Exfoliation of Al-Residual Multilayer MXene Using Tetramethylammonium Bases for Conductive Film Applications
- 1Department of Electronics, Graduate School of Engineering, Nagoya University, Nagoya, Japan
- 2Department of Chemistry, Graduate School of Science, Nagoya University, Nagoya, Japan
- 3PRESTO, Japan Science and Technology Agency, Saitama, Japan
- 4Research Center for Materials Science, Nagoya University, Nagoya, Japan
This study describes the concise exfoliation of multilayer Ti3C2Tx MXene containing residual aluminum atoms. Treatment with tetramethylammonium base in a co-solvent of tetrahydrofuran and H2O produced single-layer Ti3C2Tx, which was confirmed via atomic force microscopy observations, with an electrical conductivity 100+ times that of Ti3C2Tx prepared under previously reported conditions. The scanning electron microscopy and X-ray diffraction measurements showed that the exfoliated single-layer Ti3C2Tx MXenes were reconstructed to assembled large-domain layered films, enabling excellent macroscale electric conductivity. X-ray photoelectron spectroscopy confirmed the complete removal of residual Al atoms and the replacement of surface fluorine atoms with hydroxy groups. Using the exfoliated dispersion, a flexible transparent conductive film was formed and demonstrated in an electrical application.
Introduction
Since first reported by Naguib et al. (2011), MXenes have attracted wide attention as novel analogs of two-dimensional layer nanomaterials such as graphene, hexagonal boron nitride, and transition-metal dichalcogenides. MXenes show high mechanical durability and transparency conferred by the layer structure, along with unique chemical natures and excellent electrical characteristics (Naguib et al., 2014; Verger et al., 2019; Wang et al., 2020). The general formula of MXenes is Mn+1XnTx (M: early transition metals, X: carbon and/or nitrogen, T: a terminal functional group such as F, OH, O). The electronic band structures of MXenes depends on the combination of their M, X, and T atoms. For instance, the simple titanium carbide family (e.g., Ti2CTx, Ti3C2Tx) shows a metallic property with high charge-carrier density, whereas Cr2TiC2F2 and Cr2TiC2(OH)2 are thought to behave as semiconductors. Moreover, MXenes with hydrophilic terminal functional groups are dispersible in water and polar organic solvents without surfactants; accordingly unlike other nanomaterials, they can act as scaffolds for chemical modification. These advantages are expected to be exploited in a wide range of applications, such as Li- or Na-ion batteries (Naguib et al., 2012; Liang et al., 2015; Natu et al., 2018), transparent conductive films (Dillon et al., 2016; Hantanasirisakul et al., 2016; Zhou et al., 2021), catalysts for hydrogen evolution (Seh et al., 2016; Intikhab et al., 2019) and supercapacitors (Lukatskaya et al., 2013; Dall’Agnese et al., 2015; Wang et al., 2015).
Typically, MXenes are produced from the MAX phase (Mn+1AlXn) in a hydrogen fluoride (HF) treatment, which removes the atomically thin layer of aluminum atoms (Naguib et al., 2011; Lukatskaya et al., 2013; Luo et al., 2016). To date, researchers can easily access commercially available MXenes, without using a hazardous HF acid (the highest hazard level in the health section of NFPA 704). However, these MXenes maintain their multilayer structure via covalent bonding between the layers and residual Al atoms. Such MXenes are rarely dispersed and immediately precipitate out even after vigorous sonication. Furthermore, films prepared from filtrates of these MXenes are electrically non-conductive. To realize the above applications, conductive liquid-state MXene materials are desired for fabrication processes such as spray coating, spin cast, and filtration. Therefore, a concise and HF-free method that exfoliates and disperses MXene as a single-layer nanomaterial by removing the Al atoms is required.
