological aspects of digestive diseases, contributing to disease progression. Understanding the mechanisms and signaling pathways by which probiotics and postbiotics regulate apoptosis could reveal new therapeutic targets for treating digestive diseases. This review focuses on the beneficial effects of probiotics and postbiotics in regulating apoptosis across a range of liver diseases, including non-alcoholic fatty liver disease, liver injury, cirrhosis, and liver cancer. It also explores their effects on gastrointestinal diseases, such as colorectal cancer, colitis, gastrointestinal injury, and infectious diarrhea. Furthermore, some probiotics help balance the gut microbiota, enhance intestinal barrier function, and regulate the immune system, all of which are closely associated with apoptosis. Moreover, emerging technologies, such as encapsulation methods, have been developed to stabilize probiotics, primarily based on experimental findings from rodent and human studies.
1 Introduction
The age-standardized incidence of digestive diseases worldwide is 95,582 per 100,000, representing over one-third of the total prevalence of all diseases (1). Digestive disorders result in millions of medical visits and billions of dollars in economic costs annually in the United States (2). The prevalence of digestive diseases is rising globally, placing a significant burden on public health (3, 4). Despite significant advancements in medical devices and healthcare, many patients still experience poor quality of life and prognosis, highlighting the need for more treatment options. Recent studies have shown that both probiotics and postbiotics have gained significant attention for their potential to improve various digestive diseases by regulating host immune function, maintaining intestinal barrier integrity, and modulating the gut microbiota (5), as shown in Figure 1.
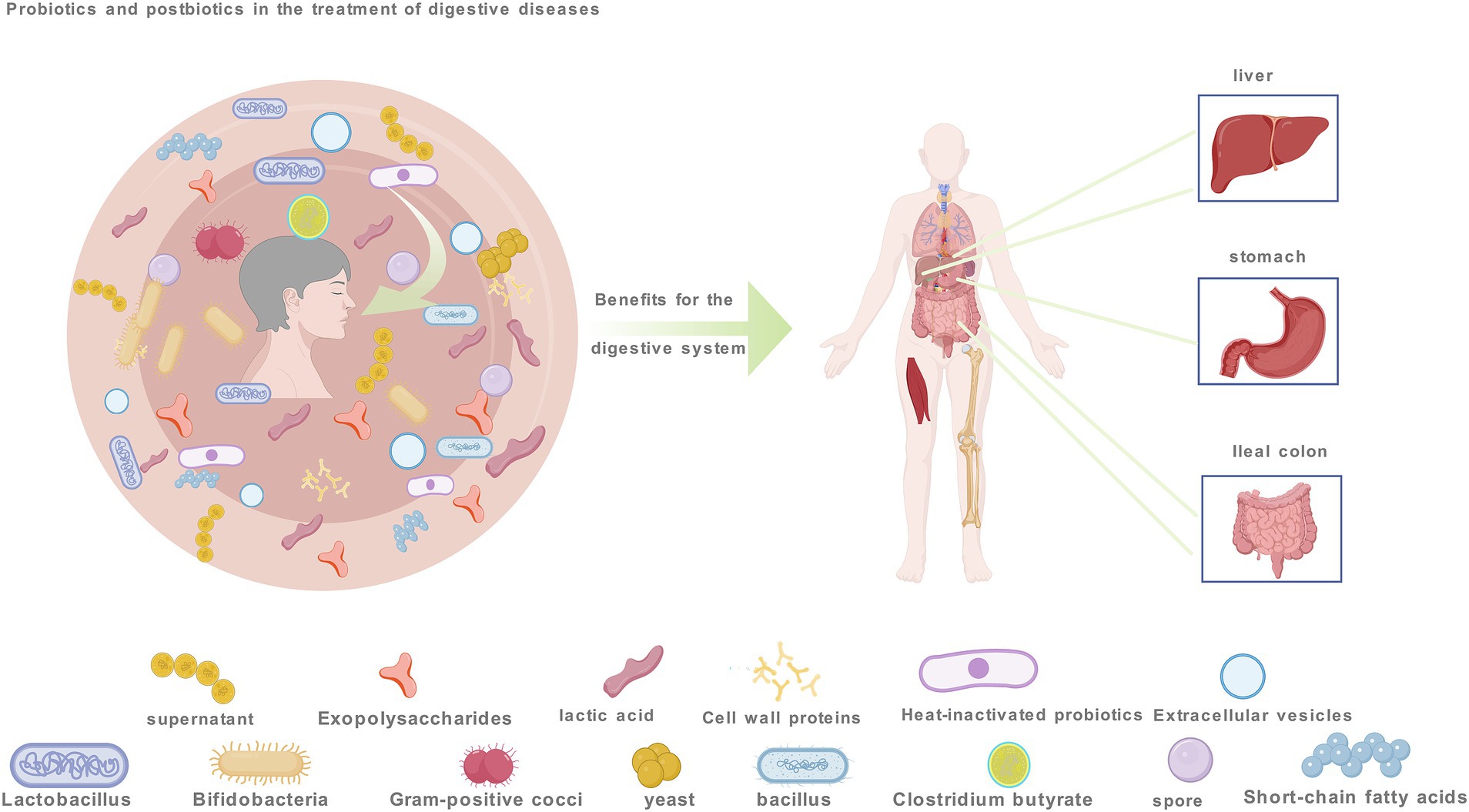
Figure 1. Probiotic and postbiotics aimed at improving gastrointestinal disorders by modulating apoptosis encompasses. These include probiotics like Lactobacillus and Bifidobacterium, Gram-positive cocci, yeast, Bacillus, Clostridium butyricum, postbiotics such as extracellular vesicles and lactic acid, spores and extracellular polysaccharides.
Apoptosis, or programmed cell death (6), is a crucial process that maintains tissue homeostasis by removing damaged or dysfunctional cells (7). However, its dysregulation is a critical factor in the development of digestive diseases (8–10). In inflammatory bowel disease, excessive apoptosis of intestinal epithelial cells disrupts the mucosal barrier, worsening inflammation and microbial translocation, while impaired apoptosis of immune cells prolongs chronic inflammation (11). In colorectal cancer, the promotion of apoptosis in cancer cells is driven by key genes such as P53, K-ras, and Bcl, which are associated with intrinsic apoptosis pathways. These mechanisms contribute to inhibiting tumor growth and reducing chemotherapy resistance (9, 12). In gastrointestinal infections, rotavirus enters mucosal epithelial cells via virulence factors such as sialic acid and histo-blood group antigens, triggering apoptosis. This process results in mucosal damage and delayed healing (13). These findings highlight the importance of targeting apoptotic pathways in developing therapeutic strategies for digestive diseases, with probiotics and postbiotics emerging as promising modulators.
Probiotics (it refers to active microorganisms that confer benefits to the host), either directly or through the secretion of metabolites or the regulation of host signaling pathways such as Bcl-2/Bax, caspase cascades, and key anticancer pathways, can inhibit excessive apoptosis or induce apoptosis in abnormal cells while protecting normal cells. These mechanisms contribute to alleviating pathological conditions, as seen with Lactobacillus plantarum, Lactobacillus rhamnosus, and others (9, 14, 15). Postbiotics (it is a collective term for the metabolic components of probiotics after processing), as functional metabolites of probiotics, can directly or indirectly target key nodes in the apoptosis process, including short-chain fatty acids, heat-killed probiotics, etc. (15, 16). How do probiotics and postbiotics regulate apoptosis in different types of gastrointestinal diseases?
2 Probiotics, postbiotics, and apoptosis
Apoptosis is a crucial mechanism in the growth and development of multicellular organisms (7). The exogenous pathway, mediated by membrane receptors and regulated by Bcl-2 family proteins, and the endogenous pathway, mediated by mitochondria, are both closely linked to caspase regulation (17). When a cell detects internal abnormalities, it activates an intrinsic apoptosis program, initiating endogenous apoptosis. This process involves Bcl family proteins (pro-apoptotic members such as Bax, Bak, Bim, Bid, and PUMA, and anti-apoptotic members such as Bcl-2, Bcl-xl, Bcl-w, and MCL1) which alter the permeability of the mitochondrial outer membrane (18–22). This results in the release of cytochrome c, formation of the apoptosome with APAF1, and activation of caspase-9, which in turn activates apoptotic executor proteins caspase-3, -6, and -7, ultimately leading to apoptosis (23). The regulation of Bcl-2 protein transcription and phosphorylation in apoptosis involves CDK and p53 (24). The ERK1/2 and MAPK1 pathways promote cell survival partly by phosphorylating BIM, leading to its proteasomal degradation and inhibition of apoptosis (25). Probiotics are live, non-pathogenic microorganisms (26). This paper typically contains one or more microbial strains, with the main components including Lactobacillus spp., Bifidobacterium spp., Gram-positive cocci spp., yeast spp., Bacillus spp., and Clostridium butyricum spp. This paper highlights that most probiotics regulate apoptosis through intrinsic pathways, influencing the expression of Bcl family proteins, caspases, cytochrome c, mitochondria, and key anticancer pathways, such as the EGFR/PI3K/AKT signaling cascade, mTOR pathway, P38/JNK pathway, TLR4/JNK/NF-κB pathway, TLR4/MAPK pathway, Wnt/β-catenin pathway, and cAMP-dependent signaling pathway, as shown in Figure 2. When a cell receives an external death signal, exogenous apoptosis is triggered through a cascade of reactions. This process involves the binding of ligands (FASL, TNF-a, TRAIL) (27–31) to their corresponding receptors (FAS, TNFRs, TRAILRS) (23, 32–34) followed by a cascade of reactions that activate the cleavage of caspase-8 and -10. Subsequently, the cleavage of apoptosis executor proteins caspase-3, -6, -7 and BID is triggered, ultimately leading to apoptosis (35). BID serves as a link between the exogenous apoptosis pathway and the mitochondrial pathway (36), while the activation of promoter caspases is negatively regulated by c-FLIP (37). Probiotics observed in liver and colon cancer have been found to induce apoptosis through core apoptotic pathways similar to antitumor drugs, involving AKT, RAS, Raf, MEK, ERK, and mTOR kinase signaling pathways (38–42), and inhibiting growth factor receptors such as EGFR, Her2/Neu, other ERBB family members, c-Met, and NTRK (43–48), as depicted in Figure 2. Some probiotics regulate apoptosis through both endogenous and exogenous pathways, such as L. plantarum C88, including the toll-like receptor signaling pathway. Postbiotics refer to “inanimate microorganisms and/or their components that are beneficial to host health” (49), including heat-killed bacteria, extracts of extracellular polysaccharides (EPS) (50), extracellular vesicles (EVs) (51), cell wall protein components (52), spores (53), short-chain fatty acids (SCFAs) (9), lactic acid (54) and others, as depicted in Figure 1. They regulate multiple core signaling pathways associated with growth and development, control the production and function of apoptosis factors, reduce apoptosis in healthy cells, selectively promote apoptosis in cancer cells, and contribute to overall body health. Compared with active probiotics, postbiotics, with their microbial composition similar to pharmacological molecules, offer advantages in absorption, metabolism, and excretion (55), making them more reliable dietary supplements, such as heat-killed yeast (HKY) (56), and EVs of L. rhamnosus PTCC1637 (51). The regulation of apoptosis may be intricately linked to certain bacterial components. Advancements in science and technology have facilitated the mass production of probiotics, such as the engineering of bacteria (e.g., butyrate synthesized by E. coli Nissle 1917). Furthermore, nanomaterials and microgels significantly enhance the precision of anti-cancer targeting of probiotics (57). As shown in Figure 2, probiotic and postbiotics regulate apoptosis to alleviate digestive diseases through both intrinsic and extrinsic pathways, promoting cancer cell apoptosis by targeting tumor-associated signaling pathways and key molecular targets.
