- 1Department of Chemistry, Faculty of Science, Naresuan University, Phitsanulok, Thailand
- 2Research Center for Academic Excellence in Petroleum, Petrochemicals and Advanced Materials, Naresuan University, Phitsanulok, Thailand
- 3Department of Industrial Engineering, Faculty of Engineering, Naresuan University, Phitsanulok, Thailand
- 4Centre of Excellence for Innovation and Technology for Water Treatment, Naresuan University, Phitsanulok, Thailand
- 5Department of Civil Engineering, Faculty of Engineering, Naresuan University, Phitsanulok, Thailand
- 6Department of Biochemistry, Faculty of Medical Science, Naresuan University, Phitsanulok, Thailand
- 7Materials Science Research Center, Faculty of Science, Chiang Mai University, Chiang Mai, Thailand
The silicon dioxide (SiO2)–coated bismuth vanadate (BiVO4) composites as visible–driven–photocatalysts were successfully synthesized by the co–precipitation method. The effects of SiO2 coating on the structure, optical property, morphology and surface properties of the composites were investigated by X–ray diffraction (XRD), UV–visible diffuse reflectance spectroscopy (DRS), transmission electron microscopy (TEM) and Brunauer–Emmette–Teller (BET) measurements. The photocatalytic activity of monoclinic BiVO4 and BiVO4/SiO2 composites were evaluated according to the degradation of methylene blue (MB) dye aqueous solution under visible light irradiation. The SiO2−coated BiVO4 composites showed the enhancing photocatalytic activity approximately threefold in comparison with the single phase BiVO4.
Introduction
Nowadays, the advanced oxidation process is known as an effective method for water purification and wastewater treatment. One of the most famous advanced oxidation process is heterogeneous photocatalysis; the contaminant (i.e., organic compounds) containing in the water and wastewater is finally degraded to carbon dioxide (Legrini et al., 1993; Mukherjee and Ray, 1999). This process can remove the organic contaminant perfectly and does not generate the second contaminant (i.e., sludge and other organic compounds) which are required the further treatment and disposal. According to the heterogeneous photocatalysis, the titanium dioxide (TiO2) has been played a role as the important catalyst to promote the photocatalytic activity. Due to its wide band gap of 3.2 eV, the photocatalyst of TiO2 is typically activated under the UV light (the wavelength <390 nm is required), which accounts for 45–50% of solar radiation (Linsebigler et al., 1995; Bahnemann et al., 2007; Devipriya et al., 2012). This theoretical fact becomes the limitation and non-cost-effectiveness of actual photocatalytic system for purifying the water at the site.
Another catalyst of monoclinic bismuth vanadate (BiVO4) has been proposed to overcome the drawback of photocatalytic system using TiO2 and together with enhance the photocatalytic activity during implementation. Since BiVO4 has narrow band gaps of 2.4 to 2.8 eV (Kudo et al., 2001; Xie et al., 2006; Li et al., 2008), this photocatalyst can be activated by the visible light and consequences the effective use of solar energy. However, the low specific surface area and poor surface textural property are the significant disadvantages of using BiVO4 as the catalyst. Its low surface area and adsorption capacity cause the low efficiency of photocatalytic system for organic contaminant removal and also the long treatment period required. Therefore, the increase in specific surface area of BiVO4 catalyst is necessary prior to imply the photocatalytic system to the actual wastewater.
Recently, alternative composite materials have been synthesized by combining metal oxide and porous materials (i.e., alumina, silica, zeolites, carbon black, charcoal) (Belessi et al., 2007; Wang et al., 2012; Xing et al., 2016) with the aim of improving the specific surface area, pore structure, and photocatalytic activity of catalysts (Gan et al., 2003; Kimura et al., 2003). For example, the enhancement of Ag–doped TiO2 photocatalytic activity was suggested by adding the mesoporous SiO2; the excellent efficiency of methyl orange (MO) removal was achieved by 2.5 h (Roldan et al., 2015). The increasing adsorption capacity of TiO2 catalyst was observed when the catalyst was combined with SiO2; the adsorption capacity was increased (Hu et al., 2012). The SiO2 addition also enhance the separation rate of electron–hole pairs under UV excitation. Further, the deposition of gold nanoparticles (Au) on the porous SiO2-WO3 composite can enhance the methylene blue (MB) adsorption capacity; the adsorption capacity of Au–SiO2-WO3 was greater than SiO2-WO3 and WO3 respectively (DePuccio et al., 2015). The complete MB removal was achieved by 300 min under visible light, and the fast kinetic of MB removal was found in Au–SiO2-WO3 catalyst, following by Au–WO3 and WO3 catalysts.
