- 1School of Materials Science and Engineering, Jiangsu Collaborative Innovation Center of Photovolatic Science and Engineering, Changzhou University, Changzhou, China
- 2Hunan Province Cooperative Innovation Center for The Construction and Development of Dongting Lake Ecological Economic Zone, College of Chemistry and Materials Engineering, Hunan University of Arts and Science, Changde, China
Folic acid is first time applied as a carbon-nitrogen precursor to fabricate Li4Ti5O12@CN composites via ball milling Nano-TiO2, Li2CO3 and folic acid with ethanol as solvent, and then followed by heating treatment in argon. XRD, SEM, TEM, XPS, charge-discharge test and EIS are used to evaluate the influence of N-doped carbon coating on its structure, morphologies and electrochemical property. It is demonstrated that the N-doped carbon coated Li4Ti5O12 composite exhibits superior high-rate performance compared with pure Li4Ti5O12. It possesses a high discharge capacity of 174, 165 mAh g−1 at 0.5 and 10 C, respectively. Additionally, an initial specific capacity of 96.2% is obtained after 200 cycles at 10 C. The remarkable performance might be put down to the N-doped carbon layer providing efficiently electron conductive network and nanosized decreasing lithium ion diffusion path.
Introduction
In the past few years, carbon materials have been regarded as the most commercially valued lithium battery anode material (Ma et al., 2016; Long et al., 2017; Li et al., 2019; Wu et al., 2019). Unfortunately, its low lithiation potential (~0.2 V vs. Li+/Li) will result in the activation of dendritic lithium, thus further creating safety problems. Moreover, insufficient diffusion coefficient of lithium ion and large volume change will result in poor rate performance and cycle stability (Han et al., 2018; Yi et al., 2018). Therefore, it is essential to find alternative anodes with excellent electrochemical properties and outstanding safety characteristics. Among the reported alternative candidates, Spinel lithium titanium (Li4Ti5O12) is suggested as a potential anode material used in lithium-ion batteries (LIBs) due to the following reasons: (i) The higher flat discharge and charge plateau (1.55 V vs. Li+/Li) can prevent lithium metal dendrites from evolving during the electrochemical reaction process (Wang S. et al., 2017). (ii) Small volume expansion provides superior cycle stability and reversibility among charge and discharge process (Tian et al., 2017; Wang Q. et al., 2017). Nevertheless, its small lithium ion diffusion coefficients (10−9 ~10−13 cm2 S−1) and weak electrical conductivity (~10−13 S cm−1) (Jiang et al., 2017), resulting in serious electrode polarization and poor capability at high current density, has been considered as the main bottleneck of its commercial application for high-power LIBs. In order to overcover this problem, several strategies have been suggested by researchers, including synthesis of Li4Ti5O12 with porous structure (Lu et al., 2017), coating the surface of Li4Ti5O12 particles with conductive materials (Tang et al., 2017), construction of nanoscale particle size (Chiu et al., 2017), and introduction of metal and nonmetal ion into Li4Ti5O12 (Chen et al., 2017; Cheng et al., 2017;Liang et al., 2017).
Thin carbon coating on the surface of Li4Ti5O12 particles has been considered as an effective method to enhance its electrochemical properties in all reported literatures, since the carbon coating can both improve the surface electron conductivity and inhibit the growth of primary particles in the heat treatment process, this further leads to faster lithium ion diffusion. Very recently, the introduction of nitrogen-doped carbon to modify the electrode materials has been received more and more attention. It is well-known that N atoms can provide additional electrons to further increase the conductivity of the coated carbon layer (Jiang et al., 2017; Xu et al., 2017). In addition, N-doped is favorable to reduce the barrier of energy of lithium-ion penetration and enhance reaction sites (Xiong et al., 2018). We have just proposed folic acid as a new carbon nitrogen precursor to prepare Na3V2(PO4)3@CN composite material, it possesses excellent rate performance and excellent cycling performance when used as cathode electrode of sodium ion batteries. In this work, a similar strategy was suggested to prepared Li4Ti5O12@CN composite. It is well-expected that the as-prepared Li4Ti5O12@CN sample will possess excellent electrochemical characters.
