- 1Changchun Institute of Optics, Fine Mechanics and Physics, Chinese Academy of Sciences, Changchun, China
- 2State Key Laboratory of Integrated Optoelectronics, College of Electronic Science and Engineering, Jilin University, Changchun, China
- 3Key Laboratory of Icing and Anti/De-icing, China Aerodynamics Research and Development Center, Mianyang Sichuan, China
In this minireview, we comprehensively reviewed recent progress on fabricating anti-icing/de-icing surfaces by femtosecond laser technologies. Typical bioinspired micro-/nano-structures fabrication strategies, superhydrophobic surfaces with anti-icing properties, and photothermal surfaces with de-icing properties are summarized. At last, we discussed challenges and prospects in anti-icing/de-icing surfaces fabricated by femtosecond laser technologies.
1 Introduction
Icing shows serious problems in power, energy, and communications (Pan et al., 2015; Han et al., 2020a). Traditional de-icing technologies include manual, mechanical, thermal, laser, electromagnetic, and ultrasonic field (Dhyani et al., 2022; Huang et al., 2022). Among various traditional de-icing technologies, manual de-icing technology is the most commonly used. But manual de-icing is low efficiency and high cost (Cheng et al., 2022; Patel et al., 2022). In the past decade, inspired by nature, significant progress in de-icing/anti-icing has been developed (Yi et al., 2021; Jiao et al., 2023). For example, inspired by the superhydrophobic properties of lotus leaves, researchers have successfully prepared superhydrophobic surfaces for anti-icing (Han et al., 2020b; Chen et al., 2022). Inspired by moth eyes, micro-/nano-structures convert light into thermal energy under sunlight irradiation leading to ice melting, which is energy-saving, environmentally friendliness, and low-cost (Zhao et al., 2021; Chen et al., 2022; Liu et al., 2022).
Femtosecond laser fabrication technologies have advantages in ultrashort pulse duration, ultra-high instantaneous power, ultra-fine processing structure (You et al., 2020; Zheng et al., 2020; Fu et al., 2021; Zhang et al., 2021; Jin et al., 2022). In particular, the fine micro-/nano-structures play a vital role in the aspect controlling surface wettability, such as de-icing, anti-icing, superhydrophobic, superoleophobic, and slippery surface (Yong et al., 2017; Yong J. et al., 2019; Feng and Yong, 2020; Yong et al., 2020; Yong et al., 2022a). Compared with other micro-/nano-fabrication technologies, femtosecond laser shows distinguish advantages in flexible realizing three-dimensional micro-/nano-structures for a variety of materials (Liu et al., 2020; Fang et al., 2021; Somers et al., 2021; Wang et al., 2021).
In this minireview, we comprehensively reviewed recent progress on fabricating anti-icing/de-icing surfaces by femtosecond laser technologies. Typical bioinspired micro-/nano-structures fabrication strategies, superhydrophobic surfaces with anti-icing properties, and photothermal surfaces with de-icing properties are summarized. At last, we discussed challenges and prospects in anti-icing/de-icing surfaces fabricated by femtosecond laser technologies.
2 Fabrication of structured surfaces
Micro-/nano-structures are essential in superhydrophobic anti-icing surface and photothermal de-icing surfaces. Figure 1A shows the scheme for typical laser processing equipment (Yong J. L. et al., 2019; Yong et al., 2022a; Yong et al., 2022b). A femtosecond laser is focused on the material surface through an objective lens. The sample is fixed on a translation stage. During the laser treatment process, femtosecond laser direct writing occurs on materials surfaces by moving the translation stage (Figures 1B,C). In the process of femtosecond laser treatment, high temperature and high pressure will be formed on the laser focus area (Zhang et al., 2017; Zhang and Sugioka, 2019; Zhang et al., 2020; Liu et al., 2022; Zhang D. S et al., 2022). Therefore, various bio-inspired structures have been fabricated for superhydrophobic anti-icing surfaces and photothermal de-icing surfaces.
