- 1US Environmental Protection Agency, Research Triangle Park, NC, United States
- 2Department of Environmental Health Engineering, Johns Hopkins Bloomberg School of Public Health, Baltimore, MD, United States
- 3Department of Medicine, West Virginia University, Morgantown, WV, United States
- 4Department of Pathology, Duke University Medical Center, Durham, NC, United States
The retention of occupational and environmental particles in the lung is a primary determinant of biological effects. In the distal respiratory tract, particle clearance includes phagocytosis by alveolar macrophages (AMs), migration to the terminal bronchiole, and transport of AMs and particles by the mucociliary escalator. With increasing particle exposure, a focal collection of particle-laden macrophages results at the respiratory bronchiole (RB) which is that site in the clearance pathway demanding the greatest traverse by these cells after a commencement from the alveoli. With the greatest particle doses, there is “particle overload” and impaired mobility which is reflected by an excess accumulation of particle-laden macrophages throughout the RBs, alveolar ducts, and alveoli. With deposition of fibrous particles in the distal respiratory tract, the AM is unable to extend itself to enclose fibers with a major diameter of 10–20 microns or longer resulting in “frustrated phagocytosis” and longer retention times. Clearance pathways for particles are shared. There can be a summation of particle exposures with exhaustion in the capacity of the AMs for transport. Cigarette smoking (CS) is the greatest particle challenge humans encounter. Associated with its enormous magnitude, CS profoundly impacts the clearance pathways and subsequently interacts with other particle exposures to increase biological effects. Interstitial lung disease, pulmonary function, chronic obstructive pulmonary disease, infections, lung cancer, and mortality can be altered among smokers exposed to occupational and environmental particles (e.g., silica, coal mine dust, air pollution particles, other particles, and asbestos). It is concluded that both decreasing CS and controlling particle exposures are of vital importance in occupational and environmental lung disease.
Introduction
The retention of occupational and environmental particles in the lung is a primary factor in determining their biological effects. Clearance pathways for particles are shared. Cigarette smoking (CS) is currently the greatest particle challenge humans encounter, by mass, with the respiratory tract exposed to between 15,000 and 40,000 μg/cigarette. The mean diameter of this particle is frequently <0.1 micron. Associated with its enormous magnitude, CS will profoundly impact the clearance pathways and subsequently interact with other particle exposures to biological effects.
Particle clearance in the distal respiratory tract
The distribution of ventilation following air entry is affected by a gravitational gradient in the lung and, as a result, is 50% greater at the bases relative to the apices (1–3). Therefore, an increased number of particles is delivered to the lower relative to the upper lung fields. The retention of these particles is dependent on their incomplete clearance (4). The major pathway for clearance of particles deposited in the conducting airways is the mucociliary escalator which is both efficient and rapid (5). Ciliated epithelial cells which participate in this pathway continue to the terminal bronchiole (TB; sometimes designated the 16th generation of airways) but do not extend into the distal airway. The respiratory bronchioles (RBs; sometimes designated the 17th to 19th generations of airways), alveolar ducts (ADs; sometimes designated 20th to 22nd generations of airways) and alveoli (i.e., the acinus) are removed from the mucociliary escalator (although the initial respiratory bronchioles can have some ciliated cells). In the acinus, particle clearance includes (1) phagocytosis by alveolar macrophages (AMs), (2) migration of these cells with the particle along the alveolar and bronchiolar surfaces to the TBs where the mucociliary removal mechanism begins, and (3) transport of the AMs by the moving surface fluid layer (6, 7) (Figure 1A). This clearance pathway is supported by the direct observation of “dust cells” (AMs with phagocytosed particles) on the intraluminal surfaces of airways (8). AM-mediated clearance is slower than that by the mucociliary escalator in the conducting airways and particle retention half-times in the distal respiratory tract are subsequently greater (9, 10). Eventually, the AMs with particle are either conveyed to the gastrointestinal tract via swallowing or expectoration (11).
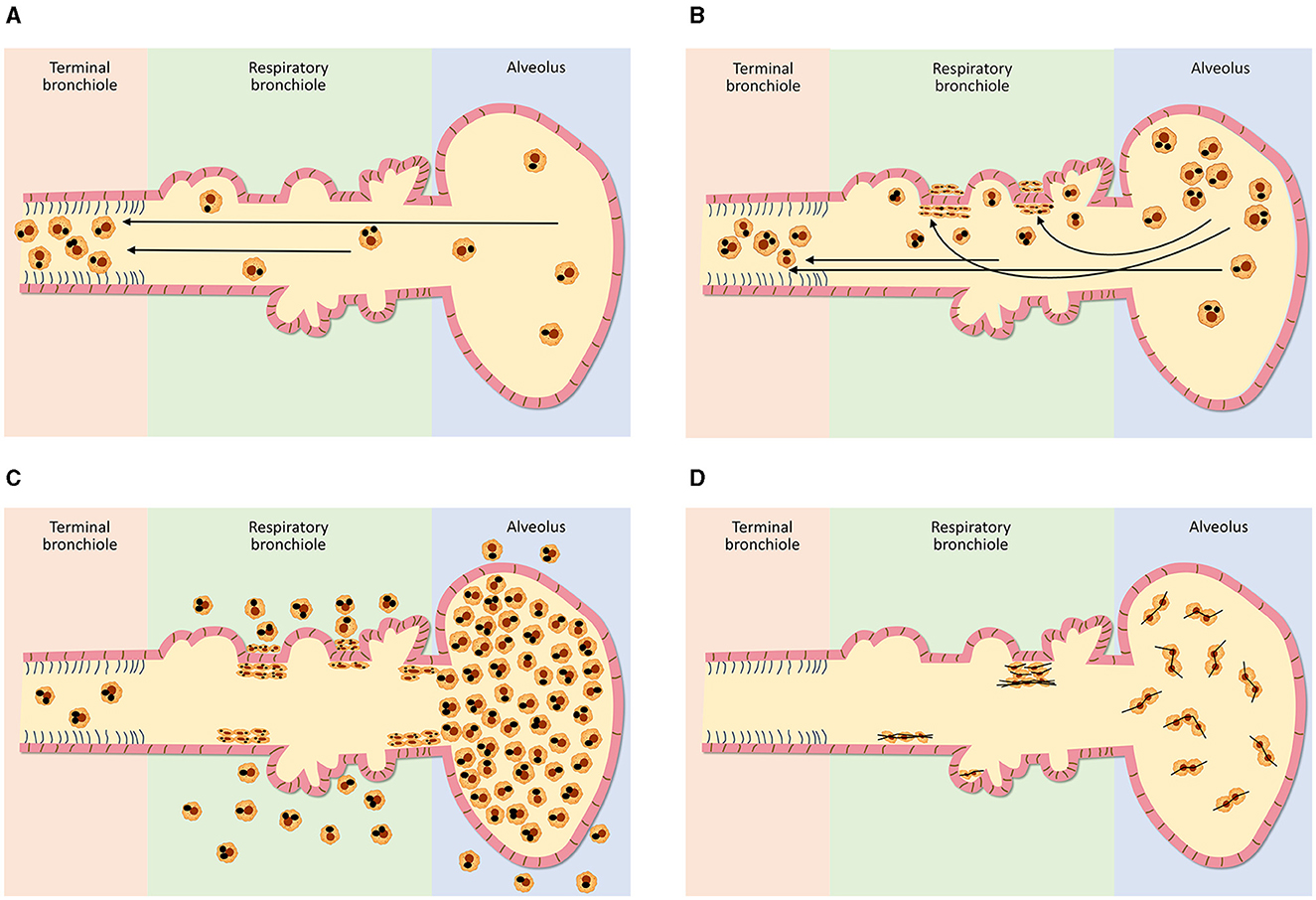
Figure 1. Schematics of clearance from the distal respiratory tract after varying doses of exposures to particles (A–C) and fibrous particles (D). In the distal respiratory tract, particle clearance includes phagocytosis by alveolar macrophages, migration with the particle to the terminal bronchiole and transport of the macrophages, with the particle, by the mucociliary escalator (A). The respiratory bronchiole is the most vulnerable site of this clearance pathway since it demands the greatest traverse of the macrophage and with increasing particle exposure, a focal collection of particle-laden macrophages (i.e., respiratory bronchiolitis) results here (B). With the greatest particle doses, there is “particle overload” and impaired mobility of alveolar macrophages which is reflected by their excess accumulation in the respiratory bronchioles, alveolar ducts, and alveoli (C). With deposition of fibrous particles in the distal respiratory tract, the AM is unable to extend itself to enclose fibers with a major diameter of 10–20 microns or longer (D). Subsequently, there is diminished clearance with this “frustrated phagocytosis” resulting in long retention times.
Particle exposure stimulates a production of mediators recognized to accelerate monocyte release from the bone marrow (12). Blood monocytes accordingly increase in number after particle-associated exposures (e.g., CS, ambient air pollution, diesel exhaust, and traffic-related pollution) (13–17). This is not unique to lung exposures and an intraperitoneal injection of various particles (i.e., silica, kaolin, and polystyrene latex) can be associated with a comparable monocytosis in the peripheral blood (18). An accelerated release of monocytes from the bone marrow after particle inhalation is followed by their recruitment into the lung with differentiation to macrophages (12, 19). Particle exposure accordingly augments the numbers of macrophages recruited into the lungs allowing for their increased participation in clearance from the distal respiratory tract (19, 20).
The effects of gravity on the distribution of blood flow are attributed to the hydrostatic pressure difference between the top and bottom of the lung (2). At the uppermost parts of the lung, the pressure within the vessels may be less than the alveolar pressure, the vessels collapse, and the alveoli receive little to no blood flow (i.e., physiological dead space). In the lower lung zones, pulmonary venous pressure exceeds alveolar pressure. Therefore, perfusion is greatest in the lower lung fields comparable to ventilation (2). With a major source of alveolar macrophages being vascular monocytes, numbers of these cells present in the lung after particle exposure will accordingly be greatest in the lower fields reflecting perfusion (21). Based on a greater availability of monocyte-derived macrophages which phagocytose particles to expedite their removal, more proficient particle clearance in the lower lung fields is predicted.
The magnitude of exposure is a major factor which impacts particle clearance from the distal respiratory tract. As it increases, AM transport of particles from the surfaces of the RBs, ADs, and alveoli to the entry site of the mucociliary escalator (i.e., the TB) is overwhelmed (Figure 1B). The RB is that site in the clearance pathway demanding the greatest traverse by these cells originating in the alveoli and destined for the TB. There results a focal collection of particle-laden macrophages in the region of the RB (i.e., respiratory bronchiolitis; RBitis) following elevated particle exposures (Figure 2). The host response to phagocytosed particles will initially include inflammation but, when prolonged, there will be associated fibrosis with deposits of reticulin/collagen (22–24). When RBitis is associated with evidence of interstitial lung disease (ILD) including diffuse pulmonary infiltrates (typically patchy ground glass opacities on a CT scan) and/or pulmonary function impairment, the disease process is referred to as RB-associated interstitial lung disease (RB-ILD). These inflammatory and fibrotic lesions follow significant exposures most frequently in cigarette smokers but they can be associated with numerous different occupational and environmental particles (25, 26). The mass flow velocity into the airways approaches zero at the RB, augmenting mechanical deposition of particulate matter (27). It is at the level of the RB that maximal dust retention will occur with sheets of dust-laden macrophages which taper in number toward the level of the AD (21). With increased magnitude of the exposure, particles can further accumulate in the same region and histopathologic observations can include RBitis, macules, nodules, and simple pneumoconiosis (e.g., silicosis, coal workers' pneumoconiosis, kaolinosis, and talcosis) (28). Particle type will also affect the histopathology. Miners can show a high prevalence of simple pneumoconiosis, an exaggerated form of an RB-ILD with deposition of dust, macrophages, and connective tissue (Figure 3A) (29). The distribution of these lesions is symmetrical involving both lungs and most frequently with a preponderance in the upper lobes (Figure 3B). Toward the bases, these lesions tend to diminish in size and number. Such a conventional presentation of simple pneumoconiosis is consistent with particle clearance being most successful in the lower lung fields. These lesions can coalesce and while most often observed with silica and coal dust, the resultant large mass (i.e., complicated pneumoconiosis) can be observed after exposures to numerous, different particles. With the development of RBitis, macules, nodules, and simple pneumoconiosis, the clearance system is still effective in diminishing particle accumulation in the most distal respiratory tract where the exchange of oxygen and carbon dioxide occurs. Therefore, pulmonary function is usually normal in patients with simple silicosis and coal workers' pneumoconiosis and even with complicated disease.
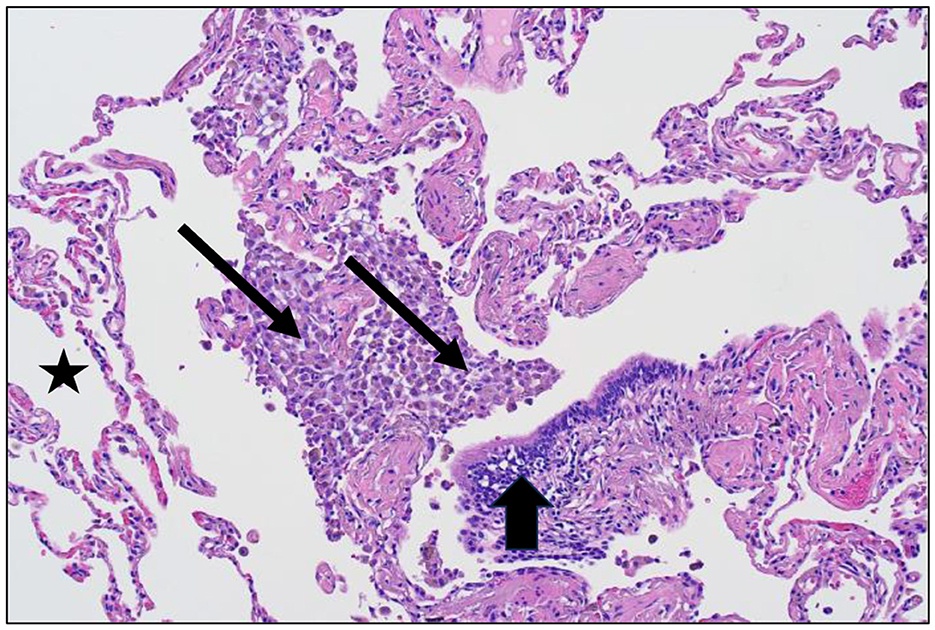
Figure 2. Lung tissue from a cigarette smoker demonstrating respiratory bronchiolitis after particle exposure (hematoxylin and eosin stain; magnification of approximately 100x). There is an accumulation of macrophages and pigmented particles evident at the respiratory bronchiole (designated by arrows) but not in the alveolar region (designated by a star) and the terminal bronchiole (designated by an arrowhead).