Experimental
Material Preparation
Li4Ti5O12@CN composite was synthesized via a solid-state process, and the detail process was described as following. Firstly, 2.2281 g of Li2CO3 (99.5%),5.9985 g of TiO2 (99.9%) and 1 g of folic acid were mixed by ball milling in ethyl alcohol for 6 h. Secondly, the solvent was evaporated by drying the ready-prepared mixture at 80°C for 2 h. Thirdly, the synthesized precursor was heated under argon atmosphere at 750°C for 8 h. For comparison, pure Li4Ti5O12 was also synthesized via the similar method without using folic acid as the starting material.
Characterization of Materials
X-ray diffraction instrument was used to investigate the structure and composition of both as-prepared Li4Ti5O12 samples. The surface chemical states of Li4Ti5O12@CN were identified by XPS. SEM was used for observing morphologies of the two ready-prepared Li4Ti5O12 samples. N-doped carbon layer was further investigated by TEM. Elemental analyzer was carried out to investigate the content of carbon and nitrogen for Li4Ti5O12@CN composite. Four-point probe method was used to investigate the electronic conductivities of both Li4Ti5O12 samples.
Electrochemical Measurements
The fabricated working electrodes consisted of as-synthesized Li4Ti5O12 sample, LA-132 and Super-P in a weight ratio of 85:5:10. The mixture was uniformly casted onto the aluminum foil and then dried in vacuum. CR2032 coin type cells were prepared in glove box with filled argon by composing of lithium piece as the counter electrode, 1 mol/L LiPF6 in EC/DEC/DMC (1:1:1 in volume) as the electrolyte. Celgard 2400 as the separator,. LAND CT2001 system were used to investigate the charge-discharge experiments between the potential range of 1–3V. Electrochemical impedance spectra (EIS) were investigated by using CHI600E electrochemical station in a frequency range of 10−2−105 Hz.
Results and Discussion
Crystal structure of both as-obtained Li4Ti5O12 samples was characterized via XRD, and the results are plotted in Figure 1. It clearly verifies that the dominating diffraction peaks of both as-synthesized Li4Ti5O12 samples are in consistence with the base peaks of spinel Li4Ti5O12 (PDF No.49-0207), demonstrating that the nitrogen-doped carbon layer coating process has little effect on the formation of spinel Li4Ti5O12 (Li et al., 2013a; Chang et al., 2014; Wang P. et al., 2017). Perhaps due to the amorphous morphology of carbon, the diffraction peak relative to carbon was not observed (Xu et al., 2017; Liu et al., 2018). The detail of the enlarged peak corresponding to Li4Ti5O12 (111) plane was described in Figure 1B. As clearly found that the central position of this peak shifts to larger angles after N-doped carbon coating, suggesting that nitrogen atoms possibly doped into the Li4Ti5O12 lattice and form a new thin layer of TiNx, similar phenomenon was also reported by Li et al. (Zhang et al., 2013). In addition, the intensity of the peaks in Li4Ti5O12@CN composite is lower than that of pure Li4Ti5O12, indicating that the N-doped carbon coating on the surface of Li4Ti5O12 will prevent the growth of particles. In order to investigate the content of carbon and nitrogen in Li4Ti5O12@CN composite, elemental analysis measurement is carried out. The content of carbon and nitrogen for Li4Ti5O12@CN composite is 1.46 and 0.24%, respectively.
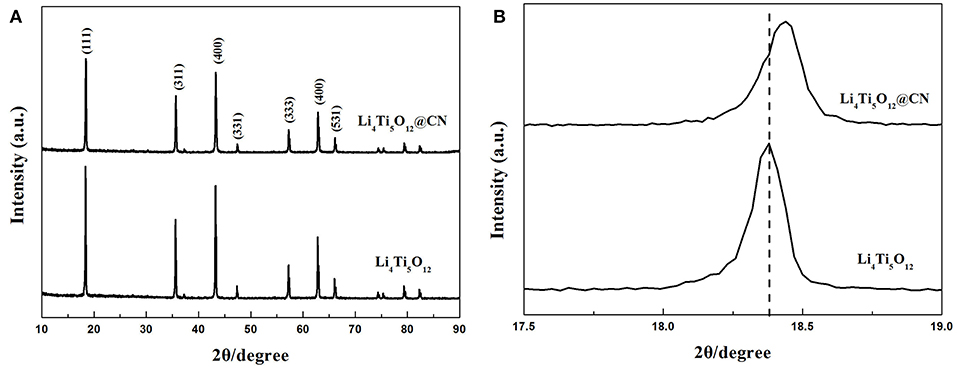
Figure 1. (A) X-ray diffraction profiles of Li4Ti5O12 and Li4Ti5O12@CN composite, (B) Enlarged (111) peak of Li4Ti5O12 and Li4Ti5O12@CN composite.