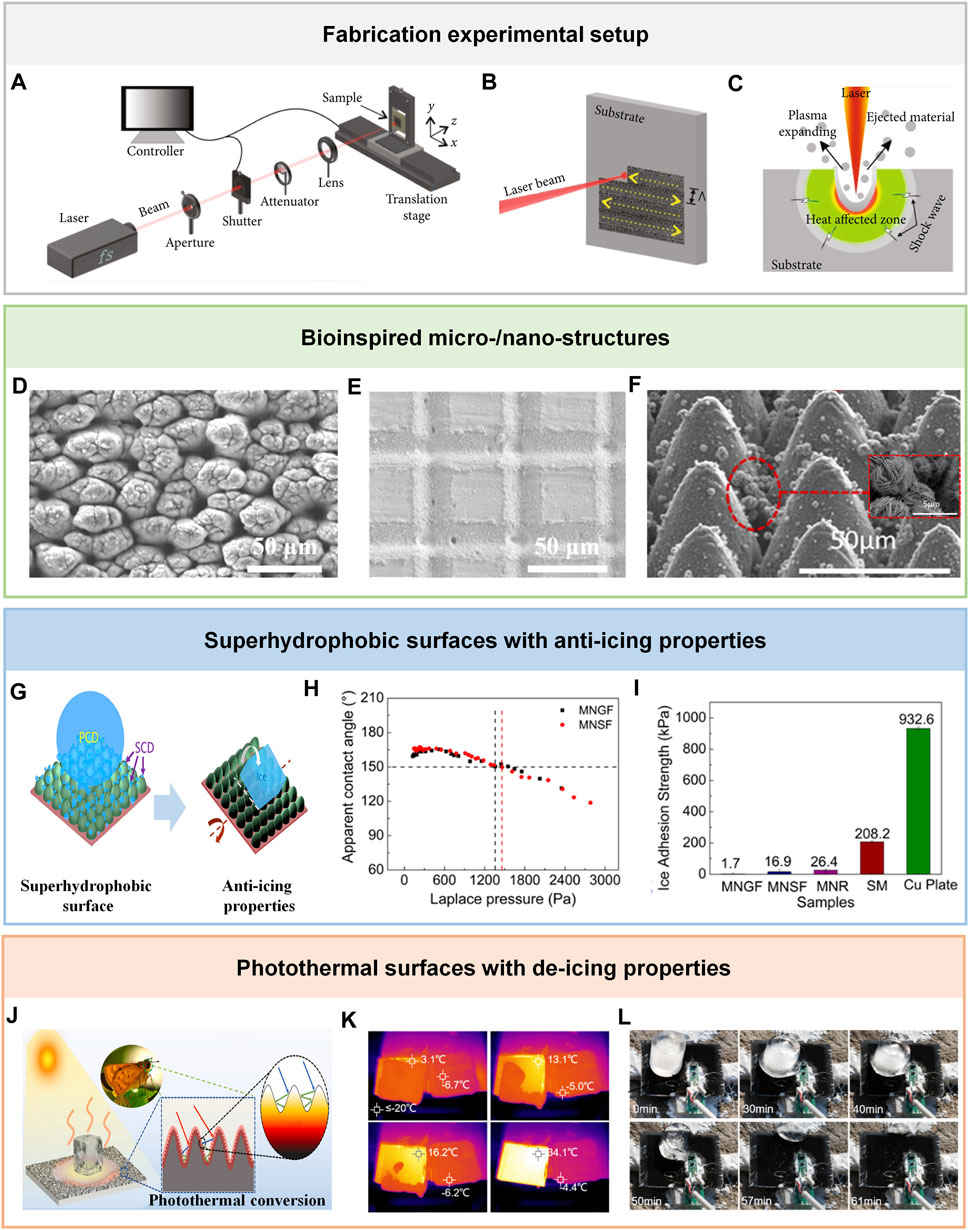
FIGURE 1. Fabrication of anti-icing/de-icing surfaces using the femtosecond laser. (A) Femtosecond laser direct writing experimental setup. (B) The scheme for laser scanning. (C) The scheme for the interaction between femtosecond laser and materials. Reproduced under the terms of the CC-BY Creative Commons Attribution 4.0 International License (Yong et al., 2022a) Copyright 2022, The Authors, Published by AAAS. Reproduced under the terms of the CC-BY Creative Commons Attribution 3.0 International License (Yong et al., 2022b) Copyright 2022, The Authors, Published by IOP Publishing Ltd. Reproduced from (Yong J. L. et al., 2019) with permission of American Chemical Society. (D) SEM images of mound structures. Reproduced from (Huang et al., 2018) with permission of Laser Institute of America. (E) SEM images of periodic square-shaped structures. Reproduced under the terms of the CC-BY Creative Commons Attribution 4.0 International License (Volpe et al., 2020) Copyright 2020, The Authors, Published by MDPI. (F) SEM images of triple-scale structures. Reproduced from (Pan et al., 2021) with permission of American Chemical Society. (G) The scheme for superhydrophobic surfaces with anti-icing properties. Reproduced from (Pan et al., 2021) with permission of American Chemical Society. (H) The relationship between CA and Laplace pressure. Reproduced from (Pan et al., 2021) with permission of American Chemical Society. (I) The ice adhesion strength of different superhydrophobic surfaces. Reproduced from (Pan et al., 2021) with permission of American Chemical Society. (J) The scheme for photothermal surfaces with de-icing properties. Reproduced from (Zhao et al., 2021) with permission of Elsevier. (K) The photothermal performance of laser-treated surfaces. Reproduced from (Chen et al., 2022) with permission of Elsevier. (L) Outdoor de-icing experiments. Reproduced from (Chen et al., 2022) with permission of Elsevier.