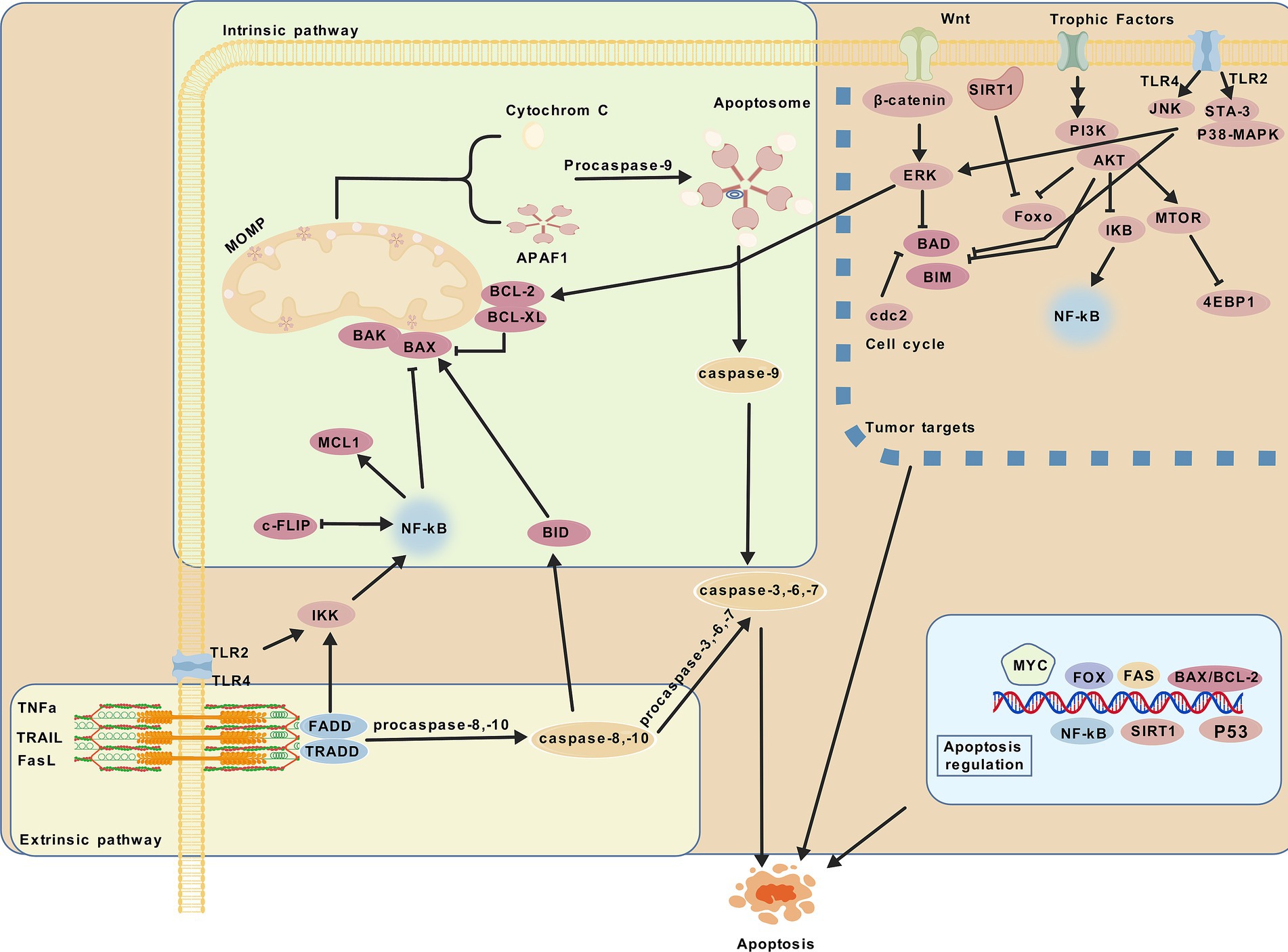
Figure 2. Modulation of apoptosis. Probiotic and postbiotics modulate apoptosis through extrinsic and intrinsic pathways, effectively influencing digestive diseases and targeting key pathways in tumors to promote cancer cell apoptosis.
3 Probiotics, postbiotics, and liver disease
3.1 Non-alcoholic fatty liver disease and liver injury
Non-alcoholic fatty liver disease (NAFLD) is a metabolic liver disorder unrelated to excessive alcohol consumption and is the most common chronic liver disease in Western countries (58). Recent studies have emphasized the important role of probiotics in treating and preventing liver diseases by modulating gut microbiota, strengthening the intestinal barrier, and interacting with the gut-liver axis (the gut-liver axis refers to the complex interplay between the gut and the liver, where gut microbiota-derived metabolites and immune-digestive interactions influence liver function, while liver-derived metabolites, in turn, regulate the gut microbiota and enhance intestinal barrier function) (59, 60). Apoptosis plays a crucial role (61). In a mouse model of NAFLD, L. johnsonii BS15 was found to prevent the disease by regulating gut microbiota and modulating immunity. The anti-apoptotic effect was linked to reduced cytochrome c content and increased uncoupling protein-2 levels in mice (62). Lc. paracasei HY7207 significantly alleviated symptoms and improved serum markers in mice with NAFLD. Additionally, the expression of Bcl-2 and Bax genes and their ratios were reduced, thereby decreasing apoptosis in human hepatocytes (8). As shown in Table 1, Lactobacillus spp. as dietary supplements, hold great potential for reducing endogenous apoptosis in the prevention and treatment of NAFLD. However, further studies are needed to determine whether they have similar effects in alcoholic fatty liver disease.
The liver, a vital metabolic organ, can be damaged by various factors, including infection, chemical toxicity, immune dysfunction, poor nutrition, circulatory issues, and genetic abnormalities (63). Supplementing with specific probiotics can alleviate the outcomes of autoimmune diseases by modulating immune responses and gut microbiota composition. In a liver injury model in lupus-prone mice, L. paracasei GMNL-32, L. reuteri GMNL-89, and L. reuteri GMNL-263 were found to downregulate liver cell apoptosis and inflammation-related marker expression. This is closely linked to the inhibition of the MAPK and NF-kB signaling pathways, with the inhibition of the IKK/NF-κB pathway downregulating caspase-3 expression to reduce liver cell apoptosis, providing a basis for these preparations to serve as alternative drugs for liver diseases in systemic lupus erythematosus (64). Furthermore, the culture supernatant of L. reuteri ZJ617 attenuates lipopolysaccharide (LPS)-induced acute liver injury by strengthening intestinal barrier integrity, modulating inflammatory responses, suppressing the hepatic TLR4/MAPK/NF-κB signaling pathway, and facilitating Beclin1-dependent autophagy. The anti-apoptotic effect, linked to downregulation of caspase-3 and Bax proteins, may result from suppression of the TLR4/MAPK signaling pathway (65). This suggests that different probiotics and postbiotics may alleviate liver injury through intersecting signaling pathways. However, further research is needed to determine whether the specific components involved are identical.
The NLRP3 inflammasome is a key component of the innate immune system (66). Bacillus amyloliquefaciens SC06 significantly inhibits the NLRP3 inflammasome and markedly reduces hepatocyte apoptosis (67). Bacillus amyloliquefaciens B10 significantly reverses the gene and protein expression of Bax, Bcl-2, and Caspase-3 induced by aflatoxin B1, but lacks exploration of the underlying molecular mechanisms (68). L. plantarum C88 has been shown to possess beneficial properties such as improving intestinal barrier function and inhibiting inflammation. It inhibited inflammation and excessive cell apoptosis mediated by the TLR2/NF-κB and TLR4/NF-κB signaling pathways, involving regulation of cell death receptors and mitochondrial pathways (14). These studies suggest a close correlation between using live Lactobacillus spp. or their supernatants to treat liver injury, enhance intestinal barrier function, and inhibit inflammation. Bacillus species present new insights into the mechanism of alleviating acute liver injury, particularly regarding apoptosis.
As shown in Table 1, live Lactobacillus spp. or their supernatants primarily affect the NF-κB and MAPK signaling pathways, reducing both endogenous and exogenous hepatocyte apoptosis. Further research is needed to explore the characteristics of other probiotics used for liver injury and their potential effects on apoptosis inhibition.
3.2 Liver cirrhosis and liver cancer
Globally, liver disease causes 40,000 deaths annually, representing 1% of global mortality (2,019 out of every 25 deaths worldwide). Hepatitis, alcoholic and non-alcoholic fatty liver disease, and chronic liver damage are closely associated with cirrhosis development. Further progression can lead to liver cancer, significantly burdening patients’ lives (69). The efficacy of chemotherapy should not overshadow its harmful effects on healthy cells. Probiotics and postbiotics have recently emerged as promising interventions for preventing and treating cirrhosis and liver cancer, and mitigating disease complications. In treating Schistosoma mansoni-induced cirrhosis, oral administration of probiotics such as L. acidophilus ATCC 4356 and L. delbrueckii subsp. bulgaricus DSM 20080, or their fermented yogurt, significantly reduce worm burden, egg production, and granuloma size and number in mouse liver tissue. Significant improvements in oxidative stress and liver fibrosis were observed, along with downregulation of caspase-3 and Bax/Bcl-2 expression (70). However, oral administration of EPS derived from L. acidophilus ATCC 4356 in hepatocellular carcinoma rats induced by diethylnitrosamine and gamma radiation exhibited potent immunomodulatory effects. This effect is attributed to the inhibition of the TLR2/STAT-3/P38-MAPK pathway related to inflammation; However, the specific mechanism of apoptosis regulation remains unclear (50). Initial findings suggest that probiotics modulate intestinal flora, reduce toxic metabolite accumulation, and influence the progression of metabolic disorders, including diabetes and insulin resistance. Recent studies demonstrate that adding an emulsion containing L. rhamnosus NCIMB 8010 and Pediococcus acidilactici NCIMB 8018 to liver cancer cells mitigates insulin resistance induced by free fatty acid accumulation, enhances cell viability, and regulates the Bax/Bcl-2 and caspase axes to improve mitochondrial dysfunction. The mechanism may involve inhibition of the fetal protein/TLR4/JNK/NF-κB pathway (71). In vitro experiments with the human liver cancer cell line HepG2 showed that EVs derived from L. rhamnosus PTCC 1637 prevent liver cancer and significantly increase the apoptosis index (Bax/Bcl-2 ratio), inducing cancer cell death (51). Further research is required to elucidate the mechanisms underlying extracellular vesicle and probiotic-host interactions. These findings demonstrate that Lactobacillus and its components, such as extracellular vesicles and polysaccharides, primarily regulate endogenous apoptosis through the Bax/Bcl-2 and caspase axis. These components are promising alternative therapies for preventing and treating liver cancer, as shown in Table 2. As a critical step in the progression of liver cancer, the significance of cirrhosis should not be overlooked while exploring probiotic strains and molecular mechanisms involved in liver cancer.
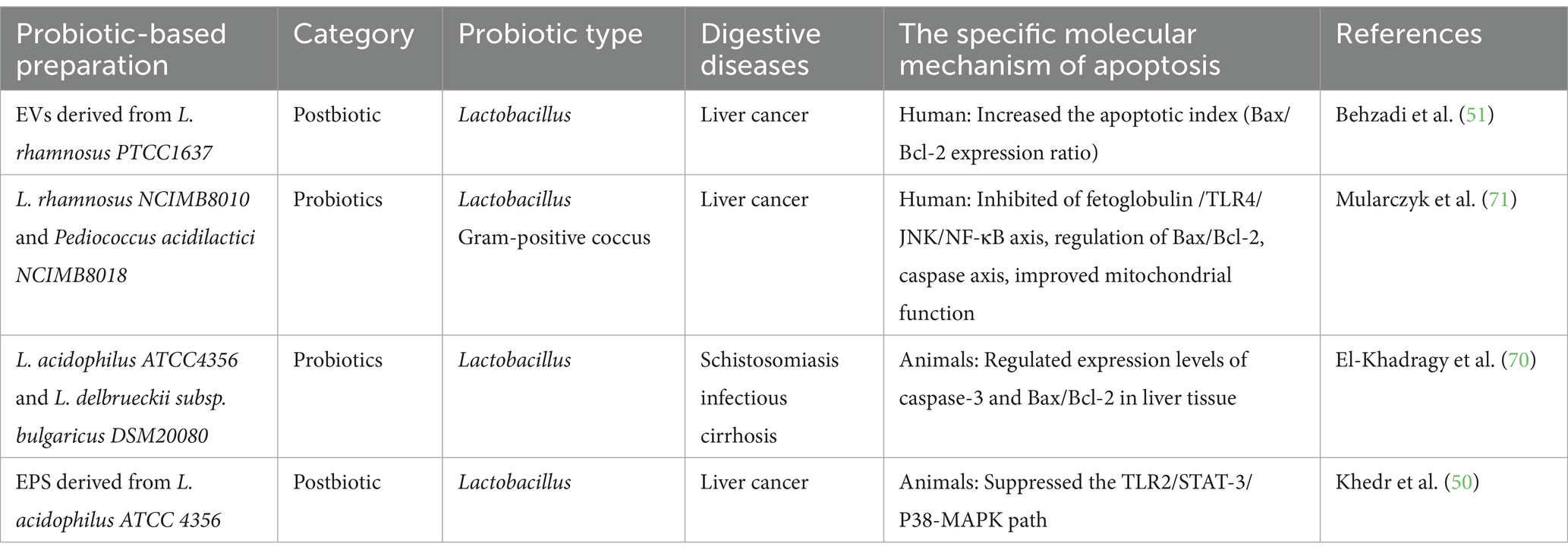
Table 2. Active probiotics and postbiotic regulate apoptosis in the treatment of liver cirrhosis and liver cancer.
