- 1Department of Epidemiology and Health Statistics, School of Public Health, Sun Yat-sen University, Guangzhou, China
- 2Department of Neurology, Xiangya Hospital, Central South University, Changsha, China
- 3Maternal and Child Healthcare Hospital of Longhua District, Shenzhen, China
- 4School of Health Management, Xinhua College of Guangzhou, Guangzhou, China
Background: Preterm birth (PTB) is a leading cause of neonatal mortality and under-five mortality worldwide, with long-term health impacts. While micronutrient supplementation shows promise in preventing PTB, its effectiveness remains controversial due to confounding factors. This study aims to elucidate the association between micronutrient supplementation and PTB risk by analyzing a large-scale children survey and employing Mendelian Randomization (MR) to address confounding factors.
Methods: This study recruited 66,728 mother-child dyads in Longhua District, Shenzhen, China in 2021. Participants provided information on micronutrient supplementation (multinutrient, folic acid, calcium, and iron) through a structured questionnaire. Logistic regression assessed the association between micronutrient supplementation and PTB in crude, adjusted, and full-inclusion models. MR analysis used summary-level GWAS data from the UK Biobank and FinnGen consortiums. The main MR analyses employed inverse variance weighting (IVW), with sensitivity analyses including MR Egger regression, weighted median, weighted mode, simple mode, and MR-PRESSO.
Results: Observational analysis indicated folic acid (OR = 0.80, 95%CI: 0.72–0.89), calcium (OR = 0.88, 95%CI: 0.80–0.96), and iron (OR = 0.92, 95%CI: 0.86–0.98) as protective factors against PTB, especially in co-supplementation, while multinutrient supplementation showed no significant effect. MR analysis indicating a consistent protective effect of calcium (ORIVW = 0.04, 95% CI: 0.004–0.42, p < 0.01, pFDR < 0.05). Sensitivity analyses supported these findings, detecting no bias or pleiotropy.
Conclusion: Combining observational data with genetic causal inference, our study confirms the protective roles of folic acid, calcium, and iron against PTB, with MR particularly highlighting calcium's causal association with reduced PTB risk. These findings provide a comprehensive understanding and underscore the importance of targeted nutritional interventions, especially calcium, in prenatal care for PTB prevention. However, given the limitations of the self-reported data and the lack of information on doses used in our study, future prospective studies with more detailed micronutrient information are needed to provide more comprehensive evidence.
1 Introduction
Preterm birth (PTB), defined as infants born before 37 weeks of gestation, is a leading cause of neonatal mortality (1). The World Health Organization reports that in 2020, approximately 9.9% of global births (13.4 million) and 6.1% of births in China (752.9 thousand) were preterm (2). Complications from PTB represent the primary cause of death in children under 5 years old, accounting for nearly 0.94 million deaths worldwide in 2019 and serving as the second leading cause of mortality in this age group in China, with 30,900 deaths in 2015 (3, 4). PTB is also associated with immediate, short-term and long-term physical, neurodevelopmental, and socioeconomic effects, such as growth retardation, psychiatric disorders, and increased risks of chronic diseases, including cardiovascular, respiratory, and endocrine/metabolic disorders in early to mid-adulthood (5–7).
Evidence suggests that most PTBs can be mitigated through cost-effective interventions, particularly those aimed at improving the nutritional status of pregnant women and fetuses (8). Micronutrients, most of which can only be acquired exogenously, support nearly all metabolic activities, thereby facilitating fetal development and maturation into a healthy neonate (9). However, deficiencies in micronutrients like iron, vitamin A, zinc, vitamin B12, and folic acid are prevalent among pregnant women worldwide (10–15). In China, the prevalence of vitamin D deficiency among pregnant women increased from 25.52% in 2012 to 41.96% in 2017 (16). Furthermore, low intake levels of iron, iodine, calcium, and folic acid have also been reported (17–20). Therefore, in the fact of micronutrients remains suboptimal among pregnant women, micronutrient supplementation plays important roles in maintaining nutritional status.
Although there have been studies on the association and mechanisms between micronutrient supplementation and PTB, most of them have been observational, and the results have not always been uniform. A meta-analysis of 91,425 participants from 18 trials showed little significant difference between multiple-micronutrient supplementation and PTB, with the confidence interval just crossing the line of no effect (21). However, a cluster randomized, double-blind trial in Bangladesh demonstrated that multiple-micronutrient supplementation reduced the risk of PTB (22). Despite the fact that prenatal iron and folic acid improved low birthweight, trials did not report significant reduction in PTB (23, 24). The association between vitamin D and PTB also presented inconsistent results across different studies (25–29). These inconsistencies may stem from small sample sizes, uncertain causal time sequences, and confounding biases (9, 26, 30). Consequently, there is a need for well-designed studies with large samples controlling for potential confounders to address these disparities.
Mendelian Randomization (MR) offers a novel approach to establish causality by leveraging genetic variants that are randomly allocated at conception, thereby minimizing confounding and reverse causation in observational studies (31). Previous MR research has focused mostly on adulthood and less on early life. Recent genome-wide association studies (GWAS) on perinatal lifestyle phenotypes and PTB provide an opportunity to investigate these associations without bias. There is a relevant MR study, but only for a single nutrient (32), so the relationship between additional nutrients and PTB remains to be explored by MR.
Our study aims to assess the association between micronutrient supplementation and PTB using a large dataset of mother-child dyads with detailed profiles, and to complement the findings with causal inference via MR analysis.
2 Methods
2.1 Observational study
2.1.1 Participants
Participants were recruited from the 2021 children survey, which included 69,638 mother-child dyads from 235 kindergartens in Longhua District, Shenzhen, China. Exclusions were made for cases with no identity record (N = 1,605), missing gestational age (N = 1,303), and unreported maternal micronutrient supplementation (N = 2), resulting in a final sample of 66,728 dyads, see Figure 1. This study received approval from the Ethics Committee of the School of Public Health, Sun Yat-sen University. Informed consent was obtained from all children's primary guardians.
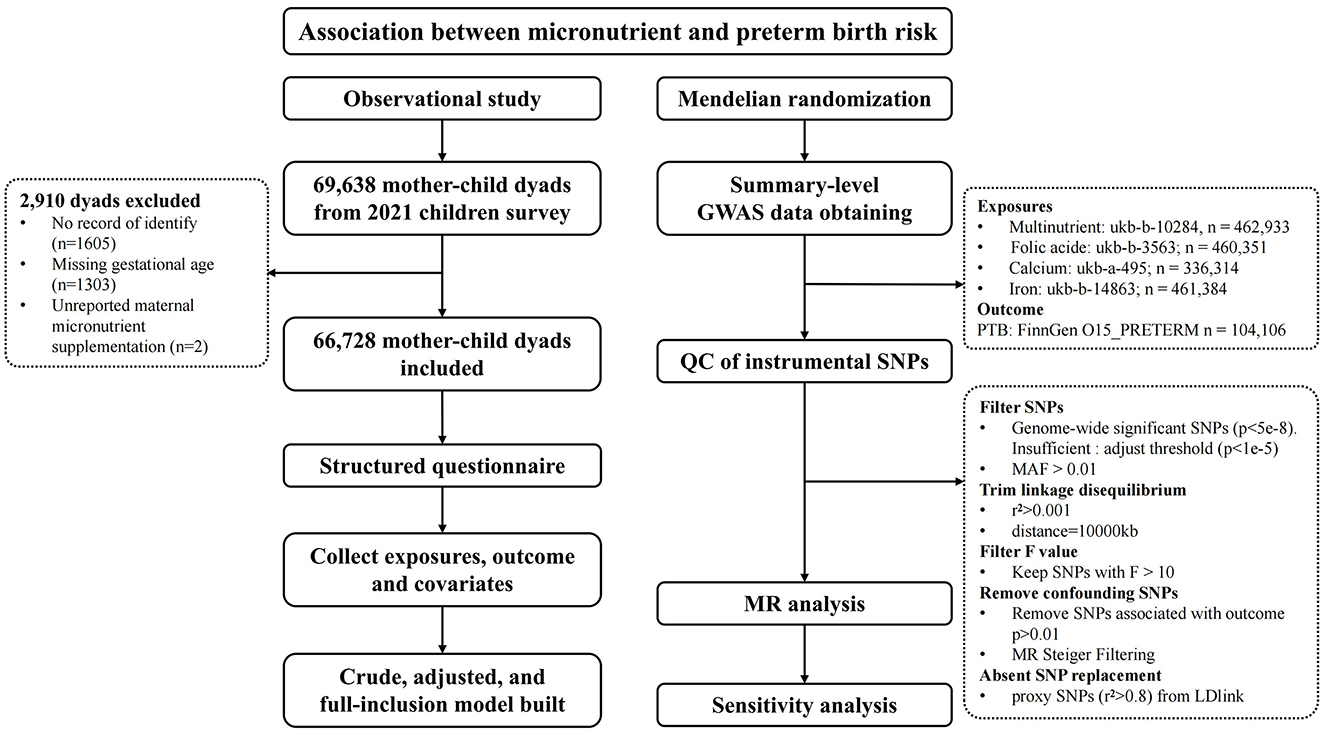
Figure 1. Study profile. GWAS, genome-wide association studies; MAF, minor allele frequency; MR, Mendelian Randomization; QC, Quality Control; SNPs, Single nucleotide polymorphisms.