This work reports on the exfoliation of Al-residual multilayer Ti3C2Tx, the most commonly used conductive MXene, with organic bases containing a tetramethylammonium cation. This treatment effectively removes the interlayer Al atoms from the MXenes. The Ti3C2Tx exfoliated in a co-solvent of tetrahydrofuran (THF) and H2O exhibited superior electrical conductivity. We also demonstrate the application of flexible transparent conductive films fabricated by spray-gun coating with the exfoliated Ti3C2Tx dispersion.
Experimental Section
Sample Preparation
Ti3C2Tx MXene (Japan Material Technologies Corporation, 4 mg), 0.2 mmol of tetramethylammonium reagent (Table 1), and 2 ml of solvent were added to a vial containing a magnetic stirring bar. After stirring at room temperature for 24 h, the mixture was transferred to a 15-ml conical tube with deionized (DI) water (0.2 ml × 4), which were degassed by N2 bubbling for 30 min prior to use. After adding 2-propanol (2 ml), the mixture was centrifuged at 4,500 rpm for 5 min and the supernatant was removed. After adding 2 ml of DI water to the sediment, the mixture was dispersed in a bath-sonicator (BM EQUIPMENT, Nanoruptor NR-350) for 40 min and centrifuged at 433 g for 30 min. The resulting supernatant was collected as the MXene dispersion. The exfoliation yield was estimated from the intensity of the absorption spectrum at 800 nm, recorded on an ultraviolet–visible spectrophotometer (JASCO V-770) after freeze-drying treatment with EYELA FDS-1000.
The sheet resistances (Rs, Ω/sq) of the films were measured using the four-probe method with a Loresta-AX resistivity meter (MCP-TP06P, Mitsubishi Chemical Analytech). Thick MXene films were prepared by vacuum filtration of the dispersions (0.1 mg in 50 ml DI water) on a MF-Millipore 47-mm MCE membrane filter (0.05-µm pore size).
Characterization
The optical absorption spectra of the dispersion sample were recorded on an ultraviolet–visible spectrophotometer (V-770, JASCO). Raman spectra in the radial-breathing mode region were recorded on an inVia Raman Microscope (Renishaw) excited by 785-nm laser light. A single-monochromator micro-Raman spectrometer was employed in the back-scattering configuration. The sample dispersions were drop-casted onto a silicon wafer before the measurement. X-ray photoelectron spectroscopy (XPS) data were obtained using an ESCALAB XI + spectrometer (Thermo Fisher Scientific) using 300 W Al–Ka radiation. To exclude the substrate signals, a highly concentrated MXene dispersion was drop-coated several times onto a Si/SiO2 wafer to form a thick MXene film (>10 nm). Atomic force microscopy (AFM) measurements were acquired using a Dimension Fastscan AFM with a NanoScope V stage controller (Bruker). The samples for AFM observation were prepared by spin-coating the Si/SiO2 wafer with 10 µl of the MXene dispersion at 400 rpm for 60 s, followed by 1,000 rpm for 60 s and 1,600 rpm for 60 s. Scanning electron microscopy (SEM) measurements were conducted in an S-4300 (Hitachi) or an ETHOS NX5000 (Hitachi). X-ray diffraction (XRD) spectra were obtained by a SmartLab (Rigaku) through Cu Kα radiation.
Fabrication of Flexible Transparent Conductive Films
Polyethylenenaphthalete (PEN) films with a thickness of 100 μm and DuPont Films (Q65FA) were surface-treated with a plasma cleaner (YAMATO PR500) at 100 W for 10 min. The MXene dispersion diluted with methanol was coated using a spray-gun (ANEST IWATA HP-TR1). The spray-coated films were dried at 30°C for 16 h under vacuum conditions (<5 Pa). The transparency was determined by the absorption spectra recorded on an ultraviolet–visible spectrophotometer (V-770, JASCO). The sheet resistance (Rs, Ω/sq) of the films was measured using the four-probe method with a Loresta-AX resistivity meter (MCP-TP06P, Mitsubishi Chemical Analytech).
