- 1Department of Chemistry, Konan University, Hyōgo, Japan
- 2Research Center for Solar Energy Chemistry, Osaka University, Osaka, Japan
- 3Institute of Applied Physics, University of Tsukuba, Ibaraki, Japan
Effects of zirconium (Zr) doping into BiVO4 powder on its structural properties and photocatalytic activity for O2 evolution were examined. The formation of BiVO4 powder crystallized in a monoclinic scheelite structure (ms-BiVO4) was achieved when the sample was doped with a relatively small amount of Zr. The photocatalytic activity of Zr-doped ms-BiVO4 powder was much higher than that of non-doped ms-BiVO4. However, further doping caused a reduction of photocatalytic activity for O2 evolution due to the occurrence of structural alterations into tetragonal scheelite and tetragonal zircon structures. Similar effects of Zr doping were also observed for the photoelectrochemical (PEC) system based on BiVO4 thin films doped with various amounts of Zr. Thus, Zr doping was confirmed to be effective for improvements of photocatalytic and PEC functions of BiVO4 for water oxidation.
Introduction
Bismuth vanadate (BiVO4), an n-type metal oxide semiconductor, has been widely studied as a potential photocatalyst and/or photoanode for oxygen (O2) production through water-splitting using sunlight due to its suitable band-gap energy (Eg), high optical absorption, reasonable band-edge alignment for O2/H2O redox potential, nontoxicity, and abundance of constituent elements (Kudo et al., 1998, 1999; Park et al., 2013; Suarez et al., 2015). Although BiVO4 cannot produce hydrogen (H2) from water because of its insufficient electron energy level of the conduction band minimum (CBM) (Kudo et al., 1999; Kato et al., 2004; Walsh et al., 2009), the compound has been used for a two-step photoexcitation system, called a Z-scheme water splitting, that is analogous to natural photosynthesis (Kato et al., 2004; Sasaki et al., 2009; Iwase et al., 2011; Martin et al., 2014; Jiang et al., 2015; Baek et al., 2017).
BiVO4 exists in three crystalline phases, namely, monoclinic scheelite (ms-BiVO4), tetragonal scheelite (ts-BiVO4), and tetragonal zircon (tz-BiVO4) structures (Lim et al., 1995). For water oxidation into O2, the first two scheelite structures are known to be active; enhanced photoactivity of scheelite BiVO4 compounds is mainly due to enhanced photon absorption properties derived from their relatively narrow band-gap energies (2.4 eV) compared to that of tz-BiVO4 (2.9 eV) (Kudo et al., 1999). Among the scheelite compounds, moreover, the ms-BiVO4 phase shows much higher activity for O2 production from water than does the ts-BiVO4 phase. The difference is likely to be due to induction of local polarization owing to an appreciable lattice distortion of the Bi-O bond in ms-BiVO4 compared to that in ts-BiVO4, leading to the enhancement of efficient electron-hole separation (Tokunaga et al., 2001).
BiVO4 possess two doping sites, Bi and V. Doping with molybdenum (Mo) or tungsten (W) at the V site has been shown to be effective for enhancing activity for water oxidation (Park et al., 2011; Ye et al., 2011; Berglund et al., 2012). Since a hexavalent form is the most stable phase for Mo and W, doping with these elements at the pentavalent V site should introduce excess electrons. Hence, increasing n-type conductivity would be effective for enhancement of the activity, though details in correlations between the enhancement of photocatalytic activity and carrier dynamics of those doped compounds have been less discussed yet (Grigioni et al., 2015). In addition, according to the study of density functional theory (DFT) (Yin et al., 2011), group IVB element, i.e., Zr and Hf, can substitute the Bi site in BiVO4 with low formation energy. Since replacement of trivalent Bi with tetravalent Zr or Hf induces enhancement of n-type conductivity, doping of these elements is expected to have effects that are similar to those of doping with Mo or W. In the present study, therefore, BiVO4 powders and films doped with Zr were prepared. Photocatalytic and PEC properties for water oxidation were investigated in relation to their structural characteristics.
