- Center for Toxicology and Health Risk Studies, Faculty of Health Sciences, Universiti Kebangsaan Malaysia, Kuala Lumpur, Malaysia
Organotin(IV) compounds have wide applications in industrial and agricultural fields owing to their ability to act as poly(vinyl chloride) stabilizers and catalytic agents as well as their medicinal properties. Moreover, organotin(IV) compounds may have applications as antitumor, anti-inflammatory, antifungal, or antimicrobial agents based on the observation of synergistic effects following the binding of their respective ligands, resulting in the enhancement of their biological activities. In this review, we describe the antiproliferative activities of organotin(IV) compounds in various human cancer cell lines based on different types of ligands. We also discuss the molecular mechanisms through which organotin(IV) compounds induce cell death via apoptosis through the mitochondrial intrinsic pathway. Finally, we present the mechanisms of cell cycle arrest induced by organotin(IV) compounds. Our report provides a basis for studies of the antitumor activities of organotin(IV) compounds and highlights the potential applications of these compounds as anticancer metallodrugs with low toxicity and few side effects.
Introduction
Tin is a silvery white metal often found in the form of tin dioxide (SnO2), derived from the Earth’s crust. The ability of tin to form stable bonds with carbon and other heteroatoms and its inert characteristics in the presence of air and water have attracted much interest in various research fields (Van der Kerk, 1975). Importantly, tin is present in a variety of inorganic/organometallic compounds. Its outer shell contains two 5s and two 5p electrons; loss of the two electrons in the 5p orbital or sharing of all four electrons with other atoms results in the formation of the Sn2+ ion and a stable electronic configuration. In aqueous media, the chemistry of tin is more complex; its stereochemistry involves the presence of inert electron pairs (resulting in stronger shielding of inner electrons compared with outer electrons). Sn4+ and Sn2+ ions, however, cannot be found in aqueous solutions. Organotin(IV) derivatives are formed via the binding of tin and carbon (Iqbal et al., 2015; Adeyemi and Onwudiwe, 2018). The molecular geometry of these compounds varies, including monomers, dimers, oligomeric ladders, cubics, butterflies, and hexameric drums, with the geometry playing a role in their biological activity (Holmes et al., 1988; Chandrasekhar et al., 2002; Chandrasekhar et al., 2005; Hadi et al., 2019a; Khan et al., 2020).
Organotin(IV) compounds also have their own role in commercial uses. According to Ross (1965) and Ghazi et al. (2018), organotin(IV) compounds are being utilized in three main areas, including 1) poly(vinyl chloride) (PVC) stabilizers, 2) industrial and agricultural biocides, and 3) catalytic agents. Since the 1940s, organotin(IV) compounds have been considered ideal stabilizer compounds to prevent thermal degradation of PVC (Martins et al., 2016). During processing, the required concentration for organotin(IV) compounds ranges from 0.5 to 3.0 parts per hundred parts of resin. The final product is a clear, colorless polymer because organotin(IV) compounds are compatible with PVC resins and plasticizers (Piver, 1973; Ghazi et al., 2018). Moreover, the capability of organotin(IV) compounds to control many fungi and bacteria enables organotin(IV)-based preservatives to be applied as industrial and agricultural biocides. In the 1960s, antifouling paint was applied in the production of marine vessels, rather than copper oxides; the organotin(IV) compounds, including tributyltin(IV) and triphenyltin(IV) compounds (e.g., oxide, chloride, acetate, and linoleate) were used to minimize fouling, leading to longer protection of up to 18–24 months (Omae, 2003). According to Piver (1973) and Sardon et al. (2013), polyurethane foams are typically prepared through a direct route involving hexamethylene diisocyanate and 1,4-butanediol with the presence of organotin(IV) catalysts. The main steps in this mechanism include chain extension, gas reaction, and crosslinking. The formation of urethane linkages involves either a Sn–O-isocyanate-insertion bond or the action of an organotin(IV) compound as a Lewis acid (Devendra et al., 2013). Besides, organotin(IV) compounds exhibit an interesting effect when catalyzing the reaction of chain extension, allowing optimum rates for both chain extension and gas formation to be achieved. The most commonly used organotin(IV) catalysts include dibutyltin(IV) diacetate, dibutyltin(IV) dilaurate, dibutyltin(IV) dichloride, dibutyltin(IV) dilaurylmercaptide, and dimethyltin(IV) dichloride (Piver, 1973; Ghazi et al., 2018).
Over 2000 tin-based pharmacological candidates have been tested by The National Cancer Institute (Nath and Saini, 2011). Organotin(IV) derivatives, hereby, have attracted much attention within the last 2 decades owing to their potential biological activities, including antitumor (Awang et al., 2011), anti-inflammatory (Niu et al., 2014), antimicrobial (Sedaghat et al., 2013), antifungal (Najeeb et al., 2009), antinematicidal, and anti-insecticidal effects (Jain et al., 2004). Driven by the clinical achievements of cisplatin, the first platinum-based chemotherapeutic drug, researchers have focused much attention on nonplatinum-based chemotherapeutics in order to improve therapeutic efficacy and prevent severe side effects related to platinum-based drugs. Among all reported nonplatinum-based drugs, organotin(IV) compounds may be the most promising metallodrugs, as, in some cases, they portray better effects than cisplatin (Hadjikakou and Hadjiliadis, 2009; Attanzio et al., 2020) and have been shown to have substantial anticancer effects (Jan et al., 2002; Samuel et al., 2002; Shuaibu et al., 2003; Tabassum and Pettinari, 2006).
Accordingly, in this review, we discuss the antiproliferative activities and mechanisms of organotin(IV) compounds to rationalize the potential of organotin(IV) compounds to be developed as effective and reliable anticancer agents in the future.
Antiproliferative Activities of Organotin(IV) Compounds
Organotin(IV) compounds have attracted much attention recently as potential metallodrugs since the discovery of their antitumor activities (Gielen et al., 1999). Based on the wide range of organic moieties and donor ligands that are attached to the metal, several diorganotin(IV) and triorganotin(IV) compounds with in vitro antitumor properties against various solid and hematologic cancers have been studied (Gielen and Tiekink, 2005; Alama et al., 2009; Hadjikakou and Hadjiliadis, 2009). These compounds show lower toxic effects, higher antiproliferative activity, better excretion properties, and fewer side effects than other platinum-based drugs, even when used at low concentrations (Florea and Busselberg, 2011; Deo et al., 2018; Rubino et al., 2018; Attanzio et al., 2020). Additionally, novel organotin(IV) compounds have been shown to have high selectivity toward various cancer cell lines, regardless of ligand diversity (Awang et al., 2014; Girasolo et al., 2017; Attanzio et al., 2020). Niu et al. (2014), Sirajuddin et al. (2014) and Hadi et al. (2019a) stated that organotin(IV) derivatives are likely to show cytotoxic effects with the following trend: RSn3+ < R2Sn2+ < R3Sn+, with triorganotin(IV) substituents demonstrating the strongest effects. Table 1 shows the half-maximal inhibitory concentration (IC50) values for organotin(IV) derivatives against various human tumor cell lines. Here, we describe studies of the antiproliferative effects of these compounds and discuss their potential application in the treatment of cancer.
Organotin(IV) Compounds Containing Hydrazone as Ligands
Devi et al. (2020) reported a series of diorganotin(IV) compounds derived from the reaction of indole-3-butyric hydrazide with salicylaldehyde and its derivatives (Scheme 1). The hydrazone Schiff base ligands were as follows: Nʹ-(2-hydroxybenzylidene)-4-(1H-indole-3-yl) butanehydrazide [H2L1], Nʹ-(2-hydroxy-5 nitrobenzylidene)-4-(1H-indole-3-yl)butanehydrazide [H2L2], Nʹ-(5-diethylamino)-2 hydroxybenzylidene-4-(1H-indole-3-yl)butanehydrazide [H2L3], and Nʹ-(3,5-dibromo-2 hydroxybenzylidene)-4-(1Hindole-3-yl)butanehydrazide [H2L4]. The geometry around the metal center indicates the tridentate nature of Schiff base ligands coordinated by nitrogen and oxygen donor sites to dialkyl/diaryltin(IV) moieties, yielding complexes with penta-coordinated geometry. The analysis of the cytotoxicity of the compounds in human lung epithelial (A549) and human breast adenocarcinoma (MCF7) cell lines showed that ethyl compounds displayed better cytotoxic activity toward both cancer cell lines. Compound 5 (Et2SnL2) exhibited the highest cytotoxic effects, with IC50 values of 13.4 μM (A549 cells) and 15.2 μM (MCF7 cells). This was followed by the ethyl compounds of H2L3 and H2L4 ligands, which resulted in stronger cytotoxic effects for both cell lines (IC50 values of less than 30 μM) in comparison with other compounds within the corresponding groups. Notably, when compared with the standard drug, doxorubicin, the ethyl compounds were approximately eight times less toxic in normal IMR90 cells. The antiproliferative activities of diorganotin(IV) compounds (1–14) decreased in the order of Et > Bu > Ph > Me.
Liu et al. (2017) synthesized and characterized four new diorganotin(IV) compounds derived from two hydrazone Schiff base ligands (Scheme 2). The compounds were Me2SnL1 (25), Ph2SnL1 (26), Me2SnL2 (27), and Ph2SnL2 (28) (H2L1 = 5-chlorosalicylaldehyde isonicotinoyl hydrazone and H2L2 = 2-hydroxy-4-methoxybenzaldehyde isonicotinoyl hydrazone) with a trinuclear centrosymmetric structure (compounds 25 and 26) or distorted trigonal bipyramid (compounds 27 and 28) for the coordination environment. The in vitro cytotoxicity of the compounds was tested against A549 human lung cancer cells and HeLa human cervical carcinoma cells. The diphenyltin(IV) compounds 26 and 28 exhibited more potent cytotoxic activity than the dimethyltin(IV) compounds 25 and 27 in both cell lines. These results may be because the phenyl groups showed better planarity in compounds 26 and 28, which may facilitate insertion into the DNA. These results were consistent with previous studies of organotin(IV) compounds conducted by Haque et al. (2015) and Shehata et al. (2015).
