- 1Guangxi Colleges and Universities Key Laboratory of Novel Energy Materials and Related Technology, Guangxi Novel Battery Materials Research Center of Engineering Technology, School of Physical Science and Technology, Guangxi University, Nanning, China
- 2State Key Laboratory of Silicon Materials, School of Materials Science and Engineering, Zhejiang University, Hangzhou, China
- 3State Key Laboratory of Advanced Power Transmission Technology, Global Energy Interconnection Research Institute Co. Ltd., Beijing, China
- 4Department of Materials Science and Engineering, Baise College, Baise, China
Aluminum hydride (AlH3) is a promising candidate for hydrogen storage due to its high hydrogen density of 10 wt%. Several polymorphs of AlH3 (e.g., α, β, and γ) have been successfully synthesized by wet chemical reaction of LiAlH4 and AlCl3 in ether solution followed by desolvation. However, the synthesis process of α'-AlH3 from wet chemicals still remains unclear. In the present work, α'-AlH3 was synthesized first by the formation of the etherate AlH3 through a reaction of LiAlH4 and AlCl3 in ether solution. Then, the etherate AlH3 was heated at 60°C under an ether gas atmosphere and in the presence of excess LiAlH4 to remove the ether ligand. Finally, α'-AlH3 was obtained by ether washing to remove the excess LiAlH4. It is suggested that the desolvation of the etherate AlH3 under an ether gas atmosphere is essential for the formation of α'-AlH3 from the etherate AlH3. The as-synthesized α'-AlH3 takes the form of rod-like particles and can release 7.7 wt% hydrogen in the temperature range 120–200°C.
Introduction
Aluminum hydride (AlH3) is a kinetically stable metal hydride under ambient conditions. It theoretically has a high hydrogen capacity of 10 wt% and can release hydrogen at temperatures below 200°C (Sandrock et al., 2005; Graetz, 2009; Graetz et al., 2011). Therefore, it has long been considered as a promising hydrogen storage media for on-board applications. There are seven known polymorphs of AlH3: α-, α'-, β-, γ-, δ-, ε-, and ζ-AlH3 (Brower et al., 1976). These AlH3 polymorphs have different structures and thermal stabilities and thus have slightly different decomposition properties and mechanisms. α-AlH3 is the most stable polymorph and will undergo direct decomposition to form Al and H2 with an increase in temperature (Sandrock et al., 2005; Graetz and Reilly, 2006; Orimo et al., 2006). The other polymorphs, such as β-AlH3 and γ-AlH3, will first transform into the more stable α-AlH3 and then decompose to form Al and H2 (Graetz and Reilly, 2006). Direct decompositions of γ-AlH3 and α'-AlH3 to form Al and H2 without the first phase transition have also been reported in the literature (Sartori et al., 2008; Liu et al., 2013; Gao et al., 2017).
The synthesis of AlH3 dates back to 1942 when Stecher and Wiberg (1942) prepared the AlH3 amine complex in an impure form. The synthesis method of AlH3 was then modified and improved by other researchers (Finholt et al., 1947; Chizinsky et al., 1955; Ashby, 1964). In 1976, Brower et al. (1976) summarized their findings on the synthesis of non-solvated AlH3 by the wet chemical method. They used LiAlH4 and AlCl3 as the starting materials and ether as the solvent. Generally, LiAlH4 was reacted with AlCl3 in the ether solution to form AlH3·nEt2O and LiCl [reaction (1)]. The precipitate LiCl was then removed by filtration, and the AlH3·nEt2O precipitated slowly during storage. The obtained solid, AlH3·nEt2O, was heated under certain conditions to remove the ether ligand [reaction (2)], which was called the desolvation process. Depending on the desolvation conditions used, AlH3 would crystalize in different structures.