4 Probiotics, postbiotics, and colitis
The global prevalence of ulcerative colitis is rising, significantly affecting quality of life [not only affects the digestive system but also influences periodontitis (72)]. Effective treatment aims to induce and maintain remission (73). Current treatments include aminosalicylates, corticosteroids, antibiotics, adjunctive medications, and immunosuppressive agents; however, their efficacy is often suboptimal and tolerability limited. Exploring alternative treatments is essential (74). The ability of probiotics to regulate gut microflora, enhance the intestinal mucosal barrier, and improve immune function has positioned them as potential biological agents for treating colitis (75). In a Dextran Sulfate Sodium Salt (DSS)-induced mouse colitis model, the supernatant of Lactobacillus GG-fermented milk inhibited intestinal epithelial cell apoptosis, potentially by activating the PI3K/Akt pathway, which upregulates the anti-apoptotic protein Bad and inhibits FOXO transcription factors. This effect is linked to the unique p40 and p75 proteins in the probiotic-fermented milk supernatant (76). A pectin/zein hydrogel bead system improves protein delivery stability (77). Preliminary studies show that tumor necrosis factor-α (TNF-α) mediates inflammatory responses in inflammatory bowel disease. Its apoptotic role makes it a key target for destroying intestinal epithelial cells and a prime focus of pharmacotherapies. Oral administration of L. BB12 and L. plantarum LB-9 downregulates TNF-α expression and modulates caspase-8-mediated extrinsic apoptosis (78, 79). Secreted factors from B. bifidum infantum 15697 reduce infections in a mouse model of necrotizing enterocolitis, prevent weight loss, and mitigate apoptosis caused by caspase-3 and caspase-7 activation, likely through NF-κB pathway inhibition (80). Combining probiotics with nanomaterials provides additional benefits, such as prolonged circulation and intestinal immunity regulation (81); Bacillus amyloliquefaciens-loaded nanoparticles enhance stability and improve endogenous apoptosis compared to free Bacillus. Specifically, They downregulate caspase-3 and cytochrome c expression while upregulating Bcl-2 and Bax, suggesting nanotechnology offers promising avenues for the food industry (82), suggesting that nanotechnology offers promising avenues for the food industry. These studies show that modulating the PI3K/Akt signaling pathway, regulating the TNF-α-mediated death receptor pathway, and inhibiting apoptosis through the Bcl-2 family and CytC-mediated mitochondrial pathway are key to the preventive and therapeutic effects of certain probiotics in colitis. Exploring novel probiotics and technologies that combine them with specific substances has further enhanced their potential benefits. As shown in Table 3, probiotics may serve as promising targeted therapeutic supplements. However, most studies still require further exploration of these novel formulations and more in-depth investigations into their molecular mechanisms, such as Limosilactobacillus reuteri FN041 (83).
5 Probiotics, postbiotics, and colorectal cancer
The prevalence of colon cancer has significantly increased in recent years due to poor dietary choices and unhealthy lifestyles, making it the second leading cause of cancer-related mortality worldwide (84). Recent studies suggest that probiotic preparations (it refers to probiotics and postbiotics) offer advantages over traditional drugs in certain cancer treatments and their side effects. When used as adjuvant therapy, probiotics can mitigate toxic side effects, optimize therapeutic outcomes, and enhance gut microflora, intestinal barrier function, and immune response (85). Inducing apoptosis in cancer cells is a key goal in advancing cancer treatments (86). A study using S. cerevisiae in a mouse model of colorectal tumors to inhibit cancer progression suggests that the downregulation of the Akt/NF-κB and Akt/mTOR signaling pathways may be linked to apoptosis in cancer cells. It also suggests a potential increase in beneficial gut microbiota and immune function regulation (87). Identifying specific S. cerevisiae strains with anti-colon cancer properties is crucial, as different strains have distinct therapeutic benefits (88). The L. paracasei K5 strain adheres to human intestinal cancer cells, potentially inducing apoptosis by regulating Bcl-2 family proteins (89). Administration of L. casei ATCC393 suppresses tumor growth and enhances apoptosis in mouse (CT26) and human (HT29) colon cancer cells by upregulating TRAIL expression and downregulating Survivin (90). The link between cell cycle regulation and apoptosis induction is well established (91). L. paracasei subsp. paracasei X12 inhibits the mTOR/4EBP1 signaling pathway, induces G1 phase arrest in HT-29 cells, suppresses cyclin E1 expression, upregulates p27, and modulates apoptosis (92). The cell wall protein component of L. paracasei ATCC25598 has been shown to mitigate apoptosis in the human intestinal Caco-2 cell line (52). This highlights the specificity of strains in regulating apoptosis mechanisms. Long-term retention of probiotics in the gut may improve disease prognosis, including cancer. L. plantaris, L. rhamnosus, L. breve, and L. luciferi extracted from human feces have anti-cancer effects by activating the Wnt/β-catenin pathway, antimicrobial peptides, and metabolites. These metabolites disrupt mitochondrial membrane integrity and trigger late apoptosis in tumors following colonization (93). Promoting the long-term presence of probiotics in the gut may improve disease outcomes, such as cancer prognosis. Similarly, a comparable study found that L. salivarius CGMCC3606 inhibits both early and late tumor formation in mice. Notably, its metabolites effectively suppress the AKT signaling pathway by inhibiting phosphorylation of AKT, cyclin D1, and COX-2, leading to apoptosis (94). But further studies are needed to understand how Saccharomyces burra metabolites promote cell apoptosis (95). These findings suggest that a single active Lactobacillus and its metabolites can induce apoptosis in cancer cells through various signaling pathways, offering promising prospects for clinical applications. Cancer development and progression are closely linked to chronic inflammation, a major contributing factor (96). L. helveticus NS8 significantly reduces tumor number and proliferation in colitis-associated colorectal cancer mice, while promoting the increase of beneficial microbiota. Upregulating caspase-3 to promote apoptosis may involve inhibiting NF-κB activation and modulating inflammatory factors (97). Recently, there has been increasing focus on the immune function and molecular mechanism of non-viable probiotics rendered inactive by physical or chemical methods, such as heat and ultraviolet radiation, in relation to diseases (49). Notably, it possesses greater stability compared to live probiotics, making it highly advantageous as a food additive (98). Compared to 5-FU, heat-inactivated S. cerevisiae PTCC5052 downregulates p-Akt1, Bcl-XL, Rel A, pro-caspase-3, and − 9, and enhances Bax and caspase-3 expression to induce cell apoptosis. The former has a more pronounced effect on Bax regulation through the Akt/NF-κB signaling pathway (56). Heat-induced apoptosis of human colorectal adenocarcinoma HT-29 cells depends on factors like time, dose, and specific strains, such as L. brevis IBRC_M1078 and L. paracasei IBRC_M1079. These probiotic strains promote apoptosis by enhancing the expression of pro-apoptotic genes like Bax, caspase-3, and caspase-8, while suppressing the anti-apoptotic gene Bcl-2 (99). The purification of probiotics and the use of their cell-free supernatant should not be overlooked (16). The cell wall protein component of L. paracasei ATCC25598, for example, induces apoptosis in intestinal Caco-2 cells and may serve as an anticarcinogenic agent (52). The effect of Lactobacillus cell-free supernatant (LCFS) on apoptosis induction in human colon cancer cells was observed in a 3D colorectal cancer model, where it inhibited NF-κB activation and downregulated PARP1 and Bcl-XL expression (100). This offers a novel approach to investigating the anticancer properties of probiotics across various cancer types. With many chemotherapy drugs used in tumor treatment, drug resistance has emerged as a major factor contributing to the decline in drug efficacy. Recently, the use of probiotic components and metabolites has been found to effectively mitigate this issue. For example, L. plantarum CCARM0067, which produces γ-aminobutyric acid (GABA), shows anti-cancer effects on 5-fluorouracil-resistant human colorectal adenocarcinoma cells by inducing apoptosis through cIAP2 regulation and inhibition of the cAMP-dependent signaling pathway (15). The cell-free culture supernatant of this strain boosts the cancer-inhibiting effects of SMCT1/butyric acid in colorectal cancer cells, making it a potential chemotherapy enhancer for HCT116 cells resistant to 5-Fluorouracil and butyric acid. It is also closely linked to the activity pattern of caspase-3 (101). These findings offer a novel approach to chemoprevention and treatment of colorectal cancer-related diseases.
Various probiotic mixtures regulate apoptosis to improve disease outcomes. L. plantarum AdF10 and L. rhamnosus ATCC53103 enhance oxidative stress resistance and normalize p53-mediated apoptosis gene expression, potentially safeguarding against stress-induced excessive apoptosis. This may improve cellular health and reduce diseases associated with uncontrolled apoptosis (102). Celecoxib suppresses the AKT pathway, leading to decreased CD133 expression in colon cancer (103). The combination of L. acidophilus NCDC15 and L. rhamnosus GG MTCC1408, along with celecoxib, was observed to decrease tumor heterogeneity and enhance immune function and gut health. It upregulates P53 expression, downregulates K-ras proto-oncogene expression, and modulates Bax and Bcl-2 levels, potentially inhibiting tumor growth and promoting overall health (12). This suggests that the molecular mechanism of apoptosis underlying the effects of chemotherapy may be altered when combined with probiotics. This combined approach may help alleviate the severity and burden of diseases in highly susceptible individuals. However, clinical validation is required.
Recent research shows that SCFAs, such as acetate, propionate, and butyrate, act as metabolites for gut bacteria to metabolize dietary fiber. These metabolites play crucial roles in inflammation, immunity, lipid metabolism, apoptosis mechanisms, and the regulation of key targets related to disease prevention and outcomes (104). To mitigate rapid clearance and enhance bioavailability, novel short-chain fatty acid analogs (105) and probiotics combined with nanomaterials (106) have been developed. The application of engineered bacteria is highly promising. Engineered E. coli Nissle 1917 with synthetic butyrate reduced tumor volume by 70% in mice and induced apoptosis in human colorectal cancer cells through the mitochondrial pathway, independent of P53. This represents a novel approach to targeted bacterial cancer therapy (107). The latest study on microcapsules enables probiotics to exert targeted tumor therapy (108). Microencapsulated L. plantarum LAB12 significantly reduces tumor volume and weight, inhibiting angiogenesis. The anti-apoptotic effect is partially linked to upregulation of p53 and caspase-3 expression (109). In recent years, L. reuteri has shown great promise in the treatment of various digestive diseases (110). The use of L. reuteri delivered via microgel technology in colorectal cancer enhances beneficial bacterial flora, increases butyrate production, and modulates the caspase and Bcl pathways to induce apoptosis in human colorectal cancer cells (57). This technology also enhances the gastrointestinal tolerance of L. reuteri. Lactobacillus paracasei strain CMU-Pb-L5 and L. reuteri promote cancer cell apoptosis through similar mechanisms. Future research should focus on a more detailed investigation of the specific bacterial components involved in tumor growth inhibition (111). Acetyl-ethyl extract from L. plantarum ATCC14917 and L. rhamnosus ATCC7469 exhibits targeted anti-colon cancer cell activity by inducing the intrinsic apoptosis pathway, downregulating the expression of anti-apoptotic genes Bcl-2 and Bcl-XL, and upregulating the expression of pro-apoptotic genes Bak, Bad, and Bax (9). It is a potential candidate for aiding in the fight against cancer from a nutritional perspective. The studies indicate similarities in the targeted anti-cancer mechanisms of short-chain fatty acids derived from Lactobacillus, particularly in their ability to predominantly activate the intrinsic pathway to induce apoptosis in cancer cells. Compared with healthy individuals, intestinal conjugated linoleic acid (CLA) is significantly reduced in CRC patients. Besides fecal microbiota transplantation (FMT) (112), exogenous supplementation with CLA-producing Bifidobacterium breve CCFM683 and Bifidobacterium pseudocatenulatum MY40C significantly inhibits tumor progression, which is closely associated with CLA and the bbi gene responsible for its production. CCFM683 enhances intestinal barrier function by suppressing the NF-κB signaling pathway and promotes tumor cell apoptosis through the CLA-PPAR-γ axis (113), a mechanism consistent with the tumor-suppressive effects previously observed in L. plantarum CCFM8661 (114).
