- 1School of Physics and Information Technology, Shaanxi Normal University, Xi'an, China
- 2School of Electronic Information Technology, Macau University of Science and Technology, Macau, China
Gold nanorods (GNRs) has been investigated in the field of chemistry, optoelectronics, and medicine for their tenability, compatibility, electromagnetics, and excellent photonics properties. Especially, GNRs, used to generate ultrashort pulse, have been studied recently. However, multiple pulses evolution based on GNRs needs to be further explored. In this article, GNRs are synthesized by seed-mediated growth method, characterized systematically and been chosen as saturable absorber (SA) to apply in ultrafast photonics. The GNRs SA presents a saturable intensity of 266 MW/cm2, modulation depth of 0.6%, and non-saturable loss of 51%. Furthermore, a passively mode-locked erbium-doped fiber laser based on GNRs SA with femtosecond pulse is demonstrated. Thanks to the excellent properties of GNRs, by adjusting the cavity polarization direction with the proposed GNRs SA, soliton molecules operation with spectrum modulation period of 3.3 nm and pulse modulation interval of 2.238 ps is directly obtained. For the most important, 9th-order harmonic soliton molecules have been generated in the laser cavity for the first time. It is demonstrated that GNRs can be a novel type of non-linear optical (NLO) device and have potential applications in the field of ultrafast photonics.
Introduction
Many researchers have been attracted by noble metal nano-materials, such as gold, silver, due to the strong non-linear optical (NLO) effects which caused by their surface plasmon resonances (SPR) (Oh et al., 2011; Tsutsui et al., 2011; Huang et al., 2014; Komarov et al., 2015; Fan and Zhang, 2016; Yang et al., 2018). These effects enable noble metal nano-materials get many potential applications in synthesize NLO devices, such as optical detectors, optical sensors, and optical absorbers (Jain et al., 2007; Zijlstra et al., 2009). Among these noble metal nano-materials, gold nanorods (GNRs) have two SPR peaks, one is transverse SPR (TSPR) and the other is longitudinal SPR (LSPR). The LSPR is particularly sensitive to the aspect ratio of the GNRs and could be flexibly tuned through a broad spectral ranging from the visible to the near-infrared regime (Gou and Murphy, 2005; Ming et al., 2009; Kang et al., 2013; Fang et al., 2014; Lopez-Lozano et al., 2014; Burrows et al., 2016; He et al., 2016). This indicates that GNRs could be used in diverse wavebands when they are needed. In recent years, mode-locked and Q-switched fiber lasers using GNRs as SA have been demonstrated at different wavebands (1, 1.56, and 2 μm) (Kang et al., 2013, 2015; Wang X. et al., 2016; Chen et al., 2018).
Up to now, enormous experimental data and simulation results show that optical fiber lasers can produce many categories of pluses, such as conventional solitons (CSs), dissipative solitons, stretched pulse, self-similar pulse (Peng et al., 2013; Li et al., 2016; Wang Y. et al., 2016; Chai et al., 2018; Zhang et al., 2018; Wang et al., 2019). At the state of multi-soliton, harmonic mode locking (HML) would be formed when the solitons repel each other in a long range and uniformly fill in the whole laser cavity (Yang et al., 2007; Wang Z. et al., 2015; Fu et al., 2019). In addition, another kinds of multiple pulse called bound solitons (BSs) or soliton molecules would be formed as well, when the solitons repel each other in a short range and attract each other in a long range then transmit simultaneously (Komarov et al., 2008; Wang Y. et al., 2015).
Generally, fiber laser would work in one of the multi-soliton states. Nevertheless, coexistence between different kinds of multi-soliton states could also be generated in a passively mode-locked fiber lasers by precisely adjusting and optimizing the cavity parameters (Zhao et al., 2009; Luo et al., 2018; Zhang et al., 2019). Recently, researchers have reported that the two kinds of pulses, harmonic soliton molecules and rectangular noise-like pulses, can be coexisted in a fiber laser (Huang et al., 2016). In addition, different real saturable absorbers (SAs) can also be excellent composed for the generation of multiple pulses in the fiber laser cavity (Martinez et al., 2017; Lai et al., 2018). Other researchers reported that bound soliton and harmonic mode-locking soliton could be coexisted in an ultrafast fiber laser by MoS2 SA for a photonic device (Liu et al., 2018). GNRs as a promising SA need to be further investigated in aspect of generating special kinds of multiple pulses in the fiber laser cavities (Lu et al., 2018).