Organotin(IV) Compounds Containing Dithiocarbamate as Ligands
Phenyltin(IV) compounds containing (2-methoxyethyl) methyldithiocarbamate (Scheme 3) were examined to determine their in vitro cytotoxicity in K562 human erythroleukemia cells (Kamaludin et al., 2019). The cytotoxic activity of compound 19 was twice that of compound 20 (IC50 values 4.0 and 8.0 μM, respectively). Most studies have shown that triphenyltin(IV) compounds, which contain three phenyl groups attached to the central tin(IV) atom, have greater cytotoxic effects in tested cell lines compared with diphenyltin(IV) compounds. Additionally, Biplob et al. (2008) and Ray et al. (2000) demonstrated that the molecular structure of a compound plays important roles in determining its cytotoxicity, that is, shorter alkyl substitution groups were found to increase cytotoxicity.
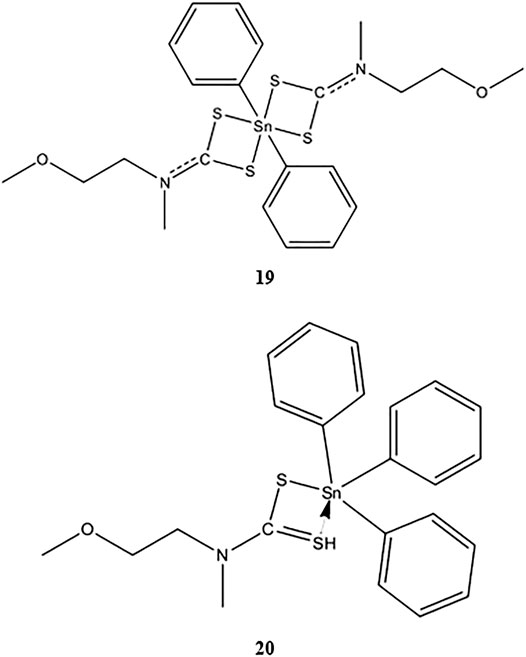
SCHEME 3. Chemical structure of 19–20 (Mohamad et al., 2016; Mohamad et al., 2018).
Four newly identified organotin(IV) compounds containing N,N-diallyldithiocarbamate (Scheme 4) were synthesized and characterized by Adeyemi et al. (2019). Compounds 22 and 23 were shown to have skewed trapezoidal–bipyramidal geometry. The antiproliferative activity of the compounds was evaluated in HeLa human cervical carcinoma cells, and the results showed that compound 24 possessed excellent cytotoxic effects (IC50 value as low as 2 μM). These findings may be explained by the lipophilicity of the compound, which was related to the presence of two phenyl groups (Kadu et al., 2015).
Two novel organotin(IV) compounds as methoxyethyldithiocarbamate ligands (Scheme 5) were synthesized and characterized in a study by Awang et al. (2016). Further determination of the cytotoxic effects of dibutyltin(IV) and diphenyltin(IV) in HL-60 human leukemia cells showed that both compounds had very low IC50 values (0.40 and 0.35 μM for compounds 29 and 30, respectively), supporting the strong cytotoxic activities of both compounds. These findings were consistent with a previous study by Awang et al. (2010) demonstrating the high cytotoxicity of dibutyltin(IV) compounds against tested cell lines.
Dithiocarbamate ligands have the potential to strongly bind and stabilize metal ions, resulting in a high oxidation number (Arafat et al., 2013). Chelation of the tin ion by dithiocarbamate ligands reduces the polar nature of the metal center, thereby enhancing permeability by increasing lipophilicity. This increased lipophilicity may enhance the biological activity of the compounds (Javed et al., 2016). Menezes et al. (2005) and Mamba et al. (2010) stated that the individual properties of organotin(IV) and dithiocarbamate constituents may induce synergistic effects, leading to better biological activities.
Organotin(IV) Compounds Containing 1,2,4-Triazolo[1,5-a]Pyrimidines as Ligands
Attanzio et al. (2020) reported the in vitro antiproliferative activity of organotin(IV) compounds derived from 1,2,4-triazolo[1,5-a]pyrimidines (Scheme 6) in three different human tumor cell lines (HCT-116 colorectal carcinoma cells, HepG2 hepatocarcinoma cells, and MCF-7 breast cancer cells). The compounds were as follows: n-Bu3Sn(5tpO) (15), n-Bu3Sn(mtpO) (16), n-Bu3Sn(HtpO2) (17), and Ph3Sn(HtpO2) (18), where 5HtpO = 4,5-dihydro-5-oxo-[1,2,4]triazolo-[1,5-a]pyrimidine, HmtpO = 4,7-dihydro-5-methyl-7-oxo-[1,2,4]triazolo-[1,5-a]pyrimidine, and H2tpO2 = 4,5,6,7-tetrahydro-5,7-dioxo-[1,2,4]triazolo-[1,5 a]-pyrimidine. Interestingly, all of the compounds possessed IC50 values in the submicromolar range (less than 1 µM) against the three cell lines. Moreover, compound 16 showed the highest cytotoxic effects in all cell lines. All of the compounds showed high selectivity indexes (SIs) toward tumor cell lines (SI > 90).
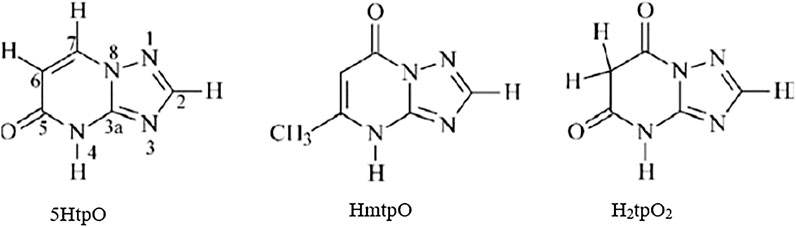
SCHEME 6. Molecular structure of 25–28 (Girasolo et al., 2005; Attanzio et al., 2020).
The involvement of 1,2,4-triazolo[1,5-a] pyrimidine in the coordination of different nitrogen atoms could be used as a model system to mimic the reactivity between purines and several metal ions. Alterations in the numbers of exocyclic moieties on the triazolopyrimidine rings could increase the versatility and cytotoxicity properties of the compounds (Salas et al., 1999; Attanzio et al., 2020).
Organotin(IV) Compounds Containing Chloride as Ligands
The antiproliferative effects of triorganotin(IV) compounds containing chloride (31 and 32) were assessed in MCF-7 human breast cancer cells. Fickova et al. (2015) showed that both tributyltin(IV) and triphenyltin(IV) had lower IC50 values (submicromolar range), demonstrating high potency of antitumor effects. Additionally, the tributyltin(IV) compound 31 showed greater growth inhibition in the tested cell lines compared with triorganotin(IV) (32). These findings were consistent with those reported by Attanzio et al. (2020) and Awang et al. (2016; 2011), who showed that butyltin(IV) compounds exhibited better cytotoxic effects than phenyltin(IV) compounds, regardless of the types of ligands attached to the central tin atom.
Organotin(IV) chloride derivatives are nanomolar agonists that can bind to retinoid X receptors (RXR) subtypes and peroxisome proliferator-activated receptor. These compounds also function as transcriptional activators. RXR subtypes modulate hormonal signals within the cells by acting as heterodimeric partners of various other nuclear receptors (Brtko and Thalhamer, 2003; Nakanishi et al., 2005; Brtko, 2007; le Maire et al., 2009; Brtko and Dvorak, 2011; Fickova et al., 2015). The organotin(IV) chloride compounds reported by Fickova et al. (2015) were shown to inhibit cell growth by modifying pro-apoptotic p53 and Bax and anti-apoptotic Bcl-2 protein levels in MCF-7 human estrogen receptor-positive breast adenocarcinoma cells; this signaling pathway was similar to that affected by other organotin(IV) derivatives.
Organotin(IV) Compounds Containing (2E)-3-(3-Nitrophenyl)prop-2-Enoic Acid as Ligands
In a previous study, conducted by Liang et al. (2014), two novel organotin(IV) carboxylates of (2E)-3-(3-nitrophenyl)prop-2-enoic acid, compounds 33 and 34 (Scheme 7), showed concentration-dependent antitumor effects in HeLa cervical adenocarcinoma cells (IC50 values of 1.76 and 6.6 μM, respectively). The structures of the compounds included bridging of the carboxylate ligand of {[Ph3SnL]·0.5C6H6}n (33), which generated one-dimensional polymeric chain structures around the five-coordinated tin centers. In contrast, [Bu2LSnOSnLBu2]2 (34) was characterized by a central rhombus Sn2O2 unit with two additional tin atoms linked at the O atoms. Overall, the findings showed that the triorganotin(IV) compound (33) showed higher antitumor activity than the diorganotin(IV) compound (34).
Organotin(IV) Compounds Containing 3-Methoxysalicylaldehyde Thiosemicarbazone as Ligands
Compounds with 3-methoxysalicylaldehyde thiosemicarbazone (H2mstsc) were evaluated to determine their antitumor activity against Jurkat E6.1 acute lymphoblastic leukemia cells (Khandani et al., 2013). Three novel diorganotin(IV) compounds, namely Ph2Sn(mstsc) (35), Me2Sn(mstsc) (36), and Bu2Sn(mstsc) (37), were synthesized and characterized. Compound 36 and 37 adopted metal coordination geometry, including a distorted square pyramid, and the crystal lattices were found to be stabilized by intermolecular hydrogen bonds. The activities of the compounds decreased in the order of 37 > 35 > 36; that is, the dibutyltin(IV) compound showed higher antitumor activity than the other two compounds. However, all compounds showed high toxicity, with IC50 values of less than 1 μM.
