- 1Laboratory of Inkjet Printing of Functional Materials, SCAMT Institute, ITMO University, Saint-Petersburg, Russia
- 2Istituto per lo Studio dei Materiali Nanostrutturati, CNR, Palermo, Italy
Silicon quantum dots (SiQDs) are semiconductor Si nanoparticles ranging from 1 to 10 nm that hold great applicative potential as optoelectronic devices and fluorescent bio-marking agents due to their ability to fluoresce blue and red light. Their biocompatibility compared to conventional toxic Group II-VI and III-V metal-based quantum dots makes their practical utilization even more attractive to prevent environmental pollution and harm to living organisms. This work focuses on their possible use for light-emitting diode (LED) manufacturing. Summarizing the main achievements over the past few years concerning different Si quantum dot synthetic methods, LED formation and characteristics, and strategies for their stabilization by microencapsulation and modification of their surface by specific ligands, this work aims to provide guidance en route to the development of the first stable Si-based light-emitting diodes.
Introduction
Silicon quantum dots are nanometer-sized particles of crystalline silicon with properties of great interest in light of photonics, microelectronics, and biotechnological applications: high quantum yield (QY, the ratio between the number of photons emitted by a fluorophore and the number of absorbed photons), high lifetime of photoluminescence (PL), wide range of wavelength emission, and non-toxicity (Pavesi and Turan, 2010). Affecting the efficient emission and absorption of light, silicon in the bulk state is a semiconductor with an indirect bandgap. Yet, a decrease in particle size to <5 nm (the excitonic Bohr radius of silicon) allows the conversion of Si particles from indirect to direct bandgap materials with a high quantum yield (QY) of photoluminescence to up to 90% (Li et al., 2016; Gelloz et al., 2019).
In silicon SiQDs of < 5 nm in size, the photoluminescence intensity dramatically increases and blue shifts with further decreasing particle size (Sychugov et al., 2016). As happens with other QDs, the optical and electrical properties of SiQDs can be controlled by varying the particle size, the crystallinity, the nature of the surface groups and the surrounding matrix, and by doping with transition metals (Sychugov et al., 2016).
The methods for producing nanosized silicon particles are rather well-studied and can be divided into chemical and physical approaches. The first include laser ablation (Li et al., 2004; Beard et al., 2007; Vendamani et al., 2015; Xin et al., 2017) and non-thermal plasma synthesis (Cheng et al., 2010; Yasar-Inceoglu et al., 2012; Liu et al., 2016). The second include electrochemical etching (Sato et al., 2009; Castaldo et al., 2014; Chen et al., 2019), reduction of silicon halides (Tilley and Yamamoto, 2006; Cheng et al., 2012; Choi et al., 2014; Sacarescu et al., 2016), thermal destruction of silicon-rich oxides (Hessel et al., 2007, 2011), hydrothermal decomposition of different Si-contained organic precursors (Lopez-Delgado et al., 2017; Liu et al., 2018b; Phan et al., 2018; Yi et al., 2019), oxidation of sodium silicide or Zintl monoclinic phase (Na4Si4) (Neiner et al., 2006; Atkins et al., 2011; Beekman et al., 2019), mechanochemistry (Chaudhary et al., 2014), processing porous silicon (Gongalsky M. et al., 2019), and others (Holmes et al., 2001; Dasog et al., 2012).
Recent reviews on the utilization of SiQDs in bioimaging and biosensing (Cheng et al., 2014; McVey and Tilley, 2014; Cheng and Guan, 2017; Ji et al., 2018), solar cells (Chen and Yang, 2015), nonlinear optics (Bisadi et al., 2015), and photonics (Priolo et al., 2014; Zhao et al., 2018) show the broad interest and scope of the research concerning these nanomaterials. Less attention has been paid to the features of Si quantum dot-based light-emitting diodes (LEDs), even though in principle one of the most valuable applications of said quantum dots would be in making new LEDs based on abundant silicon in place of current commercial LEDs, which are based on rare earths or on organic phosphors (Buckley et al., 2017).
Compared to organic phosphors, LEDs based on Si QDs are color-pure and photostable and have a narrow emission peak and a wide spectrum of emission controlled by particle size (Cheng et al., 2014). Unfortunately, Si-based LEDs have a very short lifetime. Moreover, the quantum yield is generally low. In general, the monodispersity of silicon QDs is improving lifetime of LED, but quick degradation of LEDs based on nanosized Si occurs due to diffusion and migration of Si atoms to the outer surface of the Si nanoparticle (Maier-Flaig et al., 2013a). Two possible solutions for improving stability have been investigated: microencapsulation of SiQDs or functionalization of their surface. The presence of a shell in encapsulated silicon QDs in which the shell material consists of particles with a larger bandgap, compatible with the lattice of Si QDs, makes it possible to increase the probability of radiative recombination by isolating excitons from surface states (Gong et al., 2015). Another stabilization factor for SiQDs may be encapsulation, the variants of which are considered in the examples of solid dielectric matrices for solar cell applications (Chen and Yang, 2015) and biocompatible polymer matrices for bioimaging (Dasog et al., 2016). Aiming to provide guidelines en route to the development of the first stable Si-based light-emitting diodes, in the following, we summarize recent advances in the field of Si-based LEDs, focusing on SiQD synthesis methods affording high (45–90%) QY of photoluminescence, strategies of encapsulation, and recent progress in the formation of LEDs using Si quantum dots.
SiQD Preparation
Techniques for SiQD synthesis are divided into physical and chemical methods. Most physical routes are top-down (laser generation, plasma synthesis), with bottom-up methods consisting only of plasma synthesis. Chemical methods include both top-down (decomposition of Si-based precursors, electrochemical etching) and bottom-up approaches (reduction of silicon halides, Zintl phases oxidation). Scheme 1 illustrates the main routes divided into the two main methods.