Experiment
Preparation of Powder Photocatalysts
BiVO4 powder was prepared in an aqueous nitric acid solution containing Bi3+ ions and V2O5 powder, as reported in the literature (Ng et al., 2010; Iwase et al., 2011). A 4.9-g portion of Bi(NO3)3·5H2O (Wako) was dissolved in 50 mL of 0.75 M nitric acid (Bi-soln). After addition of 0.92 g of V2O5 (Wako) to Bi-soln, the orange-colored suspension was stirred at room temperature for 48 h. A yellow powder suspension thus-obtained was filtered to collect the powder part. The powder was washed several times with water and then dried at 70°C. The thus-obtained non-doped BiVO4 powder was labeled BVO. For doping with Zr, appropriate amounts of ZrO(NO3)2·H2O (Wako) were dissolved in Bi-soln, and the reaction was performed by the same procedure as that for the preparation of non-doped BiVO4. The amounts of doped Zr were varied from 0.1 to 3.0% by changing the amount of ZrO(NO3)2·H2O added to Bi-soln. Samples as-obtained were designated Zr(x)BVO, where x denotes the molar content of doped Zr. For example, Zr(0.5)BVO represents 0.5 mol% of Zr-doped BiVO4. For comparison, BiVO4 powder doped with 0.5% of Mo [labeled M(0.5)BVO] was also prepared through the same process using a Bi-soln solution containing 8.9 mg of (NH4)6[Mo7O24]·4H2O (Wako).
Preparation of Thin-Film Electrodes
BiVO4 thin films were prepared on fluorine-doped tin-oxide-coated glass slides (FTO/glass, Aldrich) by spin coating in accordance with the literature procedure (Fuku and Sayama, 2016) with same modifications. Typically, a mixed solution (Bi: V = 1: 1) of bismuth oxide of SYM-BIO5 enhanced metal organic decomposition material (EMOD, Symetrix) and vanadium oxide of V-02 DIP COAT-PRECURSORS (High Pure Chemicals) dissolved in butyl acetate (Wako) containing ethylcellulose (Wako) as a thickener and an aggregation inhibition agent was coated on FTO/glass by spin coating (500 rpm, 15 s). Then the film was calcined at 550°C for 30 min. For Zr doping, appropriate amounts of zirconium oxide of SYM-ZRO5 EMOD (Symetrix) were added to the mixed solution; the same procedure as that for fabrication of the non-doped BiVO4 thin film was used. In the same manner as that for the above powder system, thus-obtained non-doped BiVO4 and Zr-doped BiVO4 powders were labeled BVOf and Zr(x)BVOf (x: molar amount of doped Zr), respectively.
Characterization of Powder and Thin-Film Samples
Crystalline structures of samples were analyzed by X-ray diffraction (XRD) using a Rigaku Mini Flex X-ray diffractometer (Cukaα, Ni filter). Morphologies of powder samples were examined by using a Hitachi S-5000 FEG field emission scanning electron microscope (SEM) at an acceleration voltage of 20 kV. Raman spectroscopy measurements using a JASCO NRC 3100 Laser Raman Spectrophotometer (excitation laser having a wavelength of 532 nm) were performed for structural analyses of thin-film samples.
Photocatalytic and PEC Reactions
For photocatalytic O2 evolution, 50 mg of a powder sample and 5 cm3 of 0.05 M aqueous AgNO3 (Wako) solution were added to a borosilicate-glass test tube. After replacement of air to Ar in the test tube, the suspension was photoirradiated by a Parkin Elmer CERMAX LX-300BUV Xe lamp. The amount of evolved O2 was analyzed every 0.5 h by using a Shimadzu GC-8A gas chromatograph equipped with an MS-5A column (GL sciences) and a TCD detector. PEC water oxidation was performed by using a three-electrode system consisting of the thin-film sample as a working electrode, a Pt wire as a counter electrode, and Ag/AgCl as a reference electrode. These electrodes were immersed in a phosphate buffer with pH 6.86 (Wako). The above-mentioned Xe lamp was used as a light source.