As all the above mentions, this study aimed to improve the surface morphology and photocatalytic activity of BiVO4 catalyst by coating SiO2. Various analytical techniques including X–ray diffraction (XRD), transmission electron microscopy (TEM), Brunauer–Emmett–Teller (BET) and UV–vis diffuse reflectance spectra (DRS) were used to clarify the better property of BiVO4/SiO2 composite rather than BiVO4 and SiO2. Further, the performance of BiVO4/SiO2 composites on wastewater treatment was preliminary studied in the batch test under visible light irradiation, and its performance was compared to the other two materials of BiVO4 and SiO2.
Experimental Procedure
All chemicals used were of analytical grade and were used as received without any further purification. The chemicals including tetraethyl orthosilicate (TEOS), bismuth (III) nitrate pentahydrate [Bi(NO3)3·5H2O], ammonium metavanadate (NH4VO3), methylene blue powder, sodium hydroxide pellet (NaOH), ammonia solution (28%) and nitric acid (37% HNO3) were obtained from Sigma-Aldrich. All solutions were prepared with deionized water.
Preparation of SiO2 Particles
SiO2 particles were prepared by the sol–gel method. Ammonia solution (28%) was added in 100 mL of a mixed solution of absolute ethanol/DI water (80: 20 v/v) and stirred under ultrasonic dispersion for 60 min. Then, 20 mL of tetraethyl orthosilicate (TEOS) was added drop by drop to the mixed solution and stirred for 120 min at room temperature. After the reaction was homogenized, the fine particles were separated by centrifugation with typical rotating speed of 6,000 rpm for 15 min, washed by DI water and dried at 80°C for 24 h in a hot air oven. Fine particles of SiO2 were obtained as a white powder following heat treatment at 500°C for 1 h in ambient.
Preparation of Monoclinic BiVO4 and SiO2-Coated BiVO4 Composites
Monoclinic BiVO4 were obtained by the co–precipitation method. Firstly, 12 mmol of bismuth (III) nitrate pentahydrate [Bi(NO3)3·5H2O] and the same volume of ammonium metavanadate (NH4VO3) were dissolved in 100 mL of 2 M nitric acid (HNO3) under vigorous stirring. The pH of the mixed solution was adjusted to 9 by adding 3 M sodium hydroxide (NaOH). The yellow precipitate was then separated by centrifugation at 6,000 rpm for 15 min, washed thoroughly with distilled water and ethanol and finally dried in a hot air oven at 80°C for 24 h. Crystalline monoclinic BiVO4 was formed after calcination at 550°C for 4 h.
BiVO4-coated SiO2 composites were also prepared by the same method for comparison with an additional step of adding SiO2 powder to 100 mL of 2 M HNO3.
Photocatalytic Reaction
Photocatalytic activities of the BiVO4, SiO2 and BiVO4/SiO2 composites were evaluated through degradation of methylene blue (MB) dye as a model organic pollutant under visible light. A total of 0.20 g of photocatalyst was added to 100 mL MB aqueous solution (initial concentration C0 = 20 ppm) under magnetic stirring in darkness for 60 min to achieve adsorption–desorption equilibrium. The system was irradiated by three 18 W halogen lamps (Essential MR, Philips, Thailand) to investigate photocatalytic degradation. Reduction of MB concentration over time (Ct) was recorded every 15 min by measuring the intensity change of the characteristic absorption peak at 664 nm using UV–vis double beam spectroscopy (UV−6100, Mapada).
Characterisation
Crystal phase and structure of the prepared samples were characterized by powder X–ray diffraction (XRD, Philips X'Pert MPD) using Cu Kα (λ = 1.54056 Å) radiation. Morphological changes in the composite materials were monitored by transmission electron microscopy (TEM, JSM−2010, JEOL). Brunauer–Emmett–Teller (BET) measurements (Adtosorb 1 MP, Quantachrome) were performed to compare the specific surface area of the BiVO4 and BiVO4/SiO2 composites. Measurement of UV–vis diffuse reflectance spectroscopy (DRS UV–vis, Shimadzu UV−3101PC) was carried out at room temperature to detect reflectance and absorbance spectra.