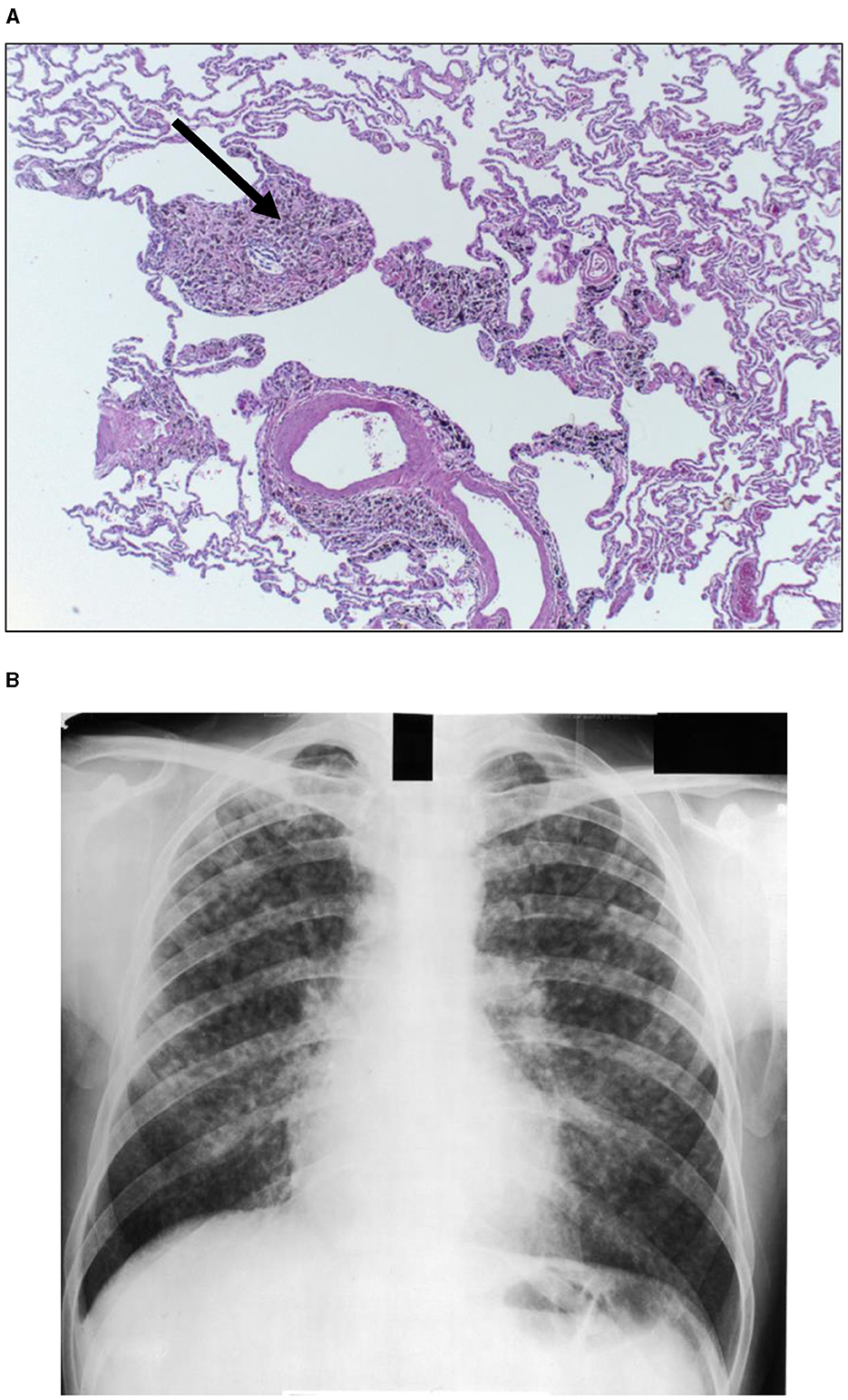
Figure 3. Human lung tissue from a coal miner reveals accumulation of particle in the region of the respiratory bronchiole recognized as a coal macule (designated by arrow) [(A); hematoxylin and eosin stain; magnification of approximately 100x]. After accretion and coalescence, these collections of macrophages, particles, and associated inflammation and fibrosis can be observed as a simple pneumoconiosis on a chest X-ray (B). Lesions are symmetrical involving both lungs and a preponderance in the upper lobes is noted.
With those particle exposures which are most extreme in magnitude, there can be a “particle overload” of the distal respiratory tract. With “particle overload,” there is a reduction in AM mobility associated with an impairment of clearance by these cells (30) (Figure 1C). Subsequently, the hallmark of the “particle-overloaded” lung is altered retention kinetics with the half-times increasing linearly with the burden (30–33). Changes in AM function with “particle overload” have been attributed to augmented particle mass, volume, and/or surface area (31, 32, 34–39). This is reflected by an excess accumulation of particle-laden macrophages frequently observed diffusely distributed throughout the RBs, ADs, and alveoli. These lesions become large, and irregular (often stellate). The histopathology is consistent with a desquamative interstitial pneumonitis (DIP) which is defined to include an alveolar accumulation of particle-laden macrophages (Figure 4). This can be observed with CS, passive smoking, and other particles and are associated with interstitial inflammation and fibrosis (40).
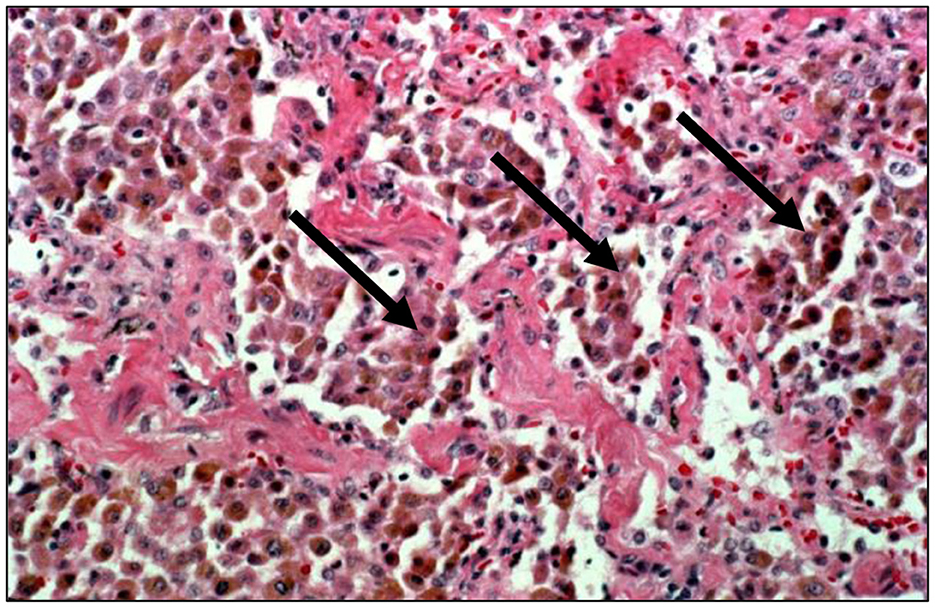
Figure 4. Human lung tissue from a smoker reveals an accumulation of macrophages in the alveolar region (hematoxylin and eosin stain; magnification of 100x). With extreme particle exposures, “particle overload” of the distal respiratory tract is reflected by an excess accumulation of particle-laden macrophages in the acinus (designated by arrows). This is pathologically recognized as desquamative interstitial pneumonitis. With smoking, the macrophages can contain fine brown granules supporting a failure of particle-laden macrophages to enter the mucociliary escalator.
An inability of the AMs to eliminate particles from the distal respiratory tract recruits alternative routes or pathways of clearance. The accumulation of AMs in the distal respiratory tract accelerates their migration with phagocytosized particles into the interstitium and subsequent transport to lymphatics, lymph nodes, and pleura (41). Particle-laden AMs penetrate the pulmonary interstitium (8, 41). AMs with phagocytosed particles then accumulate in bronchus-associated lymphoid tissue, migrate to peribronchial and perivascular lymphatics, and are transported (1) antegrade to regional lymph nodes (41–43) and (2) retrograde to the pleura (44). In animal models, AMs with phagocytosed particles can be identified in the pulmonary interstitium (45, 46), intravascular and sub-epithelial locations in the bronchioli (47), and in draining lymph nodes (45, 48). Particle numbers increase in the thoracic and retroperitoneal lymph nodes, accordingly, these appear black in smokers and coal miners (49). The lymphatics also transport particles retrograde to sub-pleural areas with access to the pleural space. While there are no direct connections with the lung, specific areas of the parietal pleura absorb and retain high concentrations of particles from the pleural space. These particle-collecting structures have been called “black spots” which can be observed in 92.7% of urban autopsies (50, 51). “Black spots” of the pleura develop in close correlation to lymphatic channels and blood vessels. AMs accordingly can redistribute a particle burden across the epithelium into the interstitium, lymphatics, lymph nodes, and pleura (6, 41). Inflammation and fibrosis can result wherever the particle is retained. In addition to pneumoconiosis in the lung parenchyma, inflammation and fibrosis can also impact the lymph nodes and the pleura. With particle exposures, lymphadenopathy with both inflammation and fibrosis is observed (42, 52–58). In the pleura, “black spots” are characterized by an inflammatory reaction to the incorporated particles and a fibrosis comparable to lymph nodes (59–61). Increased particle burdens at “black spots” following occupational exposures to mixed dusts may affect macrophage function (i.e., transport and phagocytosis) (59).
Cigarette smoking and particle clearance
The biological effects after smoking can be related to particle exposure (62–65). The mean diameter of CS particles is 0.2–0.5 μm and the deposition fraction is projected to be 70%−90% with the greatest of this occurring at the RBs (66, 67). CS accelerates a release of monocytes from the bone marrow and these are recruited to differentiate to macrophages accounting for increased numbers in the smoker's lung and allowing for their participation in clearance from the distal respiratory tract (12, 19, 21). Despite this, particle clearance was demonstrated to be impaired in smokers with 50% retention compared to 10% in non-smokers (68). Exposure to CS impacted mucociliary clearance with decreases in both ciliated cell numbers and ciliary beat frequency (69). Accordingly, CS decreases the efficiency of clearance from both the alveolar and bronchial compartments and smokers retain more particles than non-smokers (70).
CS is the paramount particle exposure and it is frequently associated with a “particle overload.” Since AMs normally direct the clearance process in the distal respiratory tract, pathways are overwhelmed with CS and particles are not successfully removed from the RBs, ADs, and alveoli; particle retention results (30). Histopathologically, smoking is characteristically by an accumulation of AMs containing fine brown granules which do not polarize light in the distal respiratory tract (Figure 4). There can be varying amounts of fibrosis in smoking-related RBitis, RB-ILD, and DIP (71). RBitis is a universal response in all smokers (i.e., smoker's bronchiolitis) characterized histologically by a patchy accumulation of pigmented, smoker's macrophages in the RB (72–75). This reflects the failure of particle-laden AMs to enter the mucociliary escalator at the TB. Loss of epithelial ciliation as well as alterations in lung architecture such as observed in chronic obstructive pulmonary disease (COPD), as well as other chronic lung diseases after long-term smoking can further particle retention. With continued smoking, greater particle deposition initiates an inflammatory and fibrotic response consistent with DIP; CS is associated with most DIP cases (76, 77). While the feature that differentiates RBitis from DIP is most frequently cited as the distribution and extent of macrophage accumulation (bronchiolocentric in RBitis and diffuse throughout the entirety of the RBs, ADs, and alveoli in DIP), there are no reliable histologic features to distinguish the two responses with complete certainty and they can be considered separate phases of a single response (78). Smoking cessation is currently considered the primary treatment for both RBitis and DIP and survival rates are high. This inflammatory response can persist after smoking cessation as CS particle persists in the lung for years (79–82).
With an absence of effective clearance pathways associated with “particle overload,” the biological response (inflammation and fibrosis) with CS will be evident in the lower lung fields where the ventilation, and exposure, is greater relative to the upper lung fields. Subsequently, radiographic evidence of smoking is recognized to include opacities in the lower lung fields reflecting the inflammation and fibrosis in response to CS particle (83–89). In the last two decades, interstitial lung abnormalities (ILAs) have been observed on 8%−20% of smokers' CT scans screened for lung cancer and without clinical symptoms (90–95). On pathological examination, ILAs include both inflammation and fibrosis (95, 96). These ILAs are a precursor to clinically evident smoking-related lung fibrosis.
Evidence supports a causative relationship between smoking and lung inflammatory and fibrotic disease (72, 77, 82, 97–103). Before chest X-rays and CT scans of the chest demonstrate any abnormalities, inflammation and fibrosis can be observed histologically in most smokers (96). This chronic pulmonary inflammation and fibrosis can progress and manifests as several different histologic patterns: (1) organizing pneumonia (OP), characterized by round or oval pale-staining deposits consisting of fibroblasts, myofibroblasts, collagen, and fibrin within RBs, alveolar ducts and alveoli; (2) nonspecific interstitial pneumonia (NSIP), characterized by inflammation and/or fibrosis in the lung interstitium occurring in a spatially homogeneous pattern and with preservation of overall lung architecture; and (3) usual interstitial pneumonia (UIP), the most severe form of lung fibrosis, characterized by heterogeneous areas of dense fibrosis interspersed with areas of relatively normal lung architecture, fibroblastic foci, and honeycombing (82, 97, 101, 104–111). OP and NSIP can represent earlier phases of the response to CS while UIP represents a response to greater doses (pack-years) and subsequently a later stage. One histologic presentation of injury may progress into the next with overlapping patterns being observed which make strict histopathologic diagnosis difficult or impossible (112, 113). OP components are common lesions in both NSIP and UIP cases, and as fibrosis evolves, pathological areas of NSIP are observed with UIP (114–119). It is the retention of particle in the distal respiratory tract with CS which is proposed to impact a dose-response fibrosis (120).