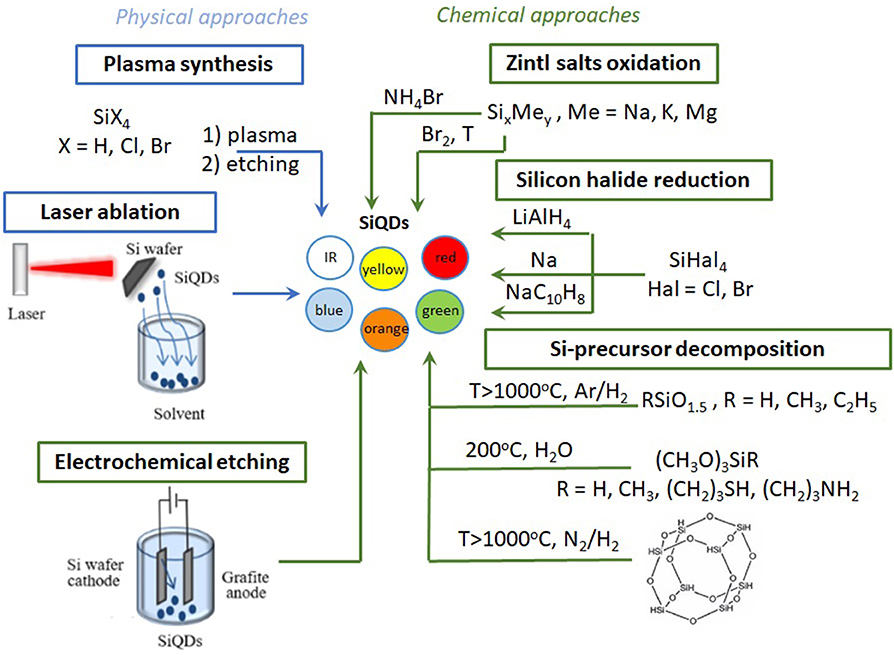
Scheme 1. Different approaches to SiQDs synthesis. Blue lines correspond to the physical approaches for SiQD synthesis; green lines correspond to the chemical approaches.
Physical Routes to Synthesizing SiQDs
Laser Generation
Irradiation of a Si plate with the light of a laser of sufficient power (number of monochromatic photons) results in the formation of QDs of high purity and crystallinity (Li et al., 2004; Beard et al., 2007; Vendamani et al., 2015; Xin et al., 2017). The low monodispersity and low stability of the resulting quantum dots are the main limitations of the method. Nevertheless, it was recently shown that, when extending the femtosecond laser ablation time from 30 to 120 min, the size of the SiQDs formed in 1-octene varied from 4.2 to 1.4 nm, with measured PL quantum yield going from 23.6 to 55.8% (Zhang et al., 2018).
Plasma Synthesis
The non-thermal plasma method is used both to obtain Si nanoparticles embedded in thin films and to synthesize free-standing silicon nanoparticles. Hot electrons in a plasma during a microwave discharge lead to the dissociation of precursor molecules such as SiX4 (X = H, Cl, Br) (Liu et al., 2016). The advantage of the method is a wide choice of fluorescent color of the nanoparticles obtained. Unlike most bottom-up methods, where only blue-green colors are available, red to orange fluorescence can be obtained using non-thermal plasma synthesis (Cheng et al., 2010; Yasar-Inceoglu et al., 2012). The method requires the utilization of special equipment. Yet, it affords nanocrystals reaching very high luminescence efficiency (QY up to 90%), as lately shown for Si/SiO2 core-shell QDs obtained via non-thermal plasma synthesis followed by formation of a thin (~1 nm) oxide shell via high-pressure water vapor annealing (Gelloz et al., 2019).
Chemical Routes to SiQDs
Electrochemical Etching
The electrochemical generation of Si quantum dots makes use of a Si wafer as the cathode and graphite as the anode. The electrolyte generally consists of aqueous HF with H2O2 or HNO3 and different additives (polyoxometalates, for example) (Kang et al., 2007). The technique allows the rapid creation of SiQDs with a range of light of fluorescence from blue to red and with rather narrow size dispersion (Sato et al., 2009; Castaldo et al., 2014; Chen et al., 2019). Originally, the Si QDs thereby obtained were characterized by low QY, but recent advances devoted to surface modification of SiQDs resulted in enhanced QY, up to 55% (Tu et al., 2016).
Zintl Salt Oxidation
The reaction of Zintl salts (MeySix, Me = Na, K, Mg, etc.) with silicon halides, gaseous bromine, or ammonium bromide in a boiling solution of glyme or under microwave irradiation affords SiQDs (Neiner et al., 2006; Atkins et al., 2011; Beekman et al., 2019). The advantages of the method are accessibility and scalability, thanks to the use of conventional reagents and equipment that is characteristic of conventional colloidal benchtop chemistry. However, the technique only affords Si nanocrystals fluorescing in the blue-green range of the light spectrum. The luminescence efficiency achieved, in terms of QY, is up to 50% (Bart van Dam et al., 2018).
Reduction of Silicon Halides
The reduction of SiCl4 using sodium naphthalenide, sodium, lithium aluminum hydride, or tetraethylorthosilicate as a reducing agent quickly produces Si nanocrystals (Cheng et al., 2012; Choi et al., 2014; Sacarescu et al., 2016). As expected, however, the particle size distribution is very wide. The addition of surfactant molecules to create micelle “nanoreactors” gives some control over the size (Tilley and Yamamoto, 2006). Usually, this method only gives blue luminescent nanocrystals of colloidal silicon, but high QY up to 90% can be obtained (Li et al., 2016).
Decomposition of Si-Containing Precursors
The hydrothermal decomposition of organosilicates such as N-[3-(trimethoxysilyl)propyl]-ethylenediamine (DAMO), 3-aminopropyl triethoxysilane (APTES), or 3-aminopropyl trimethoxysilane (APTMS), in the presence of reducing agents such as LiAlH4, sodium citrate, NaBH4, and thiourea affords Si QDs (Dasog et al., 2012; Lopez-Delgado et al., 2017; Liu et al., 2018b; Phan et al., 2018; Yi et al., 2019). By varying the reaction time, temperature, and the nature of reducing agent and of the precursor, nanocrystals with QY 65–85% can be synthesized (Ma et al., 2018; Abdelhameed et al., 2019). It is also possible to synthesize SiQDs by the thermal decomposition either of silicon monoxide (SiO) powder heated to 1,350°C (Lu et al., 2017) or of other precursors, such as silsequioxanes, followed by etching and hydrosilylation (Yu et al., 2017).