The obtained results of surface chemical state of Li4Ti5O12@CN composite evaluated by X-ray photoelectron spectroscopy (XPS) are given in Figure 2. Li1s, Ti2p, O1s, C1s, and N1s peaks are observed from the XPS spectra of Li4Ti5O12@CN composite (Figure 2A). The high-resolution N1s of Li4Ti5O12@CN composite is demonstrated in Figure 2B. As illustrated in Figure 2B, pyridine (N1), pyrrole (N2) and graphitic (N3) correspond to peaks centered at 398.2, 399.8, and 401.4eV, respectively. (Li H. et al., 2014; Long et al., 2015; Wang et al., 2015). The above results clearly showed that nitrogen-doping is successfully introduced in the carbon layer by using folic acid as a carbon-nitrogen precursor, which might result in produce the flaws in the symmetric offset spread of aromatic rings carbon, and thus further increase the diffusion of Li+ in the interface (Li et al., 2013a; Wang et al., 2015). Additionally, a peak at about 397 eV being attributed to the interaction energy of TiN is observed in Figure 2B, indicating that titanium nitride (TiN) phase is created during the sintering process (Wan et al., 2012). As well-accepted, the existence of TiN with a metallic conductivity will improve electronic conductivity (Li H. et al., 2014). The high-resolution Ti2p of Li4Ti5O12@CN composite is depicted in Figure 2C. Clearly, two peaks appeared at approximately 464.2 and 458.5eV are observed, which represents to peaks of Ti 2p1/2 and Ti 2p3/2 of Ti4+ in the sample (Li et al., 2014). In addition, two extra peaks located at about 459 and 464.7eV are detected, corresponding to the peaks of Ti 2p1/2 and Ti 2p3/2 of Ti3+ in the sample, respectively, which suggests that Ti3+ sites were introduced in the Li4Ti5O12@CN composite due to the reduced ability of N-doped carbon. Similar phenomena were also demonstrated in previous reports (Wan et al., 2012; Ming et al., 2014). It further verified that the titanium nitride (TiN) phase was formed.
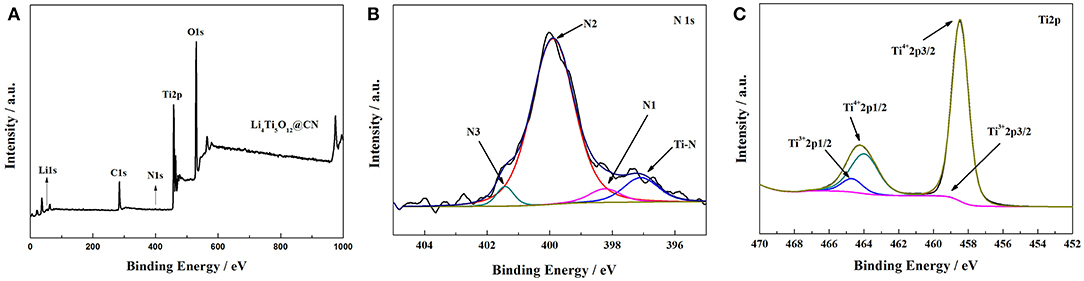
Figure 2. (A) X-ray photoelectron spectroscopy survey spectra of Li4Ti5O12@CN composite, (B) high-resolution XPS spectra of N1s, (C) high-resolution XPS spectra of Ti2p.
The different morphology and particle size between Li4Ti5O12 and Li4Ti5O12@CN composites were investigated by SEM characterization. The results are shown in Figure 3. As clearly demonstrated in Figure 3, Li4Ti5O12@CN composite possesses a much smaller particle size than that of Li4Ti5O12. It is well-accepted that in situ N-doped carbon coating will well-prohibit the Li4Ti5O12 particles from growing into larger crystals (Wang C. et al., 2014). The SEM results agree well with the XRD results. To further verify this prediction, the specific surface area of both as-obtained Li4Ti5O12 samples was investigated. Li4Ti5O12@CN composite and pure Li4Ti5O12 possess a specific surface area of 12.75 and 7.08 m2 g−1, respectively. Generally, much smaller particles of Li4Ti5O12@CN composite gives the larger specific surface area. As well-accepted that fabrication of material with much smaller particle size and larger specific surface area will decrease the lithium ion diffusion pathways and thus enhance the kinetics of lithiation/delithiation (Long et al., 2015). The carbon-nitrogen layer of Li4Ti5O12@CN composite was further studied by TEM characterization and results are demonstrated in Figure 4. It was found that a carbon-nitrogen layer with a thickness of 2 to 5 nm is formed on the exterior of Li4Ti5O12. The electronic conductivities of the as-synthesized Li4Ti5O12 samples were confirmed by four-point probe method. Pure Li4Ti5O12 and Li4Ti5O12@CN composite have the electronic conductivities of 7.67 × 10−5 and 1.06 × 10−2 S cm−1, respectively.