2.1.2 Data acquirement
Data were collected using an online structured questionnaire, which was completed under the supervision of health practitioners and kindergarten teachers. The questionnaire was administered during the children's kindergarten years (typically 3–7 years after birth), and covered demographic details, maternal condition during pregnancy (the medical history, pregnancy complications, behavior and habits, environmental exposure during pregnancy, etc.), and child birth characteristics (gestational age, birth weight, delivery mode, etc.).
Micronutrient supplementation during pregnancy (multinutrient, folic acid, calcium, and iron) was assessed through maternal self-reporting using four separate questions “Did you take multinutrient (supplements containing three or more vitamins with or without minerals)/folic acid/calcium/iron during your pregnancy?” (Answer was “NO” or “YES”). Mothers who reported “YES” were defined as exposure group, respectively, while those who reported “NO” were defined as the reference group. PTB was determined based on the mother's response to the question “What was the gestational age of your child at birth?” A gestational age of <37 weeks was classified as PTB.
Covariates were collected according to studies and the univariate analysis of our research (29, 33–39), including child's information (sex, birth season), maternal characteristic (age of conception, education, marital status, household income, pre-pregnancy BMI, weight gain during pregnancy, parity, and multiple pregnancy), maternal disease (polycystic ovarian syndrome, pregnancy-induced hypertension, pre-eclampsia, gestational diabetes mellitus, and perinatal depression), and behaviors and lifestyle during pregnancy (employment, prenatal care visit). Multiple imputation (MI) was employed for missing covariate data (40), with details and coding provided in Supplementary Table S1.
2.2 Mendelian randomization
For each micronutrient supplement as exposure, Summary-level GWAS data for micronutrient supplement were collected from the United Kingdom Biobank (UKB) cohort with European ancestry from MRC-IEU consortium, including multinutrient (Treatment/medication code: multinutrient; dataset ukb-b-10284, N = 462,933), folic acid (Vitamin and mineral supplements: Folic acid or Folate; dataset ukb-b-3563, N = 460,351), Calcium (Mineral and other dietary supplements: Calcium; dataset ukb-a-495, N = 336,314), and iron product (Mineral and other dietary supplements: Iron; dataset ukb-b-14863, N = 461,384). For PTB outcome, summary-level GWAS data with sample size of 5,480 cases and 98,626 controls of European descent was obtained from the FinnGen (https://r5.finngen.fi/pheno/O15_PRETERM).
Instrumental variables (IVs) were chosen based on strict criteria to support causal inference, including Single nucleotide polymorphisms (SNPs) selection guidelines to ensure validity and minimize confounding (41). SNPs with a significant threshold (p < 1 × 10−5) were considered as potential instrumental variables. Besides, each SNP was selected based on the following rules: (1) with a minor allele frequency (MAF) more than 0.01; (2) not possibly being in linkage disequilibrium (r2 > 0.001, distance = 10,000 kb); (3) adequate strength of IV evaluated by F-statistics (F > 10); (4) without an exorbitant association with outcome (p > 0.01); (5) avoid reverse causal effect using MR Steiger Filtering. We replaced the SNPs absent in the outcome data with their available corresponding proxy SNPs (r2 > 0.8) through LDlink (https://ldlink.nci.nih.gov/).
2.3 Statistical analysis
In the observational study, associations between micronutrient supplementation during pregnancy and PTB were analyzed using univariate and multivariate logistic regression, under three different models: the crude model, without adjustment for confounders; the adjusted model, adjusted for selected confounders; and the full-inclusion model, included all micronutrients in the model and adjusted for all confounders to address the confounding effects of co-supplementation.
In the MR process, the effect size estimates the association of the SNP with the specific phenotype expressed as β (i.e., ln OR). The Wald ratio was obtained by dividing the β-outcome by the β-exposure. Since there are more than one SNP was available, the inverse variance weighting (IVW) was considered an optimum approach for meta-analysis after vindicating the absence of horizontal pleiotropy and heterogeneity (42). For multiple comparison, the false discovery rate (FDR) correction was utilized to adjust the p-values. Sensitivity analysis includes MR Egger regression (43), weighted median (44), weighted mode (45), simple mode, and MR-PRESSO (46). MR Egger intercepts and Cochran Q statistics were conducted to evaluate heterogeneity and pleiotropy.
Statistical analyses above were performed via R version 4.2.3, with the “TwoSampleMR” v0.5.6 and “MR PRESSO” v1.0 packages. P-value of <0.05 was deemed statistically significant.
3 Results
3.1 Evidence from observational study
Table 1 presents the characteristics of the children survey sample. The mean age of mothers at conception was 29.12 years, with a mean pre-pregnancy BMI was 20.75 Kg/m2. Among the 66,728 mothers analyzed, 85.1% had attained at least a high school education, 97.4% were married, 38.3% gained <10 kg during pregnancy, 45.0% were multiparous, approximately half of the households earned <RMB 20,000 every month, 93.5% had prenatal care visit and 66.2% were employed during pregnancy. Pregnancy complications varied, with perinatal depression at 0.3% and gestational diabetes mellitus at 7.4%. Over half of the children were male, 2.5% were from multiple pregnancies, and births were evenly distributed across seasons. Micronutrient supplementation rates were 41.3% for multinutrient, 92.8% for folic acid, 86.9% for calcium, and 40.1% for iron. The prevalence of PTB was 8.0%.
The results of covariates selection are available in Supplementary Table S2. In the crude model, all four micronutrients were protective factors for PTB. In the adjusted model, compared to the reference group, supplementation with multinutrient (OR = 0.91, 95%CI: 0.85–0.96), folic acid (OR = 0.73, 95%CI: 0.66–0.80), calcium (OR = 0.79, 95%CI: 0.73–0.86) and iron (OR = 0.88, 95%CI: 0.82–0.93) were all inversely associated with PTB (Table 2).