Overall, organotin(IV) constituents play a crucial part in inducing cytotoxicity, with the ligands being involved in transporting and addressing the molecule to the target while avoiding unwanted changes within the biomolecules (Alama et al., 2009). Many organotin(IV) derivatives were synthesized and characterized according to their respective ligands, including oxides, thiolates, carboxylates, halides, fluorides, and hydroxides (Gielen, 2003; Hadi et al., 2019b), some of which were mentioned previously (Antiproliferative Activities of Organotin(IV) Compounds). These compounds were then evaluated concerning their antiproliferative activity toward various cancer cell lines. The compounds with halides ligands (Fickova et al., 2015) and oxygen-donor ligands (Attanzio et al., 2020) revealed lower IC50 values, up to the submicromolar range. Moreover, most compounds can be classified as highly toxic since their IC50 values were lower than 7.34 μM, as mentioned by How et al. (2008). Noteworthy, the various antiproliferative activities of the compounds are mainly influenced by their structure. When the electron withdrawing group(s) are attached to the tin atom, they tend to reduce the electron density in the compound; thus, the changing in the alkyl or aryl substituent in an organotin(IV) compound may contribute to a significant effect on its biological activity (Pellerito and Nagy, 2002; Iqbal et al., 2016). Moreover, the three main factors that are the nature of the organic R group, of the halide or pseudohalide X and of the donor ligand L, play a vital role in the structure and activity of organotin(IV) derivatives (L)xRnSnX4-n (Hadi et al., 2019b). In addition, the number of the alkyl substituent and its length in the organotin(IV) moiety may also contribute to the desirable cytotoxicity on various cell lines, among which the toxicity will become lesser with longer alkyl chains (Van der Kerk and Luijten, 1954; Van der Kerk and Luijten, 1956; Kamaludin et al., 2013; Hadi et al., 2019a). For example, the enzyme inhibition activity of the five-coordinated organotin(IV) carboxylates is higher than that of six-coordinated compounds (Hadi et al., 2019a), suggesting that the activity of the organotin(IV) compound can be effectively modulated by selected ligand, thereby potentially overcoming the compound limitations (Pellerito et al., 2006).
Molecular Mechanisms Underlying Organotin(IV) Compounds
The antitumor activities of organotin(IV) compounds have been extensively studied (Shaheen et al., 2017), and most previous studies have been primarily focused on synthesizing, characterizing, and screening the antitumor activities of these organotin(IV) compounds. However, the mechanism of toxicity has not been clarified for this class of metal-based drugs, particularly in in vivo studies. Therefore, information on the mechanism of action has mainly been obtained in studies of cancer cell lines. For example, Barbieri et al. (2001) showed that tin(IV) compounds induce cell death via stimulation of apoptosis, thereby resulting in antitumor toxicity. Consistent with this, we have found that most organotin(IV) compounds induce cell death via apoptosis, specifically the intrinsic mitochondrial pathway, regardless of the donor-ligand.
Some previous researchers have evaluated the molecular mechanisms underlying the cytotoxicity of organotin(IV) compounds, demonstrating the involvement of cell death via apoptosis (Ge et al., 2013; Girasolo et al., 2017; Kamaludin et al., 2019; Attanzio et al., 2020), and cell cycle arrest (Yunlan et al., 2014; Asanagi et al., 2016; Attanzio et al., 2020). Organotin compounds were said to induce apoptotic cell death by binding to DNA at external phosphate groups subsequently, altering phospholipid intracellular metabolism (Attanzio et al., 2020). Figure 1 shows a schematic representation of the potential mechanisms of organotin(IV)-induced apoptosis and cell cycle arrest in cancer cells. Moreover, we showed that the mechanisms of organotin(IV) compounds (regardless of the type of their donor-ligand) are similar to those of cisplatin, suggesting that organotin(IV) compounds may have potential applications as metal-based anticancer drugs.
The two main cell death mechanisms are apoptosis and necrosis; which mechanism is induced depends on the specific stimulus encountered and the amount of cellular energy available (Bjelaković et al., 2005). Cell death via apoptosis requires more energy via necrosis and involves the cleavage of cellular proteolytic substrates during intermediate stages. Activation of cysteine-aspartic proteases (caspases) leads to cell death via induction of DNA breaks and disruption of the adhesion of cytoskeletal proteins. Moreover, in apoptosis, there is no occurrence of enzyme release into the extracellular environment, thereby preventing damage to neighboring cells and avoiding stimulation of the immune response (Savill, 1997). Necrosis is a type of uncontrolled cell death typically triggered by external damage, such as hypoxia and inflammation. Hence, the cytoplasmic contents of the cell may be released into the surrounding tissues, resulting in widespread damage (Elmore, 2007; D’Arcy, 2019). Table 2 shows the differences between apoptosis and necrosis (Kerr et al., 1972; Majno and Joris, 1995; Trump et al., 1997; Elmore, 2007; Panawala, 2017; D’Arcy, 2019). In apoptosis, inflammation may not occur owing to lack of release of cellular contents into the surrounding microenvironment, the immediate phagocytosis of apoptotic cells prior to the formation of secondary necrosis, and lack of dissemination of anti-inflammatory cytokines by engulfing cells (Savill and Fadok, 2000; Kurosaka et al., 2003).
Hereby, the mechanism of action of organotin(IV) compound may involve triggering of the intrinsic apoptosis pathway, leading to the generation of DNA damage, oxidative stress, and subsequently, the activation of p53 (Ge et al., 2013; Fickova et al., 2015; Girasolo et al., 2017; Attanzio et al., 2020). Most cytotoxic drugs target DNA (Tabassum and Pettinari, 2006; Yusof et al., 2019). Figure 2 shows three types of reversible DNA binders (Silverman and Holladay, 2015). Previous mechanistic studies have shown that organotin(IV) compounds can interact with DNA as intercalative binding-type or groove binding-type drugs depending on the coordination number of the attachment and the nature of the groups attached to the central tin atom (Tabassum and Pettinari 2006; Rehman et al., 2016; Sirajuddin et al., 2016; Liu et al., 2017; Yusof et al., 2019). The ability of these compounds to interact with DNA (binding or cleaving) is thought to arise from the biological potency of the compounds (Yusof et al., 2019).
Oxidative stress can also be described as the disruption of the pro-oxidant/antioxidant balance in cells, resulting in substantial oxidative damage and reduced cell viability (Sinha et al., 2013). Accumulation of reactive oxygen species (ROS) following induction of oxidative stress results in oxidative damage to essential biomolecules, such as proteins, lipids, and nucleic acids (Bilinski et al., 1989; Yakes and VanHouten, 1997; Cabiscol et al., 2000; Sinha et al., 2013). The three main types of ROS include hydrogen peroxide (H2O2), hydroxyl radical (·OH), and superoxide anion (O2·-) (Mate’s et al., 2012). The formation of H2O2 by O2·- or by the action of oxidase enzymes can lead to damage. Moreover, leakage in the mitochondrial respiratory chain may cause the emergence of O2·-. The most damaging type of ROS is ·OH, which can alter DNA bases and induce DNA strand breaks, eventually resulting in DNA damage (Mate’s et al., 2010; Mate’s et al., 2012). Mitochondria are the sites of ROS accumulation (mainly O2· and ·OH) because most ROS generation occurs in the mitochondria. This may cause deleterious effects, including oxidative impairment of mitochondrial DNA and subsequent cell death via apoptosis (Orrenius et al., 2007; Ricci et al., 2008; Circu et al., 2009; Rachek et al., 2009; Sinha et al., 2013) as demonstrated by a treatment with organotin(IV) compounds.
The organotin(IV) compounds were reported to cause disruption in mitochondrial membrane following the activation of p53 that induces BH3 pro-apoptotic proteins, such as BID and BIM, which are translocated to the mitochondria (Riedl and Shi, 2004; Attanzio et al., 2020). The molecular mechanism that involves in the activation of p53 resulted in targeted cytotoxic effects via apoptosis or cell cycle arrest. p53 is a major tumor-suppressor gene that regulates various biological activities, including cell cycle arrest, cellular aging, DNA repair, and apoptosis (Kastenhuber and Lowe, 2017). Additionally, via its function as a transcription factor, p53 forms homotetramers that can activate almost 500 target genes and regulate apoptosis signaling (Riley et al., 2008; Aubrey et al., 2018).
The loss in mitochondrial membrane of the cancer cells induced by organotin(IV) compounds causing the release of mitochondrial cytochrome c into the cytosol that is regulated by Bcl-2 family proteins (e.g., Bax and Bak). This facilitates the formation of the apoptosome complex (composed of cytochrome c, the apoptotic molecule activating factor protease 1, and pro-caspase-9). This complex then induces caspase-9 activation via cleavage, followed by activation of the effector caspase, caspase-3, which executes the final steps of apoptosis (Green and Kroemer, 2004; Mohamad et al., 2005; Er et al., 2006; Giam et al., 2008; Ge et al., 2013). Caspases are defined as synthesized as zymogens and are then converted from precursors to mature proteases. Initiator caspases are activated by the oligomerization autoprocess; effector caspases are activated by other proteases, including the initiator caspase and granzyme B (Li et al., 1997; Muzio et al., 1997; Butt et al., 1998; Martin et al., 1998; Srinivasula et al., 1998; Yang et al., 1998). In addition, there are two types of overlapping signaling pathways, that is, the intrinsic and extrinsic pathways, which promote apoptosis. The type of pathway activated depends on the expression of specific initiator caspases. The intrinsic pathway is generally activated by mitochondrial dysfunction, oxidative stress, and pro-apoptotic factor expression, whereas the extrinsic pathway is activated by ligand attachment on cell death receptors (Bai and Odin, 2003). Hence, this explained that organotin(IV) compounds indeed induce apoptotic cell death via intrinsic mitochondrial pathway. Apart from that, according to Ge et al. (2013), the mitogen-activated protein kinases JNK and p38MAPK are also responsive to various types of stress stimuli and participate in the induction of apoptosis by organotin(IV) compounds via modulation of phosphorylation.