In smokers, excessive accumulation of AMs accelerates their “interstitialization” and migration into lymphatics, lymph nodes, and pleura. With the biological effects after smoking being related to particle exposure, inflammation and fibrosis then occur in the interstitium, bronchus-associated lymphoid tissue, peribronchial and perivascular lymphatics, regional lymph nodes, and pleura as well as the distal respiratory tract. Enlarged mediastinal lymph nodes occur in over 50% of heavy smokers (43).
Smoking and disease after exposure to silica
Comparable to all particles, the clearance of silica in the distal respiratory tract includes phagocytosis by AMs with transport to mucociliary escalator at the TB. With exposure to silica, there can be an RBitis which progresses to RB-ILD and pneumoconiosis (simple and complicated). In silicosis, the upper lobes reveal greater involvement since particle clearance is more effective at the bases. With greater exposures to silica, AM mobility will be impaired and the particle may not be successfully translocated to the TB. This sequence suggests a dose-response in which there is an initial increase in the accumulation of particles removed from the distal respiratory tract to the region of the RB (i.e., pneumoconiosis) followed by patterns of involvement reflecting an injury including DIP; idiopathic cases of DIP demonstrate a history of particle exposure including silica (121). In addition, alternative routes or pathways of clearance (e.g., interstitium, lymphatics, lymph nodes, and pleura) are recruited at higher exposure levels and other forms of inflammatory and fibrotic lung disease can be evident (e.g., OP, NSIP, and UIP as well as sarcoidosis) (122, 123).
Collagen deposition and fibrosis increase with particle retention in the lung and the failure of clearance pathways including alveolar macrophages to remove particle from the distal respiratory tract. A dose response of fibrotic lung injury with several different particles has been demonstrated (e.g., silica, coal mine dust, and asbestos) (124). Dissimilarities between dose-response of these particles is also evident (e.g., silica generates a greater fibrotic response relative to coal mine dust).
Interactions are described between smoking and silica exposure and these can vary widely depending on the specific endpoint (Table 1) (125). Smoking increases the total particle delivered to the distal respiratory tract and clearance mechanisms are subsequently overwhelmed. Smokers exposed to silica dust have been observed to develop silicosis more frequently than non-smokers exposed to the same dose (126–129). In one study, 85% of silicosis cases were ever smokers (vs. 70% in controls) (130). In the pottery and stone benchtop industries, silicosis was associated with smoking (131, 132). In these investigations, the types of opacities observed on the chest X-ray were not specified while others analyzed (1) only rounded opacities consistent with conventional silicosis or (2) both rounded and irregular opacities. Many of the opacities in silicosis among smokers were irregular and observed in the lower lung fields which is not considered consistent with the appearance of conventional silicosis (133, 134). Animal studies support an interaction between smoking and silica with greater biological effect initiating lung inflammation and fibrosis after combined exposures (135).
With a summation of particles in CS and silica exposure, there is an exhaustion in the capacity of the AMs for transport. Subsequently, the pattern of biological effect can change. In smokers, exposure to silica may not be associated with nodules and rounded opacities in the upper lung fields. Rather, opacities after silica exposure in smokers may be in the lower lung fields where most of the inhaled breath with particles is distributed. While smoking can increase incidence of silicosis, it might also alter the natural history of silicosis. In support of this, a slight inverse relationship between smoking and collagenization of the parenchyma was observed (after controlling for age and cumulative exposure to silica dust) among deceased miners who underwent postmortem examination between 1976 and 1981 (136). While smoking could decrease the incidence of pneumoconiosis, the silica would accumulate in different sites; these would be more distal and contribute to other types of inflammation and fibrosis (e.g., OP, NSIP, and UIP). Such patterns of fibrotic lung injury have been observed after silica exposure and almost all were among smokers (137–139).
Like numerous particle exposures, silica is associated with pulmonary function loss (140). The estimated excess loss of lung function for a 50-year-old gold miner exposed to silica associated with 24 years of underground dust exposure at an average respirable dust concentration of 300 μg/m3 was 236 mL of forced expiratory volume in 1 second (FEV1) and 217 mL of FVC (forced vital capacity) (141). By comparison, the effect of smoking one packet of cigarettes a day over 30 years was associated with an estimated loss of 552 mL of FEV1 and 335 mL of FVC in this same study. Interactions between smoking and silica exposure can be observed with potentiation of the effect of silica on pulmonary function loss, rates of airway obstruction, and prevalence of COPD (142–146). CS was found to potentiate the effect of dust, predominantly silica, on respiratory impairment in gold miners (147). Those with silicosis had greater rates of COPD with smoking (148). The two particles also interacted to impact the capacity of AMs to eliminate respiratory pathogens in workers substantiating a critical role for these cells in the prevention of infection (149, 150). Current smokers had a significantly higher risk of tuberculosis than other patients with silicosis (125, 151, 152). A negative relationship between CS and collagenization of the pleura was observed among deceased miners on postmortem examination suggesting an impact of smoking on lymphatic clearance to the pleura (136). While there is a dose-response between the cumulative exposure to silica and lung cancer among never-smokers, 91% of silica-related lung cancer cases were ever smokers (vs. 69% controls) (130, 153–155). The joint effects of smoking and silica on lung cancer incidence have been additive, super-additive, multiplicative, and even supra-multiplicative (153, 156). Excess lung cancer mortality in silica-exposed workers was restricted to those with silicosis, and the more severe the disease, the higher the neoplastic risk (153, 157, 158). One investigation demonstrated that most of the excess lung cancer risk in patients with silicosis was due to smoking, but a synergistic effect between smoking and silica/silicosis was likely (159). Finally, smoking increases mortality following silica exposures with interactions between silica and smoking increasing deaths after pulmonary diseases including pneumoconiosis and COPD (142, 143, 160, 161).
Smoking and disease after exposure to coal mine dust
The fundamental lesion of coal workers' pneumoconiosis consists of a focal aggregation of coal mine dust (CMD)-laden AMs that accumulate in the RB and TB (28). This collection of dust-laden AMs, reticulin and collagen (1–4 mm in diameter) is referred to as a coal dust macule; these are non-palpable and distributed throughout the lung with greater numbers in the upper lung fields (162). The coal macule is a RBitis. With increased exposure to CMD, AMs with particles accumulate to extend into adjacent regions as well as into the peribronchiolar interstitium, the size of the coal macule increases (some by accretion until they become visible whereas others coalesce with adjacent nodules to become macroscopically visible), and these eventually become fibrotic nodules of simple coal workers' pneumoconiosis (29). This simple pneumoconiosis demonstrates a centrilobular (centered around the RB) deposition of coal dust, AMs, inflammation, and connective tissue. Complicated lesions of coal workers' pneumoconiosis (defined pathologically and radiologically as nodules measuring 1 cm or more in diameter) follow the fusion of small opacities, an accretion and incorporation of adjacent nodules. Mediastinal and hilar adenopathy can be present but this is usually in less than one-third of the cases. Exposure to CMD can cause DIP with particle-laden (anthracotic and silica/silicate) AMs accumulated in the distal respiratory tract (163). This advances to o types of inflammation and fibrosis (e.g., OP, NSIP, and UIP). Cases of a UIP pattern of lung fibrosis with honeycombing on the CT scan was reported among coal miners (pathology was available in 2 explanted lungs and 6 open lung biopsies) (164).
The research into interactions between coal mine dust and smoking has been limited (Table 2). Early investigation suggested no influence of smoking on the coal dust macule and its direct complications; coal miners who did smoke had a greater degree of corpulmonale, more emphysema, and more bronchiolar goblet cells with chronic bronchiolitis than non-smoking coal miners (165). Coal miners with primarily irregular opacities showed a lower zone preponderance (166). In investigation, irregular opacities on radiographs of South Wales coal workers were related to age, smoking and coal work exposure (167). Similarly, irregular small opacities on the radiographs of coal miners in the USA were associated with smoking, age, years worked underground, and a diagnosis of bronchitis (168). There is a description of chronic interstitial pneumonia with honeycombing in coal miners (164). Among the 38 miners included in this report, 32 were smokers. Dust-related diffuse fibrosis was described recently in a significant number of autopsies of coal workers (15% to 20%) (169, 170). Dust-related diffuse fibrosis can resemble idiopathic pulmonary fibrosis (IPF), which is associated with smoking, but has a better prognosis. 44/45 of miners with diffuse ILD were smokers.
An interaction between smoking and CMD on pulmonary function has been demonstrated. Combined effects of smoking and CMD exposure on pulmonary function were significantly greater than that of the CS only and cumulative total exposure only (171). CMD positively correlated with abnormal rates of pulmonary function in both the smoking and non-smoking subgroups; one index (i.e., the FEV1) was negatively correlated with total dose only in the smoking subgroup (172). Spirometric indices (i.e., forced expiratory volume in one second and the ratio) were lower among those coal miners with irregular opacities possibly reflecting CS (168). Miners with coal workers' pneumoconiosis demonstrated greater rates of COPD with cigarette smoking (148). While there is little evidence of a CMD exposure-lung cancer risk, lung nodules among smokers with coal workers' pneumoconiosis had a higher risk of being lung cancer (173, 174). Finally, smoking increased mortality of those coal miners with complicated pneumoconiosis (175).
Smoking and disease after exposure to air pollution particles
Ambient air pollution particles are retained in the human lung with greater concentrations (number counts) observed in the RBs which accumulated high particle loads, typically 25–100 times the concentrations seen in the mainstem bronchus (176). The mean diameter of this air pollution particle was 0.38 micron. Using analytical electron microscopy, particles retained in autopsy lung tissue from 10 never-smokers were shown to include silica, silicates (kaolin, feldspar, mica, and talc), and metals (176, 177); the majority of these particles appeared to be crustal in origin. However, examination of human lungs confirmed that airways could also retain relatively large numbers of carbonaceous chain aggregates of particles (ultrafine in size) that appeared to be combustion products (178). Black pigmented particles accumulated in the non-smoker's lung with increasing age and this was attributable to soot in the ambient air originating from the burning of coal and wood (179). Emission source air pollution particle (e.g., environmental tobacco smoke) was associated with a RBitis comparable to other particles (25, 26). Air pollution particles were also observed in the peribronchial lymph nodes (forming “black glands”) (179). Disease after air pollution particles included a diffuse pulmonary fibrosis with exposures to combustion products of biomass fuels used for cooking and heating in poorly ventilated huts (180).
There was an interaction between air pollution and smoking observed in decreasing pulmonary function (181) (Table 3). Synergistic interactions between air pollution, specifically including particles, and smoking in contributing to cardiovascular mortality were observed in additional epidemiological investigation (182, 183). In smokers, there was a significant excess risk of daily mortality associated with ambient air pollution particles for all natural causes and cardio-respiratory diseases for men aged 30 years or older and men 65 or older (184). Mortality risks caused by all natural causes and cardiorespiratory diseases per 10 μg/m3 change in black smoke were higher in current smokers than never smokers (185). Ever-smokers were again more susceptible to excess mortality risk associated with daily air pollution (186).
Smoking and disease after exposure to other particles
Vermiculite and smoking interact to increase the interstitial markings on the chest X-rays of miners (187). The prevalence of opacities on the chest radiographs of talc millers could similarly be increased among smokers (188). Flour dust also interacts with smoking to impact an ILD observed on the CT scan (189). Regarding pulmonary function testing, the effects of cotton dust exposure and cigarette smoking on spirometry endpoints appeared to be additive (190–192).
Asbestos clearance in the distal respiratory tract
Fibrous morphology has been identified as a major characteristic in determining clearance (193). With deposition in the distal respiratory tract, the AM is unable to extend itself to enclose longer fibers (those with a major diameter of 10–20 microns or longer) resulting in incomplete or “frustrated phagocytosis”, long retention times, and persistent biological effect (e.g., inflammation, fibrosis, and neoplasia) (194) (Figure 1D). Therefore, fiber dimensions are a major determinant in the biological effect of asbestos (195). The risk for biological effect of asbestos correlates with fiber length (196, 197). Asbestos relevant to fibrosis and cancer can include those longer than 5 microns (198). Short fibers (those < 5 μm) are fully engulfed (internalized) by macrophages and removed by lung clearance more efficiently (193, 199–202). Accordingly, while short fibers may also be associated with biological effects, these are observed most frequently only at greatly increased exposure levels (203, 204).
Asbestos exposure is associated with both RBitis and DIP (205–207). With ineffective clearance, disease associated with asbestos occurs at much lower levels of exposure relative to other particles such as silica and coal (208). Subsequently, the exposure (the dose of dust retained in the lungs) is far less in asbestosis (i.e., milligrams) relative to silicosis and coal workers' pneumoconiosis (i.e., grams or tens of grams) (209, 210). In addition, the radiographic and pathologic appearance of asbestosis are distinct from other pneumoconioses associated with particle exposure (e.g., silicosis and coal workers' pneumoconiosis). Asbestosis is characterized by the presence of small irregular opacities, which are bilateral and commonly involve the lower lobes of the lungs (205, 211–216) (Figure 5). This presentation is the result of the particle being distributed to the lower lung fields and an ineffective transport from the distal respiratory tract to the entry site of the mucociliary escalator (i.e., the TB). For this same reason, asbestos exposure is not associated with complicated pneumoconiosis.