Results and Discussion
In Figure 1, the broad XRD peak at 2θ = 22–23° corresponded to the amorphous SiO2. The XRD pattern of BiVO4 without SiO2 was assigned to the standard monoclinic BiVO4 (JCPDS no. 14–0688) (Gotić et al., 2005). After coating BiVO4 with SiO2, the diffraction peaks matched well with the pure phase monoclinic BiVO4 and no peaks of any other phases or impurities were recorded. However, the diffraction intensity of BiVO4 decreased after coating SiO2, because the amorphous substance had the negative effect on crystallinity. Alternatively, self–doped Si4+ ions in the BiVO4 crystal structure might cause the decreasing crystallinity of BiVO4/SiO2 composites, and resulted in the broader peaks of the composite samples, which are similar to those reported by Phanichphant et al. (2016) for the binary composite CeO2/SiO2 photocatalyts and Kumar et al. for TiO2/SiO2 nanocomposites in solar cell applications (Arun Kumar et al., 2012).
As shown in Figure 2a, the BiVO4 demonstrated the absorption edge of the visible region at 450 nm, corresponding to the optical band gap (Eg) of 2.60 eV which was calculated by the Kubelka–Munk function (see Figure 2b) (Sirita et al., 2007). Compared to BiVO4/SiO2 composites, the value of the graph intercept was estimated at 2.30 eV, corresponding to the strong absorption edge in the visible region at 523 nm. The band gap energy of BiVO4 decreased from 2.60 to 2.30 eV in the composite materials, due to the influence of Si4+ ions doping into the lattice of BiVO4 which created the abundant doping energy levels. The estimated band gap values in this study was similar to those of BiVO4 reported by Jiang et al. (2012), who prepared the BiVO4 photocatalysts with different morphologies using the hydrothermal method. Liu et al. (2015) observed that the band gap energy of BiVO4/SiO2 catalyst estimated to be 2.32 eV, which was almost the same as that of calculate by this study.
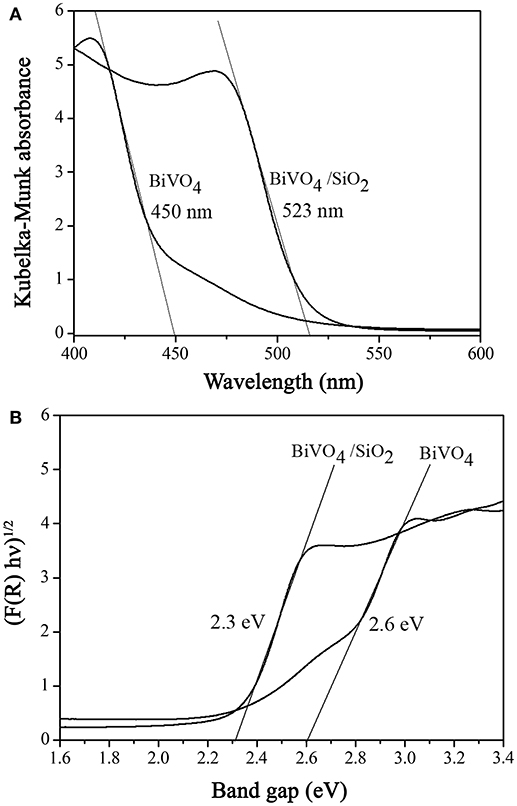
Figure 2. (A) Diffuse reflectance UV–visible spectra and (B) the plot of adsorption function vs. photon energy for determination of band gap (Eg).
The TEM images of SiO2, BiVO4 and BiVO4/SiO2 composites are presented in Figure 3. The SiO2 image shows the aggregation of spherical–shaped particles with diameters ranging of 20–30 nm (Figure 3a), while Figure 3b shows the rod–like nanostructures of monoclinic BiVO4 with the diameter of 10 nm and the length of 60 nm. Typical TEM images are used for characterizing the composite materials and proving the heterojunction formation between BiVO4 and SiO2, which demonstrated that the rod–like BiVO4 core was covered by the SiO2 particles growing on the surface (Figure 3c).