In addition to modulation of apoptosis, cancer cells also exhibit loss of cell cycle control, resulting in dysregulated cell division and proliferation (Senese et al., 2014; Kartal-Yandim et al., 2015) and lack of cell cycle arrest upon induction of cellular stress. The cell cycle checkpoint can prevent DNA damage in cells in response to chemotherapeutic drugs (Sancar et al., 2004), giving the cells a sufficient amount of time to recover. In contrast, carcinogenesis can cause disruption of all cell cycle checkpoints. However, Linke et al. (1996) showed that treatment with chemotherapeutic drugs and gamma radiation may trigger DNA damage, resulting in cell cycle arrest at G0/G1 phase. This is aligned with the previous studies that described the involvement of the p53/p21WAF1 signaling pathway in cell cycle arrest induced by organotin(IV) compounds.
The occurrence of cell cycle arrest at different phases is modulated by p53-dependent transcriptional activation of p21WAF1, which binds to and regulates several cyclins (Abbas and Dutta, 2009; Chen, 2016). During G1 phase, cells enter the cell cycle and prepare themselves for DNA duplication in S phase. Subsequently, in G2 phase, DNA damage is repaired before cells enter M (mitosis) phase, during which chromatids and daughter cells separate (Bible and Kaufmann, 1997; Carlson et al., 1999; Jackson et al., 2000; Senderowicz and Sausville, 2000; Hirose et al., 2001; Sausville et al., 2001; Shapiro et al., 2001). The cells then enter a quiescent (inactive) stage. Disruption of G2 phase may allow the cells to preserve their viability following induction by drugs that affect the main checkpoint kinase, Chk1 (Hapke et al., 2001; Xiao et al., 2003). Indeed, cell cycle checkpoints are considered strong drug targets because of their ability to control the cell cycle. Hence, researchers in the field of anticancer drugs often evaluate the effects of drugs on the cell cycle in cancer cells. Organotin(IV) compounds have been shown to cause G2/M phase arrest in several cancer cell lines (Yunlan et al., 2014; Asanagi et al., 2016; Attanzio et al., 2020) and G0/G1 phase arrest in other cancer cell lines (Attanzio et al., 2020) as shown in Figure 3. The arrest of proliferation at different cell cycle phases highlights the important properties of organotin(IV) compounds and ligand moieties in modulating biological activity.
As described above, most studies of organotin(IV) compounds have been performed in cancer cell lines. However, Verginadis et al. (2011) and Barbieri et al. (2000) found that organo-based compounds could also show antitumor activity in tumor-bearing animals. Indeed, triorganotin(IV) compounds show potent antitumor activities and prolong survival time in tumor-bearing animals (Barbieri et al., 2000).
Concluding Remarks
In this review, we discussed the strong cytotoxic effects of organotin(IV) compounds. Most organotin(IV) compounds have been shown to activate apoptosis via the intrinsic mitochondrial apoptosis pathway, suggesting potential applications in anticancer chemotherapy (Cima and Ballarin, 1999; Pellerito et al., 2005). The ideal characteristics of anticancer drugs include the ability to induce apoptotic cell death (Yamaguchi et al., 2007; Husaini et al., 2017) and selectivity toward cancerous cells. Thus, organotin(IV) compounds show high potential for use as chemotherapeutic metallopharmaceuticals based on their excellent cytotoxic characteristics. However, more studies of the mechanisms of action of these compounds both in vitro and in vivo are required in order to elucidate the exact mechanisms through which cell death is induced.
Author Contributions
SA and NK conceived the presented idea and worked on the manuscript. SA wrote the paper. All authors provided critical feedback and helped shape the context of the paper.
Funding
This study was financially supported by the Malaysian Ministry of Higher Education (FRGS/1/2019/STG04/UKM/03/3).
Conflict of Interest
The authors declare that the research was conducted in the absence of any commercial or financial relationships that could be construed as a potential conflict of interest.
Publisher’s Note
All claims expressed in this article are solely those of the authors and do not necessarily represent those of their affiliated organizations, or those of the publisher, the editors and the reviewers. Any product that may be evaluated in this article, or claim that may be made by its manufacturer, is not guaranteed or endorsed by the publisher.
Acknowledgments
We would like to thank the Malaysian Ministry of Higher Education for the financial support (FRGS/1/2019/STG04/UKM/03/3). We also acknowledge the Faculty of Health Sciences, Universiti Kebangsaan Malaysia, for providing essential facilities which enabled this study to take place.
References
Abbas, T., and Dutta, A. (2009). p21 in Cancer: Intricate Networks and Multiple Activities. Nat. Rev. Cancer 9, 400–414. doi:10.1038/nrc2657
Adeyemi, J., and Onwudiwe, D. (2018). Organotin(IV) Dithiocarbamate Complexes: Chemistry and Biological Activity. Molecules 23, 2571–2598. doi:10.3390/molecules23102571
Adeyemi, J. O., Onwudiwe, D. C., Ekennia, A. C., Anokwuru, C. P., Nundkumar, N., Singh, M., et al. (2019). Synthesis, Characterization and Biological Activities of Organotin(IV) Diallyldithiocarbamate Complexes. Inorg. Chim. Acta 485: 64–72. doi:10.1016/j.ica.2018.09.085
Alama, A., Tasso, B., Novelli, F., and Sparatore, F. (2009). Organometallic Compounds in Oncology:implications of Novel Organotins as Antitumor Agents. Drug Discov. 14, 500–508. doi:10.1016/j.drudis.2009.02.002
Arafat, Y., Ali, S., Shahzadi, S., and Shahid, M. (2013). Preparation, Characterization, and Antimicrobial Activities of Bimetallic Complexes of Sarcosine with Zn(II) and Sn(IV). Bioinorg. Chem. App. 2013, 351262. doi:10.1155/2013/351262
Asanagi, M., Yamada, S., Hirata, N., Itagaki, H., Kotake, Y., Sekino, Y., et al. (2016). Tributyltin Induces G2/M Cell Cycle Arrest via NAD(+)-dependent Isocitrate Dehydrogenase in Human Embryonic Carcinoma Cells. J. Toxicol. Sci. 41 (2), 207–215. doi:10.2131/jts.41.207
Attanzio, A., D’Agostino, S., Busà, R., Frazzitta, A., Rubino, S., Girasolo, M. A., et al. (2020). Cytotoxic Activity of Organotin(IV) Derivatives with Triazolopyrimidine Containing Exocyclic Oxygen Atoms. Molecules 25 (859), 1–16. doi:10.3390/molecules25040859
Aubrey, B. J., Kelly, G. L., Janic, A., Herold, M. J., and Strasser, A. (2018). How Does P53 Induce Apoptosis and How Does This Relate to P53-Mediated Tumour Suppression?. Cell Death Differ 25, 104–113. doi:10.1038/cdd.2017.169
Awang, N., Aziz, Z. A., Kamaludin, N. F., and Chan, K. M. (2014). Cytotoxicity and Mode of Cell Death Induced by Triphenyltin (IV) Compounds In Vitro. Online J. Biol. Sci. 14 (2), 84–93. doi:10.3844/ojbssp.2014.84.9310.3844/ojbsci.2014.84.93
Awang, N., Baba, I., Yousof, N. S. A. M., and Kamaludin, N. F. (2010). Synthesis and Characterization of Organotin(IV) N-Benzyl-N-Isopropyldithiocarbamate Compounds: Cytotoxic Assay on Human Hepatocarcinoma Cells (HepG2). Am. J. Appl. Sci. 7 (8), 1047–1052. doi:10.3844/ajassp.2010.1047.1052
Awang, N., Kamaludin, N. F., Baba, I., Chan, K. M., Rajab, N. F., and Hamid, A. (2016). Synthesis, Characterization and Antitumor Activity of New Organotin(IV) Methoxyethyldithiocarbamate Complexes. Orient. J. Chem. 32 (1), 101–107. doi:10.13005/ojc/320110
Awang, N., Kamaludin, N. F., and Ghazali, A. R. (2011). Cytotoxic Effect of Organotin(IV) Benzylosopropyldithiocarbamate on Chang Liver Cell and Hepatocarcinoma hepG2 Cell. Pak J. Biol. Sci. 14 (15), 786–774. doi:10.3923/pjbs.2011.768.774
Bai, J., and Odin, J. A. (2003). Apoptosis and the Liver: Relation to Autoimmunity and Related Conditions. Autoimmun. Rev. 2, 36–42. doi:10.1016/s1568-9972(02)00125-8
Barbieri, F., Sparatore, F., Cagnoli, M., Bruzzo, C., Novelli, F., and Alama, A. (2001). Antiproliferative Activity and Interactions with Cell-Cycle Related Proteins of the Organotin Compound Triethyltin(IV) Lupinylsulfide Hydrochloride. Chem. Biol. Interact. 134, 27–39. doi:10.1016/s0009-2797(00)00249-0
Barbieri, F., Viale, M., Sparatore, F., Favre, A., Cagnoli, M., Bruzzo, C., et al. (2000). Cytotoxicity In Vitro and Preliminary Antitumor Activity In Vivo of a Novel Organotin Compound. Anticancer Res. 20, 977–980.