Although some studies have reported silica-encased GNRs can also be used as SA to produce ultrashort pulses, the preparation process of SA is more complex and only traditional solitons are obtained in fiber laser cavity (Wang X. et al., 2016). In this article, GNRs are successfully synthesized by seed-mediated growth method and characterized by scanning electron microscope (SEM), transmission electron microscope (TEM), and absorption spectra in the visible and near-infrared regions (Johnson et al., 2002; Perezjuste et al., 2005; Chow, 2017). Then, harmonic mode-locking of soliton molecules in EDF laser based on GNRs SA is studied. Depositing the GNRs dispersion on a taper fiber, whose waist diameter and length of 15 μm and 1.5 mm, respectively, to fabricate SA. Using the GNRs SA to obtain ultrashort pulse in infrared region. The GNRs SA has been studied with a modulation depth of 0.6% and non-saturable loss of 51%. By adjusting the laser's polarization direction, 740.6 fs soliton molecules are directly obtained from the Er-doped fiber laser based on GNRs SA. Increasing the pump power, 9th-order harmonic soliton molecules are generated in the laser cavity for the first time. It's much faster than many works before (Kang et al., 2013). The SA exhibits excellent stability, after a month, a steady pulse can be obtained as before. This work further confirms that the GNRs are promising high-performance SA with many potential applications in many fields such as optical fiber communications, optical logic signal processing, and even materials processing, etc.
Materials and Synthesis of GNRs
Materials
Chloroauric acid (HAuCl4), hexadecyltrimethyl ammonium bromide (CTAB), sodium borohydride (NaBH4), silver nitrate (AgNO3), hydrochloric acid (HCl), ascorbic acid (AA). All the glass wares are cleaned with deionized water by ultrasonic cleaning machines prior to the experiments.
Synthesis of GNRs
GNRs are synthesized by using the seed-mediated growth method reported previously. Briefly, HAuCl4 (marked solution B) is added to 8 mL CTAB (marked solution A) and mixed in a magnetic stirrer at 35°C, NaBH4 is added to the mixture with continuous stirring for 2 min. The color of the solution immediately changes from luminous yellow to dark brown. This change suggests that gold nanoparticles are successfully synthesized. The formed gold nanoparticles dispersion is sat in an incubator for 2 h to grow into seed solution and the seed solution are stable for a couple of weeks.
AgNO3 (marked solution c) and HAuCl4 (marked solution b) are added, respectively, to 20 mL CTAB (marked solution a), then added HCl to the solution (a) and mixed on a magnetic stirrer at 35°C (marked solution 1), AA is added to growth solution. Adding seed solution to growth solution and setting the mixture in an incubator for 12 h. Using deionized water to wash resulting solution repeatedly and centrifuging the resulting solution at 8,000 revolutions per min for 10 min once a time. Keeping the resulting solution in cold storage for further characterization. Figure 1A shows the preparation process of GNRs. Figure 1B shows the photograph of GNRs solution.
Characterization of Gold Nanorods (GNRs)
The SEM (the scale bar is 400 nm) and TEM (the scale bar is 40 nm) images of samples are shown in Figures 2a–c to characterize the obtained GNRs. Obviously, rod-shaped structure of samples are in the majority. By measuring we get the aspect ratio of GNRs about 2.5–8.5. It is interesting to note that seed particles also produce a significant amount of small rods and other secondary shapes, such as cone-shaped. In Figure 2d shows TEM image (HR-TEM) of GNRs. We can clearly observe a crystal lattice of GNRs in this image. The inset of Figure 2d shows the corresponding selective electron diffraction pattern. The GNRs prepared by seed growth method are single crystal structure, which is also indicated by the high resolution TEM of GNRs and the corresponding selective electron diffraction pattern.
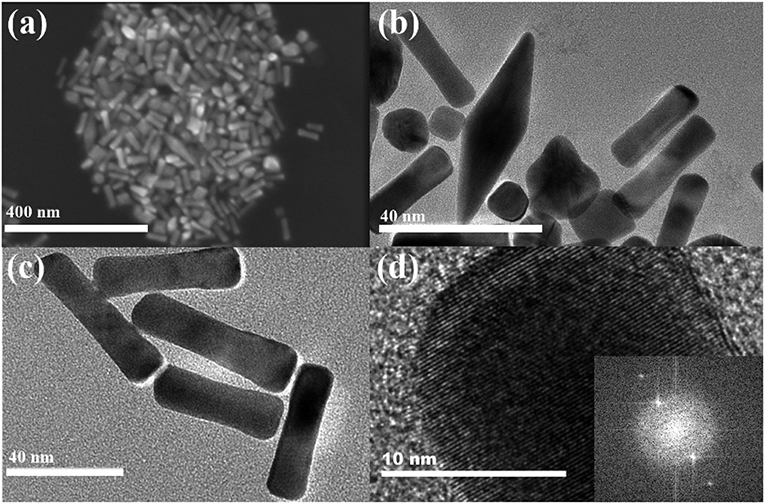
Figure 2. (a) SEM image of gold nanomaterials. (b,c) TEM images of GNRs with 40 nm scale. (d) HR-TEM image of GNR with 10 nm scale, the inset shows the power spectrum of the image.