Using the above-mentioned processing equipment, various bioinspired micro-/nano-structures, such as mound structures, periodic square-shaped structures, microcones structures, Siberian-cocklebur-like structures, and moth-eye-inspired structures, have been fabricated (Huang et al., 2018; Volpe et al., 2020; Ge et al., 2021; Pan et al., 2021). For example, as shown in Figure 1D, Huang et al. reported mound-structured surfaces on an aluminum alloy surface (Huang et al., 2018). The tall and short mound surfaces were fabricated by controlling the femtosecond laser fluence, laser spot radius, and different laser pulses. As shown in Figure 1E, Volpe et al. fabricated periodic square-shaped structures on aluminum alloy by scanning in parallel and perpendicular directions (Volpe et al., 2020). The depth is ∼8 μm, and the hatch distance is 10 μm–500 μm. Impressively, Pan et al. fabricated a triple-scale surface (Pan et al., 2021). The microcones were prepared by ultrafast laser ablation. The nano grasses and micro flower were formed on microcones structures after chemical oxidation (Figure 1F).
3 Superhydrophobic surfaces for anti-icing
Typically, the contact angle of water droplets on superhydrophobic surfaces is above 150°. Therefore, water droplets roll freely on superhydrophobic surfaces. The reason for superhydrophobic anti-icing surfaces are as follows (Figure 1G): i. Water droplets are hard to stay on superhydrophobic surfaces. ii. The formation time of ice crystals will be delayed. iii. The adhesion will be decreased.
When it comes to superhydrophobic surfaces fabricated by femtosecond laser technology, Huang et al. fabricated a superhydrophobic aluminum alloy surface by combining femtosecond laser technology with surface chemistry modification technology (Huang et al., 2018). The freezing delay can be up to 530 s because of excellent superhydrophobic properties. As a pioneer, Zhong’s group prepared a superhydrophobic surface with triple-scale structures (Pan et al., 2021). Notably, the contact angle of water drops is above 150° (Figure 1H). The ice adhesion is ∼1.7 kPa at -25°C (Figure 1I). Further, Zhong’s group developed superhydrophobic surfaces with robust icephobic performance by modification of polydimethylsiloxane on superhydrophobic surfaces (Che et al., 2022). In addition to post-modifying, Yin et al. prepared superhydrophobic polytetrafluoroethylene (PTFE) only by femtosecond laser technology (Yin et al., 2018). The contact angle of water drops is 157°. The water froze on the untreated PTFE after ∼14 min. In contrast, the water froze on the treated PTFE after ∼33 min.