Results and Discussion
Structural and Photocatalytic Properties of Zr-doped BiVO4 Powders
Figure 1 shows XRD patterns of BVO, Zr(0.5)BVO, and Mo(0.5)BVO powders. For all of the samples, observed diffractions were assignable to scheelite BiVO4; there was no diffraction derived from any other impurities. Moreover, observation of two reflections at 2θ of ca. 19°, indexed to the (101) and (011) reflections, indicates that crystalline structures of these powders are classified to the monoclinic crystal system (JCPDS 75-1866). Similar behavior was also observed for the peaks at 2θ of ca. 35° which are indexed to the (200) and (020) reflections of ms-BiVO4 (JCPDS 75-1866). It should be noted that the XRD pattern of M(0.5)BVO showed slight shifts of all of the peaks toward low 2θ angle regions. Since the ionic radius of Mo6+ (0.062 nm) is larger than that of V5+ (0.052 nm) (Yin et al., 2011), the observed shifts indicate incorporation of Mo at the V site in the ms-BiVO4 crystalline lattice. On the other hand, there was no appreciable peak shift observed for the Zr(0.5)BVO sample: occurrence of peak shifts toward high 2θ angle regions was expected when the doped Zr4+ (0.079 nm) was assumed to occupy the Bi3+ (0.111 nm) site (Grigioni et al., 2015). However, there was no appreciable shift observed. Further studies for identification of the doping site(s) for the present Zr dopant are needed.
Figure 2 shows typical SEM images of BVO, Zr(0.5)BVO, and Mo(0.5)BVO powders. The BVO sample consisted of aggregates of angular shaped crystals with submicron sizes. Similar morphologies were also observed for Zr(0.5)BVO and Mo(0.5)BVO powders. As for the results of above XRD analyses are concerned, there are almost no structural and morphological differences between these samples.
For the evaluation of photocatalytic activity for water oxidation, BVO, Zr(0.5)BVO, and Mo(0.5)BVO powders were photoirradiated with a Xe lamp (Eg > 2.4 eV) in an aqueous Ag(NO3)3 solution under Ar. Figure 3 shows typical time course curves of O2 evolution over these powders. As expected from the literature, all of the samples having a monoclinic scheelite structure exhibited appreciable activity for this reaction. Samples, especially the Zr(0.5)BVO sample, showed higher activity than that of the BVO sample. Although the effectiveness of Mo doping is well known in the literature (Park et al., 2011; Ye et al., 2011; Berglund et al., 2012; Wang et al., 2016) and the effectiveness of Zr doping for methylene blue photodegradation have been investigated (Karunakaran et al., 2014), the results of the present study are the first demonstration of enhancement of photocatalytic activity for water oxidation induced by Zr doing into the ms-BiVO4 lattice.
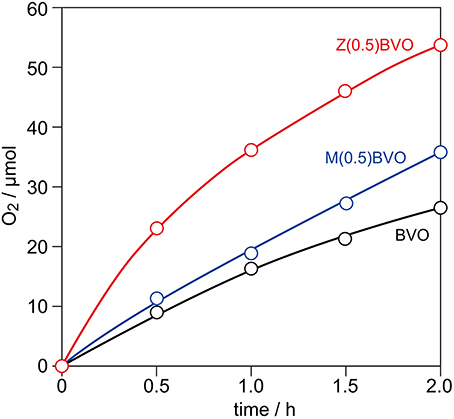
Figure 3. Time course curves of O2 evolution from 0.05 M aqueous AgNO3 solution over BVO, Zr(0.5)BVO, and Mo(0.5)BVO powders under photoirradiation with a 300 W Xe lamp in Ar.
Next, the effects of different amounts of Zr doping in the BiVO4 lattice on structural and photocatalytic properties were examined. Figure 4 shows XRD patterns of powder samples with various amounts of doped Zr. It is clear from the figure that appreciable structural alterations occurred upon increment of the Zr content. In the XRD pattern of the Zr(1.0)BVO sample, appreciable changes in the diffraction pattern were observed at 2θ of ca. 19° and ca. 35°: additional reflections appeared between the (101) and (011) reflections and also between the (200) and (002) reflections (Figures 4A,B). (Park et al., 2011; Berglund et al., 2012) These changes were pronounced for the Zr(3.0)BVO sample: these reflections merge into single peaks in the XRD pattern of the sample, indicating structural transition into tetragonal scheelite (ts-BiVO4) (JCPDS 14-0133). It should be noted that several peaks other than the scheelite form, which are assignable to diffractions of the tetragonal zircon form of BiVO4 (tz-BiVO4), were also observed in the XRD pattern of the Mo(3.0)BVO sample. As can be expected from the literature, these structural transitions were detrimental to photocatalytic activity. Indeed, the rate of O2 evolution, defined as the amount of O2 evolved in the first hour of photoirradiation, reached a maximum for the Zr(0.5)BVO sample and tended to decrease with an increase in the amount of doped Zr as shown in Figure 5. It should be noted that the highest activity was also obtained over the Mo(0.5)BVO sample for the Mo-doped system, and further doping was detrimental to photocatalytic activity because of the occurrence of structural transitions similar to those for the Zr-doped system (data not shown).