The first charge-discharge cycle for as-synthesized Li4Ti5O12 and Li4Ti5O12@CN composite at a rate of 0.5 C between a voltage range of 1–3V are described in Figure 5. Both as-prepared Li4Ti5O12 samples possess a voltage plateau at ~1.55V, suggesting a two-phase reaction is carried out on the basis of the redox pair of Ti3+/Ti4+ (Wang et al., 2012; Wang B. et al., 2014). However, the potential separation between the charge and discharge curves of Li4Ti5O12@CN composite material is much smaller than that of Li4Ti5O12, indicating that the Li4Ti5O12@CN electrode has less polarization and better reaction kinetics, which demonstrates that the enhancement of electrical conductivity of Li4Ti5O12 after introducing N-doped carbon coating (Li et al., 2013a; Zhang et al., 2014).
The rate capabilities of Li4Ti5O12@CN composite and Li4Ti5O12 are shown in Figure 6. Pure Li4Ti5O12 obtained a discharge capacity of 158 mAh g−1 at a low rate of 0.5 C, and the capacity decreases remarkably as the rate increased from 0.5 to 1, 2, 5, and 10 C, respectively. Especially, its discharge capacity is only 79 mAh g−1 at 10 C. The poor rate properties of pure Li4Ti5O12 could be due to its poor conductivity. While much-improved discharge capacity at each rate for Li4Ti5O12@CN composite in comparison with pure Li4Ti5O12. At 0.5, 5, and 10 C, its discharge capacity were 174, 168, and 165 mAh g−1, respectively. The superior rate properties of Li4Ti5O12@CN composite could be due to three reasons: (i) As demonstrated in SEM results (Seen in Figure 4), the smaller particle size for Li4Ti5O12@CN composite is favorable for the faster Li+ diffusion and further enhancement of kinetic coefficient of lithium ion embedded into Li4Ti5O12 structure (Long et al., 2015). (ii) The electronic conductivity of Li4Ti5O12 is deemed to be enhanced by N-doped carbon coating (Xu et al., 2017), and the defects in the carbon layer caused by N-doping facilitate Li+ migration in the interface (Li et al., 2013b). (iii) The electronic conductivity properties of Li4Ti5O12 will be further increased with the existence of TiNx in the composite.
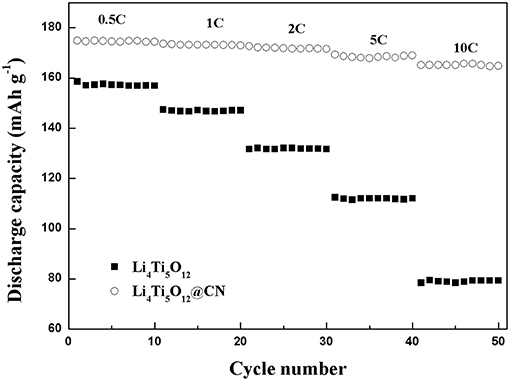
Figure 6. Rate property of Li4Ti5O12 and Li4Ti5O12@CN composite at different rates from 0.5 C to10 C.
The cyclic property of Li4Ti5O12@CN composite at a rate of 10 C is depicted in Figure 7. As clearly seen, the initial discharge capacity of Li4Ti5O12@CN composite is 165 mAh g−1, and 96.2% of its capacity is obtained after 200 cycles, suggesting that the as-synthesized Li4Ti5O12@CN composite possesses good cycle stability.