Results and Discussion
Optimization of Exfoliation Conditions
Initially, etching reagents other than HF acid were explored for removing residual Al atoms from Ti3C2Tx MXene. Prior to the investigation, we tested dimethyl sulfoxide (DMSO) (Mashtalir et al., 2013) and tetrabutylammonium cations (Naguib et al., 2015) as intercalation agents that can expand the inter layer distance of MXenes. These agents did not exfoliate the Al-residual multilayered Ti3C2Tx MXene. After screening a variety of organic and inorganic fluoride reagents, it was found that only tetramethylammonium fluoride (Me4NF) in DMSO solvent obtained the desired MXene dispersion in 22% yield (Figure 1A). The optical absorption spectrum of the dispersion exhibited a peak around 800 nm, consistent with reported examples (Li et al., 2017) (Figure 1B). Because tetramethylammonium hydroxide (Me4NOH) can etch the MAX phase (Xuan et al., 2016; Yang et al., 2018), we supposed that the tetramethylammonium cations were suitably sized to penetrate the inter layers of MXenes, which were geometrically restricted by bonding with aluminum atoms.
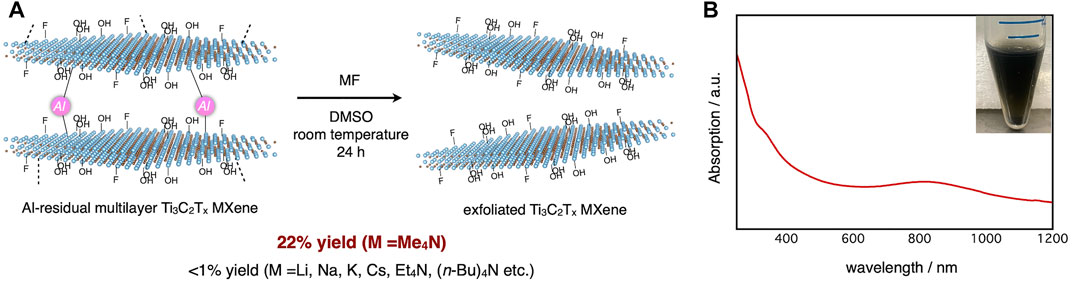
FIGURE 1. (A) Exfoliation of Al-residual Ti3C2Tx MXene with Me4NF; (B) Optical absorption spectrum (main) and typical photograph (inset) of Ti3C2Tx MXene dispersion.
Next, we investigated the effect of the counter anions on tetramethylammonium salts (Table 1). Adding Me4NOH and tetramethylammonium acetate (Me4NOAc) to Me4NF enabled the exfoliation of Ti3C2Tx MXene, implying that basic counter anions are required for removing the aluminum atoms (entries 1–6). The sheet resistance of the film fabricated with the Me4NOH dispersion was 7.1 × 103 Ω/sq., lower than those of the films produced from Me4NF-exfoliated and Me4NOAc-exfoliated MXenes (1.4 × 104 and 3.8 × 104 Ω/sq., respectively). At this stage, we selected Me4NOH as the etching reagent and screened several solvents to improve the exfoliation efficiency and electrical conductivity. When the reaction was performed in aqueous solution under conditions similar to the reported etching conditions of MAX phases, the exfoliation reached 43% yield, but the film conductivity reduced to an undesirable 2.0 × 104 Ω/sq. (entry 7 of Table 1). The exfoliation yield was only 15% in tetrahydrofuran (THF) solvent and was less than 1% in the other organic solvents (entries 8–11 in Table 1). The sheet resistance of the exfoliated MXene (2.0 × 103 Ω/sq.) was three times lower in THF than in DMSO. Surprisingly, a mixed solvent of THF and H2O was effective for both exfoliation (46% yield) and conductivity (2.0 × 103 Ω/sq.). Extending the reaction time also enhanced the exfoliation yield (entries 12 and 13 in Table 1). Under these conditions, MXenes were spontaneously exfoliated without the sonication treatment, and the sheer resistance was drastically decreased to ca. 2.0 × 102 Ω/sq. (entries 14 and 15 in Table 1). Eventually, we found that reacting MXene with Me4NOH in THF/H2O solvent for 120 h produced the desired MXene dispersion, balancing the exfoliation efficiency with high conductivity (entry 15 in Table 1).