The N2 adsorption-desorption isotherms (Figure 4a) show that the N2 adsorption of BiVO4/SiO2 composites were relatively higher than that of the pure BiVO4, however the value was much lower than that of the SiO2. The specific surface areas of SiO2, BiVO4/SiO2 composites, and BiVO4 were found to be 106.9959, 37.6851, and 19.4964 m2/g, respectively. In the meanwhile, the pore size was calculated by using the BJH method, and the results were 9.0316, 11.0776, and 11.8111 nm for SiO2, BiVO4/SiO2, and BiVO4 respectively (as summarized in Table 1). The surface area and pore size are positively related to the photocatalytic activity, therefore the photocatalytic activity of BiVO4/SiO2 composites were higher than that of pure BiVO4. Even though the surface area of SiO2 was higher than the BiVO4/SiO2 composite, the adsorption of pollutant by SiO2 with high specific surface area have only the ability to transfer pollutants to alternative phases, but not completely get rid of them. Therefore, the photocatalytic process based on using the hydroxyl radicals is required in this study.
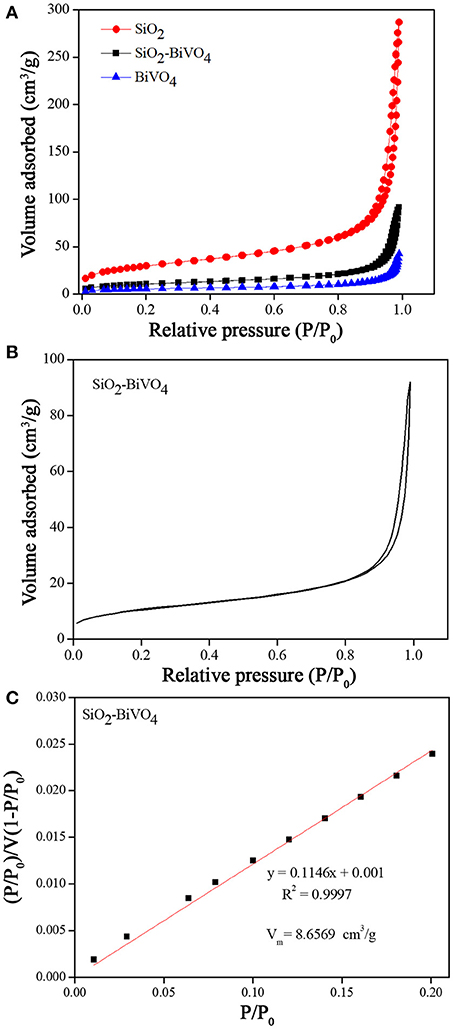
Figure 4. (A), (B) N2 adsorption-desorption isotherms, and (C) BET linear plot of relative pressure.
Figure 4b shows the N2 adsorption-desorption isotherms of BiVO4/SiO2 composites in the relative pressure (P/P0) range 0.00–1.00. The curve exhibited Type IV isotherm characteristic with a small hysteresis loop at the relative pressure of 0.80–1.00. This indicated the existence of mesopores in the sample with the pore diameter ranging of 2–50 nm (Brunauer et al., 1940; Bae et al., 2010).
The information from the isotherm can be used to determine the specific surface area from the mathematical relations in Equation (1) and Equation (2) below (Itodo et al., 2010; Thommes et al., 2015)
where,
P0, Initial pressure of N2; P, Equilibrium pressure of N2 adsorption; Vm, Monolayer capacity; V, Amount of N2 adsorbed at standard temperature and pressure (STP).
where,
A, Cross-sectional area of the adsorbed N2; m, Adsorbate molecular weight; Na, Avogadro's number.
The intercept and slope of the plot in Figure 4c were used to calculate the maximum volume of gas adsorbed at the monolayer (Vm), it was 8.6569 cm3/g. The specific surface area was also calculated via the Vm value (see Equation 2). The result showed that the surface area of BiVO4/SiO2 composites was 37.6851 m2/g.
Figure 5a presents the degradation efficiency of MB as a function of Ct/C0 and visible irradiation time. The C0 was the initial concentration of MB before irradiation and Ct was the MB concentration at the interval irradiation time (t, min). For using the SiO2 as catalyst, the MB was removed of 83% under the dark adsorption, and only 5% of MB was further degraded under the visible light. For using the single phase monoclinic BiVO4, the MB was removed around 10% under the dark adsorption, and 40% of MB was further degreased under the visible light irradiation. When the BiVO4/SiO2 composites was used, the MB removal efficiency reached 35 and 86% under the dark adsorption and visible light irradiation. As above explanation, the specific surface area of BiVO4/SiO2 composites were increased from BiVO4, due to the SiO2 coating. The increasing specific surface area resulted in the high adsorption of MB molecules during 60 min of the darkness, and then the adsorbed MB was continuously degraded by photocatalytic activity during visible light. These results illustrated that the photocatalytic activity of BiVO4 was enhanced by coating the SiO2 particles.