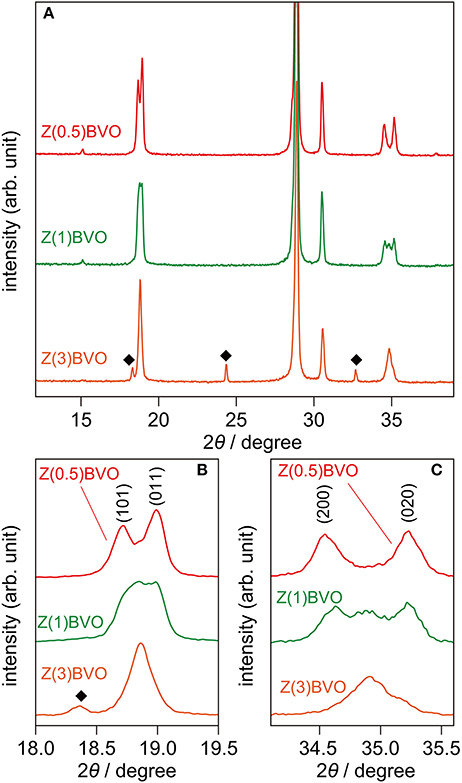
Figure 4. (A) XRD patterns of Zr(0.5)BVO, Zr(1.0)BVO, and Mo(3.0)BVO powders. (B,C) Magnified views at 2θ ranges of 18.0°-19.5° and 34.1°-35.6°, respectively.
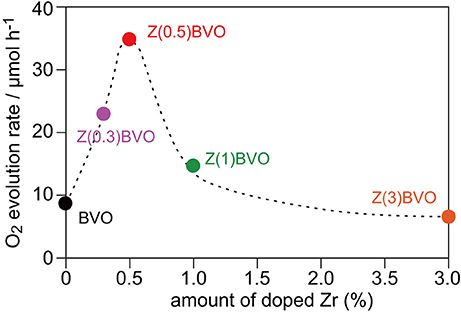
Figure 5. Dependence of rate of O2 evolution on amount of doped Zr. Photocatalytic reactions were performed in 0.05 M aqueous AgNO3 solutions under photoirradiation with a 300 W Xe lamp in Ar.
PEC O2 Evolution Over Zr-doped BiVO4
In order to examine the impact of Zr doping on the PEC property for O2 evolution over the BiVO4 photoanode, Zr-doped BiVO4 thin films were fabricated on FTO/glass substrates. Figure 6 shows Raman spectra of thus-obtained BVOf, Zr(0.5)BVOf, Zr(1.0)BVOf, and Zr(3.0)BVOf films. The dominant Raman bands observed in all of the samples at ca. 830 cm−1 were assigned to the symmetric V-O stretching mode (νs(V-O)); weak shoulders at ca. 710 cm−1 were assigned to the asymmetric V-O stretching mode (νas(V-O)). Both of the Raman signals are typically observed in ms-BiVO4. It should be noted that slight red shifts of νs(V-O) bands were observed for Zr-doped samples with relatively low Zr contents (Zr(0.5)BVOf and Zr(1.0)BVOf). Since similar red shifts were reported in the literature for the Mo-doped ms-BiVO4 system (Merupo et al., 2015), these shifts would be due to incorporation of Zr into the crystalline lattice of ms-BiVO4. The red shifts were pronounced with an increase in the amount of doped Zr (i.e., Zr(3.0)BVOf). Appreciable broadening was also observed for the νs(V-O) Raman band of the sample. The fact that ts-BiVO4 exhibits the νs(V-O) band at 823 cm−1 (Nikam and Joshi, 2016) suggests incorporation of the ts-BiVO4 phase in the sample, as expected from the above-described results for the powder system.