Bible, K. C., and Kaufmann, S. H. (1997). Cytotoxic Synergy between Flavopiridol and Various Antineoplastic Agents: the Importance of Sequence of Administration. Cancer Res. 57, 3375–3380.
Bilinski, T., Litwinska, J., Blaszczynski, M., and Bajus, A. (1989). Superoxide Dismutase Deficiency and the Toxicity of the Products of Autooxidation of Polyunsaturated Fatty Acids in Yeast. Biochim. Biophys. Acta 1001, 102–106. doi:10.1016/0005-2760(89)90312-3
Biplob, K., Baul, T. S. B., and Chatterjee, A. (2008). P53-dependent Antiproliferative and Anticancer Effect of Novel Alkyl Series of Diorganotin (IV) Compounds. Invest. New Drugs 27, 319–326. doi:10.1007/s10637-008-9176-6
Bjelaković, G., Nagorni, A., Bjelaković, M., Stamenković, I., Arsić1, R., and Katić, V. (2005). Apoptosis: Programmed Cell Death and its Clinical Implications. FU Med. Biol. 12 (1), 6–11.
Brtko, J., and Dvorak, Z. (2011). Role of Retinoids, Rexinoids and Thyroid Hormone in the Expression of Cytochrome P450 Enzymes. Curr. Drug Metab. 12, 71–88. doi:10.2174/138920011795016881
Brtko, J. (2007). Retinoids, Rexinoids and Their Cognate Nuclear Receptors: Character and Their Role in Chemoprevention of Selected Malignant Diseases. Biomed. Pap. Med. Fac. Univ. Palacky Olomouc Czech Repub 151, 187–194. doi:10.5507/bp.2007.033
Brtko, J., and Thalhamer, J. (2003). Renaissance of the Biologically Active Vitamin A Derivatives: Established and Novel Directed Therapies for Cancer and Chemoprevention. Curr. Pharm. Des. 9, 2067–2077. doi:10.2174/1381612033454144
Butt, A. J., Harvey, N. L., Parasivam, G., and Kumar, S. (1998). Dimerization and Autoprocessing of the Nedd2 (Caspase-2) Precursor Requires Both the Prodomain and the Carboxyl-Terminal Regions. J. Biol. Chem. 273, 6763–6768. doi:10.1074/jbc.273.12.6763
Cabiscol, E., Piulats, E., Echave, P., Herrero, E., and Ros, J. (2000). Oxidative Stress Promotes Specific Protein Damage in Saccharomyces cerevisiae. J. Biol. Chem. 35, 27393–27398. doi:10.1074/jbc.M003140200
Carlson, B., Lahusen, T., Singh, S., Loaiza-Perez, A., Worland, P. J., Pestell, R., et al. (1999). Down-regulation of Cyclin D1 by Transcriptional Repression in MCF-7 Human Breast Cancer Cells Induced by Flavopiridol. Cancer Res. 59, 4634–4641.
Chandrasekhar, V., Gopal, K., Sasikumar, P., and Thirumoorthi, R. (2005). Organooxotin Assemblies from SnC Bond Cleavage Reactions. Coord. Chem. Rev. 249, 1745–1765. doi:10.1016/j.ccr.2005.03.028
Chandrasekhar, V., Nagendran, S., and Baskar, V. (2002). Organotin Assemblies Containing Sn-O Bonds. Coord. Chem. Rev. 235, 1–52. doi:10.1016/S0010-8545(02)00178-9
Chen, J. (2016). The Cell-Cycle Arrest and Apoptotic Functions of P53 in Tumor Initiation and Progression. Cold Spring Harb Perspect. Med. 6, 1–15. doi:10.1101/cshperspect.a026104
Cima, F., and Ballarin, L. (1999). TBT‐induced Apoptosis in Tunicate Haemocytes. Appl. Organomet. Chem. 13 (10), 697–703. doi:10.1002/(SICI)1099-0739(199910)13:10<697::AID-AOC916>3.0.CO;2-%23
Circu, M. L., Moyer, M. P., Harrison, L., and Aw, T. Y. (2009). Contribution of Glutathione Status to Oxidant-Induced Mitochondrial DNA Damage in Colonic Epithelial Cells. Free Radic. Biol. Med. 47, 1190–1198. doi:10.1016/j.freeradbiomed.2009.07.032
D’Arcy, M. S. (2019). Cell Death: a Review of the Major Forms of Apoptosis, Necrosis and Autophagy. Cell Biol Int 43, 582–592. doi:10.1002/cbin.11137
Deo, K. M., Ang, D. L., McGhie, B., Rajamanickam, A., Dhiman, A., Khoury, A., et al. (2018). Platinum Coordination Compounds with Potent Anticancer Activity. Coord. Chem. Rev. 375, 148–163. doi:10.1016/j.ccr.2017.11.014
Devendra, R., Edmonds, N. R., and Söhnel, T. (2013). Computational and Experimental Investigations of the Urethane Formation Mechanism in the Presence of Organotin(IV) Carboxylate Catalysts. J. Mol. Catal. A. Chem. 366: 126–139. doi:10.1016/j.molcata.2012.09.015
Devi, J., Yadav, J., Kumar, D., Jindal, D. K., and Basu, B. (2020). Synthesis, Spectral Analysis and In Vitro Cytotoxicity of Diorganotin (IV) Complexes Derived from Indole-3-Butyric Hydrazide. Appl. Organomet. Chem. 1–17. doi:10.1002/aoc.5815
Elmore, S. (2007). Apoptosis: a Review of Programmed Cell Death. Toxicol. Pathol. 35 (4), 495–516. doi:10.1080/01926230701320337
Er, E., Oliver, L., Cartron, P.-F., Juin, P., Manon, S., and Vallette, F. M. (2006). Mitochondria as the Target of the Pro-apoptotic Protein Bax. Biochim. Biophys. Acta 1757, 1301–1311. doi:10.1016/j.bbabio.2006.05.032
Fickova, M., Macho, L., and Brtko, J. (2015). A Comparison of the Effects of Tributyltin Chloride and Triphenyltin Chloride on Cell Proliferation, Proapoptotic P53, Bax, and Antiapoptotic Bcl-2 Protein Levels in Human Breast Cancer MCF-7 Cell Line. Toxicol. Vitro 29, 727–731. doi:10.1016/j.tiv.2015.02.007
Florea, A.-M., and Busselberg, D. (2011). Cisplatin as an Anti-tumor Drug: Cellular Mechanisms of Activity, Drug Resistance andiInduced Side Effects. Cancers 3, 1351–1371. doi:10.3390/cancers3011351
Ge, R., Maa, W. H., Li, Y. L., and Li, Q. S. (2013). Apoptosis Induced Neurotoxicity of Di-n-butyl-di-(4 Chlorobenzohydroxamato). Toxicol. Vitro 27, 92–102. doi:10.1016/j.tiv.2012.08.009
Ghazi, D., Rasheed, Z., and Yousif, E. (2018). Review of Organotin Compounds: Chemistry and Applications. IJREI 2 (4), 340–348.
Giam, M., Huang, D. C., and Bouillet, P. (2008). BH3-only Proteins and Their Roles in Programmed Cell Death. Oncogene 27, 128–136. doi:10.1038/onc.2009.50
Gielen, M. (2003). An Overview of Forty Years Organotin Chemistry Developed at the Free Universities of Brussels ULB and VUB. J. Braz. Chem. Soc. 14, 870–877. doi:10.1590/S0103-50532003000600003
Gielen, M., Dalil, H., Biesemans, M., Mahieu, B., de Vos, D., and Willem, R. (1999). Di and Tri-organotin Derivatives of 3S,4S–3-[(R)-1-(tert-butyldimethylsilyloxy) ethyl]-4-[(R)-1-carboxyethyl]-2-azetidinone: Synthesis, Characterization and In Vitro Antitumour Activity. Appl. Organomet. Chem. 13, 515–520. doi:10.1002/(sici)1099-0739(199907)13:7<515::aid-aoc875>3.0.co;2-b
Gielen, M., and Tiekink, E. R. T. (2005). “Tin Compounds and Their Therapeutic Potential,” in Metallotherapeutic Drugs and Metal-Based Diagnostic Agents, the Use of Metals in Medicine. West Sussex, United Kingdom: J. Wiley & Sons, 421–439. doi:10.1002/0470864052
Girasolo, M. A., Tesoriere, L., Casella, G., Attanzio, A., Capobianco, M. L., Sabatino, P., et al. (2017). A Novel Compound of Triphenyltin(IV) with N-Tert-Butoxycarbonyl-Lornithine Causes Cancer Cell Death by Inducing a P53-dependent Activation of the Mitochondrial Pathway of Apoptosis. Inorg. Chim. Acta 456, 1–8. doi:10.1016/j.ica.2016.11.012
Girasolo, M. A., Di Salvo, C., Schillaci, D., Barone, G., Silvestri, A., and Ruisi, G. (2005). Synthesis, Characterization, and In Vitro Antimicrobial Activity of Organotin(IV) Complexes with Triazolo-Pyrimidine Ligands Containing Exocyclic Oxygen Atoms. J. Organomet. Chem. 690, 4773–4783. doi:10.1016/j.jorganchem.2005.07.072
Green, D. R., and Kroemer, G. (2004). The Pathophysiology of Mitochondrial Cell Death. J. Sci. 305, 626–629. doi:10.1126/science.1099320
Hadi, A. G., Jawad, K., Ahmed, D. S., and Yousif, E. (2019a). Synthesis and Biological Activities of Organotin (IV) Carboxylates: A Review. Sys. Rev. Pharm. 10 (1), 26–31. doi:10.5530/srp.2019.1.5
Hadi, A. G., Jawad, K., and Yousif, E. (2019b). Medicinal Applications of Organotin (IV) Complexes and its Important Place as Effective Antitumor Agents. Indian J. Public Health Res. Dev. 10 (6), 1171–1177. doi:10.5958/0976-5506.2019.01449.9
Hadjikakou, S. K., and Hadjiliadis, N. (2009). Antiproliferative and Anti-tumor Activity of Organotin Compounds. Coord. Chem. Rev. 253, 235–249. doi:10.1016/j.ccr.2007.12.026
Hapke, G., Yin, M. B., and Rustum, Y. M. (2001). Targeting Molecular Signals in Chk1 Pathways as a New Approach for Overcoming Drug Resistance. Cancer Metastasis Rev. 20, 109–115. doi:10.1023/a:1013116826788
Haque, R. A., Salam, S. A., and Arafath, M. A. (2015). New Organotin(IV) Complexes with N(4)-methylthiosemicarbazone Derivatives Prepared from 2,3-dihydroxybenzaldehyde and 2-Hydroxy-5-Methylbenzaldehyde: Synthesis, Characterization, and Cytotoxic Activity. J. Coord. Chem. 68 (16), 2953–2967. doi:10.1080/00958972.2015.1057133
Hirose, Y., Berger, M. S., and Pieper, R. O. (2001). Abrogation of the Chk1 Mediated G2 Checkpoint Pathway Potentiates Temozolomide-Induced Toxicity in a P53-independent Manner in Human Glioblastoma Cells. Cancer Res. 61, 5843–5849.