Template Synthesis
SiQDs could be obtained in gram-scale quantity by metallothermal reduction. In one approach, mesoporous SiO2 obtained via template-assisted sol-gel synthesis is reduced using magnesium powder at 500°C to yield silicon nanocrystals that are reacted with trioctylphosphine oxide to yield hydroxyl-terminated, encapsulated Si QDs exhibiting red luminescence (Dasog et al., 2012). Free-standing NCs liberated using HF acid and further functionalized with alkyl groups yield NCs that are dispersible in organic solvents with QY up to 48% (Kirshenbaum et al., 2018).
Data concerning the state of the art in SiQDs synthesis with respect to luminescence efficiency (QY of PL 45–90%), wavelength of emission, average nanoparticle size, and surface group are summarized in Table 1A.
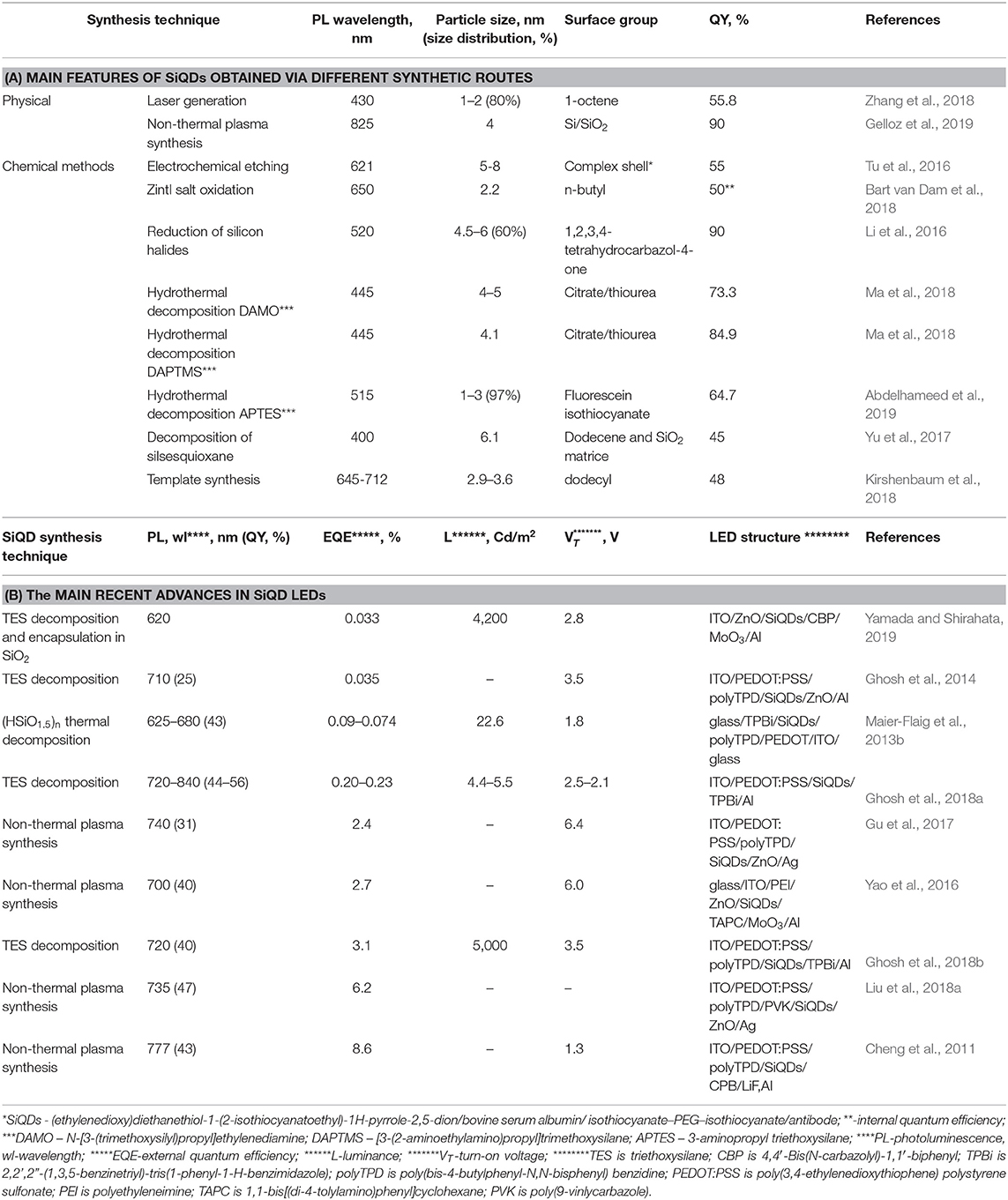
Table 1. Main features of SiQDs obtained via different synthetic routes and the main recent advances in SiQD LEDs.
Size Separation of SiQDs
Size separation methods of SiQDs are important because precise monodispersity is required in many applications, including LED devices (Maier-Flaig et al., 2013a), due to the dependence of luminescence on the nanocrystal size and size distribution, influencing the purity of the emitted color and the quantum yield. Several techniques can be applied for size-separation of QDs: field flow fractionation, membrane methods, and size exclusion chromatography (Mori, 2015). Field flow fractionation is directed by variant force fields: crossflow stream, temperature gradient, electrical potential gradient, centrifugal, dielectrophoretic, and magnetic forces. However, the application of an electric or magnetic field imposes restrictions on the particles used, since they must contain ionic/dipole fragments or have magnetic properties (Mastronardi et al., 2012). Ultracentrifugation and size-selective precipitation are the most common and scalable approaches to produce SiQDs with polydispersity index <1.01 (Rinck et al., 2015; Brown et al., 2017).