Recent studies have found that new probiotics are effective in colorectal cancer. The novel probiotic strains Streptococcus salivarius CP163 and S. salivarius CP208, originating from human colostrum, demonstrate multifaceted anti-cancer properties. These include directly adhering to cancer cells, secreting short-chain fatty acids, inducing cancer cell DNA fragmentation and morphological alterations, modulating caspase-2 activity, and triggering apoptosis. This study unveils an innovative biological strategy for using functional foods in colon cancer prevention (115). Additionally, in vitro experiments have shown that Ligilactobacillus salivarius LZZAY01 promotes cancer cell apoptosis (116). In summary, as shown in Table 4, probiotics or postbiotic play a crucial role in exerting anti-colorectal cancer effects through various signaling pathways related to tumorigenesis or by directly promoting the expression of proteins involved in cell apoptosis. Compared to conventional chemotherapeutic agents, microbial preparations have minimal or absent toxic side effects, providing them with a significant advantage in biological applications. The development of novel technologies, such as microencapsulation and nanomaterials, has further enhanced the stability and tumor specificity of these microbial preparations, which are used as nutritional dietary supplements for colorectal cancer prevention and treatment. Therefore, due to their excellent gastrointestinal tolerance and biological safety profiles, these microbial preparations are expected to have broader clinical applications.
6 Probiotics, postbiotic, and gastrointestinal damage
Encountering harmful substances and microorganisms is inevitable in daily life. When the immune system weakens, direct or indirect contact with the gastrointestinal damage system can the intestinal barrier, leading to microbiota imbalance, intestinal damage, and diarrhea. Historically, gastrointestinal injuries were mainly treated with pharmaceuticals and surgery; recent studies have shown that probiotics can offer protection. Maintaining the intestinal barrier function is crucial for gastrointestinal health, as shown in various studies (117). An in vitro study showed that Clostridium tyrobutyricum protects porcine epithelial cells from lipopolysaccharide-induced injury by preserving intestinal barrier function and inhibiting the P38/JNK signaling pathway. Downstream genes, including AP-1, ELK-1, ATF-2, and p53, are downregulated, while Stat3 activates anti-apoptotic Bcl-2 and downregulates pro-apoptotic Bax and caspase-3/-8, reducing intestinal cell apoptosis (118). Ochratoxin A, a significant toxin for humans and animals, has been detected in in vitro models of Ochratoxin A-induced cell injury. Bacillus subtilis CW14 upregulated DNA repair genes and downregulated death receptor pathway genes to reduce apoptosis. These effects may be mediated by activation of toll-like receptor signaling pathways (119). The specific mechanism requires further investigation. Cadmium, a heavy metal, can damage various bodily systems through long-term environmental exposure, with the gastrointestinal tract being the primary target organ (120, 121); Administration of multi-strain probiotics (L. rhamnosus IRBC_M10783, L. helveticus TG_34, Lactobacillus casei IRBC_M10782) significantly reduces intestinal tissue damage in mice compared to untreated controls. Probiotics modulate immune function by upregulating p53, Bax, and caspase-3, while downregulating Bcl-2 to reduce apoptosis (122). Similarly, Lactobacillus rhamnosus, Lactobacillus fermentum, and Lactobacillus brevis inhibit indomethacin-induced mucosal cell apoptosis. Future research should focus on a more detailed investigation of the molecular mechanisms underlying the probiotic effects (123).
Research has shown that lactic acid, a Lactobacillus metabolite, mitigates ethanol-induced gastric mucosal damage by reducing local inflammation. It protects the stomach by inducing apoptosis through downregulation of IL-1β, TNF-α, and IL-6, as well as Bax and caspase-3, and by upregulating genes maintaining gastric mucosal integrity (10). However, the underlying mechanism remains unclear, and further research is needed to explore additional applications of probiotics in this field (54). Radiotherapy is a common and effective treatment for gastrointestinal tumors, but it inevitably damages adjacent healthy tissue (124). Restoring gut microbiota can partially alleviate this damage through fecal transplantation and probiotic therapy. The key is finding ways to mitigate the impact of stomach acid and ionizing radiation on the microbiota (125, 126). A recent study on probiotic spore layers (spore ghosts) showed that oral administration of three clinically approved probiotics (Bacillus coagulans, Bacillus subtilis, and Bacillus licheniformis) significantly enhanced the population of beneficial intestinal flora in mice. This effect was attributed to their exceptional stomach acid tolerance and biocompatibility. They also improved intestinal barrier function and reduced radiation-induced apoptosis in intestinal epithelial cells (53). These studies collectively indicate that apoptosis is a key mechanism through which various probiotics and their metabolites to prevent and treat gastrointestinal injury. Certain forms, such as spores, enhance gastrointestinal tolerance and play a crucial role in maintaining the integrity of the gastrointestinal barrier. Consequently, they hold promising potential as candidates for addressing gastrointestinal injury, as illustrated in Table 5. However, further research is needed to explore the use of additional probiotics, particularly through clinical trials.
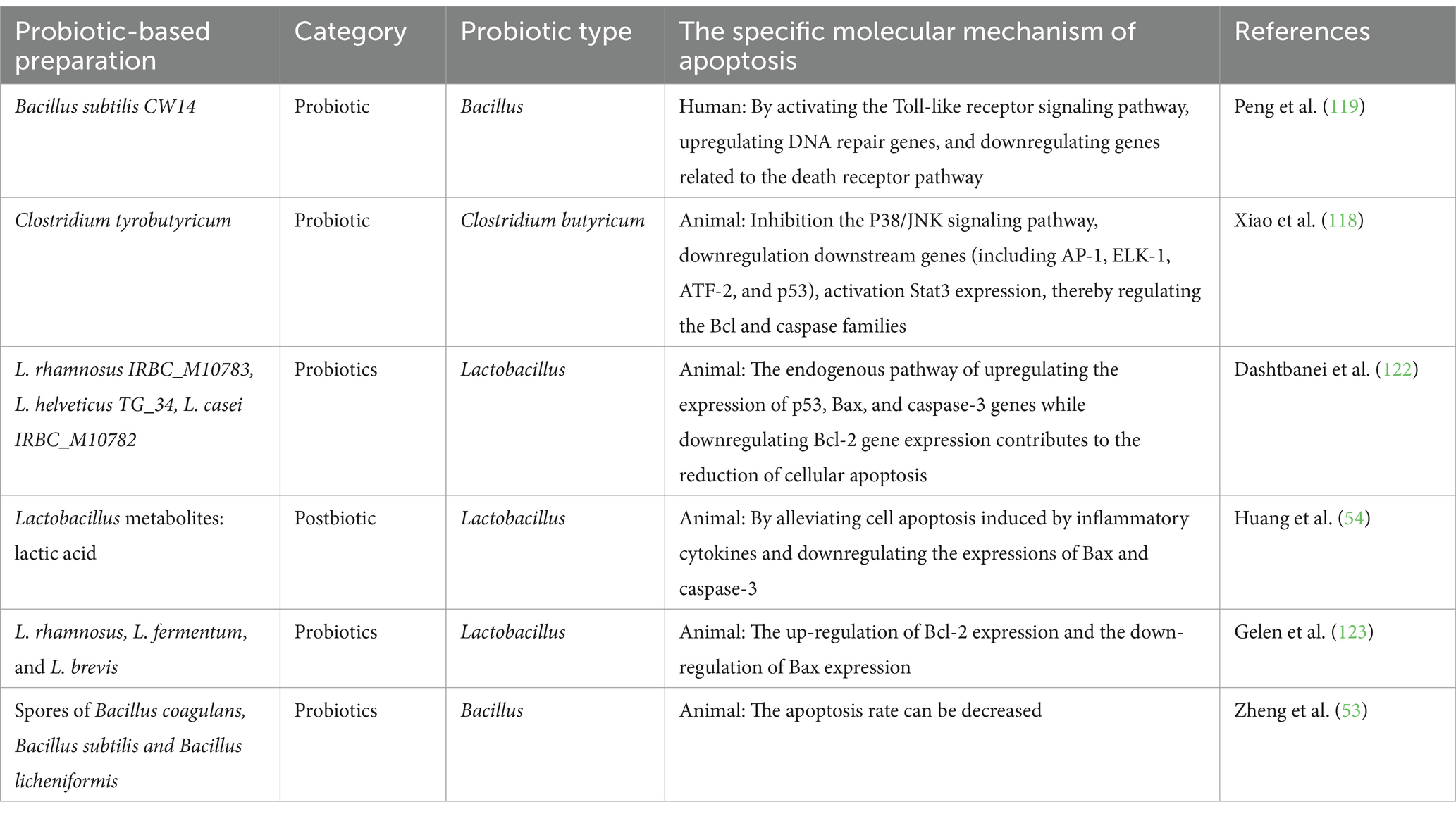
Table 5. Probiotics and postbiotic regulate endogenous and exogenous cell apoptosis to alleviate gastrointestinal injury.
7 Probiotics, postbiotic, and infectious diarrhea
In the United States, acute diarrheal diseases cause approximately 179 million outpatient visits annually (127). Probiotics and postbiotics, along with rehydration, medication, and improved hygiene, offer a promising approach for controlling early-stage gastrointestinal infections, providing a significant solution to this global health issue (128, 129). They can significantly modulate gut microbiota, enhance intestinal barrier function, and regulate immune responses, thereby exerting probiotic properties to alleviate diarrhea. Rotavirus is widely recognized as the leading cause of severe gastrointestinal infections in infants and children (130). In an experimental study with viral intervention in weaning piglets, LGG was found to significantly alleviate diarrhea caused by viral infection, upregulate the Bcl-2 gene, and downregulate the Bax gene, reducing apoptosis of jejunal mucosal cells (131). A recent in vitro study using a human model showed that both LGG and its conditioned medium (mLGG) reduced elevated caspase-3 activity, potentially through inhibition of the NF-κB signaling pathway. Their benefits differ: live LGG primarily suppresses enterotoxic and cytotoxic effects, while mLGG exerts postbiotic effects mainly by inhibiting chloride ion secretion pathways (129). The findings suggest that the mechanism through which LGG inhibits apoptosis and alleviates virus-induced diarrhea may be species-specific. Additionally, the addition of Bacillus clausii mixed strains (O/C, T, SIN, N/R) and their supernatant reduced intestinal cell apoptosis rates. This mechanism may involve activation of the cellular TLR3 pathway and suppression of NF-κB1, TRAF6, and MyD88. These findings encourage further exploration of the effects of Bacillus clausii on gastrointestinal infections caused by other pathogens in future studies (132). The 3D8 single-chain variable region protein shows potential antiviral activity by penetrating cells and degrading nucleic acids. Oral administration of L. paracasei ATCC334, which produces recombinant 3D8 single-chain variable region protein, reduces norovirus coat protein VP1 expression and increases the expression of the anti-apoptotic protein survivin in mice (133). Salmonella typhimurium is a prevalent foodborne pathogen, while Bacillus subtilis Gbi-30, Bacillus indium ATCC6633, and Bacillus coagulans IBRC-M10981 inhibit its growth in spore and heat-inactivated forms without toxic effects on intestinal cells. These strains are recommended for the prevention and treatment of disease (134). These studies show that probiotics and postbiotics can reduce gastrointestinal cell apoptosis induced by viral or bacterial infections, as shown in Table 6. This effect is likely due to their anti-inflammatory properties, maintenance of intestinal barrier function, and direct pathogen-targeting mechanisms. However, the precise molecular mechanism through which probiotics reduce gastrointestinal cell apoptosis following viral infection remains unclear. Future research should explore the effects and mechanisms of different probiotic strains on various pathogens, such as Helicobacter pylori-induced gastrointestinal infections, and their impact on intestinal cell apoptosis.
8 Probiotic, postbiotic, and safety
Probiotics and postbiotics offer numerous health benefits; however, their potential adverse effects in specific conditions should not be overlooked. First, individuals with underlying diseases and immunocompromised conditions may be at risk of infections when consuming probiotics, particularly those with weakened immune systems, such as chemotherapy patients, pediatric patients, and individuals with HIV (135). In some cases, commercially available probiotics have been associated with infections, such as Lactobacillus rhamnosus, which has been reported to cause bacteremia (136). Second, excessive or long-term probiotic consumption may lead to mild gastrointestinal symptoms, such as bloating and indigestion, particularly in individuals with inflammatory bowel disease (IBD). However, these symptoms generally subside as the gut microbiota adapts (137). Furthermore, probiotics may influence drug interactions, potentially reducing the efficacy of concomitant medications. For example, in antibiotic-associated diarrhea, probiotics may delay the restoration of normal gut microbiota (138).