Holmes, R. R., Swamy, K. C. K., Schmid, C. G., and Day, R. O. (1988). Organotin Clusters. 4. Cubic, Butterfly, and Oxygen-Capped Clusters of N-Butyloxotin Phosphinates. A New Class of Organotin Compounds. J. Am. Chem. Soc. 110, 7060–7066. doi:10.1021/ja00229a019
How, F. N. F. K. A., Crouse, M. I. M., Tahir, M. T. H., Tarafder, , and Cowley, A. R. (2008). Synthesis, Characterization and Biological Studiesof S-Benzyl-&beta-N-(-Benzoyl) Dithiocarbazate and its Metal Complexes. Polyhedron 27, 3325–3329. doi:10.1016/j.poly.2008.07.022
Husaini, R., Ahmad, M., and Zakaria, Z. (2017). Effectiveness of Imatinib Mesylate over Etoposide in the Treatment of Sensitive and Resistant Chronic Myeloid Leukaemia Cells In Vitro. Exp. Ther. Med. 13, 3209–3216. doi:10.3892/etm.2017.4443
Iqbal, H., Ali, S., and Shahzadi, S. (2016). Anti-inflammatory and Acute Toxicity Study of Organotin (IV) Comp-Lexes: a Review. Chem. J. 6, 59–73.
Iqbal, H., Ali, S., and Shahzadi, S. (2015). Antituberculosis Study of Organotin(IV) Complexes: A Review. Cogent Chem. 1, 1029039. doi:10.1080/23312009.2015.1029039
Jackson, J. R., Gilmartin, A., Imburgia, C., Winkler, J. D., Marshall, L. A., and Roshak, A. (2000). An Indolocarbazole Inhibitor of Human Checkpoint Kinase (Chk1) Abrogates Cell-Cycle Arrest Caused by DNA Damage. Cancer Res. 60, 566–572.
Jain, M., Gaur, S., Singh, V. P., and Singh, R. V. (2004). Organosilicon (IV) and Organotin (IV) Complexes as Biocides and Nematicides: Synthetic, Spectroscopic and Biological Studies of N\N Donor Sulfonamide Imine and its Chelates. Main Gr. Met. Compd. 18, 73–82. doi:10.1002/aoc.573
Jan, C.-R., Jiann, B.-P., Lu, Y.-C., Chang, H.-T., Su, W., Chen, W.-C., et al. (2002). Effect of the Organotin Compound Triethyltin on Ca2+ Handling in Human Prostate Cancer Cells. Life Sci. 70 (11), 1337–1345. doi:10.1016/s0024-3205(01)01500-4
Javed, F., Sirajuddin, M., Ali, S., Khalid, N., Tahir, M. N., Shah, N. A., et al. (2016). Organotin(IV) Derivatives of O-Isobutyl Carbonodithioate: Synthesis, Spectroscopic Characterization, X-ray Structure, HOMO/LUMO and In Vitro Biological Activities. Polyhedron 104, 80–90. doi:10.1016/j.poly.2015.11.041
Kadu, R., Roy, H., and Singh, V. K. (2015). Diphenyltin(IV) Dithiocarbamate Macrocyclic Scaffolds as Potent Apoptosis Inducers for Human Cancer HEP 3B and IMR 32 Cells: Synthesis, Spectral Characterization, Density Functional Theory Study and In Vitro Cytotoxicity. Appl. Organomet. Chem. 29 (11), 746–755. doi:10.1002/aoc.3362
Kamaludin, N. F., Awang, A., Baba, I., Hamid, A., and Meng, C. K. (2013). Synthesis, Characterization and crystal Structure of Organotin(IV) N-Butyl-N-Phenyldithiocarbamate Compounds and Their Cytotoxicity in Human Leukemia Cell Lines. Pak. J. Biol. Sci. 16, 12–21. doi:10.3923/pjbs.2013.12.21
Kamaludin, N. F., Ismail, N., Awang, N., Mohamad, R., and Pim, N. U. (2019). Cytotoxicity Evaluation and the Mode of Cell Death of K562 Cells Induced by Organotin (IV) (2-methoxyethyl) Methyldithiocarbamate Compounds. J. Appl. Pharm. Sci. 9 (6), 10–15. doi:10.7324/JAPS.2019.90602
Kartal-Yandim, M., Adan-Gokbulut, A., and Baran, Y. (2015). Molecular Mechanisms of Drug Resistance and its Reversal in Cancer. Crit. Rev. Biotechnol. 36 (11), 1–11. doi:10.3109/07388551.2015.10159510.3109/07388551.2015.1015957
Kastenhuber, E. R., and Lowe, S. W. (2017). Putting P53 in Context. Cell 170 (6), 1062–1078. doi:10.1016/j.cell.2017.08.028
Kerr, J. F., Wyllie, A. H., and Currie, A. R. (1972). Apoptosis: a Basic Biological Phenomenon with Wide-Ranging Implications in Tissue Kinetics. Br. J. Cancer 26, 239–257. doi:10.1038/bjc.1972.33
Khan, A., Parveen, S., Khalid, A., and Shafi, S. (2020). Recent Advancements in the Anticancer Potentials of Phenylorganotin(IV) Complexes. Inorg. Chim. Acta 505, 1–43. doi:10.1016/j.ica.2020.119464
Khandani, M., Sedaghat, T., Erfani, N., Haghshenas, M. R., and Khavasi, H. R. (2013). Synthesis, Spectroscopic Characterization, Structural Studies and Antibacterial and Antitumor Activities of Diorganotin Complexes with 3-methoxysalicylaldehyde Thiosemicarbazone. J. Mol. Struct. 1037, 136–143. doi:10.1016/j.molstruc.2012.12.061
Kurosaka, K., Takahashi, M., Watanabe, N., and Kobayashi, Y. (2003). Silent Cleanup of Very Early Apoptotic Cells by Macrophages. J. Immunol. 171, 4672–4679. doi:10.4049/jimmunol.171.9.4672
le Maire, A., Grimaldi, M., Roecklin, D., Dagnino, S., Vivat-Hannah, V., Balaguer, P., et al. (2009). Activation of RXR-PPAR Heterodimers by Organotin Environmental Endocrine Disruptors. EMBO Rep. 10, 367–373. doi:10.1038/embor.2009.8
Li, P., Nijhawan, D., Budihardjo, I., Srinivasula, S. M., Ahmad, M., Alnemri, E. S., et al. (1997). Cytochrome C and dATP Dependent Formation of Apaf-1/caspase-9 Complex Initiates an Apoptotic Protease cascade. Cell 91, 479–489. doi:10.1016/s0092-8674(00)80434-1
Liang, J., Du, D., Xiao, X., Hana, X., Zhu, D., and Mei, Z. (2014). A Series of Organotin(IV) Complexes Based on (E)-3-(3-nitrophenyl) Acrylic Acid: Syntheses, crystal Structures and Biological Activities. Inorg. Chem. Commun. 40, 133–137. doi:10.1016/j.inoche.2013.12.001
Linke, S. P., Clarkin, K. C., Leonardo, A. D., Tsou, A., and Wahl, G. M. (1996). A Reversible, P53-dependent G0/G1 Cell Cycle Arrest Induced by Ribonucleotide Depletion in the Absence of Detectable DNA Damage. Genes Dev. 10, 934–937. doi:10.1101/gad.10.8.934
Liu, K., Yan, H., Chang, G., Li, Z., Niu, M., and Hong, M. (2017). Organotin(IV) Complexes Derived from Hydrazone Schiff Base: Synthesis, crystal Structure, In Vitro Cytotoxicity and DNA/BSA Interactions. Inorg. Chim. Acta 464, 137–146. doi:10.1016/j.ica.2017.05.017
Majno, G., and Joris, I. (1995). Apoptosis, Oncosis, and Necrosis. An Overview of Cell Death. Am. J. Pathol. 146, 3–15.