Non-solvated α-, β-, and γ-AlH3 have been successfully synthesized by the wet chemical method (Brinks et al., 2006, 2007a,b; Graetz and Reilly, 2006; Orimo et al., 2006; Liu et al., 2013; Gao et al., 2017). These are the polymorphs of AlH3 that have been intensively studied. However, the intrinsic decomposition properties of α'-AlH3 are still unclear due to the fact that pure and non-solvated α'-AlH3 is hard to synthesyze. As far as we know, the synthesis of pure and non-solvated α'-AlH3 by the wet chemical method has not yet been reported in the open literature. Although Brower et al. (1976) suggested that α'-AlH3 can be synthesized by the slow desolvation of AlH3·nEt2O, no characterized product of α'-AlH3 was disclosed.
In 2006, Brinks et al. (2006) utilized the cryomilling method to prepare α'-AlD3 from a mixture of 3LiAlD4 + AlCl3. It was shown that cryomilling at a temperature as low as 77 K resulted in the formation of only AlD3 and LiCl. The AlD3 obtained was a mixture of 2/3α-AlD3 + 1/3α'-AlD3 (Brinks et al., 2006). Another work by Sartori et al. (2009) showed that the yield of AlD3 was increased by using 3NaAlH4 + AlCl3 or 3LiAlD4 +AlBr3 as the raw materials. In addition, the relative amount of α'-AlD3 over α-AlD3 was increased from 0.63–0.67 to 1.05 by the addition of FeF3 into the 3LiAlD4 + AlCl3 mixture. Although α'-AlH3 can be obtained by the cryomilling method, the unwanted product of LiCl salt is difficult to remove. Moreover, the α'-AlH3 prepared by this method is usually accompanied by α-AlH3 polymorphs.
In the present work, the synthesis of non-solvated and pure α'-AlH3 by the wet chemical method is studied. The decomposition properties of α'-AlH3 will also be preliminarily revealed.
Experimental Details
Synthesis of α'-AlH3
The synthesis process of α'-AlH3 employed here is similar to that reported by Brower et al. (1976). However, some conditions needed to be modified. In detail, 1 M ether (Sinopharm Group, Analytical purity) solution of LiAlH4 (TCI, 98% purity) was mixed with 1 M ether solution of AlCl3 (Aldrich, 99.99% purity) at a molar ratio of 4:1. It should be noted that LiAlH4 was used in excess. Brower et al. (1976) found that the etherate AlH3 will decompose to Al if heated under a vacuum, but, in the presence of excess LiAlH4, the ether can be removed without decomposition. LiAlH4 will react with AlCl3 upon mixing in the ether solution to form the etherate AlH3 (AlH3·nEt2O) and LiCl precipitate based on reaction (3). The mixed solution was stirred for 2 min to ensure that the reaction was completed. Immediately after that, the LiCl precipitate was removed by filtration and the liquid ether was removed by slowly evacuation at room temperature. The dry and white residue obtained, which was a mixture of 4AlH3·nEt2O + LiAlH4, was ground to powder with a mortar and pestle for heating treatment. Powder samples were then heated at certain temperatures for various durations under certain atmospheres to remove the ether ligand [reaction (4)]. The conditions used for heat treatment significantly impact the desolvation products of the 4AlH3·nEt2O + LiAlH4 mixture, as will be shown in the next section. Finally, the desolvated 4AlH3·nEt2O + LiAlH4 mixture was ether-washed to remove the excess LiAlH4, and AlH3 was obtained.
Characterizations of α'-AlH3
Powder X-ray diffraction (XRD, PANalytical X'Pert Pro, Cu Kα, 40 kV, 40 mA) was used to study the phase structures of the samples. The samples for XRD studies were sealed with an amorphous membrane to protect them from oxidation during the sample transformations and measurements. Scanning electronic microscopy (SEM, FEI SIRION-100, 25 kV) was used to study the morphology of the as-synthesized α'-AlH3. The hydrogen desorption property of the as-synthesized α'-AlH3 was studied by using a home-made Sieverts-type hydrogen sorption measurement apparatus based on the volumetric method. Experimentally, the samples were sealed in a reactor and were heated under an initial vacuum gradually from room temperature to the set temperature with a heating rate of 2°C/min.