4 Photothermal surfaces for de-icing
Photothermal surfaces convert solar energy into heat to melt the ice on the surface. Photothermal surfaces for de-icing show great features of low-cost and energy saving. As shown in Figure 1J, the incident light reflects between the micro-/nano-structures, reducing the reflectivity of materials and improving the absorption of materials. Therefore, sunlight is trapped in micro-/nano-structures, leading to enhancing light absorption.
It is worth noting that femtosecond laser technology can fabricate micro-/nano-structures to improve photothermal conversion ability for de-icing. For example, Zhao et al. fabricated moth-eye-inspired texturing surfaces for photothermal de-icing surfaces (Zhao et al., 2021). The remelted particles wrapped the micro-mountain, increasing optical path and light absorption. Moth-eye-inspired texturing surface temperatures rise from room temperature (∼30°C) to ∼80°C under one sun (1 kW/m2) irradiation for 300 s. After 180 s of illumination, the ice and melted water slide away. Moreover, Chen et al. prepared cauliflower-like surfaces for durable photothermal de-icing (Chen et al., 2022). Because of the combination of chemical reaction treatment, nanoscale structures are grown on the aluminum surface. The absorptivity reaches 97.3%. The high absorptivity results in better photothermal conversion capability, which is helpful to improve the photothermal deicing ability. The surface temperature increases by 48.5 °C within 300 s under one sun (1 kW/m2) irradiation (Figure 1K), and the ice can melt in 2 min (Figure 1L).
5 Conclusion and outlook
In this minireview, we comprehensively reviewed fabricating anti-icing/de-icing surfaces by femtosecond laser technologies. Typical bioinspired micro-/nano-structures fabrication strategies, superhydrophobic surfaces for anti-icing, and photothermal surfaces for de-icing are summarized. The superhydrophobic anti-icing surface and the photothermal de-icing surface depend on the bioinspired micro-/nano-structures. In the future, new concept micro-/nano-structures can be designed and fabricated to improve anti-icing and de-icing performance. For example, as a pioneer, Chen’s group reported the slippery liquid-infused porous surfaces for excellent ice resistance performance (Zhang J. L et al., 2022). The ice-delay time of slippery liquid infused porous surfaces was extended by 21.5% compared with the superhydrophobic surface. Furthermore, new fabrication technologies (such as laser interference and multi-beam parallel processing) will be explored to efficiently prepare large-area anti-icing and de-icing surfaces using an optical processing system design. In the future, significant progress will contribute to femtosecond laser technologies that enable anti-icing/de-icing surfaces into potential applications in aircraft, ships, and aerospace surfaces.
Author contributions
All authors listed have made a substantial, direct and intellectual contribution to the work, and approved it for publication.
Funding
This work was supported by the Key Laboratory of Icing and Anti/De-icing of CARDC Nos. # IADL 20210404; the National Natural Science Foundation of China (NSFC) under Grant Nos. #62275100, #61905087, and #61935008; Tsinghua University (School of Materials Science and Engineering)-AVIC Aerodynamics Research Institute Joint Research Center for Advanced Materials and Anti-Icing Nos. #JCAMAI-2020-03; Jilin Province Development and Reform Commission Project Nos. #2022C047-4.
Conflict of interest
The authors declare that the research was conducted in the absence of any commercial or financial relationships that could be construed as a potential conflict of interest.
Publisher’s note
All claims expressed in this article are solely those of the authors and do not necessarily represent those of their affiliated organizations, or those of the publisher, the editors and the reviewers. Any product that may be evaluated in this article, or claim that may be made by its manufacturer, is not guaranteed or endorsed by the publisher.