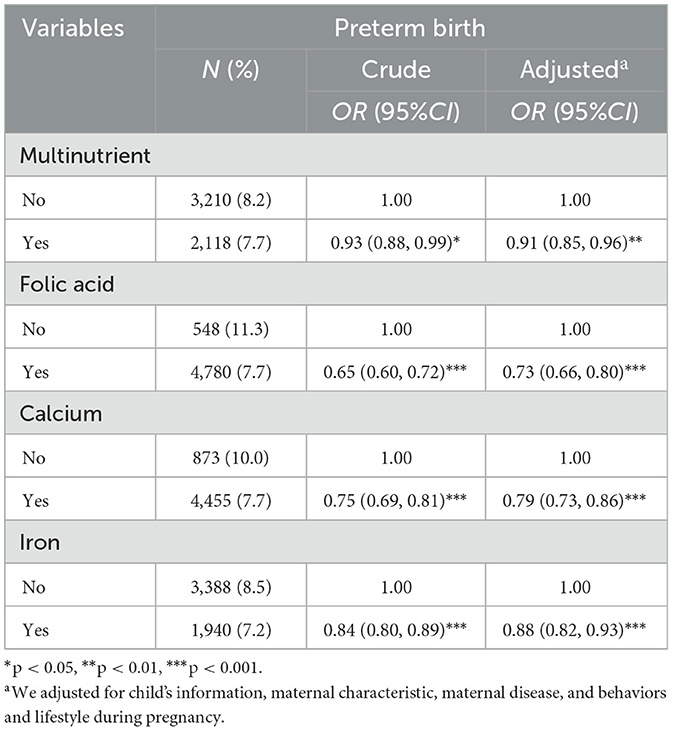
Table 2. Associations between micronutrient supplementation during pregnancy and preterm birth in crude and adjusted model (N = 66,728).
Multivariate logistic regression analyses, controlling for other micronutrients, confirmed folic acid (OR = 0.80, 95%CI: 0.72–0.89), calcium (OR = 0.88, 95%CI: 0.80–0.96), and iron (OR = 0.92, 95%CI: 0.86–0.98) as protective against PTB. However, the protective effects were reduced, and multinutrient showed no significant effect (Table 3).These associations across crude, adjusted, and full-inclusion model analyses were illustrated in Figure 2.
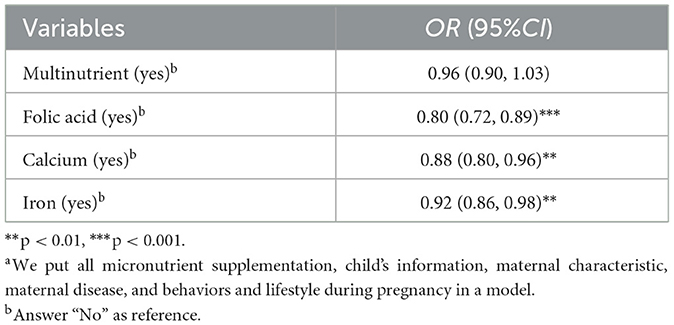
Table 3. Associations between micronutrient supplementation during pregnancy and preterm birth in full-inclusion model (N = 66,728)a.
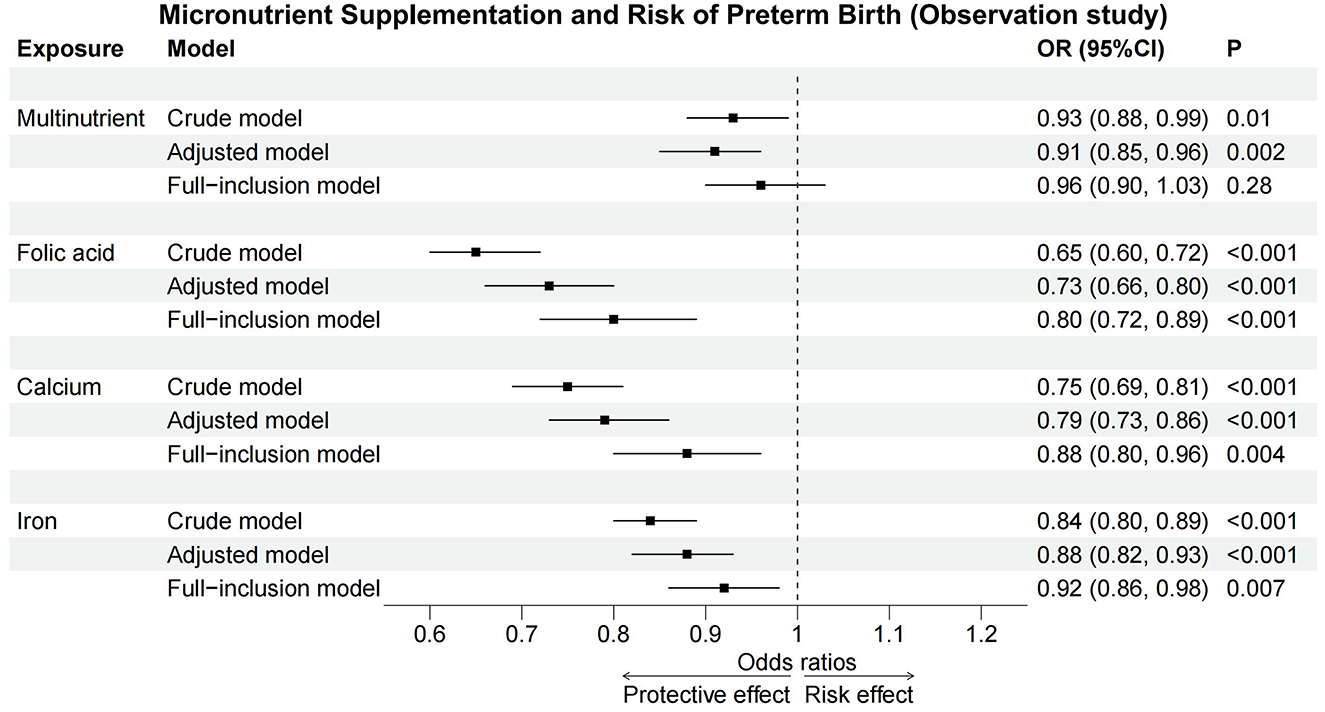
Figure 2. Forest plot of observation study. Across crude, adjusted, and full-inclusion model, folic acid, calcium, and iron acted as protective factors against PTB, while multinutrient supplementation showed no significant effect in full-inclusion model. CI, confidence interval; OR, odds ratio.
To further explore the variation in the protective effect of multinutrient, the co-supplementation characteristics of different micronutrients are presented in Supplementary Table S3. A stratified analysis of the association between multinutrient supplementation and PTB under the other three micronutrient supplementation scenarios revealed a marginal statistical difference between the two folic acid subgroups, suggesting a potential interaction between multinutrient and folic acid (Supplementary Table S4).
3.2 Mendelian randomization and sensitivity analyses
MR analyses revealed a significant causal relationship between calcium intake and reduced PTB risk, with ORIVW of 0.04 (95%CI: 0.004–0.42, p < 0.01, Table 4). After the FDR correction, calcium still significantly associated with lower risk of PTB (pFDR <0.05). For other micronutrients, results were less conclusive. Folic acid and iron supplementation displayed considerable uncertainty in their associations with PTB (ORIVW = 13.14, 95% CI: 0.02–8,436.32 for folic acid; ORIVW = 2.13, 95% CI: 0.01–248.88 for iron), and multinutrients also showed no significant correlation, albeit with marginal significance (ORIVW = 0.01, 95% CI: 0.001–1.26, p = 0.061). Detailed information about the genetic instrumental variables used for MR analysis is presented in Supplementary Table S5.
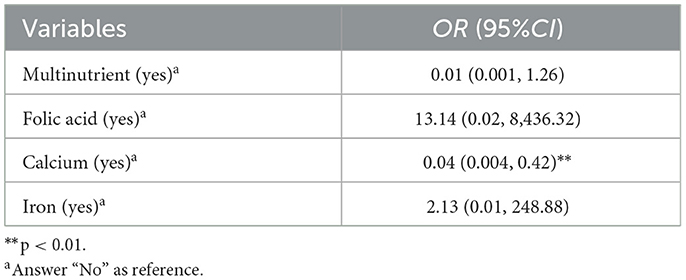
Table 4. Associations between micronutrient supplementation during pregnancy and preterm birth using IVW methods in MR analysis (N = 66,728).