Microscopic Observations and Assembled Structural Analysis
Next, the exfoliated Ti3C2Tx MXenes prepared by the spin-coating method were characterized by AFM. A typical AFM image is shown in Figure 2A and the height profile is shown in Figure 2B. The MXene flakes were approximately 2 nm thick, consistent with previous measurements of single-layer MXene (Xuan et al., 2016). Without the sonication treatment, the exfoliated sample presented MXene sheets with an approximate size of >5 µm (Figure 2A), but after sonication, small flakes (<500 nm in size) were observed (Figure 2C). Because tiny defective pinholes were detected on the surface of the large MXene sheet (Figure 2A), it appeared that sonication proceeded by tearing the sheets into small pieces. In the macroscale film, the sheet resistance would be increased by the increased number of contact-resistance points between the networked MXene flakes.
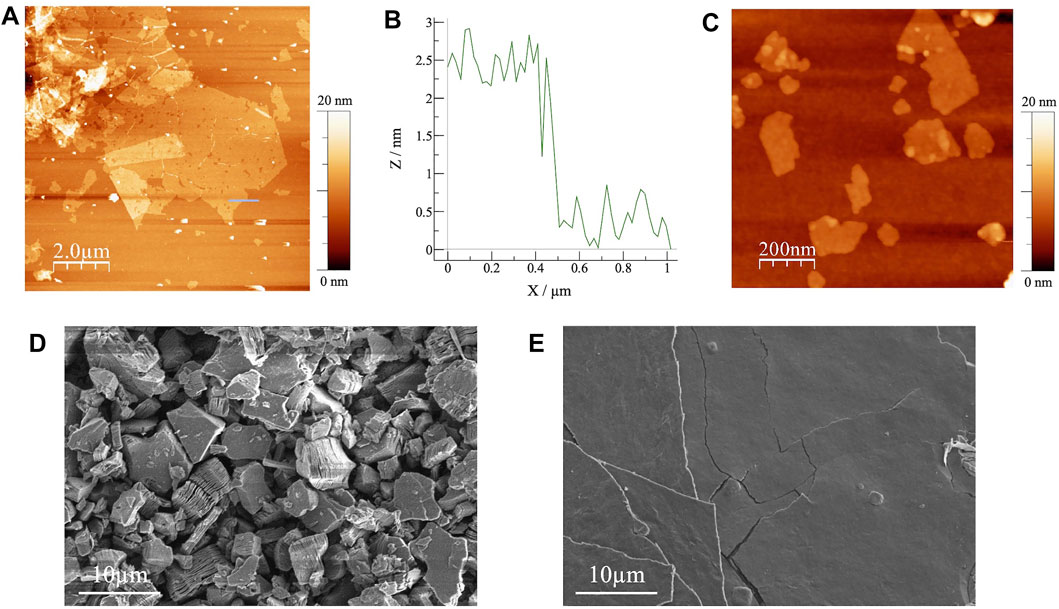
FIGURE 2. (A) AFM image and (B) the corresponding height profile of exfoliated Ti3C2Tx MXene without sonication; (C) AFM image of exfoliated Ti3C2Tx MXene prepared by the sonication treatment; SEM images of (D) pristine and (E) exfoliated Ti3C2Tx MXene.