Microencapsulation of SiQDs
The application of SiQDs requires their chemical and physical stabilization (Maier-Flaig et al., 2013a; Buckley et al., 2017). Modern stabilization techniques include ligand exchange (Purkait et al., 2016) and microencapsulation in inorganic (Chen and Yang, 2015) or organic (polymer) (Dasog et al., 2016) matrixes. Organosilicon polymers, in particular, are promising candidates for encapsulation due to their affinity to SiQDs, high transparency in the visible region, and high thermal and photostability (Pagliaro, 2009; Vinogradov and Vinogradov, 2014).
Hydrolysis and Polycondensation of Trichlorosilane and Methyl Trichlorosilane
The first attempt at the encapsulation of SiQDs involved thermal processing of hydrogen silsesquioxane SiQD precursor in 5% H2/95%Ar in the presence of a silica matrix (HSiO1.5)n or of a methyl-modified silica matrix [(HSiO1.5)n(CH3SiO1.5)m], in turn obtained from hydrolytic polycondensation of HSiCl3 or of HSiCl3 and (CH3)3SiCl (Henderson et al., 2009). An important finding was that greater networking and cross-linking density of (HSiO1.5)n, resulted in the formation of smaller SiQDs in comparison to HSQ, whereas increasing the amount of methyl groups in the organically modified silica (ORMOSIL) produced larger Si nanocrystals (Palmisano et al., 2006).
Encapsulation in Mesoporous Silica
A straightforward approach lately demonstrated to increase the water dispersibility and photostability of SiQDs requires encapsulation into mesoporous silica through a simple condensation reaction in which mesoporous silica dispersed in toluene is mixed with a SiQD solution in C2H5OH, followed by heating to 110°C for reflux for 3 h. The resulting encapsulated SiQD has excellent hydrophilicity, good biocompatibility, low cytotoxicity, retains a high surface area, and exhibits better fluorescence stability in acidic solutions, making it ideally suited for biological applications (Huang et al., 2018; Phatvej et al., 2019). A procedure for obtaining SiQDs capped with an Si–C bonded alkyl layer by heating in an ultrahigh vacuum at 200°C has been reported (Chao et al., 2007). This type of encapsulation may be useful for the controlled preparation of new quantum-confined silicon structures and could facilitate their mass spectroscopic study (Gongalsky M. B. et al., 2019).
Application of SiQDs in Led Devices
The main characteristics of LEDs are the turn-on voltage (VT), which characterizes the beginning of working of the device, luminance (L), which describes the brightness of the device, and external quantum efficiency (EQE, Equation 1), which is associated with the efficiency of the diode (Ghosh et al., 2018a):
where q is the electron charge, P is the optical power density, J is the current density, and E is the energy of a photon emitted by the LED. Table 1B summarizes recent advances in SiQD LEDs producing light-emitting diodes with the highest EQEs. Table 1 also includes a description of the LED design. We briefly remind the reader that design generally involves one electrode being made from indium tin oxide (ITO) glass and the other from aluminum or silver. The multilayer structure of a typical LED consists of an electron injection layer, an electron transfer layer, an optically active layer, a hole transfer layer, and a hole injection layer (Khriachtchev, 2016). Nanoparticles of ZnO are usually used as the material for the electron transfer layer (Yang et al., 2016; Kim and Park, 2017), whereas MoO3, WO3 are often used in the hole transfer layer due to the simplicity of their synthesis (Son et al., 2009; Wang et al., 2014). The best values of EQE for SiLED are 8.6% for near-infrared emission (Cheng et al., 2011), while for white, red, and orange emissions, values of EQE are less than 0.1% (Maier-Flaig et al., 2013b; Yamada and Shirahata, 2019). There are several ways of improving LED characteristics, including using a device with an inverted structure of instead of a direct one (Yao et al., 2016; Ghosh et al., 2018b; Yamada and Shirahata, 2019), the control of the thickness of the SiQDs and polyTPD layers (Ghosh et al., 2014), the control of the size-dependence of SiQDs (Maier-Flaig et al., 2013a), and using aromatic ligands on SiQD surfaces instead of aliphatic ones (Liu et al., 2018a).
Conclusions and Perspectives
Significant progress has been made in the synthesis of Si quantum dots with high quantum yield. The development of light-emitting diodes based on SiQDs has so far been limited by their short lifetime, ranging from several hours (Gu et al., 2017; Liu et al., 2018a) to several days (Maier-Flaig et al., 2013b). Fundamental recent works aimed at investigating the origins of such low lifetimes have shown that the destruction of the diode is associated with the migration and diffusion of nanoparticles, as well as with the appearance of macro- and microscopic surface defects on the layers (Maier-Flaig et al., 2013a). Monodisperse SiQDs, in any case, are less prone to migration, allowing for a considerably longer lifetime of the device. Furthermore, significant progress in nanomaterial synthesis, translating into photoluminescence quantum yield of up to 90%, has not been matched in electroluminescence, as Si-based LEDs generally achieve QY of up to 47%. Intense research efforts are currently being aimed at developing SiQDs with high QY and external quantum efficiency and long lifetime. Along with the use of monodisperse Si nanoparticles, their microencapsulation holds promise for the first practical applications. While, for certain photonics technologies such as photoluminescence-based sensing in biology, encapsulation of SiQDs in mesoporous silica particles is already suitable for practical uses, this is not yet the case for LEDs. Due to the low cost, large abundance, and excellent health and environmental profile of silicon, when and if the issue of their poor stability will be solved, the use of silicon quantum dots will develop to become the dominant technology in photonics.
Author Contributions
MA and SM organized and wrote the manuscript. AV and MP discussed the results. All authors approved this manuscript.
Funding
This work was financially supported by the Russian Science Foundation (project No 16-19-10346).