Sensitivity analysis results, highly consistent with the IVW method, are documented in Supplementary Table S6 and illustrated in Figure 3 and Supplementary Figure S1, showcasing a uniform trend across various MR methods. Forest plots and leave-one-out analyses for each SNP indicated no significant bias from outlier SNPs in the association between micronutrient supplementation and PTB (Supplementary Figures S2, S3). Funnel plots and the MR Egger intercept test found no evidence of directional pleiotropy or significant heterogeneity across all micronutrient supplementation analyses (Supplementary Figure S4). According to the Q-test, there is no conspicuous evidence supporting heterogeneity in the results of all micronutrient supplementations. There is also no suggestion of pleiotropy detected by the MR Egger intercept test among exposures. No horizontal pleiotropy was addressed in either the preliminary study or validation of the MR-PRESSO global test (Supplementary Tables S7–S9).
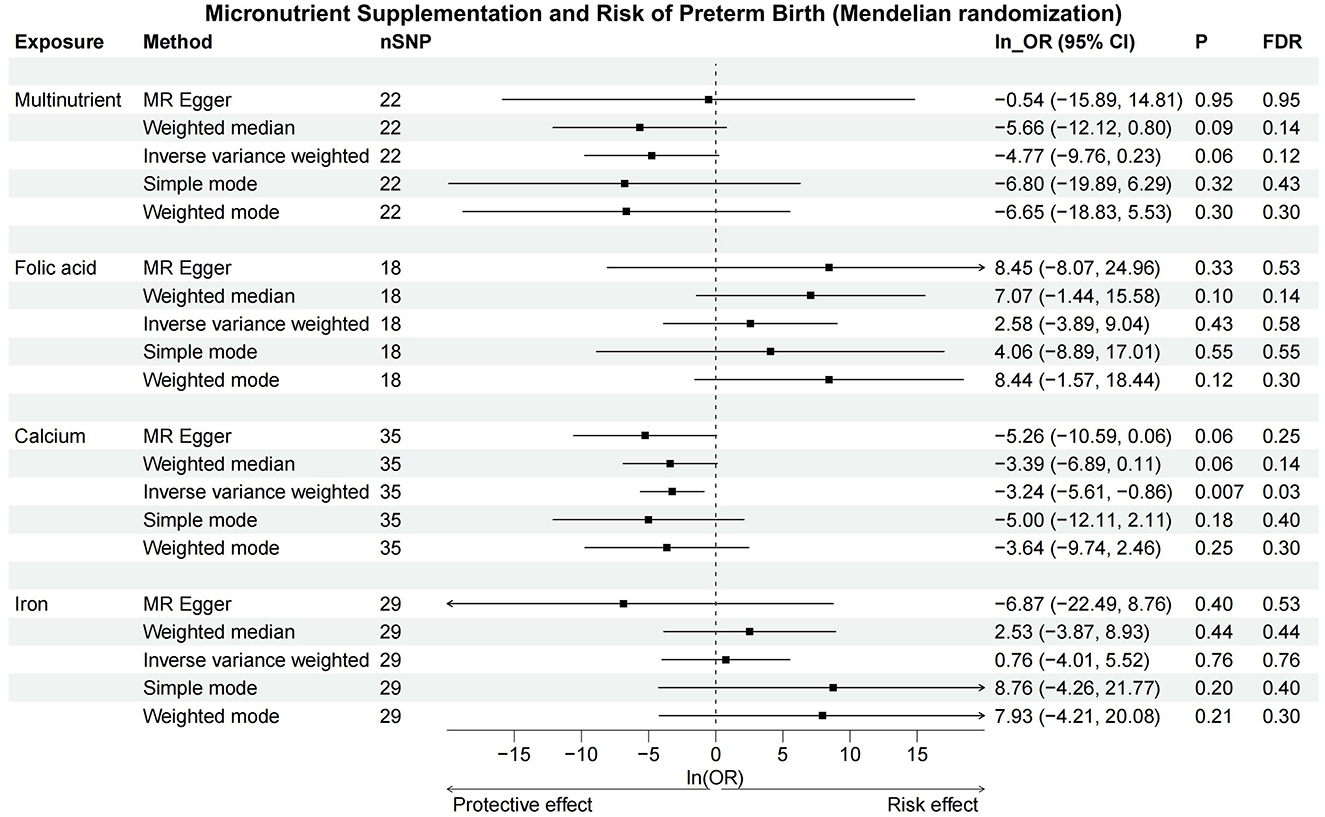
Figure 3. Forest plot of Mendelian randomization. The IVW and weighted median methods indicate a significant reduction in PTB risk with increased calcium intake, but no significant associations were found for multinutrient, folic acid, or iron supplements. CI, confidence interval; FDR, false discovery rate; OR, odds ratio; SNP, single nucleotide polymorphisms.
3.3 Integrated observational and MR findings
Combining observational and MR findings, calcium supplementation demonstrated a significant protective effect against PTB in both analytical approaches. In contrast, folic acid and iron were significant only in observational analyses, while multinutrient supplementation showed no significant association in either analytical approach, as illustrated in Figure 4.
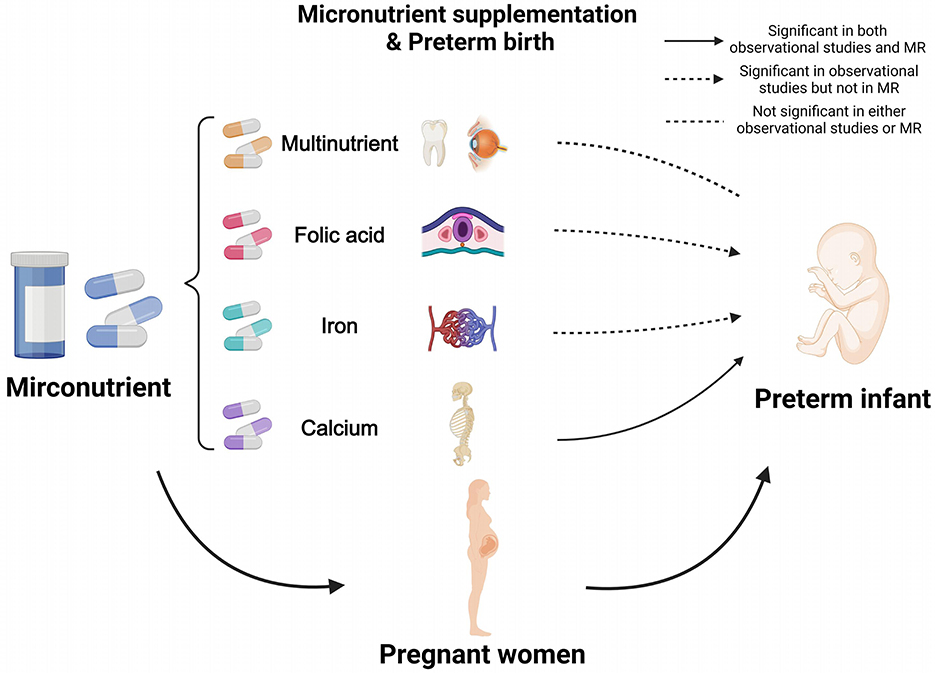
Figure 4. Results of combining observational study and Mendelian randomization. Calcium supplementation showed a significant protective effect against PTB in both analyses, whereas folic acid and iron were significant only in observational analyses, and multinutrient were not significant in either. MR, Mendelian Randomization. Created in BioRender. Ding, R. (2025) https://BioRender.com/itsfbzv.