References
Che, C. H., Tia, Z., Lu, X., Jian, G. C., Hu, X. Y., Wan, L. Z., et al. (2022). Micro-nano-nanowire triple structure-held pdms superhydrophobic surfaces for robust ultra-long-term icephobic performance. ACS Appl. Mat. Interfaces 14 (20), 23973–23982. doi:10.1021/acsami.2c02992
Chen, C. H., Tian, Z., Luo, X., Jiang, G. C., Hu, X. Y., Wang, L. Z., et al. (2022). Cauliflower-like micro-nano structured superhydrophobic surfaces for durable anti-icing and photothermal de-icing. Chem. Eng. J. 450, 137936. doi:10.1016/j.cej.2022.137936
Cheng, P. F., Wang, D., and Schaaf, P. (2022). A review on photothermal conversion of solar energy with nanomaterials and nanostructures: From fundamentals to applications. Adv. Sustain. Syst. 6 (9), 2200115. doi:10.1002/adsu.202200115
Dhyani, A., Choi, W., Golovin, K., and Tuteja, A. (2022). Surface design strategies for mitigating ice and snow accretion. Matter 5 (5), 1423–1454. doi:10.1016/j.matt.2022.04.012
Fang, L. N., Li, J. C., Zhang, J. R., and Han, D. D. (2021). Femtosecond laser structuring for flexible surface-enhanced Raman spectroscopy substrates. IEEE Photonics J. 13 (2), 1–8. doi:10.1109/jphot.2021.3069648
Feng, N., and Yong, J. L. (2020). Femtosecond laser microfabrication of porous superwetting materials for oil/water separation: A mini-review. Front. Chem. 8, 585723. doi:10.3389/fchem.2020.585723
Fu, X. Y., Cai, Q., Ma, J. N., Zhu, L., Han, D. D., and Zhang, Y. L. (2021). Free-standing and flexible graphene supercapacitors of high areal capacitance fabricated by laser holography reduction of graphene oxide. Appl. Phys. Lett. 118 (7), 071601. doi:10.1063/5.0038508
Ge, C. F., Yuan, G., Guo, C. L., Ngo, C. V., and Li, W. (2021). Femtosecond laser fabrication of square pillars integrated Siberian-Cocklebur-like microstructures surface for anti-icing. Mater. Des. 204, 109689. doi:10.1016/j.matdes.2021.109689
Han, D. D., Cai, Q., Chen, Z. D., Li, J. C., Mao, J. W., Lv, P., et al. (2020a). Bioinspired surfaces with switchable wettability. Front. Chem. 8, 692. doi:10.3389/fchem.2020.00692
Han, D. D., Chen, Z. D., Li, J. C., Mao, J. W., Jiao, Z. Z., Wang, W., et al. (2020b). Airflow enhanced solar evaporation based on Janus graphene membranes with stable interfacial floatability. ACS Appl. Mat. Interfaces 12, 25435–25443. doi:10.1021/acsami.0c05401
Huang, C. J., Bell, R., Tsubaki, A., Zuhlke, C. A., and Alexander, D. R. (2018). Condensation and subsequent freezing delays as a result of using femtosecond laser functionalized surfaces. J. Laser Appl. 30 (1), 011501. doi:10.2351/1.4986058
Huang, W., Huang, J. X., Guo, Z. G., and Liu, W. M. (2022). Icephobic/anti-icing properties of superhydrophobic surfaces. Adv. Colloid Interface Sci. 304, 102658. doi:10.1016/j.cis.2022.102658
Jiao, Z. Z., Zhou, H., Han, X. C., Han, D. D., and Zhang, Y. L. (2023). Photothermal responsive slippery surfaces based on laser-structured graphene@PVDF composites. J. Colloid Interface Sci. 629, 582–592. doi:10.1016/j.jcis.2022.08.153
Jin, Z., Peng, Y., Fang, Y., Ye, Z., Fan, Z., Liu, Z., et al. (2022). Photoinduced large polaron transport and dynamics in organic–inorganic hybrid lead halide perovskite with terahertz probes. Light. Sci. Appl. 11 (1), 209. doi:10.1038/s41377-022-00872-y
Liu, R. J., Zhang, D. S., Ji, S. H., Cai, Y. Y., Liang, C. H., and Li, Z. G. (2022). Femtosecond laser generated hierarchical macropore/LIPSS metasurfaces and their ultrabroadband absorbance, photothermal properties, and thermal-induced reflectance oscillation. ACS Appl. Electron. Mat. 4 (3), 990–1001. doi:10.1021/acsaelm.1c01180