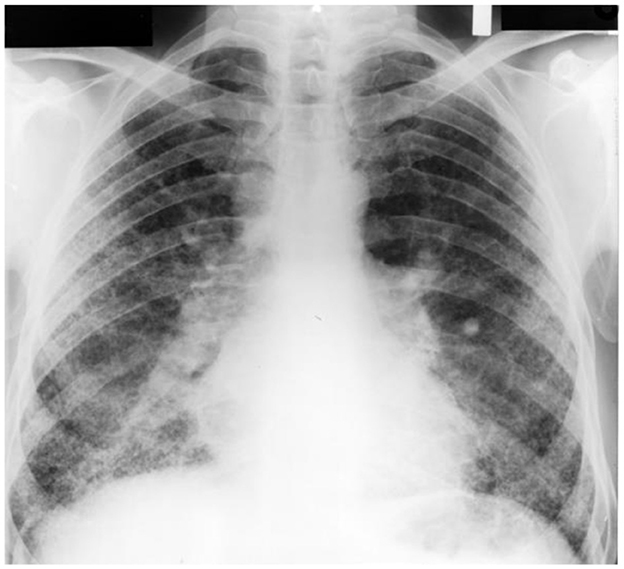
Figure 5. A chest radiograph of inflammatory and fibrotic injury following asbestos exposure. This is characterized by bilateral, small irregular opacities involving the lower lobes of the lungs. The dimensions of asbestos preclude efficient clearance (“frustrated phagocytosis”). Subsequently, in contrast to particles, the associated inflammation and fibrosis is in the lower lung fields where delivery is greater relative to the upper lung fields.
The distribution of mineral fibers in the lung determines the site and severity of disease (217). With decreased mobility of the AM following “frustrated phagocytosis,” asbestos is translocated to the interstitium where it is transported via lymphatics antegrade to the lymph nodes and retrograde to the pleura and the peritoneum (218, 219). As a result, thoracic lymph node enlargement occurs frequently with asbestos exposure (220). From the lungs, asbestos fibers can migrate to pleural and peritoneal spaces following patterns of lymphatic drainage. In addition, a proportion of asbestos that reaches the pleura will pass through the pleural space and exit through the stomata. Subsequently, the fibers are sequestered in the “black spots” in the parietal pleura. Fibers that reach the pleural space can interrupt flow with interception of the asbestos within the walls of the stomatal openings as well as in the lymph vessels walls. This increases an accumulation of pleural macrophages attempting to phagocytose retained, long fibers. The most frequently documented pleural response to fibers are effusions (i.e., inflammation) and plaques (i.e., fibrosis). The latter are dense bands or weaves of collagen, frequently calcified, and occur on the parietal pleura at sites where the stomata are in greatest profusion. The pleural response is observed with numerous particle exposures with the severity being dependent on the biological activity of the dust (i.e., carbonaceous < mixed mineral dust < silica < short asbestos < long asbestos). Pleural plaques are a special case of a “black spot” caused by fibers eliciting an extreme collagenous response. Finally, there is a risk of cancer (mesothelioma and lung cancer in the pleura and lung respectively) among workers exposed to asbestos which increases with exposure to longer fibers (200, 221–224). Animal investigation supports that longer fibers (>10 μm) are more carcinogenic to the lung (221).
Smoking and disease after exposure to asbestos
There is an interaction between smoking and asbestos exposure to impact an increased incidence of small opacities observed on both the chest X-ray and CT scan as well as in pathological specimens (89, 225–227) (Table 4). A dose-response pattern emerged between increasing pack-years and parenchymal opacities (228). In those reports where the type of interaction has been addressed, the data in general have favored an additive effect but this could be greater (93, 221). In the US Navy's Asbestos Medical Surveillance Program, the prevalence of definite radiologic parenchymal abnormalities (ILO category ≥ 1/0) was 3.10 percent for 32,233 smokers vs. 1.09 percent for 13,414 non-smokers. Mechanistic endpoints also support interactions between smoking and asbestos exposure in lavage cellularity in patients with asbestosis (229). However, increases in profusion of asbestosis with smoking have been attributed to diffuse interstitial fibrosis (88, 213). Smoking can also increase the risk for pleural thickening/plaques (230–234). Plaques in ever smokers are proposed to result with clearance pathways decreased by smoking leading to higher exposures of the pleura.
Reduction in both FVC and FEV1/FVC were more frequent in insulators who smoked compared with non-smokers or smokers in the general population supporting an interaction (235, 236). Occupational exposure to asbestos in the cement industry was a risk factor for increased lung function decline which synergistically interacted with smoking (237). There was an interaction between asbestos exposure and smoking in the impact on pulmonary function (238, 239). Additive but not synergistic effects between exposures to smoking and asbestos were present for manifestations of asbestosis including bilateral fine crackles, clubbing, dyspnea, radiographic abnormality, decreased forced vital capacity, and decreased single breath diffusing capacity of the lung for carbon monoxide (240).
Finally, an interaction between smoking and exposure to asbestos with lung cancer risk was confirmed (241–250). This can range from additive to supra-additive to multiplicative.
Conclusions
There is an interaction between smoking and other particles which impacts the biological effects of occupational and environmental exposures. This interaction is mediated by an impact of smoking on clearance. Smoking will almost always increase the risk for or exacerbate many particle-related lung diseases. In occupational and environmental lung disease, both decreasing CS and controlling particle exposures are of vital importance (251–253).
Author contributions
AG: Writing – original draft, Writing – review & editing. MS: Writing – original draft, Writing – review & editing. RS: Writing – original draft, Writing – review & editing. EP: Writing – original draft, Writing – review & editing. VR: Writing – original draft, Writing – review & editing.
Funding
The author(s) declare that no financial support was received for the research and/or publication of this article.
Conflict of interest
The authors declare that the research was conducted in the absence of any commercial or financial relationships that could be construed as a potential conflict of interest.
Generative AI statement
The author(s) declare that no Gen AI was used in the creation of this manuscript.
Publisher's note
All claims expressed in this article are solely those of the authors and do not necessarily represent those of their affiliated organizations, or those of the publisher, the editors and the reviewers. Any product that may be evaluated in this article, or claim that may be made by its manufacturer, is not guaranteed or endorsed by the publisher.
Abbreviations
AD, alveolar duct; AM, alveolar macrophage; CMD, coal mine disease; COPD, chronic obstructive pulmonary disease; CS, cigarette smoking; DIP, desquamative interstitial pneumonitis; FEV1, forced expiratory volume in 1 second; FVC, forced vital capacity; ILA, interstitial lung abnormality; ILD, interstitial lung disease; IPF, idiopathic pulmonary fibrosis; NSIP, nonspecific interstitial pneumonia; OP, organizing pneumonia; RB, respiratory bronchiole; RB-ILD, respiratory bronchiolitis-interstitial lung disease; RBitis, respiratory bronchiolitis; TB, terminal bronchiole; UIP, usual interstitial pneumonia.
References
1. West JB. Pulmonary blood flow and gas exchange. In:West JB., , editor. Respiratory Physiology: People and Ideas. New York: Oxford University Press (1996). p. 140–169. doi: 10.1007/978-1-4614-7520-0_5
2. Galvin I, Drummond GB, Nirmalan M. Distribution of blood flow and ventilation in the lung: gravity is not the only factor. Br J Anaesth. (2007) 98:420–8. doi: 10.1093/bja/aem036
3. Lambert AR, O'Shaughnessy P, Tawhai MH, Hoffman EA, Lin C-L. Regional deposition of particles in an image-based airway model: large-eddy simulation and left-right lung ventilation asymmetry. Aerosol Sci Technol. (2011) 45:11–25. doi: 10.1080/02786826.2010.517578
4. Lee KP, Kelly DP. Translocation of particle-laden alveolar macrophages and intra-alveolar granuloma formation in rats exposed to Ludox colloidal amorphous silica by inhalation. Toxicology. (1993) 77:205–22. doi: 10.1016/0300-483X(93)90161-K
5. Ferin J. Observations concerning alveolar dust clearance. Ann NY Acad Sci. (1972) 200:66–72. doi: 10.1111/j.1749-6632.1972.tb40178.x
6. Oberdorster G. Lung dosimetry: pulmonary clearance of inhaled particles. Aerosol Sci Technol. (1993) 18:279–89. doi: 10.1080/02786829308959605
7. Kohlfaerber T, Pieper M, Münter M, Holzhausen C, Ahrens M, Idel C, et al. Dynamic microscopic optical coherence tomography to visualize the morphological and functional micro-anatomy of the airways. Biomed Opt Express. (2022) 13:3211–23. doi: 10.1364/BOE.456104
8. Lehnert BE. Pulmonary and thoracic macrophage subpopulations and clearance of particles from the lung. Environ Health Perspect. (1992) 97:17–146. doi: 10.2307/3431327
9. Oberdörster G, Cox C, Gelein R. Intratracheal instillation versus intratracheal-inhalation of tracer particles for measuring lung clearance function. Exp Lung Res. (1997) 23:17–34. doi: 10.3109/01902149709046045
10. Brown JS, Zeman KL, Bennett WD. Regional deposition of coarse particles and ventilation distribution in healthy subjects and patients with cystic fibrosis. J Aerosol Med. (2001) 14:443–54. doi: 10.1089/08942680152744659
11. Fritsch P, Masse R. Overview of pulmonary alveolar macrophage renewal in normal rats and during different pathological processes. Environ Health Perspect. (1992) 97:59–67. doi: 10.1289/ehp.929759
12. Goto Y, Ishii H, Hogg JC, Shih CH, Yatera K, Vincent R, et al. Particulate matter air pollution stimulates monocyte release from the bone marrow. Am J Respir Crit Care Med. (2004) 170:891–7. doi: 10.1164/rccm.200402-235OC
13. Cekici Y, Yilmaz M, Secen O. New inflammatory indicators: association of high eosinophil-to-lymphocyte ratio and low lymphocyte-to-monocyte ratio with smoking. J Int Med Res. (2019) 47:4292–303. doi: 10.1177/0300060519862077