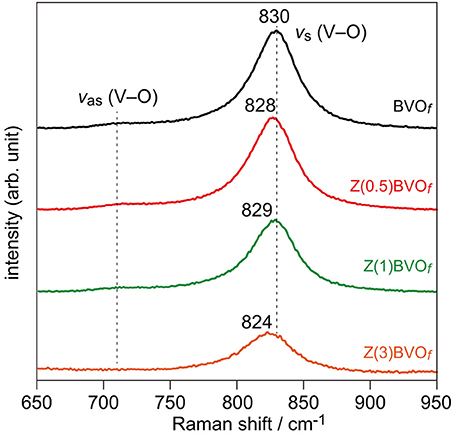
Figure 6. Raman spectra of BVOf, Zr(0.5)BVOf, Zr(1.0)BVOf, and Zr(3.0)BVOf films deposited on FTO/glass substrates.
Upon photoirradiation with a Xe lamp (Eg > 2.4 eV) in a standard three-electrode system, BiVO4 and Zr-doped BiVO4 films undergo carrier generations and separations. Positive holes that have accumulated at surfaces of these films oxidize water into O2, whereas electrons flow through the outer circuit to generate photocurrents. Hence, the PEC activity for water oxidation can be evaluated by the magnitude of anodic photocurrents during photoirradiation. Figure 7 shows typical current density-voltage scans of BVOf, Zr(0.5)BVOf, Zr(1.0)BVOf, and Zr(3.0)BVOf under photoirradiation. None of the films provided appreciable anodic photocurrents when the current density-voltage scan was performed in the dark, indicating that the observed currents were derived from PEC water oxidation. Similar to the above-described results for photocatalytic O2 evolution in the powder photocatalytic system, significant improvement of PEC water oxidation was achieved for Zr doping with a relatively low content (Zr(0.5)BVOf), whereas the films with higher contents of doped Zr became detrimental due to the structural change from active ms-BiVO4 to inactive (or less active) ts-BiVO4 and/or tr-BiVO4 crystals.
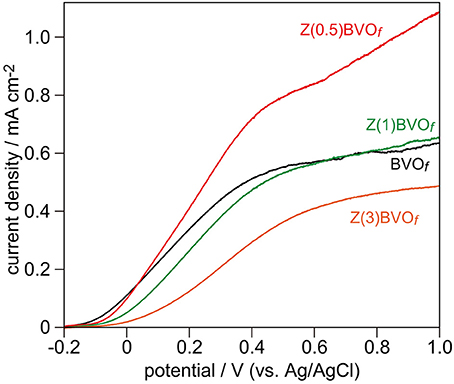
Figure 7. Linear sweep voltammogram (LSV) scans of BVOf, Zr(0.5)BVOf, Zr(1.0)BVOf, and Zr(3.0)BVOf films deposited on FTO/glass substrates. LSV curves were obtained in pH 6.86 phosphate buffer solution under photoirradiation of these thin films with a 300 W Xe lamp.
Conclusions
In this study, we proved the effectiveness of Zr doping into an ms-BiVO4 crystal for improvements of its photocatalytic and PEC functions for water oxidation to produce O2. The doped Zr was assumed to be replaced with the Bi site: due to the replacement of electron-rich Zr, n-type doping would occur, similar to Mo and/or W doping in the ms-BiVO4 crystal. However, detailed investigation of the in crystallographic and electric structures, e.g., the actual doping site(s) of Zr in the crystalline lattice of ms-BiVO4, and quantitative evaluation of carrier densities of samples with and without doped Zr have not been performed. Further studies along these lines are now in progress.
Author Contributions
SI managed all the experiments and wrote the manuscript; TK performed experiments for fabrication of powder and thin film samples, and evaluated photocatalytic and PEC properties of them; YH performed experiments for fabrication of powder samples, and evaluated photocatalytic properties of them; NK performed experiments for fabrication of thin film samples and evaluated PEC properties of them; TH performed experiments for fabrication of powder and thin film samples, evaluated photocatalytic and PEC properties of them, and characterized them by SEM; MR performed experiments and analyses for characterization of powder and thin film samples by XRD and Raman spectroscopy; MI performed experiments and analyses for characterization of powder and thin film samples by XRD; TS performed experiments and analyses for characterization of powder and thin film samples by XRD and Raman spectroscopy, and summarized all the experimental data.
Conflict of Interest Statement
The authors declare that the research was conducted in the absence of any commercial or financial relationships that could be construed as a potential conflict of interest.