Conflict of Interest
The authors declare that the research was conducted in the absence of any commercial or financial relationships that could be construed as a potential conflict of interest.
References
Abdelhameed, M., Aly, S., Maity, P., Manni, E., Mohammed, O. F., and Charpentier, P. A. (2019). Impact of the chemical nature and position of spacers on controlling the optical properties of silicon quantum dots. Phys. Chem. Chem. Phys. 21, 17096–17108. doi: 10.1039/C9CP03537K
Atkins, T. M., Thibert, A., Larsen, D. S., Dey, S., Browning, N. D., and Kauzlarich, S. M. (2011). Femtosecond ligand/core dynamics of microwave-assisted synthesized silicon quantum dots in aqueous solution. J. Am. Chem. Soc. 133, 20664–20667. doi: 10.1021/ja207344u
Bart van Dam, B., Osorio, C. I., Hink, M. A., Muller, R., Koenderink, A. F., and Dohnalova, K. (2018). High internal emission efficiency of silicon nanoparticles emitting in the visible range. ACS photonics 5, 2129–2136. doi: 10.1021/acsphotonics.7b01624
Beard, M. C., Knutsen, K. P., Yu, P., Luther, J. M., Song, Q., Metzger, W. K., et al. (2007). Multiple exciton generation in colloidal silicon nanocrystals. Nano Lett. 7, 2506–2512. doi: 10.1021/nl071486l
Beekman, M., Kauzlarich, S. M., Doherty, L., and Nolas, G. S. (2019). Zintl phases as reactive precursors for synthesis of novel silicon and germanium-based materials. Materials 12:1139. doi: 10.3390/ma12071139
Bisadi, Z., Mancinelli, M., Manna, S., Tondini, S., Bernard, M., Samusenko, A., et al. (2015). Silicon nanocrystals for nonlinear optics and secure communications. Appl. Mater. Sci. 212, 2659–2671. doi: 10.1002/pssa.201532528
Brown, S. L., Miller, J. B., Anthony, R. J., Kortshagen, U. R., Kryjevski, A., and Hobbie, E. K. (2017). Abrupt size partitioning of multimodal photoluminescence relaxation in monodisperse silicon nanocrystals. ACS nano 11, 1597–1603. doi: 10.1021/acsnano.6b07285
Buckley, S., Chiles, J., McCaughan, A. N., Moody, G., Silverman, K. L., Stevens, M. J., et al. (2017). All-silicon light-emitting diodes waveguide-integrated with superconducting single-photon detectors. Appl. Phys. Lett. 111:141101. doi: 10.1063/1.4994692
Castaldo, A., Antonaia, A., and Addonizio, M. L. (2014). Synthesis of silicon quantum dots in zinc silicate matrix by low-temperature process: optical, structural and electrical characterization. Thin Solid Films 562, 172–180. doi: 10.1016/j.tsf.2014.04.044
Chao, Y., Šiller, L., Krishnamurthy, S., Coxon, P. R., Bangert, U., Gass, M., et al. (2007). Evaporation and deposition of alkyl-capped silicon nanocrystals in ultrahigh vacuum. Nat Nanotechnol. 2, 486–489. doi: 10.1038/nnano.2007.224
Chaudhary, A. L., Sheppard, D. A., Paskevicius, M., Saunders, M., and Buckley, C. E. (2014). Mechanochemical synthesis of amorphous silicon nanoparticles. RSC Advances, 4, 21979–21983. doi: 10.1039/C3RA47431C
Chen, X., and Yang, P. (2015). Preparation and photovoltaic properties of silicon quantum dots embedded in a dielectric matrix: a review. J. Mater. Sci. Mater. Electr, 26, 4604–4617. doi: 10.1007/s10854-015-3147-4
Chen, Y., Sun, L., Liao, F., Dang, Q., and Shao, M. (2019). Fluorescent-stable and water-soluble two-component-modified silicon quantum dots and their application for bioimaging. J. Lumin. 215:116644. doi: 10.1016/j.jlumin.2019.116644
Cheng, K. Y., Anthony, R., Kortshagen, U. R., and Holmes, R. J. (2010). Hybrid silicon nanocrystal–organic light-emitting devices for infrared electroluminescence. Nano Lett., 10, 1154–1157. doi: 10.1021/nl903212y
Cheng, K. Y., Anthony, R., Kortshagen, U. R., and Holmes, R. J. (2011). High-efficiency silicon nanocrystal light-emitting devices. Nano lett. 11, 1952–1956. doi: 10.1021/nl2001692
Cheng, X., Gondosiswanto, R., Ciampi, S., Reece, P. J., and Gooding, J. J. (2012). One-pot synthesis of colloidal silicon quantum dots and surface functionalization via thiolene click chemistry. Chem. Commun. 48:11874–11876. doi: 10.1039/c2cc35954e
Cheng, X., and Guan, B. (2017). Optical biosensing and bioimaging with porous silicon and silicon quantum dots (Invited Review). Prog. Electromagn. Res. 160, 103–121. doi: 10.2528/PIER17120504
Cheng, X., Lowe, S. B., Reece, P. J., and Gooding, J. J. (2014). Colloidal silicon quantum dots: from preparation to the modification of self-assembled monolayers (SAMs) for bio-applications. Chem. Soc. Rev. 43, 2680–2700. doi: 10.1039/c3cs60353a