14. Nielsen H. A quantitative and qualitative study of blood monocytes in smokers. Eur J Respir Dis. (1985) 66:327–32.
15. Nemmar A, Inuwa IM. Diesel exhaust particles in blood trigger systemic and pulmonary morphological alterations. Toxicol Lett. (2008) 176:20–30. doi: 10.1016/j.toxlet.2007.09.006
16. Bruske I, Hampel R, Socher MM, Ruckerl R, Schneider A, Heinrich J, et al. Impact of ambient air pollution on the differential white blood cell count in patients with chronic pulmonary disease. Inhal Toxicol. (2010) 22:245–52. doi: 10.3109/08958370903207274
17. Su TC, Hwang JJ, Yang YR, Chan CC. Association between long-term exposure to traffic-related air pollution and inflammatory and thrombotic markers in middle-aged adults. Epidemiology. (2017) 28 Suppl 1:S74–81. doi: 10.1097/EDE.0000000000000715
18. van Waarde D, Hulsing-Hesselink E, Sandkuyl LA, van Furth R. Humoral regulation of monocytopoiesis during the early phase of an inflammatory reaction caused by particulate substances. Blood. (1977) 50:141–54. doi: 10.1182/blood.V50.1.141.141
19. Chaudhuri N, Jary H, Lea S, Khan N, Piddock KC, Dockrell DH, et al. Diesel exhaust particle exposure in vitro alters monocyte differentiation and function. PLoS ONE. (2012) 7:e51107. doi: 10.1371/journal.pone.0051107
20. Rom WN. Basic mechanisms leading to focal emphysema in coal workers' pneumoconiosis. Environ Res. (1990) 53:16–28. doi: 10.1016/S0013-9351(05)80127-6
21. Wallace WA, Gillooly M, Lamb D. Intra-alveolar macrophage numbers in current smokers and non-smokers: a morphometric study of tissue sections. Thorax. (1992) 47:437–40. doi: 10.1136/thx.47.6.437
22. Lippmann M, Yeates DB, Albert RE. Deposition, retention, and clearance of inhaled particles. Br J Ind Med. (1980) 37:337–62. doi: 10.1136/oem.37.4.337
23. Hiller FC. Deposition of sidestream cigarette smoke in the human respiratory tract. Prev Med. (1984) 13:602–7. doi: 10.1016/S0091-7435(84)80010-9
24. Fraig M, Shreesha U, Savici D, Katzenstein A-L. Respiratory bronchiolitis: a clinicopathologic study in current smokers, ex-smokers, and never-smokers. Am J Surg Pathol. (2002) 26:647–53. doi: 10.1097/00000478-200205000-00011
25. Woo OH, Yong HS, Oh YW, Lee SY, Kim HK, Kang EY. Respiratory bronchiolitis-associated interstitial lung disease in a nonsmoker: radiologic and pathologic findings. AJR Am J Roentgenol. (2007) 188:W412–4. doi: 10.2214/AJR.05.0835
26. Sismanlar T, Aslan AT, Turktas H, Memis L, Griese M. Respiratory bronchiolitis-associated interstitial lung disease in childhood: new sequela of smoking. Pediatrics. (2015) 136:e1026–9. doi: 10.1542/peds.2015-1303
27. Clarà PC, Jerez FR, Ramírez JB, González CM. Deposition and clinical impact of inhaled particles in the lung. Arch Bronconeumol. (2023) 59:377–82. doi: 10.1016/j.arbres.2023.01.016
28. Tsuda A, Henry FS, Butler JP. Gas and aerosol mixing in the acinus. Respir Physiol Neurobiol. (2008) 163:139–49. doi: 10.1016/j.resp.2008.02.010
29. Akira M, Suganuma N. Imaging diagnosis of pneumoconiosis with predominant nodular pattern: HRCT and pathologic findings. Clin Imaging. (2023) 97:28–33. doi: 10.1016/j.clinimag.2023.02.010
30. Oberdorster G. Lung particle overload: implications for occupational exposures to particles. Regul Toxicol Pharmacol. (1995) 21:123–35. doi: 10.1006/rtph.1995.1017
31. Morrow PE. Possible mechanisms to explain dust overloading of the lungs. Fund Appl Toxicol. (1988) 10:369–384. doi: 10.1093/toxsci/10.3.369
32. Morrow PE. Dust overloading of the lungs: update and appraisal. Toxicol Appl Pharmacol. (1992) 113:1–12. doi: 10.1016/0041-008X(92)90002-A
33. Yu RC, Rappaport SM. Relation between pulmonary clearance and particle burden: a Michaelis-Menten-like kinetic model. Occup Environ Med. (1996) 53:567–572. doi: 10.1136/oem.53.8.567
34. Cullen RT, Tran CL, Buchanan D, Davis JM, Searl A, Jones AD, et al. Inhalation of poorly soluble particles. I. Differences in inflammatory response and clearance during exposure. Inhal Toxicol. (2000) 12:1089–1111. doi: 10.1080/08958370050166787
35. Oberdörster G, Ferin J, Lehnert BE. Correlation between particle size, in vivo particle persistence, and lung injury. Environ Health Perspect. (1994) 102 Suppl 5:173–179. doi: 10.1289/ehp.94102s5173
36. Tran CL, Buchanan D, Cullen RT, Searl A, Jones AD, Donaldson K. Inhalation of poorly soluble particles. II. Influence of particle surface area on inflammation and clearance. Inhal Toxicol. (2000) 12:1113–1126. doi: 10.1080/08958370050166796
37. Warheit DB, Hansen JF, Yuen IS, Kelly DP, Snajdr SI, Hartsky MA. Inhalation of high concentrations of low toxicity dusts in rats results in impaired pulmonary clearance mechanisms and persistent inflammation. Toxicol Appl Pharmacol. (1997) 145:10–22. doi: 10.1006/taap.1997.8102
38. Kawasaki H. A mechanistic review of particle overload by titanium dioxide. Inhal Toxicol. (2017) 29:530–40. doi: 10.1080/08958378.2018.1430192
39. Yu CP, Chen YK, Morrow PE. An analysis of alveolar macrophage mobility kinetics at dust overloading of the lungs. Fundam Appl Toxicol. (1989) 13:452–9. doi: 10.1093/toxsci/13.3.452
40. Ischander M, Fan LL, Farahmand V, Langston C, Yazdani S. Desquamative interstitial pneumonia in a child related to cigarette smoke. Pediatr Pulmonol. (2014) 49:E56–8. doi: 10.1002/ppul.22812
41. Harmsen AG, Muggenburg BA, Snipes MB, Bice DE. The role of macrophages in particle translocation from lungs to lymph nodes. Science. (1985) 230:1277–80. doi: 10.1126/science.4071052
42. Baldwin DR, Lambert L, Pantin CF, Prowse K, Cole RB. Silicosis presenting as bilateral hilar lymphadenopathy. Thorax. (1996) 51:1165–7. doi: 10.1136/thx.51.11.1165
43. Kirchner J, Kirchner EM, Goltz JP, Lorenz V-M, Kickuth R. Prevalence of enlarged mediastinal lymph nodes in heavy smokers–a comparative study. Eur Radiol. (2011) 21:1594–9. doi: 10.1007/s00330-011-2111-9
44. Takano APC, Justo LT, Dos Santos NV, Marquezini MV, de André PA, da Rocha FMM, et al. Pleural anthracosis as an indicator of lifetime exposure to urban air pollution: an autopsy-based study in Sao Paulo. Environ Res. (2019) 173:23–32. doi: 10.1016/j.envres.2019.03.006
45. Ferin J, Oberdörster G. Translocation of particles from pulmonary alveoli into the interstitium. J Aerosol Med. (1992) 5:179–187. doi: 10.1089/jam.1992.5.179
46. Bermudez E, Mangum JB, Asgharian B, Wong BA, Reverdy EE, Janszen DB, et al. Long-term pulmonary responses of three laboratory rodent species to subchronic inhalation of pigmentary titanium dioxide particles. Toxicol Sci. (2002) 70:86–97. doi: 10.1093/toxsci/70.1.86
47. Eydner M, Schaudien D, Creutzenberg O, Ernst H, Hansen T, Baumgärtner W, et al. Impacts after inhalation of nano- and fine-sized titanium dioxide particles: morphological changes, translocation within the rat lung, and evaluation of particle deposition using the relative deposition index. Inhal Toxicol. (2012) 24:557–569. doi: 10.3109/08958378.2012.697494
48. Lauweryns JM, Baert JH. Alveolar clearance and the role of the pulmonary lymphatics. Am Rev Respir Dis. (1977) 115:625–683.
49. Dodson RF, Shepherd S, Levin J, Hammar SP. Characteristics of asbestos concentration in lung as compared to asbestos concentration in various levels of lymph nodes that collect drainage from the lung. Ultrastruct Pathol. (2007) 31:95–133. doi: 10.1080/01913120701423907
50. Boutin C, Dumortier P, Rey F, Viallat JR, De Vuyst P. Black spots concentrate oncogenic asbestos fibers in the parietal pleura. Thoracoscopic and mineralogic study. Am J Respir Crit Care Med. (1996) 153:444–9. doi: 10.1164/ajrccm.153.1.8542156
51. Mitchev K, Dumortier P, De Vuyst P. 'Black Spots' and hyaline pleural plaques on the parietal pleura of 150 urban necropsy cases. Am J Surg Pathol. (2002) 26:1198–206. doi: 10.1097/00000478-200209000-00010
52. Tosi P, Franzinelli A, Miracco C, Leoncini L, Minacci C, Baldelli C, et al. Silicotic lymph node lesions in non-occupationally exposed lung carcinoma patients. Eur J Respir Dis. (1986) 68:362–9.
53. Murray J, Webster I, Reid G, Kielkowski D. The relation between fibrosis of hilar lymph glands and the development of parenchymal silicosis. Br J Ind Med. (1991) 48:267–9. doi: 10.1136/oem.48.4.267
54. Seaton A, Cherrie JW. Quartz exposures and severe silicosis: a role for the hilar nodes. Occup Environ Med. (1998) 55:383–6. doi: 10.1136/oem.55.6.383
55. Wong TW Yu TS, Tam CM, Wang XR. A silicosis patient presenting with an enlarged supraclavicular lymph node. Respirology. (1998) 3:281–3. doi: 10.1111/j.1440-1843.1998.tb00136.x
56. Ooi CG, Khong PL, Cheng RS, Tan B, Tsang F, Lee I, et al. The relationship between mediastinal lymph node attenuation with parenchymal lung parameters in silicosis. Int J Tuberc Lung Dis. (2003) 7:1199–206.
57. Honma K, Nelson G, Murray J. Intrapulmonary lymph nodes in South African miners–an autopsy survey. Am J Ind Med. (2007) 50:261–4. doi: 10.1002/ajim.20436
58. Cox-Ganser JM, Burchfiel CM, Fekedulegn D, Andrew ME, Ducatman BS. Silicosis in lymph nodes: the canary in the miner? J Occup Environ Med. (2009) 51:164–9. doi: 10.1097/JOM.0b013e31818f6a0f
59. Müller K-M, Schmitz I, Konstantinidis K. Black spots of the parietal pleura: morphology and formal pathogenesis. Respiration. (2002) 69:261–7. doi: 10.1159/000063630
60. Zeren EH, Colby TV, Roggli VL. Silica-induced pleural disease: an unusual case mimicking malignant mesothelioma. Chest. (1997) 112:1436–8. doi: 10.1378/chest.112.5.1436
61. Tsuchiya K, Toyoshima M, Fukada A, Akahori D, Funai K, Suda T. Lupus pleuritis with silicotic nodules in the parietal pleura. Intern Med. (2018) 57:1277–80. doi: 10.2169/internalmedicine.9193-17
62. Kabat GC. Aspects of the epidemiology of lung cancer in smokers and nonsmokers in the United States. Lung Cancer. (1996) 15:1–20. doi: 10.1016/0169-5002(95)00566-8
63. Rutgers SR, Postma DS, ten Hacken NH, Kauffman HF, van Der Mark TW, Koëter GH, et al. Ongoing airway inflammation in patients with COPD who do not currently smoke. Thorax. (2000) 55:12–8. doi: 10.1136/thorax.55.1.12
64. Ghio AJ, Hilborn ED, Stonehuerner JG, Dailey LA, Carter JD, Richards JH, et al. Particulate matter in cigarette smoke alters iron homeostasis to produce a biological effect. Am J Respir Crit Care Med. (2008) 178:1130–8. doi: 10.1164/rccm.200802-334OC
65. Sangani RG, Ghio AJ. Lung injury after cigarette smoking is particle related. Int J Chron Obstruct Pulmon Dis. (2011) 6:191–8. doi: 10.2147/COPD.S14911
66. National Research Council. Environmental Tobacco Smoke: Measuring Exposures and Assessing Health Effects. Washington DC: National Academy Press (1986).
67. Kim SY, Sim S, Choi HG. Active and passive smoking impacts on asthma with quantitative and temporal relations: a Korean Community Health Survey. Sci Rep. (2018) 8:8614. doi: 10.1038/s41598-018-26895-3
68. Cohen D, Arai SF, Brain JD. Smoking impairs long-term dust clearance from the lung. Science. (1979) 204:514–7. doi: 10.1126/science.432655
69. Simet SM, Sisson JH, Pavlik JA, Devasure JM, Boyer C, Liu X, et al. Long-term cigarette smoke exposure in a mouse model of ciliated epithelial cell function. Am J Respir Cell Mol Biol. (2010) 43:635–40. doi: 10.1165/rcmb.2009-0297OC
70. Sturm R. Theoretical model of clearance in the tracheobronchial airways of healthy subjects and smokers. J Public Health Emerg. (2018) 2:13. doi: 10.21037/jphe.2018.03.04
71. Yousem SA. Respiratory bronchiolitis-associated interstitial lung disease with fibrosis is a lesion distinct from fibrotic nonspecific interstitial pneumonia: a proposal. Mod Pathol. (2006) 11:1474–9. doi: 10.1038/modpathol.3800671
72. Margaritopoulos GA, Vasarmidi E, Jacob J, Wells AU, Antoniou KM. Smoking and interstitial lung diseases. Eur Respir Rev. (2015) 24:428–35. doi: 10.1183/16000617.0050-2015
73. Caminati A, Harari S. Smoking-related interstitial pneumonias and pulmonary Langerhans cell histiocytosis. Proc Am Thorac Soc. (2006) 3:299–306. doi: 10.1513/pats.200512-135TK
74. Moon J, Du Bois RM, Colby TV, Hansell DM, Nicholson AG. Clinical significance of respiratory bronchiolitis on open lung biopsy and its relationship to smoking related interstitial lung disease. Thorax. (1999) 54:1009–14. doi: 10.1136/thx.54.11.1009
75. Wells AU, Nicholson AG, Hansell DM. Challenges in pulmonary fibrosis. 4: smoking-induced diffuse interstitial lung diseases. Thorax. (2007) 62:904–10. doi: 10.1136/thx.2004.031021
76. Godbert B, Wissler MP, Vignaud JM. Desquamative interstitial pneumonia: an analytic review with an emphasis on aetiology. Eur Respir Rev. (2013) 22:117–23. doi: 10.1183/09059180.00005812
77. Margaritopoulos GA, Harari S, Caminati A, Antoniou KM. Smoking-related idiopathic interstitial pneumonia: a review. Respirology. (2016) 21:57–64. doi: 10.1111/resp.12576
78. Bak SH, Lee HY. Overlaps and uncertainties of smoking-related idiopathic interstitial pneumonias. Int J Chron Obstruct Pulmon Dis. (2017) 12:3221–9. doi: 10.2147/COPD.S146899
79. Camner P, Philipson K. Tracheobronchial clearance in smoking-discordant twins. Arch Environ Health. (1972) 25:60–3. doi: 10.1080/00039896.1972.10666136
80. Marques LJ, Teschler H, Guzman J, Costabel U. Smoker's lung transplanted to a nonsmoker. Long-term detection of smoker's macrophages. Am J Respir Crit Care Med. (1997) 156:1700–2. doi: 10.1164/ajrccm.156.5.9611052
81. Tazelaar HD, Wright JL, Churg A. Desquamative interstitial pneumonia. Histopathology. (2011) 58:509–16. doi: 10.1111/j.1365-2559.2010.03649.x
82. Attili AK, Kazerooni EA, Gross BH, Flaherty KR, Myers JL, Martinez FJ. Smoking-related interstitial lung disease: radiologic-clinical-pathologic correlation. Radiographics. (2008) 28:1383–96. doi: 10.1148/rg.285075223
84. Epstein DM, Miller WT, Bresnitz EA, Levine MS, Gefter WB. Application of ILO classification to a population without industrial exposure: findings to be differentiated from pneumoconiosis. AJR Am J Roentgenol. (1984) 142:53–8. doi: 10.2214/ajr.142.1.53
85. Wiggins J, Strickland B, Turner-Warwick M. Combine cryptogenic fibrosing alveolitis and emphysema: the value of high-resolution computed tomography in assessment. Respir Med. (1990) 84:365–9. doi: 10.1016/S0954-6111(08)80070-4
86. Dick JA, Morgan WK, Muir DF, Reger RB, Sargent N. The significance of irregular opacities on the chest roentgenogram. Chest. (1992) 102:251–60. doi: 10.1378/chest.102.1.251
87. Hnizdo E, Cowie RL, Hay M, Glyn Thomas R. Association of silicosis, lung dysfunction and emphysema in gold miners. Thorax. (1993) 48:746–9. doi: 10.1136/thx.48.7.746
89. Weiss W. Cigarette smoke, asbestos and small irregular opacities. Am Rev Respir Dis. (1984) 130:293–301l. doi: 10.1164/arrd.1984.130.2.293
90. Araki T, Putman RK, Hatabu H, Gao W, Dupuis J, Latourelle JC, et al. Development and progression of interstitial lung abnormalities in the Framingham Heart Study. Am J Respir Crit Care Med. (2016) 194:1514–22. doi: 10.1164/rccm.201512-2523OC
91. Podolanczuk AJ, Oelsner EC, Barr RG, Hoffman EA, Armstrong HF, Austin JH, et al. High attenuation areas on chest computed tomography in community-dwelling adults: the Mesa study. Eur Respir J. (2016) 48:1442–52. doi: 10.1183/13993003.00129-2016
92. Ash SY, Harmouche R, Ross JC, Diaz AA, Hunninghake GM, Putman RK, et al. The objective identification and quantification of interstitial lung abnormalities in smokers. Acad Radiol. (2017) 24:941–6. doi: 10.1016/j.acra.2016.08.023
93. Iwasawa T, Takemura T, Ogura T. Smoking-related lung abnormalities on computed tomography images: comparison with pathological findings. Jpn J Radiol. (2018) 36:165–80. doi: 10.1007/s11604-017-0713-0
94. Hoyer N, Wille MMW, Thomsen LH, Wilcke T, Dirksen A, Pedersen JH, et al. Interstitial lung abnormalities are associated with increased mortality in smokers. Respir Med. (2018) 136:77–82. doi: 10.1016/j.rmed.2018.02.001
95. Sangani RG, Deepak V, Ghio AJ, Forte MJ, Zulfikar R, Patel Z, et al. Interstitial lung abnormalities and interstitial lung diseases associated with cigarette smoking in a rural cohort undergoing surgical resection. BMC Pulm Med. (2022) 22:172. doi: 10.1186/s12890-022-01961-9
96. Miller ER, Putman RK, Vivero M, Hung Y, Araki T, Nishino M, et al. Histopathology of interstitial lung abnormalities in the context of lung nodule resections. Am J Respir Crit Care Med. (2018) 197:955–8. doi: 10.1164/rccm.201708-1679LE
97. Marten K, Milne D, Antoniou KM, Nicholson AG, Tennant RC, Hansel TT, et al. Non-specific interstitial pneumonia in cigarette smokers: a CT study. Eur Radiol. (2009) 19:1679–85. doi: 10.1007/s00330-009-1308-7
98. Barroso E, Hernandez L, Gil J, Garcia R, Aranda I, Romero S. Idiopathic organizing pneumonia: a relapsing disease: 19 years of experience in a hospital setting. Respiration. (2007) 74:624–31. doi: 10.1159/000103240
99. Sveinsson OA, Isaksson HJ, Sigvaldason A, Yngvason F, Aspelund T, Gudmundsson G. Clinical features in secondary and cryptogenic organising pneumonia. Int J Tuberc Lung Dis. (2007) 11:689–94.