The kinetics of MB degradation was analyzed using the pseudo–first order model, which was given in Equation (3) (Yetim and Tekin, 2017). In Figure 5b, the correlation of–ln Ct/C0 and t were positive with linear equation; the kinetic constant (k) were 0.0073 min−1 for BiVO4 and 0.0207 min−1 for BiVO4/SiO2 composites. The kinetic constant of MB degradation using BiVO4/SiO2 composites was approximately threefold higher than that using the single phase BiVO4.
where k is the apparent rate constant of the pseudo–first order reaction (min−1).
Since the photocatalytic degradation of dyes is associated with dye adsorption onto the surface of BiVO4/SiO2. Furthermore, photocatalytic degradation occurs at or near the surface of the catalyst rather than in the bulk solution. Thus the higher photocatalytic activity of BiVO4/SiO2 is consistent with the higher adsorption of MB on the surface of BiVO4/SiO2 photocatalyst. Mesoporous SiO2 adsorbent enriches the MB molecules around the BiVO4 surface as shown in Figure 6 and the visible–light photocatalytic activity of the BiVO4 interface in the composite materials is then excited to generate electrons (e–) and holes (h+). Subsequently, photoexcited electrons in the valance band and hole in the conduction band of BiVO4 react with oxygen, water and hydroxide ions to produce free superoxide radicals (O) and hydroxyl radicals (OH•) as the main active oxidizing species, which then react with MB molecules during the photocatalytic process (Lin et al., 2014; Zhou et al., 2014). The final products of MB aqueous solution photocatalytic degradation are oxidized to CO2, H2O, CO2, NH, NO, and SO (Houas et al., 2001; Luan and Hu, 2012).
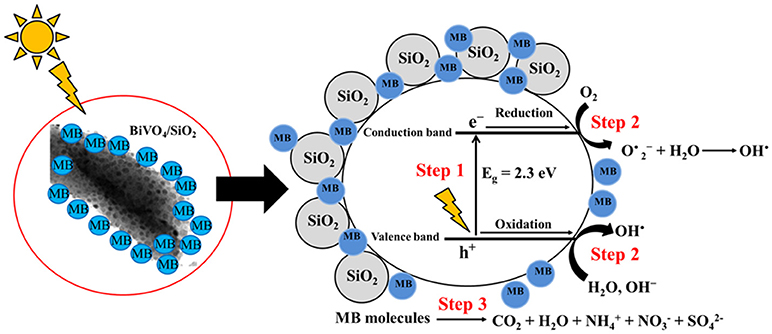
Figure 6. The proposed mechanism of photogenerated charge carriers of BiVO4 in BiVO4/SiO2 heterojunction.
Conclusions
BiVO4/SiO2 composites consisting of spherical SiO2 particles coated on BiVO4 nanorods were successfully prepared by co–precipitation. The composites exhibited higher photocatalytic activity compared to single monoclinic BiVO4 by degrading MB under visible–light irradiation due to the greater surface area of mesoporous SiO2. Fabrication of heterogeneous semiconductors using mesoporous materials can produce promising alternative photocatalysts for wastewater treatment under light irradiation by combining adsorption and photocatalytic processes.
Author Contributions
DC designed and performed the experiments and wrote the manuscript. SP, AN advised the data analysis and edited manuscript. PJ and WK advised the data analysis. All authors reviewed the approved the manuscript.
Conflict of Interest Statement
The authors declare that the research was conducted in the absence of any commercial or financial relationships that could be construed as a potential conflict of interest.
Acknowledgments
This work has been supported by grant from the National Research Council of Thailand (NRCT) under Grant No. R2561B075. The Office of the Higher Education Commission and the Thailand Research Fund (TRF) under Grant No. MRG6080097 is also acknowledged for financial support.