Figure 2D shows a SEM image of pristine Ti3C2Tx MXene filtrated on the MCE membrane filter. Although the inter layer distances were slightly expanded, most of the flakes maintained the multilayer structure after the sonication treatment. Judging from the polycrystalline-like morphology, a conductivity network is not easily formed in this film. The lateral size of the multilayer Ti3C2Tx was 5–10 μm, compatible to that of exfoliated Ti3C2Tx in the AFM measurement. Conversely, an SEM image of the exfoliated Ti3C2Tx confirmed the formation of paper-like film structures (Figure 2E). In addition, larger domain films, compared with the observed lateral size in AFM, were obtained by the random stacking of the exfoliated flakes, which were similar to other two-dimensional nanomaterials (Dikin et al., 2007; Yun et al., 2017).
The detailed structural changes during the exfoliation and film formation processes were uncovered using XRD measurements (Figure 3). The XRD pattern of the pristine Ti3C2Tx was in good agreement with that of the HF-treated Ti3C2Tx (Naguib et al., 2011) (Figure 3A). After the exfoliation process, the (002) peak (2θ = 8.84°) shifted to a lower angle (2θ = 6.12°), corresponding to an expansion of the average inter layer spacing from 2.00 to 2.88 nm (Figure 3B). A similar expansion of the inter layer distance was reported in previous exfoliation studies using organic intercalation agents (Mashtalir et al., 2013; Naguib et al., 2015). Moreover, the relative intensity of the (002) peak in the spectrum of the exfoliated Ti3C2Tx was obviously enhanced, indicating that the single-layer flakes were stacked with a well-alignment. Figure 3C shows a schematic image of the exfoliation and film formation processes. The multilayer Al-residual Ti3C2Tx was exfoliated by the treatment with Me4NOH. Thereafter, the obtained single-layer Ti3C2Tx dispersion was reconstructed to assembled large-domain layered films, enabling excellent macroscale electric conductivity.
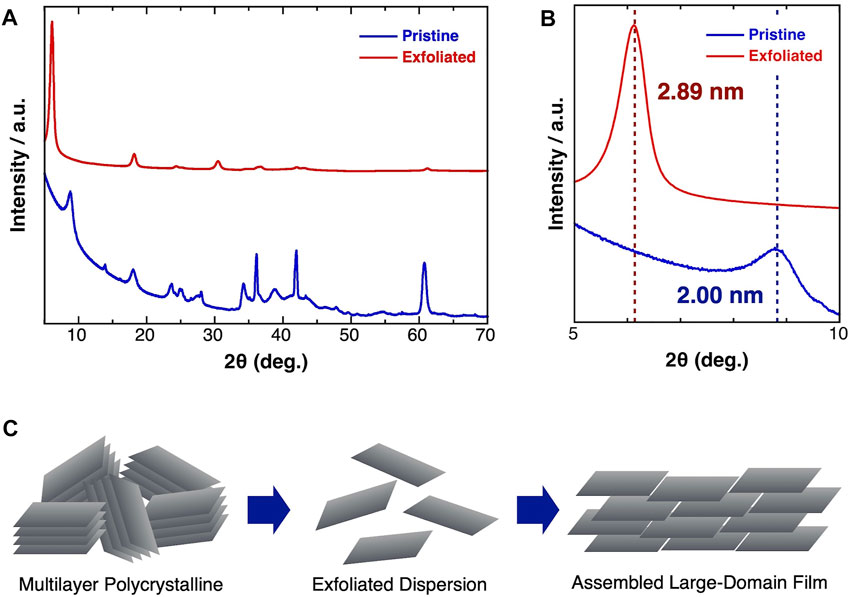
FIGURE 3. (A) Whole region and (B) magnified XRD pattern of (blue) pristine and (red) exfoliated Ti3C2Tx MXene. (C) Schematic image of the exfoliation process and the film formation process of the exfoliated MXene.