Choi, J.-H., Dung, M. X., and Jeong, H. D. (2014). Novel synthesis of covalently linked silicon quantum dote polystyrene hybrid materials: silicon quantum dote polystyrene polymers of tunable refractive index. Mater. Chem. Phys. 148, 463–472. doi: 10.1016/j.matchemphys.2014.08.016
Dasog, M., Kehrle, J., Rieger, B., and Veinot, J. G. (2016). Silicon nanocrystals and silicon-polymer hybrids: synthesis, surface engineering, and applications. Angew. Chem. Int. Ed. 55, 2322–2339. doi: 10.1002/anie.201506065
Dasog, M., Yang, Z., and Veinot, J. G. (2012). Size-controlled solid state synthesis of luminescent silicon nanocrystals using Stöber silica particles. CrystEngComm. 14, 7576–7578. doi: 10.1039/C2CE25950H
Gelloz, B., Juangs, F. B., Nozaki, T., Koji, A., Koshida, N., and Jin, L. (2019). Si/SiO2 core/shell luminescent silicon nanocrystals and porous silicon powders with high quantum yield, long lifetime and good stability. Front. Phys. 7:47. doi: 10.3389/fphy.2019.00047
Ghosh, B., Hamaoka, T., Nemoto, Y., Takeguchi, M., and Shirahata, N. (2018a). Impact of anchoring monolayers on the enhancement of radiative recombination in light-emitting diodes based on silicon nanocrystals. J. Phys. Chem. C 122, 6422–6430. doi: 10.1021/acs.jpcc.7b12812
Ghosh, B., Masuda, Y., Wakayama, Y., Imanaka, Y., Inoue, J. I., Hashi, K., et al. (2014). Hybrid white light emitting diode based on silicon nanocrystals. Adv. Funct. Mater. 24, 7151–7160. doi: 10.1002/adfm.201401795
Ghosh, B., Yamada, H., Chinnathambi, S., Özbilgin, I. N. G., and Shirahata, N. (2018b). Inverted device architecture for enhanced performance of flexible silicon quantum dot light-emitting diode. J. Phys. Chem. Lett. 9, 5400–5407. doi: 10.1021/acs.jpclett.8b02278
Gong, K., Martin, J. E., Shea-Rohwer, L. E., Lu, P., and Kelley, D. F. (2015). Radiative lifetimes of zincblende CdSe/CdS quantum dots. J. Phys. Chem. C, 119, 2231–2238. doi: 10.1021/jp5118932
Gongalsky, M., Tsurikova, U. A., Storey, C. J., Evstratova, Y. V., Kudryavtsev, A., Canham, L. T., et al. (2019). The effects of drying technique and surface pre-treatment on the cytotoxicity and dissolution rate of luminescent porous silicon quantum dots in model fluids and living cells. Faraday Discuss. doi: 10.1039/C9FD00107G
Gongalsky, M. B., Kargina, J. V., Cruz, J. F., Sanchez-Royo, J. F., Chirvony, V., Osminkina, L. A., et al. (2019). Formation of Si/SiO2 luminescent quantum dots from mesoporous silicon by sodium tetraborate/citric acid oxidation treatment. Front. Chem. 7:165. doi: 10.3389/fchem.2019.00165
Gu, W., Liu, X., Pi, X., Dai, X., Zhao, S., Yao, L., et al. (2017). Silicon-quantum-dot light-emitting diodes with interlayer-enhanced hole transport. IEEE Photonics J. 9, 1–10. doi: 10.1109/JPHOT.2017.2671023
Henderson, E. J., Kelly, J. A., and Veinot, J. G. C. (2009). Influence of HSiO1.5 Sol–Gel polymer structure and composition on the size and luminescent properties of silicon nanocrystals. Chem. Mater. 21, 5426–5434. doi: 10.1021/cm902028q
Hessel, C. M., Reid, D., Panthani, M. G., Rasch, M. R., Goodfellow, B. W., Wei, J., et al. (2011). Synthesis of ligand-stabilized silicon nanocrystals with size-dependent photoluminescence spanning visible to near-infrared wavelengths. Chem. Mater. 24, 393–401. doi: 10.1021/cm2032866
Hessel, C. M., Summers, M. A., Meldrum, A., Malac, M., and Veinot, J. G. (2007). Direct patterning, conformal coating, and erbium doping of luminescent nc-Si/SiO2 thin films from solution processable hydrogen silsesquioxane. Adv. Mater. 19, 3513–3516. doi: 10.1002/adma.200700731
Holmes, J. D., Ziegler, K. J., Doty, R. C., Pell, L. E., Johnston, K. P., and Korgel, B. A. (2001). Highly luminescent silicon nanocrystals with discrete optical transitions. J. Am. Chem. Soc. 123, 3743–3748. doi: 10.1021/ja002956f
Huang, L., Mu, Y., Chen, J., Tian, J., Huang, Q., Huang, H., et al. (2018). One-pot ultrafast preparation of silica quantum dots and their utilization for fabrication of luminescent mesoporous silica nanoparticles. Mater. Sci. Eng. C 93, 679–685. doi: 10.1016/j.msec.2018.08.035
Ji, X., Wang, H., Song, B., Chu, B., and He, Y. (2018). Silicon nanomaterials for biosensing and bioimaging analysis. Front. Chem. 6:38. doi: 10.3389/fchem.2018.00038