4 Discussion
In our study based on the 2021 children survey, folic acid, calcium, and iron supplementation were associated with a reduced risk of PTB, particularly in co-supplementation. MR analysis further supported a potential causal relationship between calcium supplementation and lower PTB risk, as indicated by maternal genetic instruments. No significant association was observed between multinutrient supplementation and PTB in either observational or MR analysis.
Similar to our findings, studies have found that not only did multinutrient supplementation fail to significantly reduce the risk of PTB (47–49), but supplementation with individual vitamins, such as vitamins A, B12, and D, also showed no significant association (27–29, 50–52). One possible explanation for these non-significant findings is the heterogeneity among studies and the low doses of vitamins used (53). Interestingly, when measuring the levels of these vitamins in the body, such as 25OHD levels, an association with PTB is often observed (33, 51, 54–56). This discrepancy may stem from the fact that the associations between oral vitamin supplementation and PTB are influenced by confounding factors, whereas vitamin levels measured in the blood are less affected by environmental variables (57, 58). Notably, while crude and adjusted models in our study showed an association between multinutrient supplementation and PTB, this association disappeared after controlling for other micronutrients. This observation aligns with other studies, suggesting that interactions between multinutrient and other micronutrients may drive the observed significant association rather than indicating an independent effect of multinutrient (59). Further high-quality research is needed to better understand these complex interactions in the future.
Folic acid, a water-soluble vitamin B that cannot be synthesized by the body, is significant in neural tube development and is the only perinatal supplement recommended to prevent fetal neural tube defects (60, 61). Observational studies showed that folic acid supplementation reduces the risk of PTB (62), while randomized controlled trials reported no statistically significant effects (63), as did our study. Iron is critical for the delivery of blood oxygen to the fetus, and nearly 40% of pregnant women worldwide suffer from anemia, primarily due to iron deficiency (64, 65). Several meta-analyses included randomized controlled trials showed little or no association between iron supplementation and PTB, as the CI for the pooled effect for PTB just crossed the line of no effect (23, 24). However, other researchers have found that either iron deficiency or excess in pregnant women can lead to PTB, presenting a U-shaped curve for risk associated with iron supplementation (66–68). These results indicate that more prospective controlled trials, as well as more detailed dosage data, are still needed before any firm conclusions can be drawn for these micronutrients.
Calcium is involved in the formation of fetal bones and teeth, as well as in physiological processes such as nerve conduction and muscle contraction. Calcium supplementation may reduce hypertension as well as excessive uterine muscle contractions, as calcium is known to suppress the renin-angiotensin system and decrease the contraction of vascular smooth muscle cells (69–71). Results similar to our results were also seen in cross-sectional and prospective cohort studies (72, 73). Notably, after controlling for other micronutrients, the protective effect of calcium was reduced, suggesting a potential synergistic effect of co-supplementation. However, studies have revealed that simultaneous supplementation of vitamin D and calcium may increase the risk of PTB (53, 59). These contradictory findings warrant careful consideration for pregnant women receiving both vitamin D and calcium supplementation.
MR employs genetic variants as IVs to deduce causal relationships from observational datasets, contingent upon certain prerequisites. These prerequisites include: (1) a robust association of IVs (SNPs) with the targeted exposures; (2) IVs' independence from confounders; and (3) IVs influencing the outcome solely via the exposures (74). MR has the potential to surmount typical observational study challenges, including confounding, reverse causation, and measurement errors (75). Nonetheless, MR findings necessitate prudent interpretation due to possible impacts from heterogeneity, pleiotropy, and GWAS quality. Further research conducted two-sample MR incorporating multiple sensitivity analyses to mitigate these biases (76).
Previous MR study suggested a lack of evidence for a causal relationship between Vitamin D deficiency and PTB, as indicated by an OR (95% CI) of 1.01 (0.93–1.10) (32). This finding is partially corroborated by our MR results concerning multinutrient use. Our MR study further confirmed that calcium intake had a significant causal effect on decreased risk of PTB, as indicated by the negative and significant ORIVW. The results remained stable across various sensitivity analyses, indicating no heterogeneity or pleiotropy. Therefore, in addition to folic acid and iron, which are recommended by the WHO's essential drug list for pregnant women (77, 78), our study also suggests that calcium supplementation may be a potential customized preventive approach for PTB.
There are potential limitations to this study. Firstly, the 2021 children survey utilized a structured questionnaire to collect data retrospectively, which may have introduced recall bias. Micronutrient supplementation was defined using binary self-reported questions by mothers, without collecting detailed information on the doses of specific micronutrients, potentially missing certain dose-response relationships, and information on the components of multinutrient and their interactions. Additionally, the types of micronutrients collected in the survey were limited, which constrained the comprehensiveness of the findings. Future research should include more detailed information such as dosage, specific components, and a wider variety of micronutrients, to provide more comprehensive evidence. Second, the observational study predominantly involved an Asian population, whereas the MR analysis was based on a European population. This potential discrepancy in the population demographics may influence the generalizability of the causal inferences drawn. Third, despite adhering to stringent selection criteria for instrumental variables to confirm the genetic instruments' quality, the current dearth of comprehensive GWAS on PTB limits our findings. Further studies, therefore, should aim for independent validation utilizing GWAS on larger prospective PTB studies to strengthen causal interpretations.
5 Conclusion
In conclusion, our findings indicate that folic acid, calcium, and iron are protective factors against PTB, with MR analysis supporting calcium's causal association with reduced PTB risk. Neither observational nor MR analyses showed a significant effect of multinutrient supplementation on PTB. These results provide a comprehensive understanding of the association between micronutrient supplementation during pregnancy and PTB, and underscore the importance of calcium supplementation in preventing PTB, thereby informing more comprehensive guidelines for pregnant women. Future prospective studies with more detailed information on micronutrients are crucial to draw definitive conclusions about micronutrient prevention of PTB.
Data availability statement
The raw data supporting the conclusions of this article will be made available by the authors, without undue reservation.
Ethics statement
The studies involving humans were approved by the Institutional Review Board of the School of Public Health, Sun Yat-sen University, Guangzhou, China (protocol code: No.2015-016). The studies were conducted in accordance with the local legislation and institutional requirements. Written informed consent for participation in this study was provided by the participants' legal guardians/next of kin.
Author contributions
LD: Writing – original draft, Conceptualization, Data curation, Formal analysis, Visualization. YL: Formal analysis, Visualization, Writing – original draft. XY: Investigation, Resources, Writing – review & editing. GW: Investigation, Resources, Writing – review & editing. DS: Investigation, Resources, Writing – review & editing. DX: Investigation, Resources, Writing – review & editing. YZ: Investigation, Resources, Writing – review & editing. MZ: Data curation, Validation, Writing – review & editing. WY: Project administration, Supervision, Writing – review & editing. WC: Supervision, Writing – review & editing, Project administration.
Funding
The author(s) declare that no financial support was received for the research and/or publication of this article.
Acknowledgments
The authors would like to thank all the participants in the study as well as the clinicians at Maternal and Child Healthcare Hospital of Longhua District, for recruiting participants and collecting data. Additionally, we want to express our gratitude for the drawing materials provided by BioRender.
Conflict of interest
The authors declare that the research was conducted in the absence of any commercial or financial relationships that could be construed as a potential conflict of interest.
Publisher's note
All claims expressed in this article are solely those of the authors and do not necessarily represent those of their affiliated organizations, or those of the publisher, the editors and the reviewers. Any product that may be evaluated in this article, or claim that may be made by its manufacturer, is not guaranteed or endorsed by the publisher.