Chemical Structure Characterizations
We now report the Raman spectra of pristine and exfoliated MXenes excited by a 785-nm laser (Figure 4). The sharp peaks at 106, 204, and 380 cm−1 in both spectra were assigned to the plasmonic, A1g (Ti, C, T), and Eg (T) peaks of Ti3C2Tx MXenes, respectively. After exfoliation, the A1g peak originating from carbon atoms shifted from 709 to 737 cm−1, close to the typical value of single-layer Ti3C2Tx MXene. This phenomenon strongly suggested that most of the exfoliated MXenes in the dispersion maintained the single-layer state (Sarycheva and Gogotsi, 2020). In addition, the intensity of the A1g peak of the hydroxy groups at around 510 cm−1 and the full width at half maximum of the Eg (T) peak were slightly increased, implying that surface oxidation occurred during the etching reaction.
The chemical structures of the MXenes were further clarified in XPS measurements. The survey spectra of pristine and exfoliated MXenes presented the distinct peaks of titanium (3p: 36 eV, 3s: 61 eV, 2p: 459 eV, and 2s: 563 eV), carbon (1s: 284 eV), oxygen (1s: 531 eV), and fluorine (1s: 691 eV) atoms (Figure 5A). The peak assigned to Al 2p was clearly absent in the high-resolution spectrum of the exfoliated sample (Figure 5B). As expected, the content percentage of aluminum atoms dropped from 1.6 to <0.1 at.% (Table 2). The F 1s peak was also depressed in the survey spectrum of the exfoliated sample. The intensity of the F 1s peaks drastically reduced from 21.5 to 5.4, indicating that the substitution reaction of Ti–F to Ti–OH bonds was promoted by the Me4NOH reagent. Meanwhile, the hydroxy group population increased, consistent with the behavior of the A1g (OH) peak in the Raman spectrum.
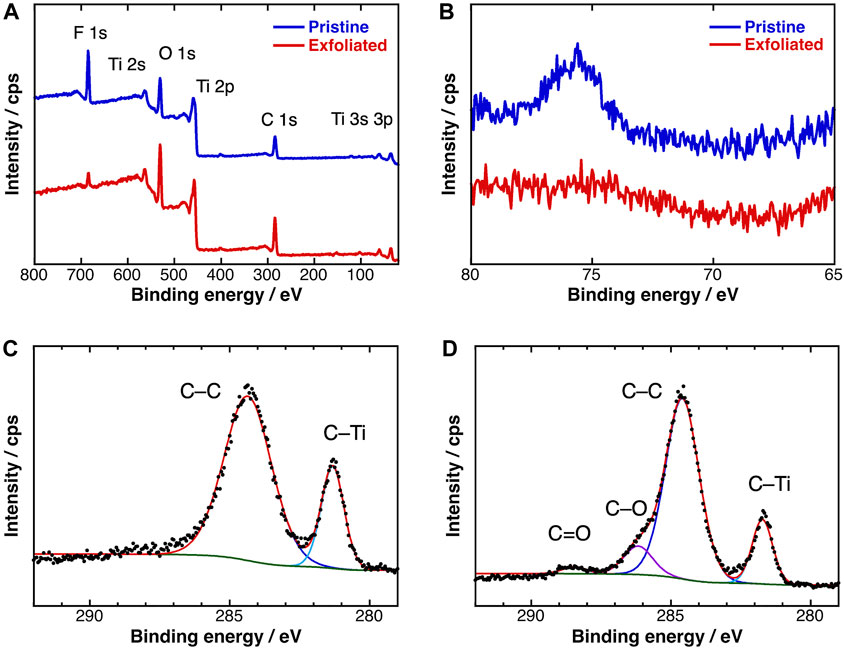
FIGURE 5. (A) Survey and (B) Al 2p XPS spectra of (blue) pristine and (red) exfoliated Ti3C2Tx MXene; C 1p XPS spectra of (C) pristine and (D) exfoliated Ti3C2Tx MXene.
Figures 5C,D display the HR C 1s spectra of the pristine and exfoliated MXenes, respectively. After separating the peaks, the major peaks at 281 and 284 eV were assigned to C–Ti and C–C bonds, respectively. In the spectrum of the exfoliated sample, the shoulder band at 286.2 eV originated from the carbon–oxygen single bond (C–O) of hydroxy groups, and the negligibly weak peak at 288.5 eV was attributable to carbon–oxygen double bonds (C=O). The generation of these oxidized carbon peaks suggest that partial chemical etching of the titanium atoms with Me4NOH exposed the inner carbon atoms. Such structural defects are consistent with the pinholes observed in the AFM image of the large-sized MXene sheets.