Mamba, S. M., Mishra, A. K., Mamba, B. B., Njobeh, P. B., Dutton, M. K., and Fosso-Kankeuc, E. (2010). Spectral, thermal and In Vitro Antimicrobial Studies of Cyclohexylamine-N-Dithiocarbamate Transition Metal Complexes. Spectrochim. Acta A. Mol. Biomol. Spectrosc. 77 (3), 579–587. doi:10.1016/j.saa.2010.06.002
Martin, D. A., Siegel, R. M., Zheng, L., and Lenardo, M. J. (1998). Membrane Oligomerization and Cleavage Activates the Caspase-8 (FLICE/MACH-1) Death Signal. J. Biol. Chem. 273, 4345–4349. doi:10.1074/jbc.273.8.4345
Martins, L., Hazra, S., Silva, M., and Pombeiro, L. (2016). A Sulfonated Schiff Base Dimethyltin(IV) Coordination Polymer: Synthesis, Characterization and Application as a Catalyst for Ultrasound- or Microwave-Assisted Baeyer-Villiger Oxidation under Solvent-free Conditions. RSC Adv. 6, 78225–78233. doi:10.1039/c6ra14689a
Mate´s, J. A., Segura, F. J., Alonso, J. M., and Javier, M. (2012). Oxidative Stress in Apoptosis and Cancer: an Update. Arch. Toxicol. 86 (11), 1649–1665. doi:10.1007/s00204-012-0906-3
Mate´s, J. M., Segura, J. A., Alonso, F. J., and Marquez, J. (2010). Roles of Dioxins and Heavy Metals in Cancer and Neurological Diseases Using ROS-Mediated Mechanisms. Free Radic. Biol. Med. 49, 1328–1341. doi:10.1016/j.freeradbiomed.2010.07.028
Menezes, D. C., Vieiraa, F. T., de Lima, G. M., Porto, A. O., Cortés, M. E., Ardisson, J. D., et al. (2005). Tin(IV) Complexes of Pyrrolidinedithiocarbamate: Synthesis, Characterisation and Antifungal Activity. Eur. J. Med. Chem. 40 (12), 1277–1282. doi:10.1016/j.ejmech.2005.07.008
Mohamad, N., Gutiérrez, A., Núñez, M., Cocca, C., Martín, G., Cricco, G., et al. (2005). Mitochondrial Apoptotic Pathways. J. Biocell 29, 149–161. doi:10.32604/biocell.2005.29.149
Mohamad, R., Awang, N., Kamaludin, N. F., Jotani, M. M., and Tiekink, E. R. T. (2018). Crystal Structures and Hirshfeld Surface Analyses of Bis [ N,N-bis( 2-methoxyethyl) Dithiocarbamato- κ2 S,S′]di-n-Butyltin(IV) and [N-(2-methoxyethyl)-N-methyldithiocarbamato-κ2 S,S′]Triphenyltin(IV). Acta Crystallograph. Sect. E Crystallograph. Commun. 74, 302–308. doi:10.1107/S2056989018001901
Mohamad, R., Awang, N., and Kamaludin, N. F. (2016). Synthesis and Characterisation of New Organotin (IV) (2-Methoxyethyl)-Methyldithiocarbamate Complexes. Res. J. Pharm. Biol. Chem. Sci. 7, 1920–1925.
Muzio, M., Chinnaiyan, A. M., Kischkel, F. C., O'Rourke, K., Shevchenko, A., Ni, J., et al. (1997). FLICE, a Novel FADD-Homologous ICE/CED-3- Like-Protease, Is Recruited to Teh CD95 (Fas/APO-1) Death Inducing Signaling Complex. Cell 85, 817–827. doi:10.1016/S0092-8674(00)81266-0
Najeeb, D., Shalan, N., Ibraheem, H., Farina, Y., and Yousif, E. (2009). Synthesis and Fungicidal Activity of Some Diorganotin(IV) with Benzamidocysteine. Al-nahrain Univ. J. 12 (1), 24–28. doi:10.22401/JNUS.12.1.04
Nakanishi, T., Nishikawa, J., Hiromori, Y., Yokoyama, H., Koyanagi, M., Takasuga, S., et al. (2005). Trialkyltin Compounds Bind Retinoid X Receptor to Alter Human Placental Endocrine Functions. Mol. Endocrinol. 19, 2502–2516. doi:10.1210/me.2004-0397
Nath, M., and Saini, P. K. (2011). Chemistry and Applications of Organotin(IV) Complexes of Schiff Bases. Dalton Trans. 40, 7077–7121. doi:10.1039/c0dt01426e
Niu, Li., Li, Y., and Li, Q. (2014). Medicinal Properties of Organotin Compounds and Their Limitations Caused by Toxicity. Inorg. Chim. Acta 423, 2–31. doi:10.1016/j.ica.2014.05.007
Omae, I. (2003). Organotin Antifouling Paints and Their Alternatives. Appl. Organomet. Chem. 17, 81–105. doi:10.1002/aoc.396
Orrenius, S., Gogvadze, V., and Zhivotovsky, B. (2007). Mitochondrial Oxidative Stress: Implications for Cell Death. Annu. Rev. Pharmacol. Toxicol. 47, 143–183. doi:10.1146/annurev.pharmtox.47.120505.105122
Pellerito, C., Agati, P. D., Fiore, T., Mansueto, C., Mansueto, V., Stocco, G., et al. (2005). Synthesis, Structural Investigations on Organotin(IV) Chlorin-E6 Complexes, Their Effect on Sea Urchin Embryonic Development and Induced Apoptosis. J. Inorg. Biochem. 99 (6), 1294–1305. doi:10.1016/j.jinorgbio.2005.03.002
Pellerito, C., Nagy, L., Pellerito, L., and Szorcsik, A. (2006). Biological Activity Studies on organotin(IV)n+ Complexes and Parent Compounds. J. Organomet. Chem. 691 (8), 1733–1747. doi:10.1016/j.jorganchem.2005.12.025
Pellerito, L., and Nagy, L. (2002). Organotin(IV)n+ Complexes Formed with Biologically Active Ligands: Equilibrium and Structural Studies, and Some Biological Aspects. Coord. Chem. Rev. 224 (1-2), 111–150. doi:10.1016/S0010-8545(01)00399-X
Piver, W. T. (1973). Organotin Compounds: Industrial Applications and Biological Investigation. Environ. Health Perspect., 61–79. doi:10.1289/ehp.730461
Rachek, L. I., Yuzefovych, L. V., Ledoux, S. P., Julie, N. L., and Wilson, G. L. (2009). Troglitazone, but Not Rosiglitazone, Damages Mitochondrial DANN and Induces Mitochondrial Dysfunction and Cell Death in Human Hepatocytes. Toxicol. Appl. Pharmacol. 240, 348–354. doi:10.1016/j.taap.2009.07.021
Ray, D., Sarma, K. D., and Antony, A. (2000). Differential Effects of Tri-n-butylstannyl Benzoates on Induction of Apoptosis in K562 and MCF-7 Cells. IUMBM Life 49, 519–525. doi:10.1080/15216540050167061
Rehman, W., Yasmeen, R., Rahim, F., Waseem, M., Guo, C. Y., Hassan, Z., et al. (2016). Synthesis Biological Screening and Molecular Docking Studies of Some Tin(IV) Schiff Base Adducts. J. Photochem. Photobiol. B, Biol. 164, 65–72. doi:10.1016/j.jphotobiol.2016.09.018
Ricci, C., Pastukh, V., Leonard, J., Turrens, J., Wilson, G., Schaffer, D., et al. (2008). Mitochondrial DNA Damage Triggers Mitochondrial-Superoxide Generation and Apoptosis. Am. J. Physiol. Cel Physiol. 294, 413–422. doi:10.1152/ajpcell.00362.2007
Riedl, S. J., and Shi, Y. (2004). Molecular Mechanisms of Caspase Regulation during Apoptosis. Nat. Rev. Mol. Cel Biol. 5, 897–907. doi:10.1038/nrm1496
Riley, T., Sontag, E., Chen, P., and Levine, A. (2008). Transcriptional Control of Human P53-Regulated Genes. Nat. Rev. Mol. Cel Biol. 9, 402–412. doi:10.1038/nrm2395
Ross, A. (1965). Industrial Applications or Organotin Compounds. Ann. N. Y Acad. Sci. 125, 107–123. doi:10.1111/j.1749-6632.1965.tb45382.x
Rubino, S., Pibiri, I., Minacori, C., Alduina, R., Di Stefano, V., Orecchio, S., et al. (2018). Synthesis, Structural Characterization, Anti-proliferative and Antimicrobial Activity of Binuclear and Mononuclear Pt(II) Complexes with Perfluoroalkyl-Heterocyclic Ligands. Inorg. Chim. Acta 483, 180–190. doi:10.1016/j.ica.2018.07.039
Salas, J. M., Romero, M. A., Sánchez, M. P., and Quirós, M. (1999). Metal Complexes of [1,2,4]triazolo-[1,5-A]pyrimidine Derivatives. Coord. Chem. Rev. 193, 1119–1142. doi:10.1016/S0010-8545(99)00004-1
Samuel, M. P., de Vos, D., Raveendra, D., Sarma, J. A. R. P., and Roy, S. (2002). 3-D QSAR Studies on New Dibenzyltin(IV) Anticancer Agents by Comparative Molecular Field Analysis (CoMFA). Bioorg. Med. Chem. Lett. 12 (1), 61–64. doi:10.1016/S0960-894X(01)00684-9
Sancar, A., Lindsey-Boltz, L. A., Unsal-Kacmaz, K., and Linn, S. (2004). Molecular Mechanisms of Mammalian DNA Repair and the DANN Damage Checkpoints. Annu. Rev. Biochem. 73, 39–85. doi:10.1146/annurev.biochem.73.011303.073723
Sardon, H., Engler, A. C., Chan, J., García, J., Coady, D., Pascual, A., et al. (2013). Organic Acid-Catalyzed Polyurethane Formation via a Dual-Activated Mechanism: Unexpected Preference of Nactivation over O-Activation of Isocyanates. J. Am. Chem. Soc. 135(43): 16235–16241. doi:10.1021/ja408641g
Sausville, E. A., Arbuck, S. G., Messmann, R., Headlee, D., Bauer, K. S., Lush, R. M., et al. (2001). Phase I Trial of 72 hour Continuous Infusion UCN-01 in Patients with Refractory Neoplasms. J. Clin. Oncol. 19, 2319–2333. doi:10.1200/JCO.2001.19.8.2319
Savill, J. (1997). Recognition and Phagocytosis of Cells Undergoing Apoptosis. Br. Med. Bull. 53, 491–508. doi:10.1093/oxfordjournals.bmb.a011626
Savill, J., and Fadok, V. (2000). Corpse Clearance Defines the Meaning of Cell Death. Nature 407, 784–788. doi:10.1038/35037722
Sedaghat, T., Golalzadeh, A., and Motamedi, H. (2013). Diorganotin Complexes with N(4)-Phenylthiosemicarbazones: Synthesis, Spectroscopic Characterization, and Antibacterial Activity. Phosphorus Sulfur Silicon Relat. Elem 188, 1694–1702. doi:10.1080/10426507.2013.777727
Senderowicz, A. M., and Sausville, E. A. (2000). Preclinical and Clinical Development of Cyclin-dependent Kinase Modulators. J. Natl. Cancer Inst. 92, 376–387. doi:10.1093/jnci/92.5.376
Senese, S., Lo, Y. C., Huang, D., Zangle, T. A., Gholkar, A. A., Robert, L., et al. (2014). Chemical Dissection of the Cell Cycle: Probes for Cell Biology and Anti-cancer Drug Development. Cell Death Dis 5 (10), e1462. doi:10.1038/cddis.2014.420
Shaheen, F., Ali, S., and Shahzadi, S. (2017). Synthesis, Characterization, DNA Interaction and Anticancer Activity of Organotin(IV) Complexes with Sodium 3-(1h-Indol-3-Yl) Propanoate. Der Chemica Sinica 8 (6), 494–502.