Supplementary material
The Supplementary Material for this article can be found online at: https://www.frontiersin.org/articles/10.3389/fpubh.2025.1451006/full#supplementary-material
References
1. Lawn JE, Blencowe H, Oza S, You D, Lee AC, Waiswa P, et al. Every Newborn: progress, priorities, and potential beyond survival. Lancet. (2014) 384:189–205. doi: 10.1016/S0140-6736(14)60496-7
2. Ohuma EO, Moller AB, Bradley E, Chakwera S, Hussain-Alkhateeb L, Lewin A, et al. National, regional, and global estimates of preterm birth in 2020, with trends from 2010: a systematic analysis. Lancet. (2023) 402:1261–71. doi: 10.1016/S0140-6736(23)00878-4
3. Perin J, Mulick A, Yeung D, Villavicencio F, Lopez G, Strong KL, et al. Global, regional, and national causes of under-5 mortality in 2000-19: an updated systematic analysis with implications for the Sustainable Development Goals. Lancet Child Adolesc Health. (2022) 6:106–15. doi: 10.1016/S2352-4642(21)00311-4
4. He C, Liu L, Chu Y, Perin J, Dai L, Li X, et al. National and subnational all-cause and cause-specific child mortality in China, 1996-2015: a systematic analysis with implications for the Sustainable Development Goals. Lancet Global health. (2017) 5:e186–e97. doi: 10.1016/S2214-109X(16)30334-5
5. Ramaswamy VV, Abiramalatha T, Bandyopadhyay T, Shaik NB, Bandiya P, Nanda D, et al. ELBW and ELGAN outcomes in developing nations-Systematic review and meta-analysis. PLoS ONE. (2021) 16:e0255352. doi: 10.1371/journal.pone.0255352
6. Sarda SP, Sarri G, Siffel C. Global prevalence of long-term neurodevelopmental impairment following extremely preterm birth: a systematic literature review. J Int Med Res. (2021) 49:3000605211028026. doi: 10.1177/03000605211028026
7. Crump C. An overview of adult health outcomes after preterm birth. Early Hum Dev. (2020) 150:105187. doi: 10.1016/j.earlhumdev.2020.105187
8. WHO. Preterm Birth: Fact Sheet Geneva: World Health Organization (2023). Available online at: https://www.who.int/en/news-room/fact-sheets/detail/preterm-birth (accessed March 10, 2024).
9. Gernand AD, Schulze KJ, Stewart CP, West Jr KP, Christian P. Micronutrient deficiencies in pregnancy worldwide: health effects and prevention Nature reviews. Endocrinology. (2016) 12:274–89. doi: 10.1038/nrendo.2016.37
10. Black RE, Victora CG, Walker SP, Bhutta ZA, Christian P, de Onis M, et al. Maternal and child undernutrition and overweight in low-income and middle-income countries. Lancet. (2013) 382:427–51. doi: 10.1016/S0140-6736(13)60937-X
11. WHO. Global prevalence of vitamin A deficiency in populations at risk 1995-2005: WHO global database on vitamin A deficiency. Geneva: World Health Organization (2009).
12. Akhtar S. Zinc status in South Asian populations–an update. J Health Popul Nutr. (2013) 31:139–49. doi: 10.3329/jhpn.v31i2.16378
13. Lindström E, Hossain MB, Lönnerdal B, Raqib R, El Arifeen S, Ekström EC. Prevalence of anemia and micronutrient deficiencies in early pregnancy in rural Bangladesh, the MINIMat trial. Acta Obstet Gynecol Scand. (2011) 90:47–56. doi: 10.1111/j.1600-0412.2010.01014.x
14. Shamim AA, Kabir A, Merrill RD, Ali H, Rashid M, Schulze K, et al. Plasma zinc, vitamin B(12) and α-tocopherol are positively and plasma γ-tocopherol is negatively associated with Hb concentration in early pregnancy in north-west Bangladesh. Public Health Nutr. (2013) 16:1354–61. doi: 10.1017/S1368980013000475
15. Siddiqua TJ, Ahmad SM, Ahsan KB, Rashid M, Roy A, Rahman SM, et al. Vitamin B12 supplementation during pregnancy and postpartum improves B12 status of both mothers and infants but vaccine response in mothers only: a randomized clinical trial in Bangladesh. Eur J Nutr. (2016) 55:281–93. doi: 10.1007/s00394-015-0845-x
16. Hu Y, Wang R, Mao D, Chen J, Li M, Li W, et al. Vitamin D nutritional status of chinese pregnant women, comparing the Chinese National Nutrition Surveillance (CNHS) 2015-2017 with CNHS 2010-2012. Nutrients. (2021) 13:2237. doi: 10.3390/nu13072237
17. Han T, Dong J, Zhang J, Zhang C, Wang Y, Zhang Z, et al. Nutrient supplementation among pregnant women in China: an observational study. Public Health Nutr. (2022) 25:1537–42. doi: 10.1017/S1368980021001269
18. Chen X, Wu C, Wang Z, Wu C, Guo Y, Zhu X, et al. Iodine nutrition status and thyroid autoimmunity during pregnancy: a cross-sectional study of 4635 pregnant women. Nutr J. (2022) 21:7. doi: 10.1186/s12937-022-00760-6
19. Yang J, Dang S, Cheng Y, Qiu H, Mi B, Jiang Y, et al. Dietary intakes and dietary patterns among pregnant women in Northwest China. Public Health Nutr. (2017) 20:282–93. doi: 10.1017/S1368980016002159
20. Jiang S, Wang J, Duan Y, Pang X, Bi Y, Zhang H, et al. Folic acid status and associated factors for pregnant Chinese women—China, 2015. China CDC weekly. (2021) 3:226–31. doi: 10.46234/ccdcw2021.065
21. Keats EC, Haider BA, Tam E, Bhutta ZA. Multiple-micronutrient supplementation for women during pregnancy. Cochrane Database Syst Rev. (2019) 3:Cd004905. doi: 10.1002/14651858.CD004905.pub6
22. West Jr KP Shamim AA Mehra S Labrique AB Ali H Shaikh S et et al Effect of maternal multiple micronutrient vs iron-folic acid supplementation on infant mortality and adverse birth outcomes in rural Bangladesh: the JiVitA-3 randomized trial. JAMA. (2014) 312:2649–58. doi: 10.1001/jama.2014.16819
23. Peña-Rosas JP, De-Regil LM, Garcia-Casal MN, Dowswell T. Daily oral iron supplementation during pregnancy. Cochrane Database Syst Rev. (2015) 2015:Cd004736. doi: 10.1002/14651858.CD004736.pub5
24. Imdad A, Bhutta ZA. Routine iron/folate supplementation during pregnancy: effect on maternal anaemia and birth outcomes. Paediatr Perinat Epidemiol. (2012) 26:168–77. doi: 10.1111/j.1365-3016.2012.01312.x
25. Zhang H, Wang S, Tuo L, Zhai Q, Cui J, Chen D, et al. Relationship between maternal vitamin d levels and adverse outcomes. Nutrients. (2022) 14:4230. doi: 10.3390/nu14204230
26. Mansur JL, Oliveri B, Giacoia E, Fusaro D, Costanzo PR. Vitamin D: Before, during and after Pregnancy: Effect on Neonates and Children. Nutrients. (2022) 14:1990. doi: 10.3390/nu14091900
27. Mojibian M, Soheilykhah S, Fallah Zadeh MA, Jannati Moghadam M. The effects of vitamin D supplementation on maternal and neonatal outcome: a randomized clinical trial. Iran J Reprod Med. (2015) 13:687–96. Available online at: https://pmc.ncbi.nlm.nih.gov/articles/PMC4695683/
28. Hossain N, Kanani FH, Ramzan S, Kausar R, Ayaz S, Khanani R, et al. Obstetric and neonatal outcomes of maternal vitamin D supplementation: results of an open-label, randomized controlled trial of antenatal vitamin D supplementation in Pakistani women. J Clin Endocrinol Metab. (2014) 99:2448–55. doi: 10.1210/jc.2013-3491