Results and Discussion
On the synthesis of AlH3 by the wet chemical reaction in the ether solution, the conditions (desolvation aid, temperature, time, atmosphere) used in the desolvation stage [reaction (4)] significantly affect the desolvation product of AlH3·nEt2O (Brower et al., 1976). α-AlH3 can be obtained by heating the AlH3·nEt2O at 60–80°C under a vacuum in the presence of excess LiAlH4 and LiBH4, while γ-AlH3 is formed when the AlH3·nEt2O is heated at 60–70°C under a vacuum in the presence of only excess LiAlH4 (Brower et al., 1976). It should be noted that the AlH3·nEt2O should be desolvated in the presence of excess LiAlH4 (and LiBH4), with which AlH3·nEt2O can easily transform to AlH3 without decomposition (Brower et al., 1976).
In the present work, the AlH3·nEt2O was heated under a gaseous ether atmosphere, which is the key factor for producing α'-AlH3. The ether atmosphere was generated by injecting a drop of liquid ether into the sample reactor. The liquid ether can easily transform to gaseous ether during heating to 60–80°C since the boiling point of ether is as low as 34.6°C. In this way, the AlH3·nEt2O can undergo desolvation under a gaseous ether atmosphere. Figure 1 shows the XRD patterns of the desolvation products of AlH3·nEt2O heated at 60°C for various durations under an atmosphere of gaseous ether. It can be seen that traces of α'-AlH3 formed after desolvation for 2 h. With an increase in the desolvation duration, more and more α'-AlH3 formed. The AlH3·nEt2O can totally transformed to α'-AlH3 after desolvation for 6 h.
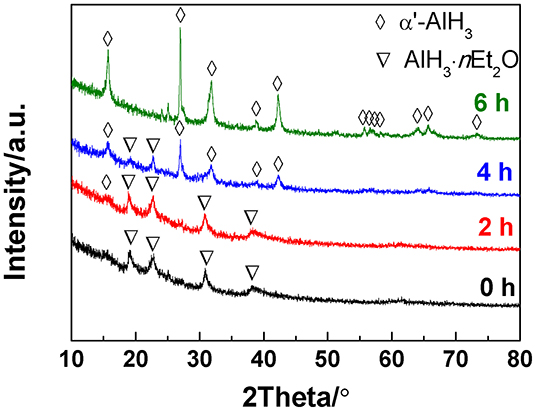
Figure 1. XRD patterns of the desolvation products of AlH3·nEt2O heated at 60°C for various durations.
When the desolvation of the AlH3·nEt2O was conducted at 75°C, the transformation to α'-AlH3 proceeded more rapidly. Figure 2 shows the XRD patterns of the desolvation products of AlH3·nEt2O after heating at 75°C for various durations under an atmosphere of gaseous ether. It was observed that some traces of α'-AlH3 formed after desolvation for only 1 h. After 4 h of desolvation, the AlH3·nEt2O had completely transformed to AlH3, which was a mixture of α'-AlH3 and α-AlH3. This means that some of the α'-AlH3 may have transformed into more stable α-AlH3 during heat treatment at 75°C. Therefore, a lower desolvation temperature (e.g., 60°C) is preferred in order to produce pure α'-AlH3.
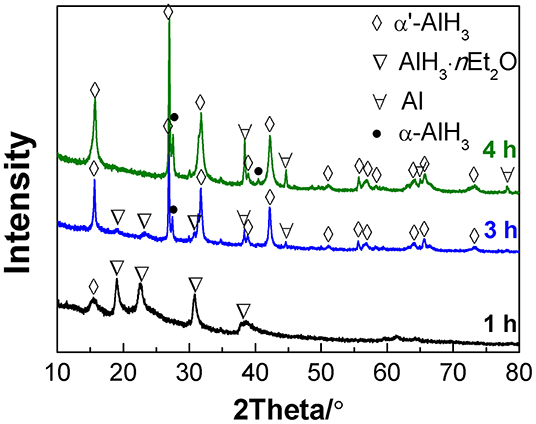
Figure 2. XRD patterns of the desolvation products of AlH3·nEt2O heated at 75°C for various durations.