To further investigate the effect of N-doped carbon coating on electrode behavior, electrochemical impedance spectroscopy measurements were performed on Li4Ti5O12 and Li4Ti5O12@CN electrodes, and the results are plotted in Figure 8. Obviously, each electrode exhibits a similar profile with a straight line at the low frequency region and a depressed semicircle at the high-middle frequency range, being correlated with Li-ion diffusion and charges transfer resistance (Rct) in the electrode/electrolyte interface, respectively (Zheng et al., 2018; An et al., 2019; He et al., 2019; Lv et al., 2019; Xiao et al., 2019; Zhou et al., 2019). As obtained from Figure 8, Li4Ti5O12@CN electrode exhibits much smaller charge-transfer resistance of 52 Ω than that of 194 Ω for pure Li4Ti5O12 electrode, suggesting the improved electronic conductivity of Li4Ti5O12@CN composite in comparison with pure Li4Ti5O12 caused by the highly electronic conductive coating with N-doped carbon as well as the presence of TiNx phase (Zhou et al., 2017). According to the following equation, the Li-ion diffusion coefficient of both as-prepared Li4Ti5O12 samples can be obtained:
The value of σ for both samples could be obtained from the lines described in Figure 9. Based on the above equations and the results from Figure 9, the obtained lithium ion diffusion coefficient for Li4Ti5O12 and Li4Ti5O12@CN composite is 6.58 × 10−11 and 2.89 × 10−9 cm2 s−1, respectively. There is no doubt that the Li4Ti5O12@CN electrode has a larger Li-ion diffusion coefficient due to the smaller particle size and the carbon layer defect of the Li4Ti5O12@CN composite, indicating that a valid enhancement of the diffusion kinetics of Li4Ti5O12 after the introduction of the N-doped carbon coating. Based on the above results and discussion, coating with N-doped carbon can dramatically improve the lithium ion and conductive property. Consequently, Li4Ti5O12@CN composite has much improved electrochemical performance in compassion with pure Li4Ti5O12.
Conclusions
In this study, N-doped carbon-coated Li4Ti5O12 is prepared with folic acid as a carbon-nitrogen source. Li4Ti5O12@CN composite has the outstanding reversible capacity, high rate capability in compassion with pure Li4Ti5O12. The discharge capacity of the Li4Ti5O12@CN composite at 10 C was 165 mAh g−1, and the initial specific capacity remained at 96.2% after 200 cycles. The superior properties of Li4Ti5O12@CN composite could be owing to the improved electronic conductivity caused by the N-doped carbon layer and the TiN phase as well as enhanced Li-ion diffusion coefficient rising from the smaller particle size and the defects in the carbon layer.
Data Availability
The raw data supporting the conclusions of this manuscript will be made available by the authors, without undue reservation, to any qualified researcher.
Author Contributions
XH and YR contributed conception and design of the study. HX carried out experiments and wrote the manuscript. XD performed analyzed experimental results. SZ revised the manuscript.
Funding
This project was financially supported by the National Nature Science Foundation of China (Nos. 21771062, 21576030 and U1607127), the Research Fund of Hunan Provincial Education Department (18A366), the Opening Project of Material Corrosion and Protection Key Laboratory of Sichuan Province of China (No. 2018CL15).
Conflict of Interest Statement
The authors declare that the research was conducted in the absence of any commercial or financial relationships that could be construed as a potential conflict of interest.