It is also important to consider the potential negative effects of postbiotics. Certain postbiotics may influence autoimmune diseases by either enhancing or suppressing the immune system (139). Additionally, probiotics may be involved in the production of toxic metabolites, such as histamine, which can trigger allergic reactions, headaches, and itching. Recent studies have shown that Lactobacillus reuteri plays a role in histamine activation and metabolism, activating the H2 receptor to exert anti-inflammatory effects (140). Moreover, other studies suggest that while the colibactin-producing Escherichia coli strain Nissle 1917 (EcN1917) exhibits significantly reduced genotoxic activity compared to other EcN strains, it may still increase the likelihood of hazardous mutations through mutagenic mechanisms (141).
In summary, for the general population, probiotics and postbiotics can serve as beneficial dietary supplements for clinical applications. However, their use should be approached with caution in immunocompromised individuals, critically ill patients, infants, and those with severe allergies due to potential risks (142).
9 Conclusions and future prospects
Growing evidence indicates a growing interest in probiotic preparations for improving gastrointestinal health. As viable candidates for treating digestive diseases, probiotics exhibit diverse mechanisms and beneficial effects, including modulating immunity, reducing inflammation, improving gut health, alleviating oxidative stress, and regulating apoptosis, with minimal safety risks (5, 143). This review systematically analyzes the ability of different probiotic types and strains to regulate apoptosis, both individually and synergistically. Different strains of the same species, probiotics themselves, and postbiotics may exhibit varying anti-apoptotic effects in disease development and progression. These effects may collectively influence specific signaling pathways and targets, particularly membrane receptors, Bcl-2 family proteins, mitochondria, and caspases. Currently, most research has been conducted in animal models, with only a limited number of studies focusing on apoptosis in cancer at the in vitro cellular level. Due to interspecies differences, the complexity of how probiotics regulate apoptosis in human diseases remains incompletely understood. Furthermore, some studies lack comprehensive evidence supporting the regulation of apoptosis. Therefore, careful interpretation of study results is crucial. These phenomena can be partially attributed to the intrinsic properties of probiotics, as well as the high diversity of the human gut microbiota, shaped by factors such as population, sex, diet, and other variables. It is essential to rigorously screen for effective and safe probiotics and postbiotics, either administered through fecal microbiota transplantation (FMT) (144) or directly demonstrating therapeutic effects in animal studies. A systematic transition from small-scale safety assessments to large-scale efficacy validation is necessary to confirm their effectiveness and safety in humans.
Finally, probiotics hold promise as dietary supplements for advancing the treatment of digestive diseases, marking a step toward precision and personalized medicine. Most importantly, further clinical studies are needed to validate the beneficial effects observed in animal models.
Author contributions
QX: Data curation, Writing – original draft. JL: Writing – original draft. PY: Resources, Software, Visualization, Writing – review & editing. TQ: Writing – review & editing. SJ: Writing – review & editing. RY: Conceptualization, Funding acquisition, Writing – review & editing.
Funding
The author(s) declare that financial support was received for the research and/or publication of this article. This work was supported by the Jiangsu Provincial Department of Science and Technology (No. BE2022698), the Wuxi Municipal Science and Technology Bureau (No. Y20222003), Medical Key Discipline Program of Wuxi Health Commission (No. CXTD202113), the Top Talent Support Program for young and middle-aged people of Wuxi Health Commission (No. BJ2023077) and the Biobank Program of Wuxi Health Commission (No. SW202201).
Acknowledgments
We would like to thank BioGDP (https://biogdp.com) for figure creating.
Conflict of interest
The authors declare that the research was conducted in the absence of any commercial or financial relationships that could be construed as a potential conflict of interest.
Generative AI statement
The authors declare that no Gen AI was used in the creation of this manuscript.
Publisher’s note
All claims expressed in this article are solely those of the authors and do not necessarily represent those of their affiliated organizations, or those of the publisher, the editors and the reviewers. Any product that may be evaluated in this article, or claim that may be made by its manufacturer, is not guaranteed or endorsed by the publisher.
Abbreviations
EPS, Extracellular polysaccharides; EVs, Extracellular vesicles; SCFAs, Short-chain fatty acids; HKY, Heat-killed yeast; NAFLD, Non-alcoholic fatty liver disease; L., Lactobacillus; Lc., Lactobacillus casei; LGGs, Lactobacillus rhamnosus supernatant; DSS, Dextran sulfate sodium salt; B. bifidum, Bifidobacterium; S., Saccharomyces.
References
1. Wang, Y, Huang, Y, Chase, RC, Li, T, Ramai, D, Li, S, et al. Global burden of digestive diseases: a systematic analysis of the global burden of diseases study, 1990 to 2019. Gastroenterology. (2023) 165:773–783.e15. doi: 10.1053/j.gastro.2023.05.050
2. Peery, AF, Crockett, SD, Murphy, CC, Jensen, ET, Kim, HP, Egberg, MD, et al. Burden and cost of gastrointestinal, liver, and pancreatic diseases in the United States: update 2021. Gastroenterology. (2022) 162:621–44. doi: 10.1053/j.gastro.2021.10.017
3. Rose, TC, Pennington, A, Kypridemos, C, Chen, T, Subhani, M, Hanefeld, J, et al. Analysis of the burden and economic impact of digestive diseases and investigation of research gaps and priorities in the field of digestive health in the European region-white book 2: executive summary. United European Gastroenterol J. (2022) 10:657–62. doi: 10.1002/ueg2.12298
4. The Lancet Gastroenterology Hepatology. Tackling the burden of digestive disorders in Europe. Lancet Gastroenterol Hepatol. (2023) 8:95. doi: 10.1016/s2468-1253(22)00431-9
5. Kumar, S, Ahmad, MF, Nath, P, Roy, R, Bhattacharjee, R, Shama, E, et al. Controlling intestinal infections and digestive disorders using probiotics. J Med Food. (2023) 26:705–20. doi: 10.1089/jmf.2023.0062
6. Xianhui Deng, ZB, Yang, X, Mei, Y, Zhou, Q, Chen, A, Yu, R, et al. Molecular mechanisms of cell death in bronchopulmonary dysplasia. Apoptosis. (2023) 28:39–54. doi: 10.1007/s10495-022-01791-4
7. Kerr, JF, Wyllie, AH, and Currie, AR. Apoptosis: a basic biological phenomenon with wide-ranging implications in tissue kinetics. Br J Cancer. (1972) 26:239–57. doi: 10.1038/bjc.1972.33
8. Kim, H-J, Jeon, H-J, Kim, D-G, Kim, J-Y, Shim, J-J, and Lee, J-H. Lacticaseibacillus paracsei Hy7207 alleviates hepatic steatosis, inflammation, and liver fibrosis in mice with non-alcoholic fatty liver disease. Int J Mol Sci. (2024) 25:9870. doi: 10.3390/ijms25189870
9. Amin, M, Navidifar, T, Saeb, S, Barzegari, E, and Jamalan, M. Tumor-targeted induction of intrinsic apoptosis in Colon Cancer cells by Lactobacillus Plantarum and Lactobacillus Rhamnosus strains. Mol Biol Rep. (2023) 50:5345–54. doi: 10.1007/s11033-023-08445-x
10. Mohamed, WA, Abd-Elhakim, YM, and Ismail, SAA. Involvement of the anti-inflammatory, anti-apoptotic, and anti-secretory activity of bee venom in its therapeutic effects on acetylsalicylic acid-induced gastric ulceration in rats. Toxicology. (2019) 419:11–23. doi: 10.1016/j.tox.2019.03.003
11. Pang, J, Al-Ani, AH, Patel, KM, Young, SN, Kong, I, Chen, J-j, et al. A Necroptotic-to-apoptotic signaling Axis underlies inflammatory bowel disease. bioRxiv. (2024). doi: 10.1101/2024.11.13.623307
12. Sharaf, LK, Sharma, M, Chandel, D, and Shukla, G. Prophylactic intervention of probiotics (L.Acidophilus, L.Rhamnosus gg) and celecoxib modulate Bax-mediated apoptosis in 1,2-Dimethylhydrazine-induced experimental Colon carcinogenesis. BMC Cancer. (2018) 18:1111. doi: 10.1186/s12885-018-4999-9
13. Amimo, JO, Raev, SA, Chepngeno, J, Mainga, AO, Guo, Y, Saif, L, et al. Rotavirus interactions with host intestinal epithelial cells. Front Immunol. (2021) 12:793841. doi: 10.3389/fimmu.2021.793841
14. Huang, L, Zhao, Z, Duan, C, Wang, C, Zhao, Y, Yang, G, et al. Lactobacillus Plantarum C88 protects against aflatoxin B(1)-induced liver injury in mice via inhibition of Nf-Kappab-mediated inflammatory responses and excessive apoptosis. BMC Microbiol. (2019) 19:170. doi: 10.1186/s12866-019-1525-4
15. An, J, Seok, H, and Ha, E-M. Gaba-producing Lactobacillus Plantarum inhibits metastatic properties and induces apoptosis of 5-Fu-resistant colorectal Cancer cells via Gabab receptor signaling. J Microbiol. (2021) 59:202–16. doi: 10.1007/s12275-021-0562-5
16. Piqué, N, Berlanga, M, and Miñana-Galbis, D. Health benefits of heat-killed (Tyndallized) probiotics: An overview. Int J Mol Sci. (2019) 20:2534. doi: 10.3390/ijms20102534
17. Pistritto, G, Trisciuoglio, D, Ceci, C, Garufi, A, and D’Orazi, G. Apoptosis as anticancer mechanism: function and dysfunction of its modulators and targeted therapeutic strategies. Aging (Albany N Y). (2016) 8:603–19. doi: 10.18632/aging.100934
18. Czabotar, PE, Westphal, D, Dewson, G, Ma, S, Hockings, C, Fairlie, WD, et al. Bax crystal structures reveal how Bh3 domains activate Bax and nucleate its oligomerization to induce apoptosis. Cell. (2013) 152:519–31. doi: 10.1016/j.cell.2012.12.031
19. Kim, H, Rafiuddin-Shah, M, Tu, HC, Jeffers, JR, Zambetti, GP, Hsieh, JJ, et al. Hierarchical regulation of mitochondrion-dependent apoptosis by Bcl-2 subfamilies. Nat Cell Biol. (2006) 8:1348–58. doi: 10.1038/ncb1499
20. Leshchiner, ES, Braun, CR, Bird, GH, and Walensky, LD. Direct activation of full-length Proapoptotic Bak. Proc Natl Acad Sci USA. (2013) 110:E986–95. doi: 10.1073/pnas.1214313110