Liu, Y. Q., Mao, J. W., Chen, Z. D., Han, D. D., Jiao, Z. Z., Ma, J. N., et al. (2020). Three-dimensional micropatterning of graphene by femtosecond laser direct writing technology. Opt. Lett. 45 (1), 113–116. doi:10.1364/ol.45.000113
Pan, P., Wu, S. P., Xiao, Y., and Liu, G. (2015). A review on hydronic asphalt pavement for energy harvesting and snow melting. Renew. Sustain. Energy Rev. 48, 624–634. doi:10.1016/j.rser.2015.04.029
Pan, R., Zhang, H. J., and Zhong, M. L. (2021). Triple-scale superhydrophobic surface with excellent anti-icing and icephobic performance via ultrafast laser hybrid fabrication. ACS Appl. Mat. Interfaces 13 (1), 1743–1753. doi:10.1021/acsami.0c16259
Patel, S., Ershad, F., Zhao, M., Isseroff, R. R., Duan, B., Zhou, Y., et al. (2022). Wearable electronics for skin wound monitoring and healing. Soft Sci. 2 (2), 9. doi:10.20517/ss.2022.13
Somers, P., Liang, Z., Johnson, J. E., Boudouris, B. W., Pan, L., and Xu, X. (2021). Rapid, continuous projection multi-photon 3D printing enabled by spatiotemporal focusing of femtosecond pulses. Light. Sci. Appl. 10 (1), 199. doi:10.1038/s41377-021-00645-z
Volpe, A., Gaudiuso, C., Di Venere, L., Licciulli, F., Giordano, F., and Ancona, A. (2020). Direct femtosecond laser fabrication of superhydrophobic aluminum alloy surfaces with anti-icing properties. Coatings 10 (6), 587. doi:10.3390/coatings10060587
Wang, H., Zhang, Y. L., Han, D. D., Wang, W., and Sun, H. B. (2021). Laser fabrication of modular superhydrophobic chips for reconfigurable assembly and self-propelled droplet manipulation. PhotoniX 2 (1), 17. doi:10.1186/s43074-021-00033-1
Yi, J., Zhou, H., Wei, W. H., Han, X. C., Han, D. D., and Gao, B. R. (2021). Micro-/nano-structures fabricated by laser technologies for optoelectronic devices. Front. Chem. 9, 823715. doi:10.3389/fchem.2021.823715
Yin, K., Du, H. F., Luo, Z., Dong, X. R., and Duan, J. A. (2018). Multifunctional micro/nano-patterned PTFE near-superamphiphobic surfaces achieved by a femtosecond laser. Surf. Coat. Technol. 345, 53–60. doi:10.1016/j.surfcoat.2018.04.010
Yong, J. L., Chen, F., Yang, Q., Huo, J. L., and Hou, X. (2017). Superoleophobic surfaces. Chem. Soc. Rev. 46 (14), 4168–4217. doi:10.1039/c6cs00751a
Yong, J. L., Singh, S. C., Zhan, Z. B., ElKabbash, M., Chen, F., and Guo, C. L. (2019b). Femtosecond-laser-produced underwater "superpolymphobic" nanorippled surfaces: Repelling liquid polymers in water for applications of controlling polymer shape and adhesion. ACS Appl. Nano Mat. 2 (11), 7362–7371. doi:10.1021/acsanm.9b01869
Yong, J. L., Yang, Q., Hou, X., and Chen, F. (2020). Relationship and interconversion between superhydrophilicity, underwater superoleophilicity, underwater superaerophilicity, superhydrophobicity, underwater superoleophobicity, and underwater superaerophobicity: A mini-review. Front. Chem. 8, 828. doi:10.3389/fchem.2020.00828
Yong, J., Yang, Q., Guo, C., Chen, F., and Hou, X. (2019a). A review of femtosecond laser-structured superhydrophobic or underwater superoleophobic porous surfaces/materials for efficient oil/water separation. RSC Adv. 9 (22), 12470–12495. doi:10.1039/C8RA10673H
Yong, J., Yang, Q., Hou, X., and Chen, F. (2022a). Nature-inspired superwettability achieved by femtosecond lasers. Ultrafast Sci. 2022, 1–51. doi:10.34133/2022/9895418