Figure 3A shows the size distribution of the gold nanomaterials. The average diameters of these GNRs are 58.7 nm. The visible-near infrared (VIS-NIR) absorption is shown in Figure 3B. The samples have three higher absorption bands peaked at 0.5, 0.8, 1.5 μm, respectively. The absorption band peaked at 0.5 μm is caused by the TSPR with the diameter of GNRs is ~14 nm. The second band peaked at 0.8 μm is caused by the small GNRs and other secondary shapes. The band we are interested in 1.5 μm caused by the LSPR of long GNRs, which provides a usability of our GNRs sample in ultrafast laser as a SA.
The fabrication processes of GNRs SA are described in Figure 4. After mechanical exfoliation, the bare fiber of single mode fiber (SMF) is burned and stretched, only in this way can we prepare a segment of taper fiber with waist diameter of 15 μm and length of 1.5 mm, this process is shown in Figure 4a. Figure 4b exhibits a model for preparing the GNRs SA by means of optical induced deposition. Injecting laser in the end of microfiber when dropping GNRs dispersion onto the surface of taper fiber. Figure 4c depicted a taper fiber reality image observed under a microscope and the black part attached to the surface of taper fiber is GNRs.
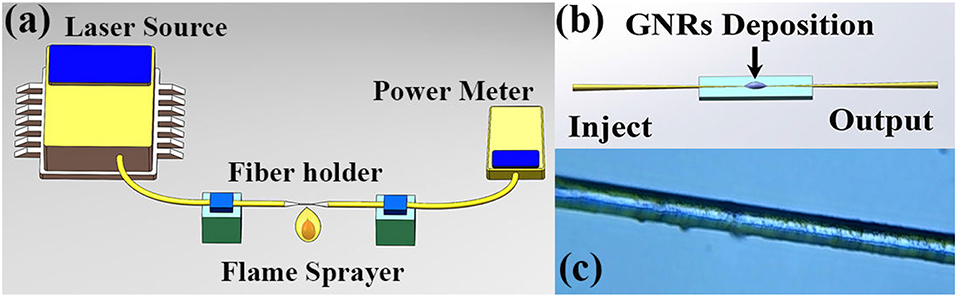
Figure 4. (a) Experimental setup for fabricating a taper fiber. (b) Schematic diagram for deposition of GNRs material on taper fiber by injecting laser. (c) Image of the microscopical taper fiber-based GNRs SA.
In order to further investigate the characteristics of GNRs, the non-linear absorption is measured by using balanced twin detector measurement technology. Figure 5a describes the measurement method of saturable absorption property about the GNRs. In this experiment, the pump source, a standard femtosecond pulse laser source whose center wavelength of 1563.3 nm, repetition rate of 21.4 MHz and pulse duration of 584 fs is divided into two parts by a 50/50 optical coupler after passing through attenuator which can be adjust the output power of standard laser source. One accesses power meter 1 directly, the other injects the GNRs SA before enters the power meter 2. The optical non-linear transmittance result is exhibited in Figure 5b. As shown here, the saturable intensity is 266 MW/cm2, the modulation depth is ~0.6%, and the non-saturable loss of 51%. Based on these experimental results, we apply GNRs as a SA in fiber laser for implementing mode-locking.
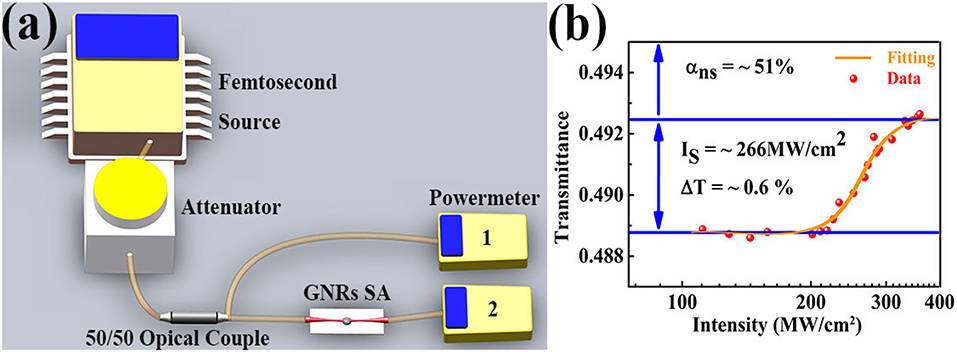
Figure 5. (a) Measurement schematic of non-linear saturable absorption. (b) The saturable absorption curvey of gold nanomaterials SA.