29. Yu L, Guo Y, Ke HJ, He YS, Che D, Wu JL. Vitamin D status in pregnant women in Southern China and risk of preterm birth: a large-scale retrospective cohort study. Med Sci Monit Int Med J Exp Clin Res. (2019) 25:7755–62. doi: 10.12659/MSM.919307
30. Ramakrishnan U, Grant F, Goldenberg T, Zongrone A, Martorell R. Effect of women's nutrition before and during early pregnancy on maternal and infant outcomes: a systematic review. Paediatr Perinat Epidemiol. (2012) 26:285–301. doi: 10.1111/j.1365-3016.2012.01281.x
31. Emdin CA, Khera AV, Kathiresan S. Mendelian randomization. JAMA. (2017) 318:1925–6. doi: 10.1001/jama.2017.17219
32. Cheng H, Chi P, Zhuang Y, Alifu X, Zhou H, Qiu Y, et al. Association of 25-hydroxyvitamin D with preterm birth and premature rupture of membranes: a Mendelian randomization study. Nutrients. (2023) 15:3593. doi: 10.3390/nu15163593
33. Miliku K, Vinkhuyzen A, Blanken LM, McGrath JJ, Eyles DW, Burne TH, et al. Maternal vitamin D concentrations during pregnancy, fetal growth patterns, and risks of adverse birth outcomes. Am J Clin Nutr. (2016) 103:1514–22. doi: 10.3945/ajcn.115.123752
34. Shao Y, Mao B, Qiu J, Bai Y, Lin R, He X, et al. Association between iron supplementation, dietary iron intake and risk of moderate preterm birth: a birth cohort study in China. Iran J Public Health. (2021) 50:1177–87. doi: 10.18502/ijph.v50i6.6416
35. Caniglia EC, Zash R, Swanson SA, Smith E, Sudfeld C, Finkelstein JL, et al. Iron, folic acid, and multiple micronutrient supplementation strategies during pregnancy and adverse birth outcomes in Botswana. Lancet Global Health. (2022) 10:e850–e61. doi: 10.1016/S2214-109X(22)00126-7
36. Papadopoulou E, Stratakis N, Roumeliotaki T, Sarri K, Merlo DF, Kogevinas M, et al. The effect of high doses of folic acid and iron supplementation in early-to-mid pregnancy on prematurity and fetal growth retardation: the mother-child cohort study in Crete, Greece (Rhea study). Eur J Nutr. (2013) 52:327–36. doi: 10.1007/s00394-012-0339-z
37. Alvestad S, Husebye ESN, Christensen J, Dreier JW, Sun Y, Igland J, et al. Folic acid and risk of preterm birth, preeclampsia, and fetal growth restriction among women with epilepsy: a prospective cohort study. Neurology. (2022) 99:e605–e15. doi: 10.1212/WNL.0000000000200669
38. Abreu AM, Young RR, Buchanan A, Lofgren IE, Okronipa HET, Lartey A, et al. Maternal blood pressure in relation to prenatal lipid-based nutrient supplementation and adverse birth outcomes in a Ghanaian cohort: a randomized controlled trial and cohort analysis. J Nutr. (2021) 151:1637–45. doi: 10.1093/jn/nxab018
39. Heaton JL, Campbell SA, Bradley HA, Mulder RT, Dixon L, Henderson J, et al. Broad-spectrum micronutrients or antidepressants for antenatal depression: effect on maternal and infant birth outcomes in an observational secondary analysis of NUTRIMUM. J Clin Psychopharmacol. (2025) 45:4–15. doi: 10.1097/JCP.0000000000001934
40. Allotey PA, Harel O. Multiple imputation for incomplete data in environmental epidemiology research. Curr Environ Health Rep. (2019) 6:62–71. doi: 10.1007/s40572-019-00230-y
41. Lawlor DA. Commentary: Two-sample Mendelian randomization: opportunities and challenges. Int J Epidemiol. (2016) 45:908–15. doi: 10.1093/ije/dyw127
42. Bowden J, Del Greco MF, Minelli C, Davey Smith G, Sheehan N, Thompson J, et al. framework for the investigation of pleiotropy in two-sample summary data Mendelian randomization. Stat Med. (2017) 36:1783–802. doi: 10.1002/sim.7221
43. Bowden J, Davey Smith G, Burgess S. Mendelian randomization with invalid instruments: effect estimation and bias detection through Egger regression. Int J Epidemiol. (2015) 44:512–25. doi: 10.1093/ije/dyv080
44. Bowden J, Davey Smith G, Haycock PC, Burgess S. Consistent estimation in mendelian randomization with some invalid instruments using a weighted median estimator. Genet Epidemiol. (2016) 40:304–14. doi: 10.1002/gepi.21965
45. Hartwig FP, Davey Smith G, Bowden J. Robust inference in summary data Mendelian randomization via the zero modal pleiotropy assumption. Int J Epidemiol. (2017) 46:1985–98. doi: 10.1093/ije/dyx102
46. Verbanck M, Chen CY, Neale B, Do R. Detection of widespread horizontal pleiotropy in causal relationships inferred from Mendelian randomization between complex traits and diseases. Nat Genet. (2018) 50:693–8. doi: 10.1038/s41588-018-0099-7
47. Wolf HT, Huusom LD, Pinborg A, Tabor A, Forman JL, Hegaard HK. Multivitamin intake does not affect the risk of preterm and very preterm birth. Danish Med J. (2020) 67:A11190665. Available online at: https://ugeskriftet.dk/dmj/multivitamin-intake-does-not-affect-risk-preterm-and-very-preterm-birth
48. Vahratian A, Siega-Riz AM, Savitz DA, Thorp JM. Multivitamin use and the risk of preterm birth. Am J Epidemiol. (2004) 160:886–92. doi: 10.1093/aje/kwh305
49. Johnston EO, Sharma AJ, Abe K. Association between maternal multivitamin use and preterm birth in 24 states, pregnancy risk assessment monitoring system, 2009-2010. Matern Child Health J. (2016) 20:1825–34. doi: 10.1007/s10995-016-1985-1
50. McCauley ME, van den Broek N, Dou L, Othman M. Vitamin A supplementation during pregnancy for maternal and newborn outcomes. Cochr Database Syst Rev. (2015) 2015:Cd008666. doi: 10.1002/14651858.CD008666.pub3
51. Rogne T, Tielemans MJ, Chong MF, Yajnik CS, Krishnaveni GV, Poston L, et al. Associations of maternal vitamin B12 concentration in pregnancy with the risks of preterm birth and low birth weight: a systematic review and meta-analysis of individual participant data. Am J Epidemiol. (2017) 185:212–23. doi: 10.1093/aje/kww212
52. Wolf HT, Hegaard HK, Huusom LD, Pinborg AB. Multivitamin use and adverse birth outcomes in high-income countries: a systematic review and meta-analysis. Am J Obstetr Gynecol. (2017) 217:404.e1–e30. doi: 10.1016/j.ajog.2017.03.029
53. Palacios C, Kostiuk LK, Peña-Rosas JP. Vitamin D supplementation for women during pregnancy. Cochr Database Syst Rev. (2019) 7:Cd008873. doi: 10.1002/14651858.CD008873.pub4
54. Bodnar LM, Platt RW, Simhan HN. Early-pregnancy vitamin D deficiency and risk of preterm birth subtypes. Obstet Gynecol. (2015) 125:439–47. doi: 10.1097/AOG.0000000000000621
55. Perez-Ferre N, Torrejon MJ, Fuentes M, Fernandez MD, Ramos A, Bordiu E, et al. Association of low serum 25-hydroxyvitamin D levels in pregnancy with glucose homeostasis and obstetric and newborn outcomes. Endocr Pract. (2012) 18:676–84. doi: 10.4158/EP12025.OR