References
An, C. S., Yuan, Y. F., Zhang, B., Tang, L. B., Xiao, B., Lu, J., et al. (2019). Graphene wrapped FeSe2 nano-microspheres with high pseudocapacitive contribution for enhanced na-ion storage. Adv. Energy Mater. 2019:1900356. doi: 10.1002/aenm.201900356
Chang, L. J., Luo, S. H., Zhang, H. L., Qi, X. W., Wang, Z. Y., Liu, Y. G., et al. (2014). Synthesis and performance of Li4Ti5O12 anode materials using the PVP-assisted combustion method. Chin. Chem. Lett. 25, 1569–1572. doi: 10.1016/j.cclet.2014.09.002
Chen, C., Liu, X., Ai, C., and Wu, Y. (2017). Enhanced lithium storage capability of Li4Ti5O12 anode material with low content Ce modification. J. Alloy. Compd. 714, 71–78. doi: 10.1016/j.jallcom.2017.04.184
Cheng, Q., Tang, S., Liu, C., Lan, Q., Zhao, J., Liang, J., et al. (2017). Preparation and electrochemical performance of Li4−xMgxTi5O12 as anode materials for lithium-ion battery. J. Alloy. Compd. 722, 229–234. doi: 10.1016/j.jallcom.2017.06.040
Chiu, H. C., Lu, X., Zhou, J., Gu, L., Reid, J., Gauvin, R., et al. (2017). Annealing-regulated elimination of residual strain-induced structural relaxation for stable high-power Li4Ti5O12 nanosheet anodes. Nano Energy 32, 533–541. doi: 10.1016/j.nanoen.2016.12.063
Han, X., Gui, X., Yi, T. F., Li, Y. W., and Yue, C. B. (2018). Recent progress of NiCo2O4-based anodes for high-performance lithium-ion batteries. Curr. Opin. Solid State Mater. Sci. 22, 109–126. doi: 10.1016/j.cossms.2018.05.005
He, Z., Li, M., Li, Y., Li, C., Yi, Z., Zhu, J., et al. (2019). ZrO2 nanoparticle embedded carbon nanofibers by electrospinning technique as advanced negative electrode materials for vanadium redox flow battery. Electrochim Acta 309, 166–176. doi: 10.1016/j.electacta.2019.04.100
Jiang, C., Liu, S., Lian, Q., Zhao, J., Ding, W., Yu, Z., et al. (2017). Nitrogen-doped carbon-coated hierarchical Li4Ti5O12-TiO2 hybrid microspheres as excellent high rate anode of Li-ion battery. Ceram. Int. 43, 11354–11360. doi: 10.1016/j.ceramint.2017.05.341
Li, H., Shen, L., Wang, J., Ding, B., Nie, P., Xu, G., et al. (2014). Design of a nitrogen-doped, carbon-coated Li4Ti5O12 nanocomposite with a core-shell structure and its application for high-rate lithium-ion batteries, ChemPlusChem 79, 128–133. doi: 10.1002/cplu.201300316
Li, H., Shen, L., Yin, K., Ji, J., Wang, J., Wang, X., et al. (2013b). Facile synthesis of N-doped carbon-coated Li4Ti5O12 microspheres using polydopamine as a carbon source for high rate lithium ion batteries. J. Mater. Chem. A 1, 7270–7276. doi: 10.1039/c3ta10623c
Li, H., Shen, L., Zhang, X., Wang, J., Nie, P., Che, Q., et al. (2013a). Nitrogen-doped carbon coated Li4Ti5O12 nanocomposite: superior anode materials for rechargeable lithium ion batteries. Power Sour. J. 221, 122–127. doi: 10.1016/j.jpowsour.2012.08.032
Li, R., Xiao, W., Miao, C., Fang, R., Wang, Z., and Zhang, M. (2019). Sphere-like SnO2/TiO2 composites as high-performance anodes for lithium ion batteries. Ceramics Int. 45, 13530–13535. doi: 10.1016/j.ceramint.2019.04.059
Li, X., Lin, H., Cui, W., Xiao, Q., and Zhao, J. (2014). Fast solution-combustion synthesis of nitrogen-modified Li4Ti5O12 nanomaterials with improved electrochemical performance. ACS Appl. Mater. Interfaces 6, 7895–7901. doi: 10.1021/am501220f
Liang, Q., Cao, N., Song, Z., Gao, X., Hou, L., Guo, T., et al. (2017). Co-doped Li4Ti5O12 nanosheets with enhanced rate performance for lithium-ion batteries. Electrochim. Acta 251, 407–414. doi: 10.1016/j.electacta.2017.08.121
Liu, H., Su, C., Li, X., and Guo, Y. (2018). Fabrication of N-doped carbon-coated Li4Ti5−xCoxO12 anode for lithium-ion batteries. Solid State Ionics 320, 113–117. doi: 10.1016/j.ssi.2018.02.035