100. Iwai K, Mori T, Yamada N, Yamaguchi M, Hosoda Y. Idiopathic pulmonary fibrosis. Epidemiologic approaches to occupational exposure. Am J Respir Crit Care Med. (1994) 150:670–5. doi: 10.1164/ajrccm.150.3.8087336
101. Baumgartner KB, Samet JM, Stidley CA, Colby TV, Waldron JA. Cigarette smoking: a risk factor for idiopathic pulmonary fibrosis. Am J Respir Crit Care Med. (1997) 155:242–8. doi: 10.1164/ajrccm.155.1.9001319
102. Morse D, Rosas IO. Tobacco smoke-induced lung fibrosis and emphysema. Annu Rev Physiol. (2014) 76:493–513. doi: 10.1146/annurev-physiol-021113-170411
103. Wick MR. Pathologic features of smoking-related lung diseases, with emphasis on smoking-related interstitial fibrosis and a consideration of differential diagnoses. Semin Diagn Pathol. (2018) 35:315–23. doi: 10.1053/j.semdp.2018.08.002
104. Romero S, Barroso E, Rodriguez-Paniagua M, Aranda FI. Organizing pneumonia adjacent to lung cancer: frequency and clinico-pathologic features. Lung Cancer. (2002) 35:195–201. doi: 10.1016/S0169-5002(01)00405-6
105. Pardo J, Panizo A, Sola I, Queipo F, Martinez-Penuela A, Carias R. Prognostic value of clinical, morphologic, and immunohistochemical factors in patients with bronchiolitis obliterans-organizing pneumonia. Hum Pathol. (2013) 44:718–24. doi: 10.1016/j.humpath.2012.07.016
106. Smith M, Dalurzo M, Panse P, Parish J, Leslie K. Usual interstitial pneumonia-pattern fibrosis in surgical lung biopsies. Clinical, radiological and histopathological clues to aetiology. J Clin Pathol. (2013) 66:896–903. doi: 10.1136/jclinpath-2013-201442
107. Huo Z, Feng R, Tian X, Zhang H, Huo L, Liu H. Clinicopathological findings of focal organizing pneumonia: a retrospective study of 37 cases. Int J Clin Exp Pathol. (2015) 8:511–6.
108. Yilmaz S, Akinci Özyürek B, Erdogan Y, Cirit Koçer B, Demirag F, Dadali Y, et al. Retrospective evaluation of patients with organizing pneumonia: is cryptogenic organizing pneumonia different from secondary organizing pneumonia? Tuberk Toraks. (2017) 65:1–8. doi: 10.5578/tt.53938
109. Beardsley B, Rassl D. Fibrosing organising pneumonia. J Clin Pathol. (2013) 66:875–81. doi: 10.1136/jclinpath-2012-201342
110. Cottin V, Donsbeck AV, Revel D, Loire R, Cordier JF. Nonspecific interstitial pneumonia: Individualization of a clinicopathologic entity in a series of 12 patients. Am J Respir Crit Care Med. (1998) 158:1286–93. doi: 10.1164/ajrccm.158.4.9802119
111. Rabeyrin M, Thivolet F, Ferretti GR, Chalabreysse L, Jankowski A, Cottin V, et al. Usual interstitial pneumonia end-stage features from explants with radiologic and pathological correlations. Ann Diagn Pathol. (2015) 19:269–76. doi: 10.1016/j.anndiagpath.2015.05.003
112. Walsh SL, Hansell DM. Diffuse interstitial lung disease: overlaps and uncertainties. Eur Radiol. (2010) 20:1859–67. doi: 10.1007/s00330-010-1737-3
113. Todd NW, Galvin JR, Sachdeva A, Luzina IG, Atamas SP, Burke AP. Microscopic organizing pneumonia and cellular non-specific interstitial pneumonia are widespread in macroscopically normal-appearing lung tissue in idiopathic pulmonary fibrosis. J Heart Lung Transplant. (2016) 35:1367–70. doi: 10.1016/j.healun.2016.07.014
114. Katzenstein AL, Zisman DA, Litzky LA, Nguyen BT, Kotloff RM. Usual interstitial pneumonia: histologic study of biopsy and explant specimens. Am J Surg Pathol. (2002) 26:1567–77. doi: 10.1097/00000478-200212000-00004
115. Du Bois R, King TE Jr. Challenges in pulmonary fibrosis x 5: the NSIP/UIP debate. Thorax. (2007) 62:1008–12. doi: 10.1136/thx.2004.031039
116. Fang X, Luo B, Yi X, Zeng Y, Liu F, Li H, et al. Usual interstitial pneumonia coexisted with nonspecific interstitial pneumonia, What's the diagnosis? Diagn Pathol. (2012) 7:167. doi: 10.1186/1746-1596-7-167
117. Li XR, Peng SC, Wei LQ. Nonspecific interstitial pneumonia overlaps organizing pneumonia in lung-dominant connective tissue disease. Int J Clin Exp Pathol. (2015) 8:11230–5.
118. Todd NW, Marciniak ET, Sachdeva A, Kligerman SJ, Galvin JR, Luzina IG, et al. Organizing pneumonia/non-specific interstitial pneumonia overlap is associated with unfavorable lung disease progression. Respir Med. (2015) 109:1460–8. doi: 10.1016/j.rmed.2015.09.015
119. Huo Z, Li J, Li S, Zhang H, Jin Z, Pang J, et al. Organizing pneumonia components in non-specific interstitial pneumonia (NSIP): a clinicopathological study of 33 NSIP cases. Histopathology. (2016) 68:347–55. doi: 10.1111/his.12761
120. Bellou V, Belbasis L, Evangelou E. Tobacco smoking and risk for pulmonary fibrosis: a prospective cohort study from the UK biobank. Chest. (2021) 160:983–93. doi: 10.1016/j.chest.2021.04.035
121. Abraham JL, Hertzberg MA. Inorganic particulates associated with desquamative interstitial pneumonia. Chest. (1981) 80:67–70. doi: 10.1378/chest.80.1_Supplement.67S
122. Monso E, Tura JM, Pujadas J, Morell F, Ruiz J, Morera J. Lung dust content in idiopathic pulmonary fibrosis: a study with scanning electron microscopy and energy dispersive x ray analysis. Br J Ind Med. (1991) 48:327–31. doi: 10.1136/oem.48.5.327
123. Guarnieri G, Bizzotto R, Gottardo O, Velo E, Cassaro M, Vio S, et al. Multiorgan accelerated silicosis misdiagnosed as sarcoidosis in two workers exposed to quartz conglomerate dust. Occup Environ Med. (2019) 76:178–80. doi: 10.1136/oemed-2018-105462
124. Morgan WKC, Seaton A. Occupational Lung Diseases. Philadelphia: WB Saunders Co. (1984). p. 260–261.
125. Tse LA Yu IT, Qiu H, Leung CC. Joint effects of smoking and silicosis on diseases to the lungs. PLoS ONE. (2014) 9:e104494. doi: 10.1371/journal.pone.0104494
126. Hessel PA, Gamble JF, Gee JB, Gibbs G, Green FH, Morgan WK, et al. Silica, silicosis, and lung cancer: a response to a recent working group report. J Occup Environ Med. (2000) 42:704–20. doi: 10.1097/00043764-200007000-00005
127. Cherry NM, Burgess GL, Turner S, McDonald JC. Crystalline silica and risk of lung cancer in the potteries. Occup Environ Med. (1998) 55:779–85. doi: 10.1136/oem.55.11.779
128. Hughes JM, Weill H, Checkoway H, Jones RN, Henry MM, Heyer NJ, et al. Radiographic evidence of silicosis risk in the diatomaceous earth industry. Am J Respir Crit Care Med. (1998) 158:807–14. doi: 10.1164/ajrccm.158.3.9709103
129. Rosenman KD, Reilly MJ, Rice C, Hertzberg V, Tseng CY, Anderson HA. Silicosis among foundry workers. Implication for the need to revise the OSHA standard. Am J Epidemiol. (1996) 144:890–900. doi: 10.1093/oxfordjournals.aje.a009023
130. Hughes JM, Weill H, Rando RJ, Shi R, McDonald AD, McDonald JC. Cohort mortality study of North American industrial sand workers. II Case-referent analysis of lung cancer and silicosis deaths. Ann Occup Hyg. (2001) 45:201–7. doi: 10.1016/S0003-4878(00)00078-8
131. Poinen-Rughooputh S, Rughooputh MS, Guo Y, Lai H, Sun W, Chen W. Sex-related differences in the risk of silicosis among Chinese pottery workers: a cohort study. J Occup Environ Med. (2021) 63:74–9. doi: 10.1097/JOM.0000000000002068
132. Hoy RF, Dimitriadis C, Abramson M, Glass DC, Gwini S, Hore-Lacy F, et al. Prevalence and risk factors for silicosis among a large cohort of stone benchtop industry workers. Occup Environ Med. (2023) 80:439–46. doi: 10.1136/oemed-2023-108892
133. Graham WGB, Ashikaga T, Hemenway D, Weaver S, O'Grady RV. Radiographic abnormalities in Vermont granite workers exposed to low levels of granite dust. Chest. (1991) 100:1507–14. doi: 10.1378/chest.100.6.1507
134. Harber P, Dahlgren J, Bunn W, Lockey J, Chase G. Radiographic and spirometric findings in diatomaceous earth workers. J Occup Environ Med. (1998) 40:22–8. doi: 10.1097/00043764-199801000-00007
135. Sager TM, Umbright CM, Mustafa GM, Yanamala N, Leonard HD, McKinney WG, et al. Tobacco smoke exposure exacerbated crystalline silica-induced lung toxicity in rats. Toxicol Sci. (2020) 178:375–90. doi: 10.1093/toxsci/kfaa146
136. Hessel PA, Sluis-Cremer GK, Lee SL. Distribution of silicotic collagenization in relation to smoking habits. Am Rev Respir Dis. (1991) 144:297–301. doi: 10.1164/ajrccm/144.2.297
137. Arakawa H, Johkoh T, Honma K, Saito Y, Fukushima Y, Shida H, et al. Chronic interstitial pneumonia in silicosis and mix-dust pneumoconiosis: its prevalence and comparison of CT findings with idiopathic pulmonary fibrosis. Chest. (2007) 131:1870–6. doi: 10.1378/chest.06-2553
138. Arakawa H, Fujimoto K, Honma K, Suganuma N, Morikubo H, Saito Y, et al. Progression from near-normal to end-stage lungs in chronic interstitial pneumonia related to silica exposure: long-term CT observations. AJR Am J Roentgenol. (2008) 191:1040–5. doi: 10.2214/AJR.07.3871
139. Kang W, Kim D, Kim MY, Kim B, Shin J, Kim EY, et al. Usual interstitial pneumonia associated with crystalline silica exposure in pneumoconiosis: a retrospective cohort study. J Occup Environ Med. (2021) 63:e905–10. doi: 10.1097/JOM.0000000000002399
140. Mohner M, Kersten N, Gellissen J. Chronic obstructive pulmonary disease and longitudinal changes in pulmonary function due to occupational exposure to respirable quartz. Occup Environ Med. (2013) 70:9–14. doi: 10.1136/oemed-2012-100775
141. Hnizdo E. Loss of lung function associated with exposure to silica dust and with smoking and its relation to disability and mortality in South African gold miners. Br J Ind Med. (1992) 49:472–9. doi: 10.1136/oem.49.7.472
142. Hnizdo E. Combined effect of silica dust and tobacco smoking on mortality from chronic obstructive lung disease in gold miners. Br J Ind Med. (1990) 47:656–64. doi: 10.1136/oem.47.10.656
143. Hnizdo E, Vallyathan V. Chronic obstructive pulmonary disease due to occupational exposure to silica dust: a review of epidemiological and pathological evidence. Occup Environ Med. (2003) 60:237–43. doi: 10.1136/oem.60.4.237
144. Fan Y, Xu W, Wang Y, Wang Y, Yu S, Ye Q. Association of occupational dust exposure with combined chronic obstructive pulmonary disease and pneumoconiosis: a cross-sectional study in China. BMJ Open. (2020) 10:e038874. doi: 10.1136/bmjopen-2020-038874
145. Leung CC, Chang KC, Law WS, Yew WW, Tam CM, Chan CK, et al. Determinants of spirometric abnormalities among silicotic patients in Hong Kong. Occup Med (Lond). (2005) 55:490–3. doi: 10.1093/occmed/kqi107
146. Yang S, Chan CK, Wang MH, Leung CC, Tai LB, Tse LA. Association of smoking cessation with airflow obstruction in workers with silicosis: A cohort study. PLoS One. (2024) 19:e0303743. doi: 10.1371/journal.pone.0303743
147. Hnizdo E, Baskind E, Sluis-Cremer GK. Combined effect of silica dust exposure and tobacco smoking on the prevalence of respiratory impairments among gold miners. Scand J Work Environ Health. (1990) 16:411–22. doi: 10.5271/sjweh.1768
148. Peng Y, Li X, Cai S, Chen Y, Dai W, Liu W, et al. Prevalence and characteristics of COPD among pneumoconiosis patients at an occupational disease prevention institute: a cross-sectional study. BMC Pulm Med. (2018) 18:22. doi: 10.1186/s12890-018-0581-0
149. Delgado L, Parra ER, Capelozzi VL. Apoptosis and extracellular matrix remodeling in human silicosis. Histopathology. (2006) 49:283–9. doi: 10.1111/j.1365-2559.2006.02477.x
150. Garcia D, Sultan NM, Yerba OR, Castro SJ, Palacios EA, Cano AD. Silicosis and smoking: intrinsic phenomenon in the respiratory system. Advances in Applied Sociology. (2018) 8:659–67. doi: 10.4236/aasoci.2018.810039
151. Hessel PA, Gamble JF, Nicolich M. Relationship between silicosis and smoking. Scand J Work Environ Health. (2003) 29:329–36. doi: 10.5271/sjweh.739
152. Leung CC, Yew WW, Law WS, Tam CM, Leung M, Chung YW, et al. Smoking and tuberculosis among silicotic patients. Eur Respir J. (2007) 29:745–50. doi: 10.1183/09031936.00134706
153. Liu Y, Steenland K, Rong Y, Hnizdo E, Huang X, Zhang H, et al. Exposure-response analysis and risk assessment for lung cancer in relationship to silica exposure: a 44-year cohort study of 34,018 workers. Am J Epidemiol. (2013) 178:1424–33. doi: 10.1093/aje/kwt139
154. Ge C, Peters S, Olsson A, Portengen L, Schüz J, Almansa J, et al. Respirable crystalline silica exposure, smoking, and lung cancer subtype risks. A pooled analysis of case-control studies. Am J Respir Crit Care Med. (2020) 202:412–21. doi: 10.1164/rccm.201910-1926OC
155. Cassidy A, 't Mannetje A, van Tongeren M, Field JK, Zaridze D, Szeszenia-Dabrowska N, et al. Occupational exposure to crystalline silica and risk of lung cancer A multicenter case-control study in Europe. Epidemiology. (2007) 18:36–43. doi: 10.1097/01.ede.0000248515.28903.3c
156. Hnizdo E, Sluis-Cremer GK. Silica exposure, silicosis, and lung cancer: a mortality study of South African gold miners. Br J Ind Med. (1991) 48:53–60. doi: 10.1136/oem.48.1.53
157. HSE. Respirable crystalline silica—phase 2. In: Hazardous Assessment Document. London: Health & Safety Executive (2003).