Acknowledgments
This work was supported by JSPS KAKENHI Grant Number JP18H05183 in Scientific Research on Innovative Areas and JSPS KAKENHI Grant No. JP18K05010 in Scientific Research (C) from MEXT Japan.
References
Baek, J. H., Kim, B. J., Han, G. S., Hwang, S. W., Kim, D. R., Cho, I. S., et al. (2017). BiVO4/WO3/SnO2 double-heterojunction photoanode with enhanced charge separation and visible-transparency for bias-free solar water-splitting with a perovskite solar cell. ACS Appl. Mater. Interfaces, 9, 1479–1487. doi: 10.1021/acsami.6b12782
Berglund, S. P., Rettie, A. J., Hoang, S., and Mullins, C. B. (2012). Incorporation of Mo and W into nanostructured BiVO4 films for efficient photoelectrochemical water oxidation. Phys. Chem. Chem. Phys. 14, 7065–7075. doi: 10.1039/c2cp40807d
Fuku, K., and Sayama, K. (2016). Efficient oxidative hydrogen peroxide production and accumulation in photoelectrochemical water splitting using a tungsten trioxide/bismuth vanadate photoanode. Chem. Commun. 52, 5406–5409. doi: 10.1039/C6CC01605G
Grigioni, I., Stamplecoskie, K. G., Selli, E., and Kamat, P. V. (2015). Dynamics of photogenerated charge carriers in WO3/BiVO4 heterojunction photoanodes. J. Phys. Chem. C 119, 20792–20800. doi: 10.1021/acs.jpcc.5b05128
Iwase, A., Ng, Y. H., Ishiguro, Y., Kudo, A., and Amal, R. (2011). Reduced graphene oxide as a solid-state electron mediator in Z-scheme photocatalytic water splitting under visible light. J. Am. Chem. Soc. 133, 11054–11057. doi: 10.1021/ja203296z
Jiang, F., Gunawan Harada, T., Kuang, Y., Minegishi, T., Domen, K., et al. (2015). Pt/In2S3/CdS/Cu2ZnSnS4 thin film as an efficient and stable photocathode for water reduction under sunlight radiation. J. Am. Chem. Soc. 137, 13691–13697. doi: 10.1021/jacs.5b09015
Karunakaran, C., Kalaivani, S., and Vinayagamoorthy, P. (2014). Electrical, optical, and visible light-photocatalytic properties of zirconium-doped BiVO4 nanoparticles. Mater. Express 4, 125–134. doi: 10.1166/mex.2014.1156
Kato, H., Hori, M., Konta, R., Shimodaira, Y., and Kudo, A. (2004). Construction of Z-scheme type heterogeneous photocatalysis systems for water splitting into H2 and O2 under visible light irradiation. Chem. Lett. 33, 1348–1349. doi: 10.1246/cl.2004.1348
Kudo, A., Omori, K., and Kato, H. (1999). A novel aqueous process for preparation of crystal form-controlled and highly crystalline BiVO4 powder from layered vanadates at room temperature and its photocatalytic and photophysical properties. J. Am. Chem. Soc. 121, 11459–11467. doi: 10.1021/ja992541y
Kudo, A., Ueda, K., Kato, H., and Mikami, I. (1998). Photocatalytic O2 evolution under visible light irradiation on BiVO4 in aqueous AgNO3 solution. Catal. Lett. 53, 229–230. doi: 10.1023/A:1019034728816
Lim, A. R., Choh, S. H., and Jang, M. S. (1995). Prominent ferroelastic domain walls in BiVO4 crystal. J. Phys. 7, 7309–7323. doi: 10.1088/0953-8984/7/37/005
Martin, D. J., Reardon, P. J. T., Moniz, S. J. A., and Tang, J. W. (2014). Visible light-driven pure water splitting by a nature-inspired organic semiconductor-based system. J. Am. Chem. Soc. 136, 12568–12571. doi: 10.1021/ja506386e
Merupo, V. I., Velumani, S., Oza, G., Makowska-Janusik, M., and Kassiba, A. (2015). Structural, electronic and optical features of molybdenum-doped bismuth vanadium oxide. Mater. Sci. Semicond. Proc. 31, 618–623. doi: 10.1016/j.mssp.2014.12.057