The morphology of the as-synthesized α'-AlH3 was studied by SEM techniques, as shown in Figure 3. It can be seen that the as-synthesized α'-AlH3 takes the form of rod-like particles with lengths of about 1 μm and widths of about 100 nm. This unique particle morphology may benefit the hydrogen desorption process of α'-AlH3 because it possesses more surface area than other morphologies such as spheres of similar dimensions.
The hydrogen desorption curve of the as-synthesized α'-AlH3 with a heating rate of 2°C/min is shown in Figure 4. As can be seen that it starts to release hydrogen at 120°C and reaches a hydrogen desorption capacity of 7.7 wt% when the temperature is increased to 200°C. After hydrogen desorption, Al is formed. It should be noted that the practical capacity is somewhat lower than the theoretical value, which may be due to the impurity of the sample. This decomposition temperature range is similar to that of α-AlH3 and γ-AlH3 (Graetz and Reilly, 2006; Liu et al., 2013).
Conclusion
Non-solvated α'-AlH3 was successfully synthesized by the wet chemical reaction of LiAlH4 and AlCl3 in ether solution followed by desolvation. The conditions used in the desolvation stage are the essential factors in producing α'-AlH3. Desolvation under a gaseous ether atmosphere is the key to the transformation of AlH3·nEt2O into non-solvated α'-AlH3. The as-synthesized α'-AlH3 particles are rod-like and can release 7.7 wt% hydrogen in the temperature range 120–200°C. The purity of the α'-AlH3 needs to be improved in future work.
Data Availability Statement
All datasets generated for this study are included in the article/supplementary material.
Author Contributions
All authors listed have made a substantial, direct and intellectual contribution to the work, and approved it for publication.
Funding
This work was financially supported by the National Natural Science Foundation of China (Nos. 51771171 and 51971199), the Education Department of Guangxi Zhuang Autonomous Region (No. 2019KY0021), and the Natural Science Foundation of Guangxi Province (2018GXNSFAA281308, 2019GXNSFBA185004).
Conflict of Interest
LX and SL were employed by the company Global Energy Interconnection Research Institute Co., Ltd.
The remaining authors declare that the research was conducted in the absence of any commercial or financial relationships that could be construed as a potential conflict of interest.
Acknowledgments
HLi would like to thank Aiqing Hu for her kind discussion.
References
Ashby, E. C. (1964). The direct synthesis of amine alanes. J. Am. Chem. Soc. 86, 1882–1883. doi: 10.1021/ja01063a066
Brinks, H. W., Brown, C., Jensen, C. M., Graetz, J., Reilly, J. J., and Hauback, B. C. (2007a). The crystal structure of γ-AlD3. J. Alloy. Compd. 441, 364–367. doi: 10.1016/j.jallcom.2006.09.139
Brinks, H. W., Istad-Lem, A., and Hauback, B. C. (2006). Mechanochemical synthesis and crystal structure of α'-AlD3 and α-AlD3. J. Phys. Chem. B 110, 25833–25837. doi: 10.1021/jp0630774
Brinks, H. W., Langley, W., Jensen, C. M., Graetz, J., Reilly, J. J., and Hauback, B. C. (2007b). Synthesis and crystal structure of β-AlD3. J. Alloy. Compd. 433, 180–183. doi: 10.1016/j.jallcom.2006.06.072
Brower, F. M., Matzek, N. E., Reigler, P. F., Rinn, H. W., Roberts, C. B., Schmidt, D. L., et al. (1976). Preparation and properties of aluminum hydride. J. Am. Chem. Soc. 98, 2450–2453. doi: 10.1021/ja00425a011
Chizinsky, G., Evans, G. G. T., Gibb, R. P., and Rice, M. J. (1955). Non-solvated aluminum hydride. J. Am. Chem. Soc. 77, 3164–3165. doi: 10.1021/ja01616a092