References
Arun Kumar, D., Merline Shyla, J., and Xavier, F. P. (2012). Synthesis and characterization of TiO2/SiO2 nano composites for solar cell applications. Appl. Nanosci. 2, 429–436. doi: 10.1007/s13204-012-0060-5
Bae, Y. S., Snurr, R. Q., and Yazaydin, O. (2010). Evaluation of the BET method for determining surface areas of MOFs and zeolites that contain ultra-micropores. Langmuir 26, 5479–5483. doi: 10.1021/la100449z
Bahnemann, W., Muneer, M., and Haque, M. M. (2007). Titanium dioxide-mediated photocatalysed degradation of few selected organic pollutants in aqueous suspensions. Catal. Today 124, 133–148. doi: 10.1016/j.cattod.2007.03.031
Belessi, V., Lambropoulou, D., and Konstantinou, I. (2007). Structure and photocatalytic performance of TiO2/clay nanocomposites for the degradation of dimethachlor. Appl. Catal. B. 73, 292–299. doi: 10.1016/j.apcatb.2006.12.011
Brunauer, S., Deming, L. S., Deming, W. E., and Teller, E. (1940). On a theory of the van der waals adsorption of gases. J. Am. Chem. Soc. 62, 1723–1732. doi: 10.1021/ja01864a025
DePuccio, D. P., Botella, P., O'Rourke, B., and Landry, C. C. (2015). Degradation of methylene blue using porous WO3, SiO2–WO3, and their Au-loaded analogs: adsorption and photocatalytic studies. ACS Appl. Mater. Interfaces 7, 1987–1996. doi: 10.1021/am507806a
Devipriya, S. P., Yesodharan, S., and Yesodharan, E. P. (2012). Solar photocatalytic removal of chemical and bacterial pollutants from water using Pt/TiO2-coated ceramic tiles. Int. J. Photoenergy 1–8. doi: 10.1155/2012/970474
Gan, L., Chen, L., and Zhang, X. (2003). Preparation of silica aerogels by non-supercritical drying. Acta Phys. Chim. Sin. 19, 504–508. doi: 10.3866/PKU.WHXB20030605
Gotić, M., Musić, S., Ivand, M., Šoufek, M., and Popovi, S. (2005). Synthesis and characterisation of bismuth (III) vanadate. J. Mol. Struct. 744, 535–540. doi: 10.1016/j.molstruc.2004.10.075
Houas, A., Lachheb, H., Ksibi, M., Elaloui, E., Guillard, C., and Herrmann, J. (2001). Photocatalytic degradation pathway of methylene blue in water. Appl. Catal. B 31, 145–157. doi: 10.1016/S0926-3373(00)00276-9
Hu, S., Li, F., and Fan, Z. (2012). Preparation of SiO2-coated TiO2 composite materials with enhanced photocatalytic activity under UV Light. Bull. Korean Chem. Soc. 33, 1895–1899. doi: 10.5012/bkcs.2012.33.6.1895
Itodo, A. U., Itodo, H. U., and Gafar, M. K. (2010). Estimation of specific surface area using langmuir isotherm method. J. Appl. Sci. Environ. Manage. 14, 141–145.
Jiang, H., Meng, X., Dai, H., Deng, J., Liu, Y., Zhang, L., et al. (2012). High performance porous spherical or octapod-like single-crystalline BiVO4 photocatalysts for the removal of phenol and methylene blue under visible-light illumination. J. Hazard. Mater. 217–218, 92–99. doi: 10.1016/j.jhazmat.2012.02.073
Kimura, I., Kase, T., Taguchi, Y., and Tanaka, M. (2003). Preparation of titania/silica composite microspheres by sol–gel process in reverse suspension. Mater. Res. Bull. 38, 585–597. doi: 10.1016/S0025-5408(03)00027-8
Kudo, A., Tokunaga, S., and Kato, H. (2001). Selective preparation of monoclinic and tetragonal BiVO4 with scheelite structure, and their photocatalytic properties. Chem. Mater. 13, 4624–4628. doi: 10.1021/cm0103390
Legrini, O., Oliveros, E., and Braun, A. M. (1993). Photochemical processes for water treatment. Chem. Rev. 93, 671–698. doi: 10.1021/cr00018a003
Li, G., Zhang, D., and Yu, J. C. (2008). Ordered Mesoporous BiVO4 through nanocasting: a superior visible light-driven photocatalyst. Chem. Mater. 20, 3983–3992. doi: 10.1021/cm800236z
Lin, H., Ye, H., Chen, S., and Chen, Y. (2014). One-pot hydrothermal synthesis of BiPO4/BiVO4 with enhanced visible-light photocatalytic activities for methylene blue degradation. RSC Adv. 4, 10968–10974. doi: 10.1039/c3ra45288c
Linsebigler, A. L., Lu, G., and Yates, J. T. (1995). Photocatalysis on TiO2 surfaces: principles, mechanisms, and selected results. Chem. Rev. 95, 735–758. doi: 10.1021/cr00035a013
Liu, B., Wang, Z., Zhou, S., and He, J. (2015). Synthesis and characterization of a novel BiVO4/SiO2 nanocomposites. Mater. Lett. 160, 218–221. doi: 10.1016/j.matlet.2015.07.104
Luan, J., and Hu, Z. (2012). Synthesis, property characterization, and photocatalytic activity of novel visible light-responsive photocatalyst Fe2BiSbO7. Int. J. Photoenergy 2012, 1–11.