Ng, Y. H., Iwase, A., Kudo, A., and Amal, R. (2010). Reducing graphene oxide on a visible-light BiVO4 photocatalyst for an enhanced photoelectrochemical water splitting. J. Phys. Chem. Lett. 1, 2607–2612. doi: 10.1021/jz100978u
Nikam, S., and Joshi, S. (2016). Irreversible phase transition in BiVO4 nanostructures synthesized by a polyol method and enhancement in photo degradation of methylene blue. RSC Adv. 6, 107463–107474. doi: 10.1039/C6RA14700C
Park, H. S., Kweon, K. E., Ye, H., Paek, E., Hwang, G. S., and Bard, A. J. (2011). Factors in the metal doping of BiVO4 for improved photoelectrocatalytic activity as studied by scanning electrochemical microscopy and first-principles density-functional calculation. J. Phys. Chem. C 115, 17870–17879. doi: 10.1021/jp204492r
Park, Y., McDonald, K. J., and Choi, K.-S. (2013). Progress in bismuth vanadate photoanodes for use in solar water oxidation. Chem. Soc. Rev. 42, 2321–2337. doi: 10.1039/C2CS35260E
Sasaki, Y., Nemoto, H., Saito, K., and Kudo, A. (2009). Solar water splitting using powdered photocatalysts driven by Z-schematic interparticle electron transfer without an electron mediator. J. Phys. Chem. C 113, 17536–17542. doi: 10.1021/jp907128k
Suarez, C. M., Hernández, S., and Russo, N. (2015). BiVO4 as photocatalyst for solar fuels production through water splitting: a short review. Appl. Catal. A Gen. 504, 158–170. doi: 10.1016/j.apcata.2014.11.044
Tokunaga, S., Kato, H., and Kudo, A. (2001). Selective preparation of monoclinic and tetragonal BiVO4 with scheelite structure and their photocatalytic properties. Chem. Mater. 13, 4624–4628. doi: 10.1021/cm0103390
Walsh, A., Yan, Y., Huda, M. N., Al-Jassim, M. M., and Wei, S.-H. (2009). Band edge electronic structure of BiVO4: elucidating the role of the Bi s and V d orbitals. Chem. Mater. 21, 547–551. doi: 10.1021/cm802894z
Wang, Q., Hisatomi, T., Jia, Q., Tokudome, H., Zhong, M., Wang, C., et al. (2016). Scalable water splitting on particulate photocatalyst sheets with a solar-to-hydrogen energy conversion efficiency exceeding 1%. Nat. Mater. 15, 611–615. doi: 10.1038/nmat4589
Ye, H., Park, H. S., and Bard, A. J. (2011). Screening of electrocatalysts for photoelectrochemical water oxidation on W-doped BiVO4 photocatalysts by scanning electrochemical microscopy. J. Phys. Chem. C 115, 12464–12470. doi: 10.1021/jp200852c
Keywords: bismuth vanadate, Zr-doping, water oxidation, crystalline structure, photocatalysis
Citation: Ikeda S, Kawaguchi T, Higuchi Y, Kawasaki N, Harada T, Remeika M, Islam MM and Sakurai T (2018) Effects of Zirconium Doping Into a Monoclinic Scheelite BiVO4 Crystal on Its Structural, Photocatalytic, and Photoelectrochemical Properties. Front. Chem. 6:266. doi: 10.3389/fchem.2018.00266
Received: 20 April 2018; Accepted: 12 June 2018;
Published: 02 July 2018.
Edited by:
Hadi Nur, University of Technology Malaysia, MalaysiaReviewed by:
Dong-Jik Kim, University of Warwick, United KingdomYing Dai, Shandong University, China
Copyright © 2018 Ikeda, Kawaguchi, Higuchi, Kawasaki, Harada, Remeika, Islam and Sakurai. This is an open-access article distributed under the terms of the Creative Commons Attribution License (CC BY). The use, distribution or reproduction in other forums is permitted, provided the original author(s) and the copyright owner(s) are credited and that the original publication in this journal is cited, in accordance with accepted academic practice. No use, distribution or reproduction is permitted which does not comply with these terms.
*Correspondence: Shigeru Ikeda, cy1pa2VkYUBjZW50ZXIua29uYW4tdS5hYy5qcA==