Finholt, A. E., Bond, A. C., and Schlesinger, H. I. (1947). Lithium aluminum hydride, aluminum hydride and lithium gallium hydride, and some of their applications in organic and inorganic chemistry. J. Am. Chem. Soc. 69, 1199–1203. doi: 10.1021/ja01197a061
Gao, S., Liu, H., Wang, X., Xu, L., Liu, S., Sheng, P., et al. (2017). Hydrogen desorption behaviors of γ-AlH3: diverse decomposition mechanisms for the outer layer and the inner part of γ-AlH3 particle. Int. J. Hydrogen Energy 42, 25310–25315. doi: 10.1016/j.ijhydene.2017.08.074
Graetz, J. (2009). New approaches to hydrogen storage. Chem. Soc. Rev. 38, 73–82. doi: 10.1039/B718842K
Graetz, J., and Reilly, J. J. (2006). Thermodynamics of the α, β and γ polymorphs of AlH3. J. Alloy. Compd. 424, 262–265. doi: 10.1016/j.jallcom.2005.11.086
Graetz, J., Reilly, J. J., Yartys, V. A., Maehlen, J. P., Bulychev, B. M., Antonov, V. E., et al. (2011). Aluminum hydride as a hydrogen and energy storage material: past, present and future. J. Alloy. Compd. 509, S517–S528. doi: 10.1016/j.jallcom.2010.11.115
Liu, H., Wang, X., Dong, Z., Cao, G., Liu, Y., Chen, L., et al. (2013). Dehydriding properties of γ-AlH3. Int. J. Hydrogen Energy 38, 10851–10856. doi: 10.1016/j.ijhydene.2013.02.095
Orimo, S., Nakamori, Y., Kato, T., Brown, C., and Jensen, C. M. (2006). Intrinsic and mechanically modified thermal stabilities of α-, β- and γ-aluminum trihydrides AlH3. Appl. Phys. A 83, 5–8. doi: 10.1007/s00339-005-3468-x
Sandrock, G., Reilly, J., Graetz, J., Zhou, W. M., Johnson, J., and Wegrzyn, J. (2005). Accelerated thermal decomposition of AlH3 for hydrogen-fueled vehicles. Appl. Phys. A 80, 687–690. doi: 10.1007/s00339-004-3105-0
Sartori, S., Istad-Lem, A., Brinks, H. W., and Hauback, B. C. (2009). Mechanochemical synthesis of alane. Int. J. Hydrogen Energy 34, 6350–6356. doi: 10.1016/j.ijhydene.2009.06.019
Sartori, S., Opalka, S. M., Løvvik, O. M., Guzik, M. N., Tang, X., and Hauback, B. C. (2008). Experimental studies of α-AlD3 and α′-AlD3 versus first-principles modelling of the alane isomorphs. J. Mater. Chem. 18, 2361–2370. doi: 10.1039/b718896j
Keywords: hydrogen storage, aluminum hydride, synthesis, desolvation, desorption
Citation: Liu H, Ma H, Zhang L, Gao S, Wang X, Xu L, Liu S, Huang X, Lu C, Luo H, Ning H, Lan Z and Guo J (2020) Wet Chemical Synthesis of Non-solvated Rod-Like α'-AlH3 as a Hydrogen Storage Material. Front. Chem. 7:892. doi: 10.3389/fchem.2019.00892
Received: 06 November 2019; Accepted: 10 December 2019;
Published: 15 January 2020.
Edited by:
Yongfeng Liu, Zhejiang University, ChinaReviewed by:
Yijing Wang, Nankai University, ChinaMohammad Ismail, University of Malaysia Terengganu, Malaysia
Ping Li, University of Science and Technology Beijing, China
Copyright © 2020 Liu, Ma, Zhang, Gao, Wang, Xu, Liu, Huang, Lu, Luo, Ning, Lan and Guo. This is an open-access article distributed under the terms of the Creative Commons Attribution License (CC BY). The use, distribution or reproduction in other forums is permitted, provided the original author(s) and the copyright owner(s) are credited and that the original publication in this journal is cited, in accordance with accepted academic practice. No use, distribution or reproduction is permitted which does not comply with these terms.
*Correspondence: Haizhen Liu, bGl1aHpAZ3h1LmVkdS5jbg==; Xinhua Wang, eGluaHdhbmdAemp1LmVkdS5jbg==; Jin Guo, Z3VvamluQGd4dS5lZHUuY24=