Long, D. H., Jeong, M. G., Lee, Y. S., Choi, W., Lee, J. K., Oh, I. H., et al. (2015). Coating lithium titanate with nitrogen-doped carbon by simple refluxing for high-power lithium-ion batteries. ACS Appl. Mater. Interfaces 7, 10250–10257. doi: 10.1021/acsami.5b00776
Long, W., Fang, B., Ignaszak, A., Wu, Z., Wang, Y. J., and Wilkinson, D. (2017). Biomass-derived nanostructured carbons and their composites as anode materials for lithium ion batteries. Chem. Soc. Rev. 46, 7176–7190. doi: 10.1039/C6CS00639F
Lu, M., Yang, H., Zhang, Y., Wang, G., and Zhuang, S. (2017). Novel insights into the charge/discharge characteristics of spherical porous Li4Ti5O12 co-coated with carbon and metal. Power Sour. J. 349, 52–56. doi: 10.1016/j.jpowsour.2017.03.027
Lv, Y. R., Zhang, L., Cheng, G., Wang, P. F., Zhang, T. Z., Wang, L., et al. (2019). Preparation of carbon nanosheet by molten salt route and its application in catalyzing VO2+/V redox reaction. J. Electrochem. 166, A953–A959. doi: 10.1149/2.0371906jes
Ma, Z., Cui, Y., Xiao, X., Deng, Y., Song, X., Zuo, X., et al. (2016). A reconstructed graphite-like carbon micro/nano-structure with higher capacity and comparative voltage plateau of graphite. J. Mater. Chem. A 4, 11462–11471. doi: 10.1039/C6TA02195F
Ming, H., Ming, J., Li, X., Zhou, Q., Wang, H., Jin, L., et al. (2014). Hierarchical Li4Ti5O12 particles co-modified with C&N towardsenhanced performance in lithium-ion battery applications. Electrochim. Acta 116, 224–229. doi: 10.1016/j.electacta.2013.11.038
Tang, B., Li, A., Tong, Y., Song, H., Chen, X., Zhou, J., et al. (2017). Carbon-coated Li4Ti5O12 tablets derived from metal-organic frameworks as anode material for lithium-ion batteries. J. Alloy. Compd. 708, 6–13. doi: 10.1016/j.jallcom.2017.02.279
Tian, Q., Chen, P., Zhang, Z., and Yang, L. (2017). Achievement of significantly improved lithium storage for novel clew-like Li4Ti5O12 anode assembled by ultrafine nanowires. Power Sour. J. 350, 49–55. doi: 10.1016/j.jpowsour.2017.03.065
Wan, Z., Cai, R., Jiang, S., and Shao, Z. (2012). Nitrogen- and TiN-modified Li4Ti5O12: one-step synthesis and electrochemical performance optimization. J. Mater. Chem. 22, 17773–17781. doi: 10.1039/c2jm33346e
Wang, B., Wang, J., Cao, J., Ge, H., and Tang, Y. (2014). Nitrogen-doped Li4Ti5O12 nanosheets with enhanced lithium storage properties. Power Sources J. 266, 150–154. doi: 10.1016/j.jpowsour.2014.05.009
Wang, C., Li, H., Fu, A., Liu, J., Ye, W., Guo, P., et al. (2014). An RAPET approach to in situ synthesis of carbon modified Li4Ti5O12 anode nanocrystals with improved conductivity. N. Chem. J. 38, 616–623. doi: 10.1039/C3NJ01319G
Wang, F., Luo, L., Du, J., Guo, L., Lia, B., and Ding, Y. (2015). Nitrogen-doped carbon decorated Li4Ti5O12 composites as anode materials for high performance lithium-ion batteries. RSC Adv. 5, 46359–46365. doi: 10.1039/C5RA05989E
Wang, L., Zhang, Z., Liang, G., Ou, X., and Xu, Y. (2012). Synthesis and electrochemical performance of Li4Ti5O12/C composite by a starch sol assisted method. Powder Technol. 215–216, 79–84. doi: 10.1016/j.powtec.2011.09.011
Wang, P., Zhang, G., Cheng, J., You, Y., Li, Y. K., Ding, C., et al. (2017). Facile Synthesis of Carbon-coated spinel Li4Ti5O12/rutile-TiO2 composites as an improved anode material in full lithium-ion batteries with LiFePO4@N-doped carbon cathode. ACS Appl. Mater. Interfaces 9, 6138–6143. doi: 10.1021/acsami.6b15982
Wang, Q., Zhang, J., Liu, W., Xie, X., and Xia, B. (2017). Quantitative investigation of the gassing behavior in cylindrical Li4Ti5O12 batteries. Power Sour. J. 343, 564–570. doi: 10.1016/j.jpowsour.2017.01.073