Shapiro, G. I., Supko, J. G., Patterson, A., Lynch, C., Lucca, J., Zacarola, P. F., et al. (2001). A Phase II Trial of the Cyclin-dependent Kinase Inhibitor Flavopiridol in Patients with Previously Untreated Stage Iv Non-small Cell Lung Cancer. Clin. Cancer Res. 7, 1590–1599.
Shehata, M. R., Mohamed, M. M. A., Shoukry, M. M., Hussein, M. A., and Hussein, F. M. (2015). Synthesis, Characterization, Equilibria and Biological Activity of Dimethyltin(IV) Complex with 1,4-piperazine. J. Coord. Chem. 68 (6), 1101–1114. doi:10.1080/00958972.2015.1007962
Shuaibu, M. N., Kanbara, H., Yanagi, T., Ichinose, A., Ameh, D. A., Bonire, J. J., et al. (2003). In Vitro trypanocidal Activity of Dibutyltin Dichloride and its Fatty Acid Derivatives. Parasitol. Res. 91 (1), 5–11. doi:10.1007/s00436-003-0861-2
Silverman, R. B., and Holladay, W. M. (2015). The Organic Chemistry of Drug Design and Drug Action. USA: Academic Press. doi:10.1016/C2009-0-64537-2
Sinha, K., Das, J., Pal, P. B., and Sil, P. C. (2013). Oxidative Stress: the Mitochondria-dependent and Mitochondria-independent Pathways of Apoptosis. Arch. Toxicol. 87 (7), 1157–1180. doi:10.1007/s00204-013-1034-4
Sirajuddin, M., Ali, S., McKee, V., Sohail, M., and Pasha, H. (2014). Potentially Bioactive Organotin(IV) Compounds: Synthesis, Characterization, In Vitro Bioactivities and Interaction with SS-DNA. Eur. J. Med. Chem. 84, 343–363. doi:10.1016/j.ejmech.2014.07.028
Sirajuddin, M., Ali, S., and Tahir, M. N. (2016). Pharmacological Investigation of Mono-, Di- and tri-Organotin(IV) Derivatives of Carbodithioates: Design, Spectroscopic Characterization, Interaction with SS-DNA and POM Analyses. Inorg. Chim. Acta 439, 145–158. doi:10.1016/j.ica.2015.10.017
Srinivasula, S. M., Ahmad, M., Fernandes-Alnemri, T., and Alnemri, E. S. (1998). Autoactivation of Procaspase-9 byApaf-1- Mediated Oligomerization. Mol. Cel 1, 949–957. doi:10.1016/S1097-2765(00)80095-7
Tabassum, S., and Pettinari, C. (2006). Chemical and Biotechnological Developments in Organotin Cancer Chemotherapy. J. Organomet. Chem. 691, 1761–1766. doi:10.1016/j.jorganchem.2005.12.033
Trump, B. F., Berezesky, I. K., Chang, S. H., and Phelps, P. C. (1997). The Pathways of Cell Death: Oncosis, Apoptosis, and Necrosis. Toxicol. Pathol. 25, 82–88. doi:10.1177/019262339702500116
Van der Kerk, G. J. M., and Luijten, J. G. A. (1954). Investigations on Organo-Tin Compounds. III. The Biocidal Properties of Organo-Tin Compounds. J. App Chem. 4 (6), 314–319. doi:10.1002/jctb.5010040607
Van der Kerk, G. J. M., and Luitjten, J. G. A. (1956). Investigations on Organo-Tin Compounds. V the Preparation and Antifungal Properties of Unsymmetrical Tri-n-alkyltin Acetates. J. App Chem. 6 (2), 56–60. doi:10.1002/jctb.5010060202
Van der Kerk, G. J. M. (1975). Organotin Chemistry: Past, Present and Future. Organotin Compd. New Chem. Appl., 1–25. doi:10.1021/ba-1976-0157.ch001
Verginadis, I. I., Karkabounas, S., Simos, Y., Kontargiris, E., Hadjikakou, S. K., Batistatou, A., et al. (2011). Anticancer and Cytotoxic Effects of a Triorganotin Compound with 2-Mercapto-Nicotinic Acid in Malignant Cell Lines and Tumor Bearing Wistar Rats. Eur. J. Pharm. Sci. 42, 253–261. doi:10.1016/j.ejps.2010.11.015
Xiao, Z., Chen, Z., Gunasekera, A. H., Sowin, T. J., Rosenberg, S. H., Fesik, S., et al. (2003). Chk1 Mediates S and G2 Arrests through Cdc25A Degradation in Response to DNA-Damaging Agents. J. Biol. Chem. 278, 21767–21773. doi:10.1074/jbc.M300229200
Yakes, F. M., and VanHouten, B. (1997). Mitochondrial DNA Damage Is More Extensive and Persists Longer Than Nuclear DNA Damage in Human Cells Following Oxidative Stress. Proc. Natl. Acad. Sci. U. S. A. 94, 516–519. doi:10.1073/pnas.94.2.514
Yamaguchi, Y., Passeron, T., Watabe, H., Yasumoto, K. I., Rouzaud, F., Hoashi, T., et al. (2007). The Effects of Dickkopf 1 on Gene Expression and Wnt Signaling by Melanocytes: Mechanisms Underlying its Suppression of Melanocyte Function and Proliferation. J. Invest. Dermatol. 127 (5), 1217–1225. doi:10.1038/sj.jid.5700629
Yang, X., Chang, H. Y., and Baltimore, D. (1998). Autoproteolytic Activation of Procaspases by Oligomerization. Mol. Cel 1, 319–325. doi:10.1016/s1097-2765(00)80032-5
Yunlan, L., Juan, Z., and Qingshan, L. (2014). Antitumor Activity of di-n-butyl-(2,6-difluorobenzohydroxamato)Tin(IV) against Human Gastric Carcinoma SGC-7901 Cells via G2/M Cell Cycle Arrest and Cell Apoptosis. Plos One 9 (3), 1–13. doi:10.1371/journal.pone.0090793
Yusof, E. N. M., Latif, M. A. M., Tahir, M. I. M., Sakoff, J. A., Simone, M. I., Page, A. J., et al. (2019). o-Vanillin Derived Schiff Bases and Their Organotin(IV) Compounds: Synthesis, Structural Characterisation, In-Silico Studies and Cytotoxicity. Int. J. Mol. Sci. 20 (854), 2–34. doi:10.3390/ijms20040854
Keywords: molecular, cytotoxicity, anticancer, application, ligand, organotin
Citation: Syed Annuar SN, Kamaludin NF, Awang N and Chan KM (2021) Cellular Basis of Organotin(IV) Derivatives as Anticancer Metallodrugs: A Review. Front. Chem. 9:657599. doi: 10.3389/fchem.2021.657599
Received: 18 February 2021; Accepted: 21 June 2021;
Published: 23 July 2021.
Edited by:
Simone Brogi, University of Pisa, ItalyCopyright © 2021 Syed Annuar, Kamaludin, Awang and Chan. This is an open-access article distributed under the terms of the Creative Commons Attribution License (CC BY). The use, distribution or reproduction in other forums is permitted, provided the original author(s) and the copyright owner(s) are credited and that the original publication in this journal is cited, in accordance with accepted academic practice. No use, distribution or reproduction is permitted which does not comply with these terms.
*Correspondence: Nurul Farahana Kamaludin, bnVydWxmYXJhaGFuYUB1a20uZWR1Lm15
†These authors have contributed equally to this work and share first authorship