Kang, Z., Tsang, C. H. A., Zhang, Z., Zhang, M., Wong, N. B., Zapien, J. A., et al. (2007). A polyoxometalate-assisted electrochemical method for silicon nanostructures preparation: from quantum dots to nanowires. J. Am. Chem. Soc. 129, 5326–5327. doi: 10.1021/ja068894w
Khriachtchev, L. (2016). Silicon Nanophotonics: Basic Principles, Current Status and Perspectives. New York, NY: Jenny Stanford Publishing. ISBN: 13: 978-981-4241-13-7
Kim, J. H., and Park, J. W. (2017). Designing an electron-transport layer for highly efficient, reliable, and solution-processed organic light-emitting diodes. J. Mater. Chem. C 5, 3097–3106. doi: 10.1039/C7TC00488E
Kirshenbaum, M. J., Boebinger, M. G., Katz, M. J., McDowell, M. T., and Dasog, M. (2018). Solid-state route for the synthesis of scalable, luminescent silicon and germanium nanocrystals. ChemNanoMat. 4, 423–429. doi: 10.1002/cnma.201800059
Li, Q., Luo, T.-Y., Zhou, M., Abroshan, H., Huang, J., Kim, H. J., et al. (2016). Silicon nanoparticles with surface nitrogen: 90% quantum yield with narrow luminescence bandwidth and the ligand structure based energy law. ACS Nano. 10, 8385–8393. doi: 10.1021/acsnano.6b03113
Li, X., He, Y., and Swihart, M. T. (2004). Surface functionalization of silicon nanoparticles produced by laser-driven pyrolysis of silane followed by HF-HNO3Etching. Langmuir 20, 4720–4727. doi: 10.1021/la036219j
Liu, X., Zhang, Y., Yu, T., Qiao, X., Gresback, R., Pi, X., et al. (2016). Optimum quantum yield of the light emission from 2 to 10 nm hydrosilylated silicon quantum dots. Part. Part. Syst. Char. 33, 44–52. doi: 10.1002/ppsc.20150014
Liu, X., Zhao, S., Gu, W., Zhang, Y., Qiao, X., Ni, Z., et al. (2018a). Light-emitting diodes based on colloidal silicon quantum dots with octyl and phenylpropyl ligands. ACS Appl. Mater. Interfaces 10, 5959–5966. doi: 10.1021/acsami.7b16980
Liu, Y., Wang, Q., Guo, S., Jia, P., Shui, Y., Yao, S., et al. (2018b). Highly selective and sensitive fluorescence detection of hydroquinone using novel silicon quantum dots. Sens. Actuat. B Chem. 275, 415–421. doi: 10.1016/j.snb.2018.08.073
Lopez-Delgado, R., Higuera-Valenzuela, H. J., Zazueta-Raynaud, A., Ramos-Carrazco, A., Pelayo, J. E., Berman-Mendoza, D., et al. (2017). Solar cell efficiency improvement employing down-shifting silicon quantum dots. Microsyst. Tech. 24, 495–502. doi: 10.1007/s00542-017-3405-x
Lu, S., Wu, B., Sun, Y., Cheng, Y., Liao, F., and Shao, M. (2017). Photoluminescence of pure silicon quantum dots embedded in an amorphous silica wire array. J. Mater. Chem. C 5, 6713–6717. doi: 10.1039/c7tc01117b
Ma, S., Yue, T., Xiao, X., Cheng, H., and Zhao, D. (2018). A proof of concept study of preparing ultra bright silicon quantum dots based on synergistic effect of reductants. J. Lumin. 201, 77–84. doi: 10.1016/j.jlumin.2018.04.006
Maier-Flaig, F., KÃbel, C., Rinck, J., Bocksrocker, T., Scherer, T., Prang, R., et al. (2013a). Looking inside a working SiLED. Nano Lett. 13, 3539–3545. doi: 10.1021/nl400975u
Maier-Flaig, F., Rinck, J., Stephan, M., Bocksrocker, T., Bruns, M., KÃbel, C., et al. (2013b). Multicolor silicon light-emitting diodes (SiLEDs). Nano Lett. 13, 475–480. doi: 10.1021/nl3038689
Mastronardi, M. L., Henderson, E. J., Puzzo, D. P., and Ozin, G. A. (2012). Small silicon, big opportunities: the development and future of colloidally-stable monodisperse silicon nanocrystals. Adv. Mater. 24, 5890–5898. doi: 10.1002/adma.201202846
McVey, B. F., and Tilley, R. D. (2014). Solution synthesis, optical properties, and bioimaging applications of silicon nanocrystals. Acc. Chem. Res. 47, 3045–3051. doi: 10.1021/ar500215v
Mori, Y. (2015). Size-selective separation techniques for nanoparticles in liquid. KONA Powder Particie J. 32, 102–114. doi: 10.14356/kona.2015023
Neiner, D., Chiu, H. W., and Kauzlarich, S. M. (2006). Low-Temperature solution route to macroscopic amounts of hydrogen terminated silicon nanoparticles. J. Am. Chem. Soc. 128, 11016–11017. doi: 10.1021/ja064177q
Pagliaro, M. (2009). Silica-Based Materials for Advanced Chemical Applications. Cambridge: Royal Society of Chemistry.
Palmisano, G., Le Bourhis, E., Ciriminna, R., Tranchida, D., and Pagliaro, M. (2006). ORMOSIL thin films: tuning mechanical properties via a nanochemistry approach. Langmuir 22, 11158–11162. doi: 10.1021/la061520w
Pavesi, L., and Turan, R., (eds.). (2010). Silicon Nanocrystals: Fundamentals, Synthesis and Applications. Weinheim: John Wiley & Sons.