Wang, S., Yang, Y., Quan, W., Hong, Y., Zhang, Z., Tang, Z., et al. (2017). Ti3+-free three-phase Li4Ti5O12/TiO2 for high-rate lithium ion batteries: capacity and conductivity enhancement by phase boundaries. Nano Energy 32, 294–301. doi: 10.1016/j.nanoen.2016.12.052
Wu, L., Zheng, J., Wang, L., Xiong, X. H., Shao, Y. Y., Wu, M. H., et al. (2019). PPy-encapsulated SnS2 nanosheets stabilized by defects on a TiO2 support as a durable anode material for lithium-ion batteries. Angew. Chem. Int. Ed. 58, 811–815. doi: 10.1002/anie.201811784
Xiao, B., Wang, P. B., He, Z. J., Yang, Z., Tang, L. B., Zheng, J. C., et al. (2019). Effect of MgO and TiO2 coating on the electrochemical performance of li-rich cathode materials for lithium-ion Batteries. Energy Technol. 2019:1800829. doi: 10.1002/ente.201800829
Xiong, Q. Q., Lou, J. J., Teng, X. J., Lu, X. X., Liu, S. Y., Chi, H. Z., et al. (2018). Controllable synthesis of N-C@LiFePO4 nanospheres as advanced cathode of lithium ion batteries. J. Alloy. Compd. 743, 377–382. doi: 10.1016/j.jallcom.2018.01.350
Xu, G., Quan, X., Gao, H., Li, J., Cai, Y., Cheng, X., et al. (2017). Facile spray drying route for large scale nitrogen-doped carbon-coated Li4Ti5O12 anode material in lithium-ion batteries. Solid State Ionics 304, 40–45. doi: 10.1016/j.ssi.2017.03.018
Yi, T. F., Zhu, Y. R., Tao, W., Luo, S. H., Xie, Y., and Li, X. F. (2018). Recent advances in the research of MLi2Ti6O14 (M = 2Na, Sr, Ba, Pb) anode materials for Li-ion batteries. Power Sour. J. 399, 26–41. doi: 10.1016/j.jpowsour.2018.07.086
Zhang, H., Deng, Q., Mou, C., Huang, Z., Wang, Y., Zhou, A., et al. (2013). Surface structure and high-rate performance of spinel Li4Ti5O12 coated with N-doped carbon as anode material for lithium-ion batteries. Power Sour. J. 239, 538–545. doi: 10.1016/j.jpowsour.2013.03.013
Zhang, J., Zhang, J., Peng, Z., Cai, W., Yu, L., Wu, Z., et al. (2014). Outstanding rate capability and long cycle stability induced by homogeneous distribution of nitrogen doped carbon and titanium nitride on the surface and in the bulk of spinel lithium titanate. Electrochim. Acta 132, 230–238. doi: 10.1016/j.electacta.2014.03.007
Zheng, J. C., Yang, Z., He, Z. J., Tong, H., Yu, W. J., and Zhang, J. F. (2018). In situ formed LiNi0.8Co0.15Al0.05O2@Li4SiO4 composite cathode material with high rate capability and long cycling stability for lithium-ion batteries. Nano Energy 53, 613–621. doi: 10.1016/j.nanoen.2018.09.014
Zhou, C. X., Wang, P. B., Zhang, B., Tang, L. B., Tong, H. J., and Zheng, J. C. (2019). Formation and effect of residual lithium compounds on Li-rich cathode material Li1.35[Ni0.35Mn0.65]O2. ACS Appl. Mater. Interfaces 11, 11518–11523. doi: 10.1021/acsami.9b01806
Keywords: Li-ion batteries, anode material, folic acid, Li4Ti5O12, N-doped carbon coating
Citation: Xiao H, Huang X, Ren Y, Ding X and Zhou S (2019) Fabrication of Li4Ti5O12@CN Composite With Enhanced Rate Properties. Front. Chem. 7:432. doi: 10.3389/fchem.2019.00432
Received: 20 March 2019; Accepted: 27 May 2019;
Published: 14 June 2019.
Edited by:
Junchao Zheng, Central South University, ChinaCopyright © 2019 Xiao, Huang, Ren, Ding and Zhou. This is an open-access article distributed under the terms of the Creative Commons Attribution License (CC BY). The use, distribution or reproduction in other forums is permitted, provided the original author(s) and the copyright owner(s) are credited and that the original publication in this journal is cited, in accordance with accepted academic practice. No use, distribution or reproduction is permitted which does not comply with these terms.
*Correspondence: Xiaobing Huang, aHhiMjIwMTcwQDEyNi5jb20=; Yurong Ren, cnlyY2hlbUAxNjMuY29t