158. Kurihara N, Wada O. Silicosis and smoking strongly increase lung cancer risk in silica-exposed workers. Ind Health. (2004) 42:303–14. doi: 10.2486/indhealth.42.303
159. Ng TP, Chan SL, Lee J. Mortality of a cohort of men in a silicosis register: further evidence of an association with lung cancer. Am J Ind Med. (1990) 17:163–71. doi: 10.1002/ajim.4700170203
160. Wang D, Yang M, Liu Y, Ma J, Shi T, Chen W. Association of silica dust exposure and cigarette smoking with mortality among mine and pottery workers in China. JAMA Netw Open. (2020) 3:e202787. doi: 10.1001/jamanetworkopen.2020.2787
161. Lai HP, Liu YW, Zhou M, Shi TM, Zhou Y, Weng SF, et al. Combined effect of silica dust exposure and cigarette smoking on total and cause-specific mortality in iron miners: a cohort study. Environ Health. (2018) 17:46. doi: 10.1186/s12940-018-0391-0
162. Heitzman ER, Naeye RL, Markarian B. Roentgen pathological correlations in coal workers' pneumoconiosis. Ann N Y Acad Sci. (1972) 200:510–26. doi: 10.1111/j.1749-6632.1972.tb40214.x
163. Jelic TM, Estalilla OC, Sawyer-Kaplan PR, Plata MJ, Powers JT, Emmett M, et al. Coal mine dust desquamative chronic interstitial pneumonia: a precursor of dust-related diffuse fibrosis and of emphysema. Int J Occup Environ Med. (2017) 8:153–65. doi: 10.15171/ijoem.2017.1066
164. Brichet A, Tonnel AB, Brambilla E, Devouassoux G, Rémy-Jardin M, Copin MC, et al. Chronic interstitial pneumonia with honeycombing in coal workers. Sarcoidosis Vasc Diffuse Lung Dis. (2002) 19:211–9.
165. Naeye RL, Mahon JK, Dellinger WS. Effects of smoking on lung structure of Appalachian coal workers. Arch Environ Health. (1971) 22:190–3. doi: 10.1080/00039896.1971.10665831
166. Laney AS, Petsonk EL. Small pneumoconiotic opacities on US coal worker surveillance chest radiographs are not predominantly in the upper lung zones. Am J Indust Med. (2012) 55:793–8. doi: 10.1002/ajim.22049
167. Cockcroft A. Prevalence and relation to underground exposure of radiological irregular opacities in South Wales coal workers with pneumoconiosis. Br J Ind Med. (1983) 40:169–72. doi: 10.1136/oem.40.2.169
168. Amandus HE, Lapp NL, Jacobson G, Reger RB. Significance of irregular small opacities in radiographs of coalminers in the USA. Br J Ind Med. (1976) 33:13–7. doi: 10.1136/oem.33.1.13
169. Petsonk EL, Rose C, Cohen R. Coal mine dust lung disease: new lessons from an old exposure. Am J Respir Crit Care Med. (2013) 187:1178–85. doi: 10.1164/rccm.201301-0042CI
170. McConnochie K, Green FHY, Vallyathan V, Wagner JC, Seal RME, Lyons JP. Interstitial fibrosis in coal workers experience in Wales and West Virginia. Ann Occup Hyg. (1988) 32:S553–60. doi: 10.1016/B978-0-08-034185-9.50063-2
171. Liu X, Salter A, Thomas P, Leigh J, Wang H. Exhaled nitric oxide levels and lung function changes of underground coal miners in Newcastle, Australia. J Toxicol Environ Health A. (2010) 73:437–44. doi: 10.1080/15287390903486592
172. Qian QZ, Cao XK, Shen FH, Wang Q. Correlations of smoking with cumulative total dust exposure and cumulative abnormal rate of pulmonary function in coal-mine workers. Exp Ther Med. (2016) 12:2942–8. doi: 10.3892/etm.2016.3700
173. Ames RG, Amandus H, Attfield M, Green FY, Vallyathan V. Does coal mine dust present a risk for lung cancer? A case-control study of US coal miners. Arch Environ Health. (1983) 38:331–3. doi: 10.1080/00039896.1983.10545816
174. Balakrishnan B, Sarkar S, Choi J, Patel R, Adkins T, Fang W. Risk factors associated with malignant lung nodules in smokers with coal worker's pneumoconiosis: an exploratory case-control study. Open Public Health J. (2024) 17:1. doi: 10.2174/0118749445267364231214050044
175. Sadler RL, Roy TJ. Smoking and mortality from coal workers' pneumoconiosis. Br J Ind Med. (1990) 47:141–2. doi: 10.1136/oem.47.2.141
176. Churg A, Brauer M. Human lung parenchyma retains PM25. Am J Respir Crit Care Med. (1997) 155:2109–11. doi: 10.1164/ajrccm.155.6.9196123
177. Churg A, Brauer M. Ambient atmospheric particles in the airways of human lungs. Ultrastruct Pathol. (2000) 24:353–61. doi: 10.1080/019131200750060014
178. Brauer M, Avila-Casado C, Fortoul TI, Vedal S, Stevens B, Churg A. Air pollution and retained particles in the lung. Environ Health Perspect. (2001) 109:1039–4. doi: 10.2307/3454959
179. Donaldson K, Wallace WA, Henry C, Seaton A. Black lungs in the general population: a new look at an old dispute. J R Coll Physicians Edinb. (2019) 49:165–70. doi: 10.4997/jrcpe.2019.219
180. Mukhopadhyay S, Gujral M, Abraham JL, Scalzetti EM, Iannuzzi MC. A case of hut lung: scanning electron microscopy with energy dispersive x-ray spectroscopy analysis of a domestically acquired form of pneumoconiosis. Chest. (2013) 144:323–7. doi: 10.1378/chest.12-2085
181. Xu X, Wang L. Synergistic effects of air pollution and personal smoking on adult pulmonary function. Arch Environ Health. (1998) 53:44–53. doi: 10.1080/00039899809605688
182. Krzyzanowski M, Wojtyniak B. Ten-year mortality in a sample of an adult population in relation to air pollution. J Epidemiol Commun Health. (1982) 36:262–8. doi: 10.1136/jech.36.4.262
183. Pope CA 3rd, Burnett RT, Thurston GD, Thun MJ, Calle EE, Krewski D, et al. Cardiovascular mortality and long-term exposure to particulate air pollution: epidemiological evidence of general pathophysiological pathways of disease. Circulation. (2004) 109:71–7. doi: 10.1161/01.CIR.0000108927.80044.7F
184. Wong C-M, Ou C-Q, Lee N-W, Chan K-P, Thach T-Q, Chau Y-K, et al. Short-term effects of particulate air pollution on male smokers and never-smokers. Epidemiology. (2007) 18:593–8. doi: 10.1097/EDE.0b013e318125713c
185. Filleul L, Rondeau V, Cantagrel A, Dartigues JF, Tessier JF. Do subject characteristics modify the effects of particulate air pollution on daily mortality among the elderly? J Occup Environ Med. (2004) 46:1115–22. doi: 10.1097/01.jom.0000144998.82543.9d
186. Sun S, Cao W, Chan K-P, Ran J, Ge Y, Zhang Y, et al. Cigarette smoking increases deaths associated with air pollution in Hong Kong. Atmos Environ. (2020) 223:117266. doi: 10.1016/j.atmosenv.2020.117266
187. McDonald JC, Sebastien P, Armstrong B. Radiological survey of past and present vermiculite miners exposed to tremolite. Br J Ind Med. (1986) 43:445–9. doi: 10.1136/oem.43.7.445
188. Wild P, Réfrégier M, Auburtin G, Carton B, Moulin JJ. Survey of the respiratory health of the workers of a talc producing factory. Occup Environ Med. (1995) 52:470–7. doi: 10.1136/oem.52.7.470
189. Said AM, AbdelFattah EB, Almawardi A-AM. Effects on respiratory system due to exposure to wheat flour. Egyptian J Chest Dis Tuberc. (2017) 66:537–48. doi: 10.1016/j.ejcdt.2016.11.006
190. Beck GJ, Maunder LR, Schachter EN. Cotton dust and smoking effects on lung function in cotton textile workers. Am J Epidemiol. (1984) 119:33–43. doi: 10.1093/oxfordjournals.aje.a113723
191. Shi NY, Lu PL. Pulmonary function study of retired cotton textile workers and the relationship to cigarette smoking. Biomed Environ Sci. (1988) 1:152–9.