Application to Flexible Transparent Conductive Films
Finally, the transparent conductive MXene films were demonstrated in an application (Figure 6). The MXene films were formed on a PEN film by spraying of the dispersion diluted in methanol solvent. Figures 6A,B are typical photographs of the fabricated MXene films. The film was bendable (Figure 5A) and sufficiently electrically conductive (Figure 6B). Figure 6C plots the transmittance at a wavelength of 600 nm versus the average sheet resistance of various MXene films. The Rs at 78% transmittance was 2.5 × 104 Ω/sq., comparable to those of previous spray-coated examples (Hantanasirisakul et al., 2016; Zhou et al., 2021). The inset of Figure 6C plots T−0.5–1 as a function of Rs. The resulting bulk-type conductivity curve is consistent with those of other MXene films (Dillon et al., 2016).
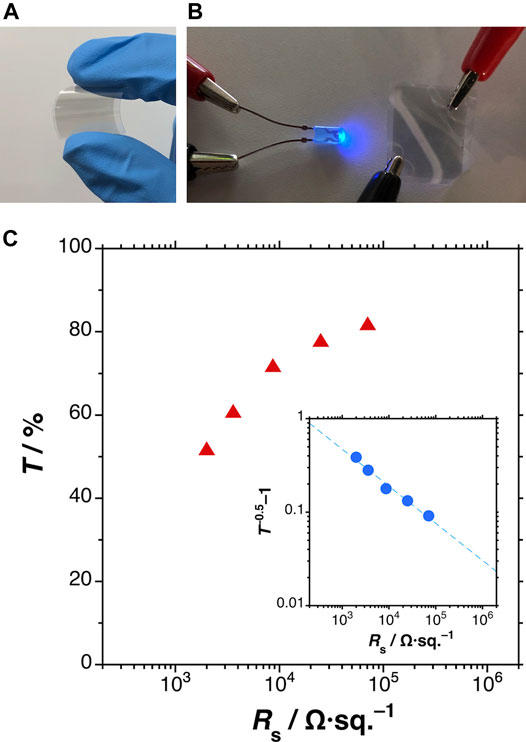
FIGURE 6. (A,B) Typical photographs of the fabricated MXene transparent conductive films; (C) plots of transmittance at 600 nm (main) and T−0.5–1 (inset) versus average sheet resistance of the MXene films.
Conclusion
In conclusion, we exfoliated Al-residual multilayered Ti3C2Tx MXene with Me4NOH in THF/H2O co-solvent. Ti3C2Tx, exfoliated into single layers without sonication, as confirmed in AFM observations and Raman spectroscopy. The conductivity was 100+ times higher than in films exfoliated under previously reported aqueous conditions. Moreover, transparent conductive films were formed on flexible PEN substrates using the synthesized Ti3C2Tx dispersion. XPS measurements of the exfoliated samples clarified that the Al atoms were removed and the Ti–F bonds transformed into Ti–OH bonds. The generated hydroxy groups, which can convert to a variety of functional groups, expand the feasibility of MXene as a conductive support material in catalysis, battery, and electrochemical applications. Thiol (Omachi et al., 2020) or amino groups (Matsumoto et al., 2020) can immobilize the metal clusters and nanomaterials, realizing novel conductive nanohybrid materials rather than the existing graphite or graphene examples. Further chemical modification of Ti3C2Tx toward electrode applications in battery systems is ongoing in our laboratory.
Data Availability Statement
The original contributions presented in the study are included in the article/Supplementary Material, further inquiries can be directed to the corresponding authors.