Laser Performance and Discussions
To check the laser performance of GNRs SA, it is incorporated into a laser cavity to generate ultrashort pulse. The schematic of the passively mode-locked EDF ring laser is shown in Figure 6. A 0.5 m-long EDF offering a laser gain is pumped by 976 nm laser diode (LD) connecting a 980/1,550 nm wavelength division multiplexer (WDM). The polarization controller (PC) are utilized to control the light polarization state for achieving mode-locking and optimizing the laser operation. A polarization-independent isolator (PI-ISO) is used to guarantee the unidirectional operation. The GNRs SA plays an essential role in this fiber laser cavity. A 20/80 fiber optical coupler (OC), the 20% is exported to detect intracavity laser performance and the other 80% turn back laser cavity to oscillate, is located in the end of cavity. The total cavity length is around ~17 m corresponding to a fundamental repetition rate of 11.3 MHz. The spectrum and pulse train are monitored by an optical spectrum analyzer (Anritsu MS9710C), a real-time oscilloscope with resolution of 1 GHz (Rigon DS6104) and a photodetector with resolution of 2 GHz (Thorlabs DET01CFC). The radio frequency (RF) spectrum of the mode-locked operation is recorded by a radio frequency spectrum analyzer (Rohde & Schwarz FSC6). Moreover, the pulse duration and modulation interval are measured by an autocorrelator (Femtochrome FR-103XL).
Then simply increasing the pump power to 153 mW, the self-started soliton molecule mode-locking operation occurs. For further exploring the mode-locking operation, the pump power is increased to 314 mW. Figure 7A shows the typical soliton molecule spectrum obtains at the pump power of 203 mW. Here, spectrum modulation period of soliton molecule is 3.3 nm. Figure 7B presents the corresponding mode-locked pulse-train and we realize the pulse period is 88.4 ns. To verify the laser stability, we measure the radio frequency (RF) spectrum of the mode locking operation with a resolution bandwidth of 30 kHz and video bandwidth of 100 Hz. As presented in Figure 7C, the fundamental frequency peak locates at 11.3 MHz determined by the cavity length of ~17 m, corresponding to the fundamental cavity repetition rate. The signal-to-noise ratio (SNR) is ~47.2 dB, indicating the high stability of mode-locking operation. Moreover, the inset of Figure 7C shows RF spectrum measured under the 22.6 MHz span. Then, by using AC, the soliton modulation period and duration of the mode-locked pulse are identified. As described in Figure 7D, the soliton molecule delivers the pulse-train with 740.6 fs duration and 2.238 ps soliton pulse modulation interval if the fit of the sech2 pulse shape is assumed. There is a formula to describe the relationship between spectrum modulation period (Δλ) and pulse modulation interval (ΔT) of soliton molecule:
Here, λ0 and c mean center wavelength of mode-locked laser operation and speed of light. Their values are 1,560 nm and 3 × 108 m/s, respectively. Through theoretical calculation, our experimental results are consistent with the theoretical predictions.
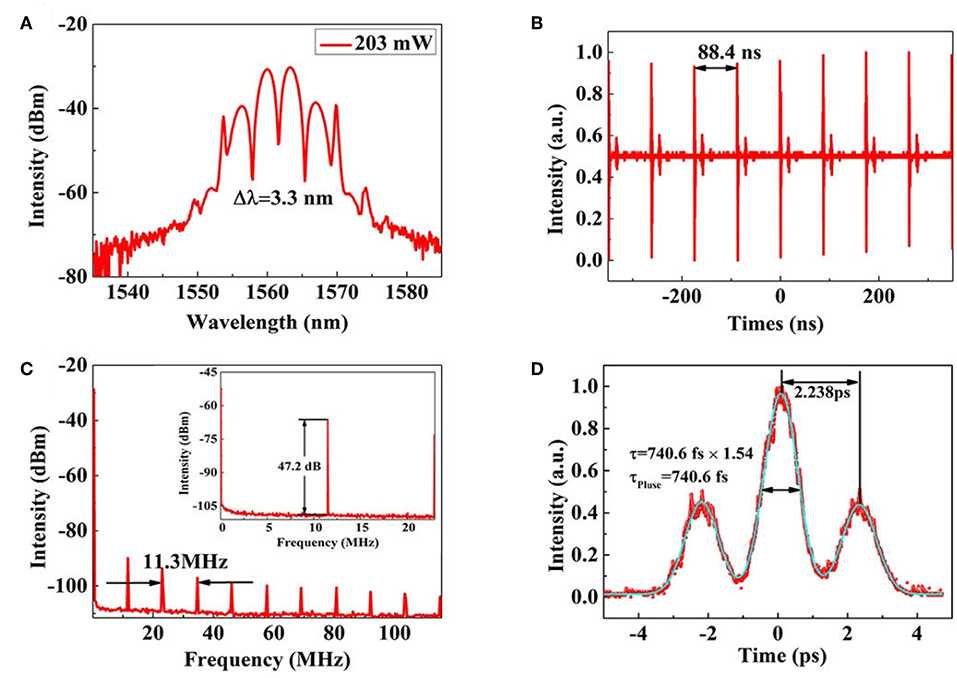
Figure 7. (A) The typical soliton molecule spectrum obtained at the pump power of 203 mW. (B) The corresponding soliton molecule pulse-sequence. (C) RF spectrum around the fundamental repetition rate; (D) autocorrelation trace of partially coherent pulse generation.