Yong, J., Yang, Q., Huo, J., Hou, X., and Chen, F. (2022b). Underwater gas self-transportation along femtosecond laser-written open superhydrophobic surface microchannels (<100 μm) for bubble/gas manipulation. Int. J. Extrem. Manuf. 4 (1), 015002. doi:10.1088/2631-7990/ac466f
You, R., Liu, Y. Q., Hao, Y. L., Han, D. D., Zhang, Y. L., and You, Z. (2020). Laser fabrication of graphene-based flexible electronics. Adv. Mat. 32, 1901981. doi:10.1002/adma.201901981
Zhang, D. S., Ranjan, B., Tanaka, T., and Sugioka, K. (2020). Carbonized hybrid micro/nanostructured metasurfaces produced by femtosecond laser ablation in organic solvents for biomimetic antireflective surfaces. ACS Appl. Nano Mat. 3 (2), 1855–1871. doi:10.1021/acsanm.9b02520
Zhang, D. S., Goekce, B., and Barcikowski, S. (2017). Laser synthesis and processing of colloids: Fundamentals and applications. Chem. Rev. 117 (5), 3990–4103. doi:10.1021/acs.chemrev.6b00468
Zhang, D. S., Li, X. Z., Fu, Y., Yao, Q. H., Li, Z. G., and Sugioka, K. (2022). Liquid vortexes and flows induced by femtosecond laser ablation in liquid governing formation of circular and crisscross LIPSS. Opto-Electronic Adv. 5 (2), 210066. doi:10.29026/oea.2021.210066
Zhang, D. S., and Sugioka, K. (2019). Hierarchical microstructures with high spatial frequency laser induced periodic surface structures possessing different orientations created by femtosecond laser ablation of silicon in liquids. Opto-Electronic Adv. 2 (3), 19000201–19000218. doi:10.29026/oea.2019.190002
Zhang, H. R., Sun, J. C., Yang, J., De Leon, I., Zaccaria, R. P., Qian, H. L., et al. (2021). Biosensing performance of a plasmonic-grating-based nanolaser. Prog. Electromagn. Research-Pier 171, 159–169. doi:10.2528/pier21092405
Zhang, J. L., Yang, Q., Cheng, Y., Fang, Z., Hou, X., and Chen, F. (2022). Slippery liquid-infused porous surface on metal material with excellent ice resistance fabricated by femtosecond bessel laser. Adv. Eng. Mat. 24 (10), 2101738. doi:10.1002/adem.202101738
Zhao, W. R., Xiao, L., He, X. Y., Cui, Z. H., Fang, J. H., Zhang, C., et al. (2021). Moth-eye-inspired texturing surfaces enabled self-cleaning aluminum to achieve photothermal anti-icing. Opt. Laser Technol. 141, 107115. doi:10.1016/j.optlastec.2021.107115
Keywords: anti-icing surface, de-icing surface, bioinspired surface, micro-/nano-fabrication, femtosecond laser
Citation: Tang B-H, Wang Q, Han X-C, Zhou H, Yan X-J, Yu Y and Han D-D (2022) Fabrication of anti-icing/de-icing surfaces by femtosecond laser. Front. Chem. 10:1073473. doi: 10.3389/fchem.2022.1073473
Received: 18 October 2022; Accepted: 10 November 2022;
Published: 24 November 2022.
Edited by:
Dongshi Zhang, Shanghai Jiao Tong University, ChinaReviewed by:
Wang Gong, Hebei University of Technology, ChinaJiale Yong, University of Science and Technology of China, China
Copyright © 2022 Tang, Wang, Han, Zhou, Yan, Yu and Han. This is an open-access article distributed under the terms of the Creative Commons Attribution License (CC BY). The use, distribution or reproduction in other forums is permitted, provided the original author(s) and the copyright owner(s) are credited and that the original publication in this journal is cited, in accordance with accepted academic practice. No use, distribution or reproduction is permitted which does not comply with these terms.
*Correspondence: Dong-Dong Han, aGFuZG9uZ2RvbmdAamx1LmVkdS5jbg==