Phan, L. M. T., Baek, S. H., Nguyen, T. P., Park, K. Y., Ha, S., Rafique, R., et al. (2018). Synthesis of fluorescent silicon quantum dots for ultra-rapid and selective sensing of Cr(VI) ion and biomonitoring of cancer cells. Mater. Sci. Eng. C 93, 429–436. doi: 10.1016/j.msec.2018.08.024
Phatvej, W., Datta, H. K., Wilkinson, S. C., Mutch, E., Daly, A. K., and Horrocks, B. R. (2019). Endocytosis and lack of cytotoxicity of alkyl-capped silicon quantum dots prepared from porous silicon. Materials 12:1702. doi: 10.3390/ma12101702
Priolo, F., Gregorkiewicz, T., Galli, M., and Krauss, T. F. (2014). Silicon nanostructures for photonics and photovoltaics. Nat. Nanotechnol. 9, 19–32. doi: 10.1038/NNANO.2013.271
Purkait, T. K., Iqbal, M., Islam, M. A., Mobarok, M. H., Gonzalez, C. M., Hadidi, L., et al. (2016). Alkoxy-terminated si surfaces: a new reactive platform for the functionalization and derivatization of silicon quantum dots. J. Am. Chem. Soc. 138, 7114–7120. doi: 10.1021/jacs.6b03155
Rinck, J., Schray, D., Kübel, C., Powell, A. K., and Ozin, G. A. (2015). Size-dependent oxidation of monodisperse silicon nanocrystals with allylphenylsulfide surfaces. Small 11, 335–340. doi: 10.1002/smll.201401965
Sacarescu, L., Roman, G., Sacarescu, G., and Simionescu, M. (2016). Fluorescence detection system based on silicon quantum dots–polysilane nanocomposites. Express Polym. Lett. 10, 990–1002. doi: 10.3144/expresspolymlett.2016.92
Sato, K., Tsuji, H., Hirakuri, K., Fukata, N., and Yamauchi, Y. (2009). Controlled chemical etching for silicon nanocrystals with wavelength-tunable photoluminescence. Chem. Commun. 25, 3759–3761. doi: 10.1039/B903313K
Son, M. J., Kim, S., Kwon, S., and Kim, J. W. (2009). Interface electronic structures of organic light-emitting diodes with WO3 interlayer: a study by photoelectron spectroscopy. Org. Electron. 10, 637–642. doi: 10.1016/j.orgel.2009.02.017
Sychugov, I., Pevere, F., Luo, J. W., Zunger, A., and Linnros, J. (2016). Single-dot absorption spectroscopy and theory of silicon nanocrystals. Phy. Rev. B 93:161413. doi: 10.1103/PhysRevB.93.161413
Tilley, R. D., and Yamamoto, K. (2006). The microemulsion synthesis of hydrophobic and hydrophilic silicon nanocrystals. Adv. Mater. 18, 2053–2056. doi: 10.1002/adma.200600118
Tu, C.-C., Chen, K.-P., Yang, T.-A., Chou, M.-Y., Lin, L. Y., and Li, Y.-K. (2016). Silicon quantum dot nanoparticles with antifouling coatings for immunostaining on live cancer cells. ACS Appl. Mater. Interfaces 8, 13714–13723. doi: 10.1021/acsami.6b02318
Vendamani, V. S., Hamad, S., Saikiran, V., Pathak, A. P., Rao, S. V., Kumar, V. R. K., et al. (2015). Synthesis of ultra-small silicon nanoparticles by femtosecond laser ablation of porous silicon. J. Mater. Sci. 50, 1666–1672. doi: 10.1007/s10853-014-8727-9
Vinogradov, A. V., and Vinogradov, V. V. (2014). Low-temperature sol–gel synthesis of crystalline materials. RSC Adv. 4, 45903–45919. doi: 10.1021/cr300399c
Wang, G., Jiu, T., Li, P., Li, J., Sun, C., Lu, F., et al. (2014). Preparation and characterization of MoO3 hole-injection layer for organic solar cell fabrication and optimization. Sol. Energy Mater. Sol. Cells 120, 603–609. doi: 10.1016/j.solmat.2013.10.002
Xin, Y., Kitasako, T., Maeda, M., and Saitow, K. I. (2017). Solvent dependence of laser-synthesized blue-emitting Si nanoparticles: size, quantum yield, and aging performance. Chem. Phys. Lett. 674, 90–97. doi: 10.1016/j.cplett.2017.02.060
Yamada, H., and Shirahata, N. (2019). Silicon quantum dot light emitting diode at 620 nm. Micromachines 10, 318. doi: 10.3390/mi10050318
Yang, G., Tao, H., Qin, P., Ke, W., and Fang, G. (2016). Recent progress in electron transport layers for efficient perovskite solar cells. J. Mater. Chem. A 4, 3970–3990. doi: 10.1039/C5TA09011C
Yao, L., Yu, T., Ba, L., Meng, H., Fang, X., Wang, Y., et al. (2016). Efficient silicon quantum dots light emitting diodes with an inverted device structure. J. Mater. Chem. C 4, 673–677. doi: 10.1039/c5tc03064a
Yasar-Inceoglu, O., Lopez, T., Farshihagro, E., and Mangolini, L. (2012). Silicon nanocrystal production through non-thermal plasma synthesis: a comparative study between silicon tetrachloride and silane precursors. Nanotechnology 23:255604.
Yi, Y., Liu, L., Zeng, W., Lv, B., and Zhu, G. (2019). Bifunctional silicon quantum dots sensing platform for selective and sensitive detection of p-dihydroxybenzene with double signals. Microchem. J. 147, 245–252. doi: 10.1016/j.microc.2019.03.018
Yu, Y., Fan, G., Fermi, A., Mazzaro, R., Morandi, V., Ceroni, P., et al. (2017). Size-dependent photoluminescence efficiency of silicon nanocrystal quantum dots. J. Phys. Chem. C 121, 23240–23248. doi: 10.1021/acs.jpcc.7b08054
Zhang, Y. X., Wu, W. S., Hao, H. L., and Shen, W. Z. (2018). Femtosecond laser-induced size reduction and emission quantum yield enhancement of colloidal silicon nanocrystals: effect of laser ablation time. Nanotechnology 29:365706. doi: 10.1088/1361-6528/aacd75
Keywords: silicon quantum dots, synthesis method, fluorescence, quantum yield, microencapsulation, light-emitting diodes
Citation: Morozova S, Alikina M, Vinogradov A and Pagliaro M (2020) Silicon Quantum Dots: Synthesis, Encapsulation, and Application in Light-Emitting Diodes. Front. Chem. 8:191. doi: 10.3389/fchem.2020.00191
Received: 13 November 2019; Accepted: 02 March 2020;
Published: 07 April 2020.
Edited by:
Eugene A. Goodilin, Lomonosov Moscow State University, RussiaReviewed by:
Clara S. B. Gomes, New University of Lisbon, PortugalLiubov A. Osminkina, Lomonosov Moscow State University, Russia
Copyright © 2020 Morozova, Alikina, Vinogradov and Pagliaro. This is an open-access article distributed under the terms of the Creative Commons Attribution License (CC BY). The use, distribution or reproduction in other forums is permitted, provided the original author(s) and the copyright owner(s) are credited and that the original publication in this journal is cited, in accordance with accepted academic practice. No use, distribution or reproduction is permitted which does not comply with these terms.
*Correspondence: Sofia Morozova, bW9yb3pvdmFAc2NhbXQtaXRtby5ydQ==