After increasing the pump power gradually to the maximum of laser diode, fiber laser is still working in the mode-locked state. Moreover, the soliton molecule could maintain stable operation well at the maximum pump power of 350 mW, which means the optical damage threshold of GNRs SA is considerable high. Figures 8A,B show the spectrum and sequence evolution of soliton molecule with pump power from 153 to 314 mW. Figure 8C depicts the variation of the soliton molecule operation output power when we increasing the pump power. More importantly, the 9th-order harmonics soliton molecule is obtained during the process of increasing pump power. Optical spectrum, pulse sequence and autocorrelation trace of 9th order harmonics soliton molecule under 314 mW of pump power are exhibited in Figures 8D–F, respectively. We can discover that 9th soliton molecule has the 3.2 nm spectrum modulation period, 101.7 MHz fundamental frequency and 0.9 ps pulse duration with 2.7 ps soliton pulse modulation interval. In the experiment, we also check the significance of GNRs SA in fiber laser. GNRs SA is removed from the laser cavity. As a result, there is no soliton molecule operation even if the PC is rotated in a large range. The results demonstrate that the ultrafast properties of GNRs are accountable for the generation of the fiber laser.
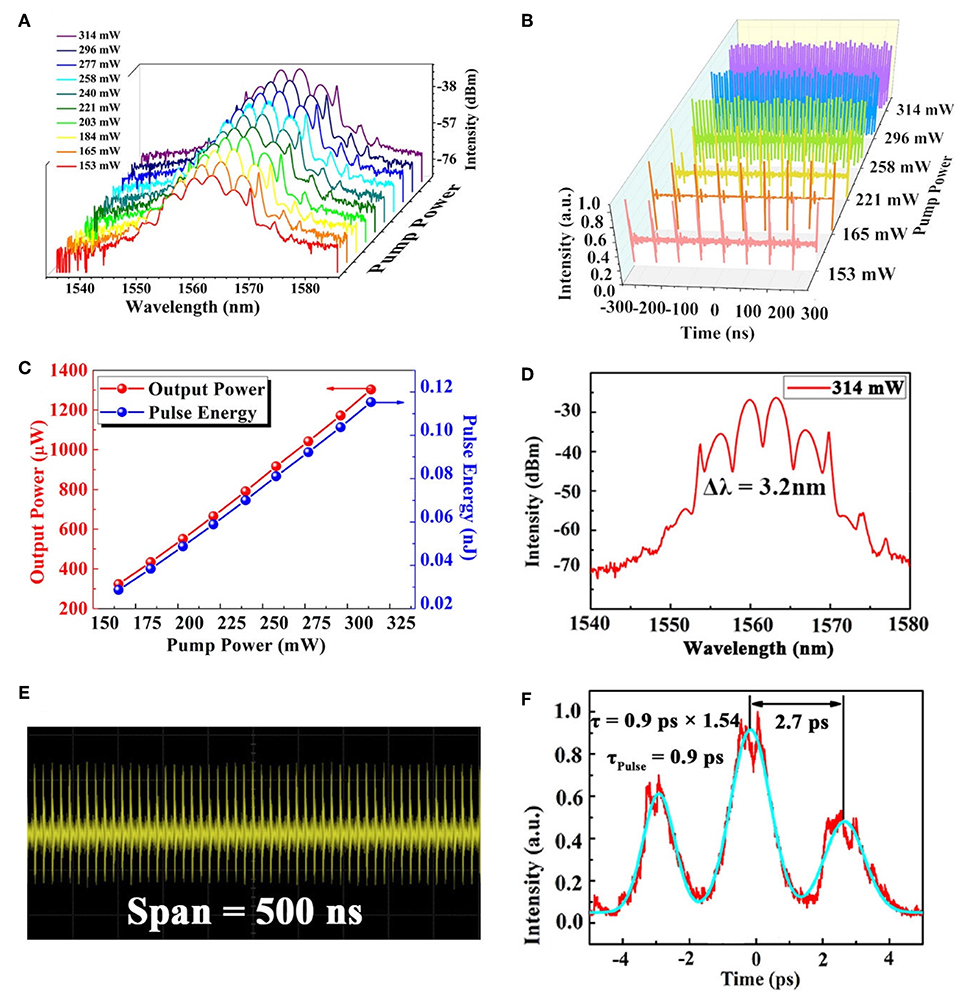
Figure 8. (A) Evolution of optical spectrum with the pump power from 153 to 314 mW. (B) Pulse sequence evolution with pump power. (C) Output power of soliton molecule as a function of pump power. (D) Optical spectrum with 314 mW of pump power. (E) Pulse sequence of higher order harmonic soliton molecule under the pump power of 314 mW. (F) Autocorrelation trace of higher order harmonic soliton molecule under the pump power of 314 mW.