21. Letai, A, Bassik, MC, Walensky, LD, Sorcinelli, MD, Weiler, S, and Korsmeyer, SJ. Distinct Bh3 domains either sensitize or activate mitochondrial apoptosis, serving as prototype Cancer therapeutics. Cancer Cell. (2002) 2:183–92. doi: 10.1016/s1535-6108(02)00127-7
22. Nijhawan, D, Fang, M, Traer, E, Zhong, Q, Gao, W, Du, F, et al. Elimination of Mcl-1 is required for the initiation of apoptosis following ultraviolet irradiation. Genes Dev. (2003) 17:1475–86. doi: 10.1101/gad.1093903
23. Jin, Z, and El-Deiry, WS. Overview of cell death signaling pathways. Cancer Biol Ther. (2005) 4:147–71. doi: 10.4161/cbt.4.2.1508
24. Nakano, K, and Vousden, KH. Puma, a novel Proapoptotic gene, is induced by P53. Mol Cell. (2001) 7:683–94. doi: 10.1016/s1097-2765(01)00214-3
25. Ley, R, Balmanno, K, Hadfield, K, Weston, C, and Cook, SJ. Activation of the Erk1/2 signaling pathway promotes phosphorylation and proteasome-dependent degradation of the Bh3-only protein, Bim. J Biol Chem. (2003) 278:18811–6. doi: 10.1074/jbc.M301010200
26. Gibson, GR, Scott, KP, Rastall, RA, Tuohy, KM, Hotchkiss, A, Dubert-Ferrandon, A, et al. Dietary prebiotics: current status and new definition. Food Sci Technol Bull Funct Foods. (2010) 7:1–19. doi: 10.1616/1476-2137.15880
27. Johnstone, RW, Frew, AJ, and Smyth, MJ. The Trail apoptotic pathway in Cancer onset, progression and therapy. Nat Rev Cancer. (2008) 8:782–98. doi: 10.1038/nrc2465
28. Pennica, D, Nedwin, GE, Hayflick, JS, Seeburg, PH, Derynck, R, Palladino, MA, et al. Human tumour necrosis factor: precursor structure, expression and homology to Lymphotoxin. Nature. (1984) 312:724–9. doi: 10.1038/312724a0
29. Schneider, P, Bodmer, JL, Holler, N, Mattmann, C, Scuderi, P, Terskikh, A, et al. Characterization of Fas (Apo-1, Cd95)-Fas ligand interaction. J Biol Chem. (1997) 272:18827–33. doi: 10.1074/jbc.272.30.18827
30. Strasser, A, Jost, PJ, and Nagata, S. The many roles of Fas receptor signaling in the immune system. Immunity. (2009) 30:180–92. doi: 10.1016/j.immuni.2009.01.001
31. Wiley, SR, Schooley, K, Smolak, PJ, Din, WS, Huang, CP, Nicholl, JK, et al. Identification and characterization of a new member of the Tnf family that induces apoptosis. Immunity. (1995) 3:673–82. doi: 10.1016/1074-7613(95)90057-8
32. Pan, G, O’Rourke, K, Chinnaiyan, AM, Gentz, R, Ebner, R, Ni, J, et al. The receptor for the cytotoxic Ligand Trail. Science. (1997) 276:111–3. doi: 10.1126/science.276.5309.111
33. MacEwan, DJ. TNF ligands and receptors--a matter of life and death. Br J Pharmacol. (2002) 135:855–75. doi: 10.1038/sj.bjp.0704549
34. Itoh, N, Yonehara, S, Ishii, A, Yonehara, M, Mizushima, S, Sameshima, M, et al. The polypeptide encoded by the Cdna for human cell surface antigen Fas can mediate apoptosis. Cell. (1991) 66:233–43. doi: 10.1016/0092-8674(91)90614-5
35. Obeng, E. Apoptosis (programmed cell death) and its signals - a review. Braz J Biol. (2021) 81:1133–43. doi: 10.1590/1519-6984.228437
36. Billen, LP, Shamas-Din, A, and Andrews, DW. Bid: A Bax-Like Bh3 Protein. Oncogene. (2008) 27:S93–S104. doi: 10.1038/onc.2009.47
37. Irmler, M, Thome, M, Hahne, M, Schneider, P, Hofmann, K, Steiner, V, et al. Inhibition of death receptor signals by cellular Flip. Nature. (1997) 388:190–5. doi: 10.1038/40657
38. Levy, DS, Kahana, JA, and Kumar, R. Akt inhibitor, Gsk690693, induces growth inhibition and apoptosis in acute lymphoblastic leukemia cell lines. Blood. (2009) 113:1723–9. doi: 10.1182/blood-2008-02-137737
39. Lin, L, Ding, D, Jiang, Y, Li, Y, and Li, S. Mek inhibitors induce apoptosis via Foxo3a-dependent Puma induction in colorectal Cancer cells. Oncogenesis. (2018) 7:67. doi: 10.1038/s41389-018-0078-y
40. Preuss, E, Hugle, M, Reimann, R, Schlecht, M, and Fulda, S. Pan-mammalian target of rapamycin (Mtor) inhibitor Azd8055 primes rhabdomyosarcoma cells for Abt-737-induced apoptosis by Down-regulating Mcl-1 protein. J Biol Chem. (2013) 288:35287–96. doi: 10.1074/jbc.M113.495986
41. Wang, X, Martindale, JL, and Holbrook, NJ. Requirement for Erk activation in cisplatin-induced apoptosis. J Biol Chem. (2000) 275:39435–43. doi: 10.1074/jbc.M004583200
42. Will, M, Qin, AC, Toy, W, Yao, Z, Rodrik-Outmezguine, V, Schneider, C, et al. Rapid induction of apoptosis by Pi3k inhibitors is dependent upon their transient inhibition of Ras-Erk signaling. Cancer Discov. (2014) 4:334–47. doi: 10.1158/2159-8290.Cd-13-0611
43. Cocco, E, Scaltriti, M, and Drilon, A. Ntrk fusion-positive cancers and Trk inhibitor therapy. Nat Rev Clin Oncol. (2018) 15:731–47. doi: 10.1038/s41571-018-0113-0
44. Fink, MY, and Chipuk, JE. Survival of Her2-positive breast Cancer cells: receptor signaling to apoptotic control centers. Genes Cancer. (2013) 4:187–95. doi: 10.1177/1947601913488598
45. Goel, S, Hidalgo, M, and Perez-Soler, R. Egfr inhibitor-mediated apoptosis in solid tumors. J Exp Ther Oncol. (2007) 6:305–20.
46. Henson, ES, Hu, X, and Gibson, SB. Herceptin sensitizes Erbb2-overexpressing cells to apoptosis by reducing Antiapoptotic Mcl-1 expression. Clin Cancer Res. (2006) 12:845–53. doi: 10.1158/1078-0432.Ccr-05-0754
47. Jung, HY, Joo, HJ, Park, JK, and Kim, YH. The blocking of C-met signaling induces apoptosis through the increase of P53 protein in lung Cancer. Cancer Res Treat. (2012) 44:251–61. doi: 10.4143/crt.2012.44.4.251
48. Smith, KM, Fagan, PC, Pomari, E, Germano, G, Frasson, C, Walsh, C, et al. Antitumor activity of Entrectinib, a Pan-Trk, Ros1, and Alk inhibitor, in Etv6-Ntrk3-positive acute myeloid leukemia. Mol Cancer Ther. (2018) 17:455–63. doi: 10.1158/1535-7163.Mct-17-0419
49. Salminen, S, Collado, MC, Endo, A, Hill, C, Lebeer, S, Quigley, EMM, et al. The international scientific Association of Probiotics and Prebiotics (Isapp) consensus statement on the definition and scope of Postbiotics. Nat Rev Gastroenterol Hepatol. (2021) 18:649–67. doi: 10.1038/s41575-021-00440-6
50. Khedr, OMS, El-Sonbaty, SM, Moawed, FSM, Kandil, EI, and Abdel-Maksoud, BE. Lactobacillus Acidophilus Atcc 4356 exopolysaccharides suppresses mediators of inflammation through the inhibition of Tlr2/Stat-3/P38-Mapk pathway in Den-induced Hepatocarcinogenesis in rats. Nutr Cancer. (2021) 74:1037–47. doi: 10.1080/01635581.2021.1934490
51. Behzadi, E, Mahmoodzadeh Hosseini, H, and Imani Fooladi, AA. The inhibitory impacts of Lactobacillus Rhamnosus gg-derived extracellular vesicles on the growth of hepatic Cancer cells. Microb Pathog. (2017) 110:1–6. doi: 10.1016/j.micpath.2017.06.016
52. Nozari, S, Faridvand, Y, Etesami, A, Ahmad Khan Beiki, M, Miresmaeili Mazrakhondi, SA, and Abdolalizadeh, J. Potential anticancer effects of Cell Wall protein fractions from Lactobacillus Paracasei on human intestinal Caco-2 cell line. Lett Appl Microbiol. (2019) 69:148–54. doi: 10.1111/lam.13198
53. Zheng, C, Niu, M, Kong, Y, Liu, X, Li, J, Gong, X, et al. Oral Administration of Probiotic Spore Ghosts for efficient attenuation of radiation-induced intestinal injury. J Nanobiotechnol. (2024) 22:303. doi: 10.1186/s12951-024-02572-8
54. Huang, Y, Zhang, J, Dong, R, Ji, X, Jiang, Y, Cen, J, et al. Lactate as a metabolite from probiotic lactobacilli mitigates ethanol-induced gastric mucosal injury: An in vivo study. BMC Complement Med Ther. (2021) 21:26. doi: 10.1186/s12906-020-03198-7
55. Homayouni Rad, A, Aghebati Maleki, L, Samadi Kafil, H, Fathi Zavoshti, H, and Abbasi, A. Postbiotics as novel health-promoting ingredients in functional foods. Health Promot Perspect. (2020) 10:3–4. doi: 10.15171/hpp.2020.02
56. Shamekhi, S, Abdolalizadeh, J, Ostadrahimi, A, Mohammadi, SA, Barzegari, A, Lotfi, H, et al. Apoptotic effect of Saccharomyces Cerevisiae on human Colon Cancer Sw480 cells by regulation of Akt/Nf-ĸb signaling pathway. Probiot Antimicrob Prot. (2019) 12:311–9. doi: 10.1007/s12602-019-09528-7
57. Li, N, Niu, L, Liu, Y, Wang, Y, Su, X, Xu, C, et al. Taking Scfas produced by Lactobacillus Reuteri orally reshapes gut microbiota and elicits antitumor responses. J Nanobiotechnol. (2024) 22:241. doi: 10.1186/s12951-024-02506-4
58. Lang, S, and Schnabl, B. Microbiota and fatty liver disease-the known, the unknown, and the future. Cell Host Microbe. (2020) 28:233–44. doi: 10.1016/j.chom.2020.07.007
59. Albillos, A, de Gottardi, A, and Rescigno, M. The gut-liver Axis in liver disease: pathophysiological basis for therapy. J Hepatol. (2020) 72:558–77. doi: 10.1016/j.jhep.2019.10.003
60. Wang, Y, Yan, H, Zheng, Q, and Sun, X. The crucial function of gut microbiota on gut-liver repair. hLife. (2025). Epub ahead of print. doi: 10.1016/j.hlife.2025.01.001
61. Meng, X, Li, S, Li, Y, Gan, R-Y, and Li, H-B. Gut Microbiota’s relationship with liver disease and role in Hepatoprotection by dietary natural products and probiotics. Nutrients. (2018) 10:1457. doi: 10.3390/nu10101457
62. Xin, J, Zeng, D, Wang, H, Ni, X, Yi, D, Pan, K, et al. Preventing non-alcoholic fatty liver disease through Lactobacillus Johnsonii Bs15 by attenuating inflammation and mitochondrial injury and improving gut environment in obese mice. Appl Microbiol Biotechnol. (2014) 98:6817–29. doi: 10.1007/s00253-014-5752-1
63. Aligita, W, Singgih, M, Sutrisno, E, and Adnyana, IK. Hepatoprotective properties of water kefir: a traditional fermented drink and its potential role. Int J Prev Med. (2023) 14:93. doi: 10.4103/ijpvm.ijpvm_29_22
64. Hsu, TC, Huang, CY, Liu, CH, Hsu, KC, Chen, YH, and Tzang, BS. Lactobacillus Paracasei Gmnl-32, Lactobacillus Reuteri Gmnl-89 and L. reuteri Gmnl-263 ameliorate hepatic injuries in lupus-prone mice. Br J Nutr. (2017) 117:1066–74. doi: 10.1017/S0007114517001039
65. Cui, Y, Qi, S, Zhang, W, Mao, J, Tang, R, Wang, C, et al. Lactobacillus Reuteri Zj617 culture supernatant attenuates acute liver injury induced in mice by lipopolysaccharide. J Nutr. (2019) 149:2046–55. doi: 10.1093/jn/nxz088
66. Wree, A, McGeough, MD, Inzaugarat, ME, Eguchi, A, Schuster, S, Johnson, CD, et al. Nlrp3 Inflammasome driven liver injury and fibrosis: roles of Il-17 and Tnf in mice. Hepatology. (2017) 67:736–49. doi: 10.1002/hep.29523
67. Wang, Q, Wang, F, Zhou, Y, Li, X, Xu, S, Tang, L, et al. Bacillus Amyloliquefaciens Sc06 attenuated lipopolysaccharide-induced acute liver injury by suppressing bile acid-associated Nlrp3 Inflammasome activation. Int Immunopharmacol. (2024) 142:142. doi: 10.1016/j.intimp.2024.113129