192. Su YM, Su JR, Sheu JY, Loh CH, Liou SH. Additive effect of smoking and cotton dust exposure on respiratory symptoms and pulmonary function of cotton textile workers. Ind Health. (2003) 41:109–15. doi: 10.2486/indhealth.41.109
193. Donaldson K, Murphy FA, Duffin R, Poland CA. Asbestos, carbon nanotubes and the pleural mesothelium: a review of the hypothesis regarding the role of long fibre retention in the parietal pleura, inflammation and mesothelioma. Part Fibre Toxicol. (2010) 7:5. doi: 10.1186/1743-8977-7-5
194. Searl A, Buchanan D, Cullen RT, Jones AD, Miller BG, Soutar CA. Biopersistence and durability of nine mineral fibre types in rat lungs over 12 months. Ann Occup Hyg. (1999) 43:143–53. doi: 10.1016/S0003-4878(99)00017-4
195. Barlow CA, Grespin M, Best EA. Asbestos fiber length and its relation to disease risk. Inhal Toxicol. (2017) 29:541–54. doi: 10.1080/08958378.2018.1435756
196. Berman DW, Crump KS, Chatfield EJ, Davis JM, Jones AD. The sizes, shapes, and mineralogy of asbestos structures that induce lung tumors or mesothelioma in AF/HAN rats following inhalation. Risk Anal. (1995) 15:181–95. doi: 10.1111/j.1539-6924.1995.tb00312.x
197. Roggli VL. The so-called short-fiber controversy: literature review and critical analysis. Arch Pathol Lab Med. (2015) 139:1052–7. doi: 10.5858/arpa.2014-0466-RA
198. Berman DW, Crump KS. A meta-analysis of asbestos-related cancer risk that addresses fiber size and mineral type. Crit Rev Toxicol. (2008) 38 Suppl 1:49–73. doi: 10.1080/10408440802273156
199. Leinardi R, Petriglieri JR, Pochet A, Yakoub Y, Lelong M, Lescoat A, et al. Distinct pro-inflammatory mechanisms elicited by short and long amosite asbestos fibers in macrophages. Int J Mol Sci. (2023) 24:15145. doi: 10.3390/ijms242015145
200. Schinwald A, Donaldson K. Use of back-scatter electron signals to visualise cell/nanowires interactions in vitro and in vivo; frustrated phagocytosis of long fibres in macrophages and compartmentalisation in mesothelial cells in vivo. Part Fibre Toxicol. (2012) 9:34. doi: 10.1186/1743-8977-9-34
201. Bernstein DM. The health effects of short fiber chrysotile and amphibole asbestos. Crit Rev Toxicol. (2022) 52:89–112. doi: 10.1080/10408444.2022.2056430
202. Boulanger G, Andujar P, Pairon JC, Billon-Galland MA, Dion C, Dumortier P, et al. Quantification of short and long asbestos fibers to assess asbestos exposure: a review of fiber size toxicity. Environ Health. (2014) 13:59. doi: 10.1186/1476-069X-13-59
203. Dodson RF, Atkinson MA, Levin JL. Asbestos fiber length as related to potential pathogenicity: a critical review. Am J Ind Med. (2003) 44:291–7. doi: 10.1002/ajim.10263
204. Suzuki Y, Yuen SR, Ashley R. Short, thin asbestos fibers contribute to the development of human malignant mesothelioma: Pathological evidence. Int J Hyg Environ Health. (2005) 208:201–10. doi: 10.1016/j.ijheh.2005.01.015
205. Roggli VL, Gibbs AR, Attanoos R, Churg A, Popper H, Cagle P, et al. Pathology of asbestosis- an update of the diagnostic criteria: Report of the asbestosis committee of the college of American pathologists and pulmonary pathology society. Arch Pathol Lab Med. (2010) 134:462–80. doi: 10.5858/134.3.462
206. Corrin B, Price AB. Electron microscopic studies in desquamative interstitial pneumonia associated with asbestos. Thorax. (1972) 27:324–31. doi: 10.1136/thx.27.3.324
207. Freed JA, Miller A, Gordon RE, Fischbein A, Kleinerman J, Langer AM. Desquamative interstitial pneumonia associated with chrysotile asbestos fibres. Br J Ind Med. (1991) 48:332–7. doi: 10.1136/oem.48.5.332
208. Rossiter CE. Evidence of dose-response relation in pneumoconiosis. Trans Soc Occup Med. (1972) 22:83–7. doi: 10.1093/occmed/22.1.83
209. Churg A, Wright JL, Vedal S. Fiber burden and patterns of asbestos-related disease in chrysotile miners and millers. Am Rev Respir Dis. (1993) 148:25–31. doi: 10.1164/ajrccm/148.1.25
210. Roggli VL, Pratt PC, Brody AR. Asbestos content of lung tissue in asbestos associated diseases: a study of 110 cases. Br J Ind Med. (1986) 43:18–28. doi: 10.1136/oem.43.1.18
211. Hnizdo E, Sluis-Cremer GK. Effect of tobacco smoking on the presence of asbestosis at postmortem and on the reading of irregular opacities on roentgenograms in asbestos-exposed workers. Am Rev Respir Dis. (1988) 138:1207–12. doi: 10.1164/ajrccm/138.5.1207
212. Berry G, Gilson JC, Holmes S, Lewinsohn HC, Roaoh SA. Asbestosis: a study of dose-response relationships in an asbestos textile factory. Br J Ind Med. (1979) 36:98–112. doi: 10.1136/oem.36.2.98
213. Finkelstein MM. A study of dose-response relationships for asbestos associated disease. Br J Ind Med. (1985) 42:319–25. doi: 10.1136/oem.42.5.319
214. Lilis R, Sellkoff IJ, Lerman Y, Seidman H, Gelb SK. Asbestosis: Interstitial pulmonary fibrosis and pleural fibrosis. In a cohort of asbestos Insulation workers: Influence of cigarette smoking. Am J Ind Med. (1986) 10:459–70. doi: 10.1002/ajim.4700100504
215. Biano PD, Golden JA, Gamsu G, Aberle DR, Gold WM. Asbestos exposure-cigarette smoking Interactions among shipyard workers. JAMA. (1988) 259:370–3. doi: 10.1001/jama.1988.03720030030028
216. Rosenstock L, Barnhart S, Heyer NJ, Pierson DJ, Hudson LD. The relation among pulmonary function, chest roentgenographic abnormalities and smoking status in an asbestos-exposed cohort. Am Rev Respir Dis. (1988) 138:272–277228. doi: 10.1164/ajrccm/138.2.272
217. Loomis D, Dement J, Richardson D, Wolf S. Asbestos fiber dimensions and lung cancer mortality among workers exposed to chrysotile. Occup Environ Med. (2010) 67:580–4. doi: 10.1136/oem.2009.050120
218. Miserocchi G, Sancini G, Mantegazza F, Chiappino G. Translocation pathways for inhaled asbestos fibers. Environ Health. (2008) 7:4. doi: 10.1186/1476-069X-7-4
219. Chua TC, Yan TD, Morris DL. Surgical biology for the clinician: peritoneal mesothelioma: current understanding and management. Can J Surg. (2009) 52:59–64.
220. Sampson C, Hansell DM. The prevalence of enlarged mediastinal lymph nodes in asbestos-exposed individuals: a CT study. Clin Radiol. (1992) 45:340–2232. doi: 10.1016/S0009-9260(05)80089-3
221. Stanton MF, Layard M, Tegeris A, Miller E, May M, Morgan E, et al. Relation of particle dimension to carcinogenicity in amphibole asbestoses and other fibrous minerals. J Natl Cancer Inst. (1981) 67:965–75.
222. Lippmann M. Asbestos exposure indices. Environ Res. (1988) 46:86–106. doi: 10.1016/S0013-9351(88)80061-6
223. Stayner L, Kuempel E, Gilbert S, Hein M, Dement J. An epidemiological study of the role of chrysotile asbestos fibre dimensions in determining respiratory disease risk in exposed workers. Occup Environ Med. (2008) 65:613–19. doi: 10.1136/oem.2007.035584
224. Finkelstein MM, Vingilis JJ. Radiographic abnormalities among asbestos-cement workers. An exposure-response study. Am Rev Respir Dis. (1984) 129:17–22236.
225. Zitting AJ, Karjalainen A, Impivaara O, Kuusela T, Mäki J, Tossavainen A, et al. Radiographic small lung opacities and pleural abnormalities in relation to smoking, urbanization status, and occupational asbestos exposure in Finland. J Occup Environ Med. (1996) 38:602–9. doi: 10.1097/00043764-199606000-00011
226. Vehmas T, Kivisaari L, Huuskonen MS, Jaakkola MS. Effects of tobacco smoking on findings in chest computed tomography among asbestos-exposed workers. Eur Respir J. (2003) 21:866–71. doi: 10.1183/09031936.03.00029002
227. Delclos GL, Wilson RK, Bradley BL. Influence of smoking on radiographic profusion and pleural changes in asbestos-exposed subjects. J Occup Med. (1990) 32:577–81. doi: 10.1097/00043764-199007000-00006
228. Pearle JL. Smoking and duration of asbestos exposure in the production of functional and roentgenographic abnormalities in shipyard workers. J Occup Med. (1982) 24:37–40.
229. Schwartz DA, Galvin JR, Merchant RK, Dayton CS, Burmeister LF, Merchant JA, et al. Influence of cigarette smoking on bronchoalveolar lavage cellularity in asbestos-induced lung disease. Am Rev Respir Dis. (1992) 145:400–5. doi: 10.1164/ajrccm/145.2_Pt_1.400
230. Baker EL, Dagg T, Greene RE. Respiratory Illness in the construction trades I The significance of asbestos-associated pleural disease among sheet metalworkers. J Occup Med. (1985) 27:483–9.
231. Weiss W, Theodos PA. Pleuropulmonary disease among asbestos workers in relation to smoking and type of exposure. J Occup Med. (1978) 20:341–5.
232. Weiss W, Levin R, Goodman L. Pleural plaques and cigarette smoking in asbestos workers. J Occup Med. (1981) 23:427–30. doi: 10.1097/00043764-198106000-00016
233. Rossiter CE, Harries PGUK. Naval dockyards asbestosis study: survey of the sample population aged 50–59 years. Br Ind Med. (1979) 36:281–91. doi: 10.1136/oem.36.4.281
234. Hillerdal G. Pleural plaques in a health survey material. Scand J Respir Dis. (1978) 59:257–63.
235. Miller A, Lilis R, Godbold J, Chan E, Wu X, Selikoff IJ. Spirometric impairments in long-term insulators. Relationships to duration of exposure, smoking, and radiographic abnormalities. Chest. (1994) 105:175–82. doi: 10.1378/chest.105.1.175
236. Lerman Y, Seidman H, Gelb S, Miller A, Selikoff IJ. Spirometric abnormalities among asbestos insulation workers. J Occup Med. (1988) 30:228–33.
237. Algranti E, Mendonça EMC, Hnizdo E, De Capitani EM, Freitas JBP, Raile V, et al. Longitudinal decline in lung function in former asbestos exposed workers. Occup Environ Med. (2013) 70:15–21. doi: 10.1136/oemed-2012-100715
238. Hall SK, Cissik JH. Effects of cigarette smoking on pulmonary function in asymptomatic asbestos workers with normal chest radiograms. Am Ind Hyg Assoc J. (1982) 43:381–6. doi: 10.1202/0002-8894(1982)043 <0381:EOCSOP>2.3.CO;2
239. Kilburn KH, Warshaw RH. Severity of pulmonary asbestosis as classified by International Labour Organisation profusion of irregular opacities in 8749 asbestos-exposed American workers. Those who never smoked compared with those who ever smoked. Arch Intern Med. (1992) 152:325–7. doi: 10.1001/archinte.1992.00400140073017
240. Samet JM, Epler GR, Gaensler EA, Rosner B. Absence of synergism between exposure to asbestos and cigarette smoking in asbestosis. Am Rev Respir Dis. (1979) 120:75–82.
241. Erren TC, Jacobsen M, Piekarski C. Synergy between asbestos and smoking on lung cancer risks. Epidemiology. (1999) 10:405–11. doi: 10.1097/00001648-199907000-00011
242. Lee PN. Relation between exposure to asbestos and smoking jointly and the risk of lung cancer. Occup Environ Med. (2001) 58:145–53. doi: 10.1136/oem.58.3.145
243. Liddell FD. The interaction of asbestos and smoking in lung cancer. Ann Occup Hyg. (2001) 45:341–56. doi: 10.1016/S0003-4878(00)00060-0
244. Case BW. Asbestos, smoking, and lung cancer: interaction and attribution. Occup Environ Med. (2006) 63:507–8. doi: 10.1136/oem.2006.027631
245. Reid A, de Klerk NH, Ambrosini GL, Berry G, Musk AW. The risk of lung cancer with increasing time since ceasing exposure to asbestos and quitting smoking. Occup Environ Med. (2006) 63:509–12. doi: 10.1136/oem.2005.025379
246. Yano E, Wang X, Wang M, Qiu H, Wang Z. Lung cancer mortality from exposure to chrysotile asbestos and smoking: a case-control study within a cohort in China. Occup Environ Med. (2010) 67:867–71. doi: 10.1136/oem.2009.051615
247. Inamura K, Ninomiya H, Nomura K, Tsuchiya E, Satoh Y, Okumura S, et al. Combined effects of asbestos and cigarette smoke on the development of lung adenocarcinoma: different carcinogens may cause different genomic changes. Oncol Rep. (2014) 32:475–82. doi: 10.3892/or.2014.3263
248. Ngamwong Y, Tangamornsuksan W, Lohitnavy O, Chaiyakunapruk N, Scholfield CN, Reisfeld B, et al. Additive synergism between asbestos and smoking in lung cancer risk: a systematic review and meta-analysis. PLoS ONE. (2015) 10:e0135798. doi: 10.1371/journal.pone.0135798
249. Zoghbi ME, Salameh P, Stücker I, Brochard P, Delva F, Lacourt A. Absence of multiplicative interactions between occupational lung carcinogens and tobacco smoking: a systematic review involving asbestos, crystalline silica and diesel engine exhaust emissions. BMC Public Health. (2017) 17:156. doi: 10.1186/s12889-017-4025-1
250. Lévêque E, Lacourt A, Philipps V, Luce D, Guénel P, Stücker I, et al. A new trajectory approach for investigating the association between an environmental or occupational exposure over lifetime and the risk of chronic disease: application to smoking, asbestos, and lung cancer. PLoS ONE. (2020) 15:e0236736. doi: 10.1371/journal.pone.0236736
251. Elmes PC. Relative importance of cigarette smoking in occupational lung disease. Br J Ind Med. (1981) 38:1–13. doi: 10.1136/oem.38.1.1
252. Girard P, Vieira T. Air pollution vs. tobacco smoking. Eur Heart J. (2019) 40:32023202. doi: 10.1093/eurheartj/ehz436
Keywords: smoking, silicosis, anthracosis, asbestosis, occupational exposures, environmental exposures
Citation: Ghio AJ, Stewart M, Sangani RG, Pavlisko EN and Roggli VL (2025) Cigarette smoking decreases macrophage-dependent clearance to impact the biological effects of occupational and environmental particle exposures. Front. Public Health 13:1558723. doi: 10.3389/fpubh.2025.1558723
Received: 10 January 2025; Accepted: 10 March 2025;
Published: 09 April 2025.
Edited by:
Andrey Korchevskiy, Chemistry & Industrial Hygiene, Inc., United StatesReviewed by:
Emma Marie Stapleton, The University of Iowa, United StatesFilippo Liviero, University of Padua, Italy
Copyright © 2025 Ghio, Stewart, Sangani, Pavlisko and Roggli. This is an open-access article distributed under the terms of the Creative Commons Attribution License (CC BY). The use, distribution or reproduction in other forums is permitted, provided the original author(s) and the copyright owner(s) are credited and that the original publication in this journal is cited, in accordance with accepted academic practice. No use, distribution or reproduction is permitted which does not comply with these terms.
*Correspondence: Andrew J. Ghio, Z2hpby5hbmR5QGVwYS5nb3Y=