As we know, not only the soliton molecules and harmonic mode-locking solution but many other kinds of pluses could also be generated in a fiber laser, such as soliton rain and noise-like pluses. Nevertheless, in our experiments, only two multi-soliton states are observed. Thanks to the high optical damage threshold of GNRs we would do more research on composite mode-locked operations based on GNRs SA and investigate the coexistence of multi-soliton dynamics.
Conclusion
In conclusion, GNRs are prepared by seed-mediated growth method and characterized by the technology of SEM, TEM, VIS-NIR absorption which noticed the micro-structure and the absorption of GNRs. By measuring the NLO response of GNRs, the obvious saturable absorption effect is identified which shows a modulation depth of 0.6%. And then, GNRs are used as a high-performance SA in a fiber laser for generating soliton molecule mode-locked pulse. By the GNRs SA, the 740.6 fs stable soliton molecule mode-locked pulse easily produced from the fiber laser. And 9th-order harmonics mode-locked soliton molecules obtained in the laser cavity for the first time. It turns out that the GNRs SA could be a really wonderful NLO material for preparation of saturable absorption device for ultrafast lasers. And has the great potential in many applications.
Data Availability Statement
All datasets generated for this study are included in the article/supplementary files.
Author Contributions
YS do experiment and write paper. XL providing guiding ideology and experimental equipment. PG process data and draw experimental images. GL provide materials needed for the experiment. PW participate in the preparation of gold nanorods. GS purchase reagents for the experiment. JL modify the article.
Funding
This research was supported by the National Natural Science Foundation of China (61605106); funded projects for the Academic Leader and Academic Backbones, Shaanxi Normal University (18QNGG006); starting Grants of Shaanxi Normal University (Grant Nos. 1112010209, 1110010717); Fundamental Research Funds For the Central Universities (GK201802006); Open Research Fund of State Key Laboratory of Pulsed Power Laser Technology, Electronic Engineering Institute (No. SKL2017KF02); Open Fund of State Key Laboratory of Information Photonics and Optical Communications (Beijing University of Posts and Telecommunications), P. R. China (IPOC2017B012); Open Research Fund of State Key Laboratory of Transient Optics and Photonics, Chinese Academy of Sciences (Nos. SKLST201401, SKLST201809). Funded by the Science and Technology Development Fund, Macau SAR (File No. 002/2016/AFJ).
Conflict of Interest
The authors declare that the research was conducted in the absence of any commercial or financial relationships that could be construed as a potential conflict of interest.
References
Burrows, N. D., Lin, W., Hinman, J. G., Dennison, J. M., Vartanian, A. M., Abadeer, N. S., et al. (2016). Surface chemistry of gold nanorods. Langmuir 32, 9905–9921. doi: 10.1021/acs.langmuir.6b02706
Chai, T., Li, X. H., Feng, T. C., Guo, P. L., Song, Y., Chen, Y., et al. (2018). Few-layer bismuthene for ultrashort pulse generation in a dissipative system based on an evanescent field. Nanoscale 10, 17617–17622. doi: 10.1039/C8NR03068E
Chen, X., Li, P., Dun, Y., Song, T., and Ma, B. (2018). Nanosecond-pulsed Q-switched Nd: YAG laser at 1064 nm with a gold nanotriangle saturable absorber. Appl. Phys. B 124:92. doi: 10.1007/s00340-018-6952-7
Chow, K. K. (2017). Low pump threshold CVD graphene based passively harmonic mode-locked fibre laser. Electron. Lett. 53, 330–331. doi: 10.1049/el.2016.4325
Fan, Z. X., and Zhang, H. (2016). Crystal phase-controlled synthesis, properties and applications of noble metal nanomaterials. Chem. Soc. Rev. 45, 63–82. doi: 10.1039/C5CS00467E
Fang, C., Jia, H., Chang, S., Ruan, Q., Wang, P., Chen, T., et al. (2014). (Gold core)/ (titania shell) nanostructures for plasmon-enhanced photon harvesting and generation of reactive oxygen species. Energy Environ. Sci. 7, 3431–3438. doi: 10.1039/C4EE01787K