68. Li, X, Lv, Z, Chen, J, Nepovimova, E, Long, M, Wu, W, et al. Bacillus Amyloliquefaciens B10 can alleviate liver apoptosis and oxidative stress induced by aflatoxin B1. Food Chem Toxicol. (2021) 151:151. doi: 10.1016/j.fct.2021.112124
69. Devarbhavi, H, Asrani, SK, Arab, JP, Nartey, YA, Pose, E, and Kamath, PS. Global burden of liver disease: 2023 update. J Hepatol. (2023) 79:516–37. doi: 10.1016/j.jhep.2023.03.017
70. El-Khadragy, MF, Al-Olayan, EM, Elmallah, MIY, Alharbi, AM, Yehia, HM, and Abdel Moneim, AE. Probiotics and yogurt modulate oxidative stress and fibrosis in livers of Schistosoma Mansoni-infected mice. BMC Complement Altern Med. (2019) 19:3. doi: 10.1186/s12906-018-2406-3
71. Mularczyk, M, Bourebaba, Y, Kowalczuk, A, Marycz, K, and Bourebaba, L. Probiotics-rich emulsion improves insulin Signalling in palmitate/Oleate-challenged human Hepatocarcinoma cells through the modulation of Fetuin-a/Tlr4-Jnk-Nf-Κb pathway. Biomed Pharmacother. (2021) 139:111560. doi: 10.1016/j.biopha.2021.111560
72. Xu, T, Zhang, L, Li, M, Zhu, H, Ni, Y, Huang, C, et al. Dextran sulfate sodium-induced colitis exacerbates periodontitis via the Nadph oxidase 2/reactive oxygen species Axis in M1-like macrophages. hLife. (2025). Epub ahead of print. doi: 10.1016/j.hlife.2025.01.006
73. Dalal, RS, Kallumkal, G, Cabral, HJ, Bachour, S, Barnes, EL, and Allegretti, JR. Comparative effectiveness of Upadacitinib versus Ustekinumab for ulcerative colitis: a multicenter retrospective cohort study. Clin Gastroenterol Hepatol. (2024) 22:666–8. doi: 10.1016/j.cgh.2023.08.021
74. Saeid Seyedian, S, Nokhostin, F, and Dargahi, MM. A review of the diagnosis, prevention, and treatment methods of inflammatory bowel disease. J Med Life. (2019) 12:113–22. doi: 10.25122/jml-2018-0075
75. Shen, Z-H, Zhu, C-X, Quan, Y-S, Yang, Z-Y, Wu, S, Luo, W-W, et al. Relationship between intestinal microbiota and ulcerative colitis: mechanisms and clinical application of probiotics and fecal microbiota transplantation. World J Gastroenterol. (2018) 24:5–14. doi: 10.3748/wjg.v24.i1.5
76. Yoda, K, Miyazawa, K, Hosoda, M, Hiramatsu, M, Yan, F, and He, F. Lactobacillus gg-fermented Milk prevents Dss-induced colitis and regulates intestinal epithelial homeostasis through activation of epidermal growth factor receptor. Eur J Nutr. (2014) 53:105–15. doi: 10.1007/s00394-013-0506-x
77. Yan, F, Cao, H, Cover, TL, Washington, MK, Shi, Y, Liu, L, et al. Colon-specific delivery of a probiotic-derived soluble protein ameliorates intestinal inflammation in mice through an Egfr-dependent mechanism. J Clin Invest. (2011) 121:2242–53. doi: 10.1172/jci44031
78. Chae, JM, Heo, W, Cho, HT, Lee, DH, Kim, JH, Rhee, MS, et al. Effects of orally-administered Bifidobacterium Animalis Subsp. Lactis strain Bb12 on dextran sodium sulfate-induced colitis in mice. J Microbiol Biotechnol. (2018) 28:1800–5. doi: 10.4014/jmb.1805.05072
79. Chae, JM, Chang, MH, Heo, W, Cho, HT, Lee, DH, Hwang, BB, et al. Lb-9, novel probiotic lactic acid Bacteria, ameliorates dextran sodium sulfate-induced colitis in mice by inhibiting Tnf-alpha-mediated apoptosis of intestinal epithelial cells. J Med Food. (2019) 22:271–6. doi: 10.1089/jmf.2018.4236
80. Weng, M, Ganguli, K, Zhu, W, Shi, HN, and Walker, WA. Conditioned medium from Bifidobacteria Infantis protects against Cronobacter Sakazakii-induced intestinal inflammation in newborn mice. Am J Physiol Gastrointest Liver Physiol. (2014) 306:G779–87. doi: 10.1152/ajpgi.00183.2013
81. Jampilek, J, Kos, J, and Kralova, K. Potential of nanomaterial applications in dietary supplements and foods for special medical purposes. Nano. (2019) 9:296. doi: 10.3390/nano9020296
82. Alkushi, AG, Abdelfattah-Hassan, A, Eldoumani, H, Elazab, ST, Mohamed, SAM, Metwally, AS, et al. Probiotics-loaded nanoparticles attenuated Colon inflammation, oxidative stress, and apoptosis in colitis. Sci Rep. (2022) 12:5116. doi: 10.1038/s41598-022-08915-5
83. Luo, Z, Sun, J, Liu, J, Yu, P, Ye, D, Qi, C, et al. Human Milk-derived Limosilactobacillus reuteri Fn041 ameliorates DSS-induced colitis by remodeling gut microbiota and metabolites in mice. Food Biosci. (2025) 63:105736. doi: 10.1016/j.fbio
84. Islam, MR, Akash, S, Rahman, MM, Nowrin, FT, Akter, T, Shohag, S, et al. Colon Cancer and colorectal Cancer: prevention and treatment by potential natural products. Chem Biol Interact. (2022) 368:110170. doi: 10.1016/j.cbi.2022.110170
85. Eslami, M, Yousefi, B, Kokhaei, P, Hemati, M, Nejad, ZR, Arabkari, V, et al. Importance of probiotics in the prevention and treatment of colorectal Cancer. J Cell Physiol. (2019) 234:17127–43. doi: 10.1002/jcp.28473
86. Tong, X, Tang, R, Xiao, M, Xu, J, Wang, W, Zhang, B, et al. Targeting cell death pathways for Cancer therapy: recent developments in necroptosis, Pyroptosis, Ferroptosis, and Cuproptosis research. J Hematol Oncol. (2022) 15:174. doi: 10.1186/s13045-022-01392-3
87. Li, JQ, Li, JL, Xie, YH, Wang, Y, Shen, XN, Qian, Y, et al. Saccharomyces Cerevisiae may serve as a probiotic in colorectal Cancer by promoting Cancer cell apoptosis. J Dig Dis. (2020) 21:571–82. doi: 10.1111/1751-2980.12930
88. Beck, LC, Masi, AC, Young, GR, Vatanen, T, Lamb, CA, Smith, R, et al. Strain-specific impacts of probiotics are a significant driver of gut microbiome development in very preterm infants. Nat Microbiol. (2022) 7:1525–35. doi: 10.1038/s41564-022-01213-w
89. Chondrou, P, Karapetsas, A, Kiousi, DE, Tsela, D, Tiptiri-Kourpeti, A, Anestopoulos, I, et al. Lactobacillus Paracasei K5 displays adhesion, anti-proliferative activity and apoptotic effects in human Colon Cancer cells. Benefic Microbes. (2018) 9:975–83. doi: 10.3920/bm2017.0183
90. Nychas, G-J, Tiptiri-Kourpeti, A, Spyridopoulou, K, Santarmaki, V, Aindelis, G, Tompoulidou, E, et al. Lactobacillus Casei exerts anti-proliferative effects accompanied by apoptotic cell death and up-regulation of Trail in Colon carcinoma cells. PLoS One. (2016) 11:e0147960. doi: 10.1371/journal.pone.0147960
91. Abd El-Hameed, RH, Mohamed, MS, Awad, SM, Hassan, BB, Khodair, MAE-F, and Mansour, YE. Novel benzo Chromene derivatives: design, synthesis, molecular docking, cell cycle arrest, and apoptosis induction in human acute myeloid leukemia hl-60 cells. J Enzyme Inhib Med Chem. (2022) 38:405–22. doi: 10.1080/14756366.2022.2151592
92. Huang, L, Shan, Y-J, He, C-X, Ren, M-H, Tian, P-J, and Song, W. Effects of L. paracasei subp. paracasei X12 on cell cycle of colon cancer HT-29 cells and regulation of mTOR signalling pathway1,2. J Funct Foods. (2016) 21:431–9. doi: 10.1016/j.jff.2015.12.024
93. Ghanavati, R, Akbari, A, Mohammadi, F, Asadollahi, P, Javadi, A, Talebi, M, et al. Lactobacillus species inhibitory effect on colorectal Cancer progression through modulating the Wnt/Β-catenin signaling pathway. Mol Cell Biochem. (2020) 470:1–13. doi: 10.1007/s11010-020-03740-8
94. Dong, Y, Zhu, J, Zhang, M, Ge, S, and Zhao, L. Probiotic Lactobacillus Salivarius Ren prevent Dimethylhydrazine-induced colorectal Cancer through protein kinase B inhibition. Appl Microbiol Biotechnol. (2020) 104:7377–89. doi: 10.1007/s00253-020-10775-w
95. Pakbin, B, Allahyari, S, Dibazar, SP, Peymani, A, Haghverdi, MK, Taherkhani, K, et al. Anticancer properties of Saccharomyces Boulardii metabolite against Colon Cancer cells. Probiot Antimicrob Prot. (2022) 16:224–32. doi: 10.1007/s12602-022-10030-w
96. Wen, Y, Zhu, Y, Zhang, C, Yang, X, Gao, Y, Li, M, et al. Chronic inflammation, Cancer development and immunotherapy. Front Pharmacol. (2022) 13:1040163. doi: 10.3389/fphar.2022.1040163
97. Rong, J, Liu, S, Hu, C, and Liu, C. Single probiotic supplement suppresses colitis-associated colorectal tumorigenesis by modulating inflammatory development and microbial homeostasis. J Gastroenterol Hepatol. (2018) 34:1182–92. doi: 10.1111/jgh.14516
98. Bae, W-Y, Jung, W-H, Shin, SL, Kwon, S, Sohn, M, and Kim, T-R. Investigation of Immunostimulatory effects of heat-treated Lactiplantibacillus Plantarum Lm1004 and its underlying molecular mechanism. Food Sci Anim Resour. (2022) 42:1031–45. doi: 10.5851/kosfa.2022.e50
99. Karimi Ardestani, S, Tafvizi, F, and Tajabadi, EM. Heat-killed probiotic Bacteria induce apoptosis of Ht-29 human Colon adenocarcinoma cell line via the regulation of Bax/Bcl2 and caspases pathway. Hum Exp Toxicol. (2019) 38:1069–81. doi: 10.1177/0960327119851255
100. Yoo, HM, Kang, D, Kim, S, Lee, J-E, and Lee, J. Evaluating cell death using cell-free supernatant of probiotics in three-dimensional spheroid cultures of colorectal Cancer cells. J Vis Exp. (2020) 160:e61285. doi: 10.3791/61285
101. Kim, H-J, An, J, and Ha, E-M. Lactobacillus Plantarum-derived metabolites sensitize the tumor-suppressive effects of butyrate by regulating the functional expression of Smct1 in 5-Fu-resistant colorectal Cancer cells. J Microbiol. (2021) 60:100–17. doi: 10.1007/s12275-022-1533-1
102. Walia, S, Kamal, R, Dhawan, DK, and Kanwar, SS. Chemoprevention by probiotics during 1,2-Dimethylhydrazine-induced Colon carcinogenesis in rats. Dig Dis Sci. (2018) 63:900–9. doi: 10.1007/s10620-018-4949-z
103. Jung, K-H, Lee, JH, Kim, M, Lee, EJ, Cho, YS, Lee, K-H, et al. Celecoxib-induced modulation of Colon Cancer Cd133 expression occurs through Akt inhibition and is monitored by 89zr Immuno-pet. Mol Imaging. (2022) 2022:4906934. doi: 10.1155/2022/4906934
104. Zhang, D, Jian, Y-P, Zhang, Y-N, Li, Y, Gu, L-T, Sun, H-H, et al. Short-chain fatty acids in diseases. Cell Commun Signal. (2023) 21:212. doi: 10.1186/s12964-023-01219-9
105. Jang, B, Yang, I-H, Cho, N-P, Jin, B, Lee, W, Jung, YC, et al. Down-regulation and nuclear localization of Survivin by sodium butyrate induces caspase-dependent apoptosis in human Oral Mucoepidermoid carcinoma. Oral Oncol. (2019) 88:160–7. doi: 10.1016/j.oraloncology.2018.11.032
106. Foglietta, F, Serpe, L, Canaparo, R, Vivenza, N, Riccio, G, Imbalzano, E, et al. Modulation of butyrate anticancer activity by solid lipid nanoparticle delivery: An in vitro investigation on human breast Cancer and leukemia cell lines. J Pharm Pharm Sci. (2014) 17:231–47. doi: 10.18433/j3xp4r
107. Chiang, C-J, and Hong, Y-H. In situ delivery of biobutyrate by probiotic Escherichia Coli for Cancer therapy. Sci Rep. (2021) 11:18172. doi: 10.1038/s41598-021-97457-3