Mukherjee, P. S., and Ray, A. K. (1999). Application photocatalysis for treatment of industrial waste water-A short review. Chem. Eng. Technol. 22, 253–260. doi: 10.1002/(SICI)1521-4125(199903)22:3< 253::AID-CEAT253>3.0.CO;2-X
Phanichphant, S., Nakaruk, A., and Channei, D. (2016). Photocatalytic activity of the binary composite CeO2/SiO2 for degradation of dye. Appl. Surf. Sci. 387, 214–220. doi: 10.1016/j.apsusc.2016.06.072
Roldan, M. V., Castro, Y., Pellegri, N., and Duran, A. (2015). Enhanced photocatalytic activity of mesoporous SiO2/TiO2 sol–gel coatings doped with Ag nanoparticles. J. Sol–Gel Sci. Technol. 76, 180–194. doi: 10.1007/s10971-015-3765-6
Sirita, J., Phanichphant, S., and Meunier, F. C. (2007). Quantitative analysis of adsorbate concentrations by diffuse reflectance FT-IR. Anal. Chem. 79, 3912–3918. doi: 10.1021/ac0702802
Thommes, M., Kaneko, K., Neimark, A. V., Olivier, J. P., Reinoso, F. R., Rouquerol, J., et al. (2015). Performance of an activated carbon-ammonia adsorption refrigeration system. Pure Appl. Chem. 87, 1051–1069.
Wang, X. D., Shi, F., Huang, W., and Fan, C. M. (2012). Synthesis of high quality TiO2 membranes on alumina supports and their photocatalytic activity. Thin Solid Films 520, 2488–2492. doi: 10.1016/j.tsf.2011.10.023
Xie, B., Zhang, H., Cai, P., Qiu, R., and Xiong, Y. (2006). Simultaneous photocatalytic reduction of Cr(VI) and oxidation of phenol over monoclinic BiVO4 under visible light irradiation. Chemosphere 63, 956–963. doi: 10.1016/j.chemosphere.2005.08.064
Xing, B., Shi, C., Zhang, C., Yi, G., Chen, L., Guo, H., et al. (2016). Preparation of TiO2/activated carbon composites for photocatalytic degradation of RhB under UV light irradiation. J. Nanomater. 2016, 1–10. doi: 10.1155/2016/8393648
Yetim, T., and Tekin, T. (2017). A kinetic study on photocatalytic and sonophotocatalytic degradation of textile dyes, Period. Polytech. Chem. Eng. 61, 102–108.
Keywords: composite materials, photocatalysis, BiVO4, BET isotherms, SiO2
Citation: Channei D, Nakaruk A, Khanitchaidecha W, Jannoey P and Phanichphant S (2018) Adsorption and Photocatalytic Processes of Mesoporous SiO2-Coated Monoclinic BiVO4. Front. Chem. 6:415. doi: 10.3389/fchem.2018.00415
Received: 09 March 2018; Accepted: 23 August 2018;
Published: 19 September 2018.
Edited by:
Hongqi Sun, Edith Cowan University, AustraliaReviewed by:
Priyabrat Mohapatra, C. V. Raman College of Engineering, IndiaM. Nageeb Rashed, Aswan University, Egypt
Copyright © 2018 Channei, Nakaruk, Khanitchaidecha, Jannoey and Phanichphant. This is an open-access article distributed under the terms of the Creative Commons Attribution License (CC BY). The use, distribution or reproduction in other forums is permitted, provided the original author(s) and the copyright owner(s) are credited and that the original publication in this journal is cited, in accordance with accepted academic practice. No use, distribution or reproduction is permitted which does not comply with these terms.
*Correspondence: Duangdao Channei, ZHVhbmdkYW9jQG51LmFjLnRo