56. Gernand AD, Simhan HN, Baca KM, Caritis S, Bodnar LM. Vitamin D, pre-eclampsia, and preterm birth among pregnancies at high risk for pre-eclampsia: an analysis of data from a low-dose aspirin trial. BJOG Int J Obstet Gynaecol. (2017) 124:1874–82. doi: 10.1111/1471-0528.14372
57. Perreault M, Moore CJ, Fusch G, Teo KK, Atkinson SA. Factors associated with serum 25-hydroxyvitamin d concentration in two cohorts of pregnant women in Southern Ontario, Canada. Nutrients. (2019) 11:123. doi: 10.3390/nu11010123
58. Al-Musharaf S, Fouda MA, Turkestani IZ, Al-Ajlan A, Sabico S, Alnaami AM, et al. Vitamin D deficiency prevalence and predictors in early pregnancy among Arab Women. Nutrients. (2018) 10:489. doi: 10.3390/nu10040489
59. Zhang J, Bai S, Lin S, Du S, Zhao X, Qin Y, et al. The association between preterm birth and the supplementation with vitamin D and calcium during pregnancy. Clin Nutr ESPEN. (2024) 63:748–56. doi: 10.1016/j.clnesp.2024.08.007
60. Mantovani E, Filippini F, Bortolus R, Franchi M. Folic acid supplementation and preterm birth: results from observational studies. Biomed Res Int. (2014) 2014:481914. doi: 10.1155/2014/481914
61. Vitagliano A, Petre GC, Francini-Pesenti F, De Toni L, Di Nisio A, Grande G, et al. Dietary supplements for female infertility: a critical review of their composition. Nutrients. (2021) 13:3352. doi: 10.3390/nu13103552
62. Zhang Q, Wang Y, Xin X, Zhang Y, Liu D, Peng Z, et al. Effect of folic acid supplementation on preterm delivery and small for gestational age births: a systematic review and meta-analysis. Reprod Toxicol. (2017) 67:35–41. doi: 10.1016/j.reprotox.2016.11.012
63. Saccone G, Berghella V. Folic acid supplementation in pregnancy to prevent preterm birth: a systematic review and meta-analysis of randomized controlled trials. Eur J Obstet Gynecol Reprod Biol. (2016) 199:76–81. doi: 10.1016/j.ejogrb.2016.01.042
64. Kassebaum NJ, Jasrasaria R, Naghavi M, Wulf SK, Johns N, Lozano R, et al. A systematic analysis of global anemia burden from 1990 to 2010. Blood. (2014) 123:615–24. doi: 10.1182/blood-2013-06-508325
65. WHO. Anaemia in Women and Children. Geneva: World Health Organization (2021). Available online at: https://www.who.int/data/gho/data/themes/topics/anaemia_in_women_and_children (accessed March 10, 2024).
66. Yuan X, Hu H, Zhang M, Long W, Liu J, Jiang J, et al. Iron deficiency in late pregnancy and its associations with birth outcomes in Chinese pregnant women: a retrospective cohort study. Nutr Metab. (2019) 16:30. doi: 10.1186/s12986-019-0360-9
67. Lao TT, Tam KF, Chan LY. Third trimester iron status and pregnancy outcome in non-anaemic women; pregnancy unfavourably affected by maternal iron excess. Human Reprod. (2000) 15:1843–8. doi: 10.1093/humrep/15.8.1843
68. Dewey KG, Oaks BM. U-shaped curve for risk associated with maternal hemoglobin, iron status, or iron supplementation. Am J Clin Nutr. (2017) 106:1694s−702s. doi: 10.3945/ajcn.117.156075
69. Bernstein K, Vink JY, Fu XW, Wakita H, Danielsson J, Wapner R, et al. Calcium-activated chloride channels anoctamin 1 and 2 promote murine uterine smooth muscle contractility. Am J Obstetr Gynecol. (2014) 211:688.e1–10. doi: 10.1016/j.ajog.2014.06.018
70. Hofmeyr GJ, Lawrie TA, Atallah Á N, Torloni MR. Calcium supplementation during pregnancy for preventing hypertensive disorders and related problems. Cochrane Database Syst Rev. (2018) 10:Cd001059. doi: 10.1002/14651858.CD001059.pub5
71. Gomes F, Ashorn P, Askari S, Belizan JM, Boy E, Cormick G, et al. Calcium supplementation for the prevention of hypertensive disorders of pregnancy: current evidence and programmatic considerations. Ann N Y Acad Sci. (2022) 1510:52–67. doi: 10.1111/nyas.14733
72. Liu D, Li S, Lei F, Zhao Y, Cheng Y, Dang S, et al. Associations between maternal calcium intake from diet and supplements during pregnancy and the risk of preterm birth in a Chinese population. Eur J Clin Nutr. (2021) 75:141–50. doi: 10.1038/s41430-020-00701-8
73. Mosha D, Liu E, Hertzmark E, Chan G, Sudfeld C, Masanja H, et al. Dietary iron and calcium intakes during pregnancy are associated with lower risk of prematurity, stillbirth and neonatal mortality among women in Tanzania. Public Health Nutr. (2017) 20:678–86. doi: 10.1017/S1368980016002809
74. Bowden J, Holmes MV. Meta-analysis and Mendelian randomization: a review. Res Synth Methods. (2019) 10:486–96. doi: 10.1002/jrsm.1346
75. Zheng J, Baird D, Borges MC, Bowden J, Hemani G, Haycock P, et al. Recent developments in Mendelian randomization studies. Curr Epidemiol Rep. (2017) 4:330–45. doi: 10.1007/s40471-017-0128-6
76. Skrivankova VW, Richmond RC, Woolf BAR, Yarmolinsky J, Davies NM, Swanson SA, et al. Strengthening the reporting of observational studies in epidemiology using Mendelian randomization: the STROBE-MR statement. JAMA. (2021) 326:1614–21. doi: 10.1001/jama.2021.18236
77. WHO. Periconceptional Folic Acid Supplementation to Prevent Neural Tube Defects. Geneva: World Health Organization (2023). Available online at: https://www.who.int/tools/elena/interventions/folate-periconceptional (accessed March 10, 2024).
78. WHO. WHO Model List of Essential Medicines 19th edition. Geneva: World Health Organization (2015). Available online at: https://publichealthupdate.com/who-model-list-of-essential-medicines-april-2015-19th-edition/ (accessed March 10, 2024).
Keywords: preterm birth, micronutrient supplementation, folic acid, iron, calcium, multinutrient, Mendelian randomization
Citation: Ding L, Liu Y, Yin X, Wen G, Sun D, Xian D, Zhao Y, Zhang M, Yang W and Chen W (2025) Association between micronutrient supplementation during pregnancy and preterm birth: evidence from a large-scale children survey and Mendelian randomization study. Front. Public Health 13:1451006. doi: 10.3389/fpubh.2025.1451006
Received: 18 June 2024; Accepted: 17 April 2025;
Published: 09 May 2025.
Edited by:
Xiang Hong, Southeast University, ChinaReviewed by:
Julia Rucklidge, University of Canterbury, New ZealandYuanqing Xia, International Peace Maternity and Child Health Hospital, China
Copyright © 2025 Ding, Liu, Yin, Wen, Sun, Xian, Zhao, Zhang, Yang and Chen. This is an open-access article distributed under the terms of the Creative Commons Attribution License (CC BY). The use, distribution or reproduction in other forums is permitted, provided the original author(s) and the copyright owner(s) are credited and that the original publication in this journal is cited, in accordance with accepted academic practice. No use, distribution or reproduction is permitted which does not comply with these terms.
*Correspondence: Weiqing Chen, Y2hlbndxQG1haWwuc3lzdS5lZHUuY24=; Weikang Yang, eWFuZ3dlaWthbmdAbGhmeXdvcmsuY29t