108. Niu, L, Liu, Y, Li, N, Wang, Y, Kang, L, Su, X, et al. Oral probiotics microgel plus Galunisertib reduced Tgf-Β blockade resistance and enhanced anti-tumor immune responses in colorectal Cancer. Int J Pharm. (2024) 652:123810. doi: 10.1016/j.ijpharm.2024.123810
109. Fareez, IM, Lim, SM, and Ramasamy, K. Chemoprevention by microencapsulated Lactiplantibacillus Plantarum Lab12 against Orthotopic colorectal Cancer mice is associated with apoptosis and anti-angiogenesis. Probiotics Antimicrob Proteins. (2022) 16:99–112. doi: 10.1007/s12602-022-10020-y
110. Peng, Y, Ma, Y, Luo, Z, Jiang, Y, Xu, Z, and Yu, R. Lactobacillus Reuteri in digestive system diseases: focus on clinical trials and mechanisms. Front Cell Infect Microbiol. (2023) 13:1254198. doi: 10.3389/fcimb.2023.1254198
111. Chang, X, Zhang, S, Li, C, Zhang, H, Yang, W, Zhang, W, et al. Inhibitory effect of Lactobacillus Paracasei Cmu-Pb-L5 in a subcutaneous transplanted tumor model of colorectal Cancer. Int J Med Sci. (2024) 21:2525–36. doi: 10.7150/ijms.99646
112. Yan, H, Ren, J, and Liu, G-H. Fecal microbiota transplantation: a new strategy to delay aging. hLife. (2023) 1:8–11. doi: 10.1016/j.hlife.2023.06.002
113. Chen, Y, Fang, H, Chen, H, Liu, X, Zhao, J, Stanton, C, et al. Bifidobacterium inhibits the progression of colorectal tumorigenesis in mice through fatty acid isomerization and gut microbiota modulation. Gut Microbes. (2025) 17:2464945. doi: 10.1080/19490976.2025.2464945
114. Chen, Y, Ma, W, Zhao, J, Stanton, C, Ross, RP, Zhang, H, et al. Lactobacillus Plantarum ameliorates colorectal Cancer by ameliorating the intestinal barrier through the Cla-Ppar-Γ Axis. J Agric Food Chem. (2024) 72:19766–85. doi: 10.1021/acs.jafc.4c02824
115. Srikham, K, and Thirabunyanon, M. Bioprophylactic potential of novel human colostrum probiotics via apoptotic induction of Colon Cancer cells and cell immune activation. Biomed Pharmacother. (2022) 149:112871. doi: 10.1016/j.biopha.2022.112871
116. Wenhong Yang, TL, An, S, Chen, R, Zhao, Y, Cui, J, Zhang, M, et al. Ligilactobacillus salivarius Lzzay01 accelerated autophagy and apoptosis in Colon Cancer cells and improved gut microbiota in Cac mice. Microbiol Spectr. (2025) 13:e0186124. doi: 10.1128/spectrum.01861-24
117. Wijtten, PJA, Jvd, M, and Verstegen, MWA. Intestinal barrier function and absorption in pigs after weaning: a review. Br J Nutr. (2011) 105:967–81. doi: 10.1017/s0007114510005660
118. Xiao, Z, Liu, L, Tao, W, Pei, X, Wang, G, and Wang, M. Clostridium Tyrobutyricum protect intestinal barrier function from Lps-induced apoptosis via P38/Jnk signaling pathway in Ipec-J2 cells. Cell Physiol Biochem. (2018) 46:1779–92. doi: 10.1159/000489364
119. Peng, M, Liu, J, and Liang, Z. Probiotic Bacillus Subtilis Cw14 reduces disruption of the epithelial barrier and toxicity of Ochratoxin a to Caco-2 cells. Food Chem Toxicol. (2019) 126:25–33. doi: 10.1016/j.fct.2019.02.009
120. Genchi, G, Sinicropi, MS, Lauria, G, Carocci, A, and Catalano, A. The effects of cadmium toxicity. Int J Environ Res Public Health. (2020) 17:3782. doi: 10.3390/ijerph17113782
121. Huang, Y-Y, Xia, M-Z, Wang, H, Liu, X-J, Hu, Y-F, Chen, Y-H, et al. Cadmium selectively induces Mip-2 and cox-2 through Pten-mediated Akt activation in Raw264.7 cells. Toxicol Sci. (2014) 138:310–21. doi: 10.1093/toxsci/kfu013
122. Dashtbanei, S, and Keshtmand, Z. A mixture of multi-strain probiotics (Lactobacillus Rhamnosus, Lactobacillus Helveticus, and Lactobacillus Casei) had anti-inflammatory, anti-apoptotic, and anti-oxidative effects in oxidative injuries induced by cadmium in small intestine and lung. Probiotics Antimicrob Proteins. (2022) 15:226–38. doi: 10.1007/s12602-022-09946-0
123. Gelen, V, Gedikli, S, Gelen, SU, Şengül, E, and Makav, M. Probiotic Bacteria protect against indomethacin-induced gastric ulcers through modulation of oxidative stress, inflammation, and apoptosis. Mol Biol Rep. (2024) 51:684. doi: 10.1007/s11033-024-09627-x
124. Wang, Z, Wang, D, Ren, X, Liu, Z, Liu, A, Li, X, et al. One stone, three birds: multifunctional Nanodots as “pilot light” for guiding surgery, enhanced radiotherapy, and brachytherapy of tumors. ACS Central Sci. (2023) 9:1976–88. doi: 10.1021/acscentsci.3c00994
125. Lee, Y, Sugihara, K, Gillilland, MG, Jon, S, Kamada, N, and Moon, JJ. Hyaluronic acid–bilirubin nanomedicine for targeted modulation of dysregulated intestinal barrier, microbiome and immune responses in colitis. Nat Mater. (2019) 19:118–26. doi: 10.1038/s41563-019-0462-9
126. Kim, SY, Shin, SJ, Song, CH, Jo, EK, Kim, HJ, and Park, JK. Destruction Ofbacillus Licheniformisspores by microwave irradiation. J Appl Microbiol. (2009) 106:877–85. doi: 10.1111/j.1365-2672.2008.04056.x
127. Meisenheimer, ES, Epstein, C, and Thiel, D. Acute diarrhea in adults. Am Fam Physician. (2022) 106:72–80.
128. Mohanty, D, Panda, S, Kumar, S, and Ray, P. In vitro evaluation of adherence and anti-infective property of probiotic Lactobacillus Plantarum Dm 69 against Salmonella Enterica. Microb Pathog. (2019) 126:212–7. doi: 10.1016/j.micpath.2018.11.014
129. Buccigrossi, V, Poeta, M, Cioffi, V, Terranova, S, Nunziata, F, Lo Vecchio, A, et al. Lacticaseibacillus Rhamnosus gg counteracts rotavirus-induced ion secretion and enterocyte damage by inhibiting oxidative stress and apoptosis through specific effects of living and Postbiotic preparations. Front Cell Infect Microbiol. (2022) 12:854989. doi: 10.3389/fcimb.2022.854989
130. Lo Vecchio, A, Liguoro, I, Dias, JA, Berkley, JA, Boey, C, Cohen, MB, et al. Rotavirus immunization: global coverage and local barriers for implementation. Vaccine. (2017) 35:1637–44. doi: 10.1016/j.vaccine.2017.01.082
131. Chakravortty, D, Mao, X, Gu, C, Hu, H, Tang, J, Chen, D, et al. Dietary Lactobacillus Rhamnosus gg supplementation improves the mucosal barrier function in the intestine of weaned piglets challenged by porcine rotavirus. PLoS One. (2016) 11:e0146312. doi: 10.1371/journal.pone.0146312
132. Paparo, L, Tripodi, L, Bruno, C, Pisapia, L, Damiano, C, Pastore, L, et al. Protective action of Bacillus Clausii probiotic strains in an in vitro model of rotavirus infection. Sci Rep. (2020) 10:12636. doi: 10.1038/s41598-020-69533-7
133. Hoang, PM, Cho, S, Kim, KE, Byun, SJ, Lee, T-K, and Lee, S. Development of Lactobacillus Paracasei harboring nucleic acid-hydrolyzing 3d8 Scfv as a preventive probiotic against murine norovirus infection. Appl Microbiol Biotechnol. (2014) 99:2793–803. doi: 10.1007/s00253-014-6257-7
134. Kawarizadeh, A, Pourmontaseri, M, Farzaneh, M, Hosseinzadeh, S, Ghaemi, M, Tabatabaei, M, et al. Interleukin-8 gene expression and apoptosis induced by Salmonella Typhimurium in the presence of Bacillus probiotics in the epithelial cell. J Appl Microbiol. (2020) 131:449–59. doi: 10.1111/jam.14898
135. EJW, MGR, and Phillips, RS. The efficacy and safety of probiotics in people with Cancer: a systematic review. Ann Oncol. (2014) 25:1919–29. doi: 10.1093/annonc/mdu106
136. Mikucka, A, Deptuła, A, Bogiel, T, Chmielarczyk, A, Nurczyńska, E, and Gospodarek-Komkowska, E. Bacteraemia caused by probiotic strains of Lacticaseibacillus Rhamnosus—case studies highlighting the need for careful thought before using microbes for health benefits. Pathogens. (2022) 11:977. doi: 10.3390/pathogens11090977
137. Dore, MP, Bibbò, S, Fresi, G, Bassotti, G, and Pes, GM. Side effects associated with probiotic use in adult patients with inflammatory bowel disease: a systematic review and Meta-analysis of randomized controlled trials. Nutrients. (2019) 11:2913. doi: 10.3390/nu11122913
138. Jotham Suez, NZ, Segal, E, and Elinav, E. The pros, cons, and many unknowns of probiotics. Nat Med. (2019) 25:716–29. doi: 10.1038/s41591-019-0439-x
139. Xiao-Jie Mi, THMT, Park, H-R, Xing Yue, X, Subramaniyam, S, Choi, HS, Kim, J, et al. Immune-enhancing effects of Postbiotic produced by Bacillus Velezensis Kh2-2 isolated from Korea foods. Food Res Int. (2022) 152:152. doi: 10.1016/j.foodres.2021.110911
140. Gao, C, Major, A, Rendon, D, Lugo, M, Jackson, V, Shi, Z, et al. Histamine H2 receptor-mediated suppression of intestinal inflammation by probiotic Lactobacillus Reuteri. MBio. (2015) 6:e01358–15. doi: 10.1128/mBio.01358-15
141. Jans, M, Kolata, M, Blancke, G, D’Hondt, A, Gräf, C, Ciers, M, et al. Colibactin-driven Colon Cancer requires Adhesin-mediated epithelial binding. Nature. (2024) 635:472–80. doi: 10.1038/s41586-024-08135-z
142. Daniel Merenstein, BP, Leyer, G, Ouwehand, AC, Preidis, GA, Elkins, CA, Hill, C, et al. Emerging issues in probiotic safety: 2023 perspectives. Gut Microbes. (2023) 15:2185034. doi: 10.1080/19490976.2023.2185034
143. Fang, J, Yang, Y, and Xie, W. Chinese expert consensus on the application of live combined Bifidobacterium, Lactobacillus, and Enterococcus powder/capsule in digestive system diseases (2021). J Gastroenterol Hepatol. (2023) 38:1089–98. doi: 10.1111/jgh.16195
144. Tian, H, Wang, X, Fang, Z, Li, L, Wu, C, Bi, D, et al. Fecal microbiota transplantation in clinical practice: present controversies and future prospects. hLife. (2024) 2:269–83. doi: 10.1016/j.hlife.2024.01.006
Keywords: probiotics, postbiotics, apoptosis, gut microbiota, digestive system diseases
Citation: Xie Q, Liu J, Yu P, Qiu T, Jiang S and Yu R (2025) Unlocking the power of probiotics, postbiotics: targeting apoptosis for the treatment and prevention of digestive diseases. Front. Nutr. 12:1570268. doi: 10.3389/fnut.2025.1570268
Edited by:
Bowen Li, Southwest University, ChinaReviewed by:
Yuanshan Cui, Capital Medical University, ChinaYuge Jiang, Anhui University of Chinese Medicine, China
Copyright © 2025 Xie, Liu, Yu, Qiu, Jiang and Yu. This is an open-access article distributed under the terms of the Creative Commons Attribution License (CC BY). The use, distribution or reproduction in other forums is permitted, provided the original author(s) and the copyright owner(s) are credited and that the original publication in this journal is cited, in accordance with accepted academic practice. No use, distribution or reproduction is permitted which does not comply with these terms.
*Correspondence: Ting Qiu, cWl1dGluZzA4MkAxNjMuY29t; Shanyu Jiang, eXMwMTI5MDA3QHNpbmEuY24=; Renqiang Yu, eXVyZW5xaWFuZzU1M0AxNjMuY29t
†These authors have contributed equally to this work