Fu, B., Li, J., Cao, Z., and Popa, D. (2019). Bound states of solitons in a harmonic graphene-mode-locked fiber laser. Photon. Res. 7, 116–120. doi: 10.1364/PRJ.7.000116
Gou, L., and Murphy, C. J. (2005). Fine-tuning the shape of gold nanorods. Chem. Mater. 17, 3668–3672. doi: 10.1021/cm050525w
He, J., Zheng, W., Ligmajer, F., Chan, C.-F., Bao, Z., Wong, K.-L., et al. (2016). Plasmonic enhancement and polarization dependence of nonlinear upconversion emissions from single gold nanorod@SiO2@CaF2:Yb3+,Er3+ hybrid core–shell–satellite nanostructures. Light 6:e16217. doi: 10.1038/lsa.2016.217
Huang, Y. F., Zhang, M., Zhao, L. B., Feng, J. M., Wu, D. Y., Ren, B., et al. (2014). Activation of oxygen on gold and silver nanoparticles assisted by surface plasmon resonances. Angew. Chem. 126, 2385–2389. doi: 10.1002/ange.201310097
Huang, Y. Q., Hu, Z. A., Cui, H., Luo, Z. C., Luo, A. P., and Xu, W. C. (2016). Coexistence of harmonic soliton molecules and rectangular noise-like pulses in a figure-eight fiber laser. Opt. Lett. 41, 4056–4059. doi: 10.1364/OL.41.004056
Jain, P. K., Huang, X., El-Sayed, I. H., and El-Sayed, M. A. (2007). Review of some interesting surface plasmon resonance-enhanced properties of noble metal nanoparticles and their applications to biosystems. Plasmonics 2, 107–118. doi: 10.1007/s11468-007-9031-1
Johnson, C. J., Dujardin, E., Davis, S. A., Murphy, C. J., and Mann, S. (2002). Growth and form of gold nanorods prepared by seed-mediated, surfactant-directed synthesis. J. Mater. Chem. 12, 1765–1770. doi: 10.1039/b200953f
Kang, Z., Gao, X., Zhang, L., Feng, Y., Qin, G., and Qin, W. (2015). Passively mode-locked fiber lasers at 1039 and 1560 nm based on a common gold nanorod saturable absorber. Opt. Mater. Express 5, 794–801. doi: 10.1364/OME.5.000794
Kang, Z., Xu, Y., Zhang, L., Jia, Z., Liu, L., Zhao, D., et al. (2013). Passively mode-locking induced by gold nanorods in erbium-doped fiber lasers. Appl. Phys. Lett. 103:041105. doi: 10.1063/1.4816516
Komarov, A., Haboucha, A., and Sanchez, F. (2008). Ultrahigh-repetition-rate bound-soliton harmonic passive mode-locked fiber lasers. Opt. Lett. 33, 2254–2256. doi: 10.1364/OL.33.002254
Komarov, A., Komarov, K., and Sanchez, F. (2015). Harmonic passive mode locking of bound-soliton structures in fiber lasers. Opt. Commun. 354, 158–162. doi: 10.1016/j.optcom.2015.05.070
Lai, X., Li, J., Luo, H., Zhu, C., Hai, Y., Shi, Y., et al. (2018). High power passively Q-switched Er3+-doped ZBLAN fiber laser at 2.8 μm based on a semiconductor saturable absorber mirror. Laser Phys. Lett. 15:085109. doi: 10.1088/1612-202X/aac549
Li, X., Wu, K., Sun, Z., Meng, B., Wang, Y., Yu, X., et al. (2016). Single-wall carbon nanotubes and graphene oxide-based saturable absorbers for low phase noise mode-locked fiber lasers. Sci. Rep. 6:25266. doi: 10.1038/srep25266
Liu, M., Luo, A. P., Xu, W. C., and Luo, Z. C. (2018). Coexistence of bound soliton and harmonic mode-locking soliton in an ultrafast fiber laser based on MoS2-deposited microfiber photonic device. Chin. Opt. Lett. 16:020008. doi: 10.3788/COL201816.020008
Lopez-Lozano, X., Barron, H., Mottet, C., and Weissker, H. C. (2014). Aspect-ratio- and size-dependent emergence of the surface-plasmon resonance in gold nanorods–an ab initio TDDFT study. Phys. Chem. Chem. Phys. 16, 1820–1823. doi: 10.1039/C3CP53702A
Lu, S., Du, L., Kang, Z., Li, J., Huang, B., Jiang, G., et al. (2018). Stable dissipative soliton generation from Yb-doped fiber laser modulated via evanescent field interaction with gold nanorods. IEEE Photon. J. 10, 1–8. doi: 10.1109/JPHOT.2018.2872405
Luo, Y., Xiang, Y., Liu, B., Qin, Y., Sun, Q., Tang, X., et al. (2018). Dispersionmanaged soliton molecules in a near zero-dispersion fiber laser. IEEE Photon. J. 10, 1–11. doi: 10.1109/JPHOT.2018.2874949
Martinez, A., Al Araimi, M., Dmitriev, A., Lutsyk, P., Li, S., Mou, C., et al. (2017). Low-loss saturable absorbers based on tapered fibers embedded in carbon nanotube/polymer composites. APL Photon. 2:126103. doi: 10.1063/1.4996918
Ming, T., Zhao, L., Yang, Z., Chen, H., Sun, L., Wang, J., et al. (2009). Strong polarization dependence of plasmon-enhanced fluorescence on single gold nanorods. Nano Lett. 9, 3896–3903. doi: 10.1021/nl902095q
Oh, M. K., Baik, H. J., Kim, S. K., and Park, S. (2011). Multiple surface plasmon resonances in silver and copper nanorods. J. Mater. Chem. 21, 19069–19073. doi: 10.1039/c1jm13613e
Peng, J., Zhan, L., Luo, S., and Shen, Q. S. (2013). Generation of soliton molecules in a normal-dispersion fiber laser. IEEE Photon. Technol. Lett. 25, 948–951. doi: 10.1109/LPT.2013.2257720
Perezjuste, J., Pastorizasantos, I., Lizmarzan, L., and Mulvaney, P. (2005). Gold nanorods: synthesis, characterization and applications. Coord. Chem. Rev. 249, 1870–1901. doi: 10.1016/j.ccr.2005.01.030
Tsutsui, Y., Hayakawa, T., Kawamura, G., and Nogami, M. (2011). Tuned longitudinal surface plasmon resonance and third-order nonlinear optical properties of gold nanorods. Nanotechnology 22:275203. doi: 10.1088/0957-4484/22/27/275203
Wang, C., Wang, L., Li, X. H., Luo, W., Feng, T. C., Zhang, Y., et al. (2019). Few-layer bismuthene for femtosecond soliton molecules generation in Er-doped fiber laser. Nanotechnology 30:025204. doi: 10.1088/1361-6528/aae8c1
Wang, X., Luo, Z., Liu, M., Tang, R., Luo, A., and Xu, W. (2016). Wavelength-switchable femtosecond pulse fiber laser mode-locked by silica-encased gold nanorods. Laser Phys. Lett. 13:045101. doi: 10.1088/1612-2011/13/4/045101
Wang, Y., Alam, S. U., Obraztsova, E. D., Pozharov, A. S., Set, S. Y., and Yamashita, S. (2016). Generation of stretched pulses and dissipative solitons at 2 mum from an all-fiber mode-locked laser using carbon nanotube saturable absorbers. Opt. Lett. 41, 3864–3867. doi: 10.1364/OL.41.003864
Wang, Y., Mao, D., Gan, X., Han, L., Ma, C., and Xi, T. (2015). Harmonic mode locking of bound-state solitons fiber laser based on MoS (2) saturable absorber. Opt. Express 23, 205–210. doi: 10.1364/OE.23.000205
Wang, Z., Zhan, L., Majeed, A., and Zou, Z. (2015). Harmonic mode locking of bound solitons. Opt. Lett. 40, 1065–1068. doi: 10.1364/OL.40.001065
Yang, L. Z., Wang, Y. C., Cheng, G. F., Wang, Y. S., and Zhao, W. (2007). Harmonic mode-locked ytterbium-doped fibre ring laser. Chin. Phys. Lett. 24, 944–946. doi: 10.1088/0256-307X/24/4/026
Yang, X., Sun, Z., Low, T., Hu, H., Guo, X., Garcia De Abajo, F. J., et al. (2018). Nanomaterial-based plasmon-enhanced infrared spectroscopy. Adv. Mater. 30:e1704896. doi: 10.1002/adma.201704896
Zhang, M., Wu, Q., Zhang, F., Chen, L., Jin, X., Hu, Y., et al. (2019). 2D black phosphorus saturable absorbers for ultrafast photonics. Adv. Opt. Mater. 7:1800224. doi: 10.1002/adom.201800224
Zhang, Y., Li, X. H., Qyyum, A., Chai, T., Guo, P. L., Feng, J. J., et al. (2018). PbS nanoparticles for ultrashort pulse generation in optical communication region. Part. Part. Syst. Charact. 35:1800341. doi: 10.1002/ppsc.201800341
Zhao, L. M., Tang, D. Y., Cheng, T. H., Lu, C., Tam, H. Y., Fu, X. Q., et al. (2009). Passive harmonic mode locking of soliton bunches in a fiber ring laser. Opt. Quant. Electron. 40, 1053–1064. doi: 10.1007/s11082-009-9301-6
Keywords: gold nanorods, non-linear optical properties, ultrafast photonics, mode-locked fiber laser, harmonic soliton molecules
Citation: Shu Y, Guo P, Li X, Li G, Wang P, Shen G and Li J (2019) Gold Nanorods as Saturable Absorber for Harmonic Soliton Molecules Generation. Front. Chem. 7:715. doi: 10.3389/fchem.2019.00715
Received: 13 May 2019; Accepted: 10 October 2019;
Published: 24 October 2019.
Edited by:
Tianyou Zhai, Huazhong University of Science and Technology, ChinaReviewed by:
Ran Long, University of Science and Technology of China, ChinaXu Peng, Hubei University, China
Tengfei Zhou, University of Wollongong, Australia
Copyright © 2019 Shu, Guo, Li, Li, Wang, Shen and Li. This is an open-access article distributed under the terms of the Creative Commons Attribution License (CC BY). The use, distribution or reproduction in other forums is permitted, provided the original author(s) and the copyright owner(s) are credited and that the original publication in this journal is cited, in accordance with accepted academic practice. No use, distribution or reproduction is permitted which does not comply with these terms.
*Correspondence: Xiaohui Li, lixiaohui0523@163.com