Author Contributions
HO and JH conceived the study. ES, MI, YS, JH, and HO developed the exfoliation conditions and performed the characterizations. RS and KA conducted the XRD measurement. YS and YM performed the conductive film applications. HO mainly prepared the manuscript and all authors discussed results, helped edit the manuscript and gave final approval for publication.
Funding
This work received financial support from JST CREST (19203618) and PRESTO (JPMJPR20B6), and JSPS Grant-in-Aid for Scientific Research (JP19H02168, 20KK0087).
Conflict of Interest
The authors declare that the research was conducted in the absence of any commercial or financial relationships that could be construed as a potential conflict of interest.
Publisher’s Note
All claims expressed in this article are solely those of the authors and do not necessarily represent those of their affiliated organizations, or those of the publisher, the editors and the reviewers. Any product that may be evaluated in this article, or claim that may be made by its manufacturer, is not guaranteed or endorsed by the publisher.
Acknowledgments
We thank Satomi Ogasawara (Nagoya Univ.) for supporting the experiments and Ayako Suzuki (Nagoya Univ.) for helping with the XPS measurements. We also acknowledge Prof. Kenichiro Itami for the use of the AFM and Raman equipment.
References
Dall’Agnese, Y., Taberna, P.-L., Gogotsi, Y., and Simon, P. (2015). Two-dimensional Vanadium Carbide (MXene) as Positive Electrode for Sodium-Ion Capacitors. J. Phys. Chem. Lett. 6, 2305–2309. doi:10.1021/acs.jpclett.5b00868
Dikin, D. A., Stankovich, S., Zimney, E. J., Piner, R. D., Dommett, G. H. B., Evmenenko, G., et al. (2007). Preparation and Characterization of Graphene Oxide Paper. Nature 448, 457–460. doi:10.1038/nature06016
Dillon, A. D., Ghidiu, M. J., Krick, A. L., Griggs, J., May, S. J., Gogotsi, Y., et al. (2016). Highly Conductive Optical Quality Solution‐Processed Films of 2D Titanium Carbide. Adv. Funct. Mater. 26, 4162–4168. doi:10.1002/adfm.201600357
Hantanasirisakul, K., Zhao, M. Q., Urbankowski, P., Halim, J., Anasori, B., Kota, S., et al. (2016). Fabrication of Ti 3 C 2 T X MXene Transparent Thin Films with Tunable Optoelectronic Properties. Adv. Electron. Mater. 2, 1600050. doi:10.1002/aelm.201600050
Intikhab, S., Natu, V., Li, J., Li, Y., Tao, Q., Rosen, J., et al. (2019). Stoichiometry and Surface Structure Dependence of Hydrogen Evolution Reaction Activity and Stability of MoxC MXenes. J. Catal. 371, 325–332. doi:10.1016/j.jcat.2019.01.037
Li, R., Zhang, L., Shi, L., and Wang, P. (2017). MXene Ti3C2: An Effective 2D Light-To-Heat Conversion Material. ACS Nano 11, 3752–3759. doi:10.1021/acsnano.6b08415
Liang, X., Garsuch, A., and Nazar, L. F. (2015). Sulfur Cathodes Based on Conductive MXene Nanosheets for High-Performance Lithium-Sulfur Batteries. Angew. Chem. Int. Ed. 54, 3907–3911. doi:10.1002/anie.201410174
Lukatskaya, M. R., Mashtalir, O., Ren, C. E., Dall’Agnese, Y., Rozier, P., Taberna, P. L., et al. (2013). Cation Intercalation and High Volumetric Capacitance of Two-Dimensional Titanium Carbide. Science 341, 1502–1505. doi:10.1126/science.1241488
Luo, J., Tao, X., Zhang, J., Xia, Y., Huang, H., Zhang, L., et al. (2016). Sn4+ Ion Decorated Highly Conductive Ti3C2 MXene: Promising Lithium-Ion Anodes with Enhanced Volumetric Capacity and Cyclic Performance. ACS Nano 10, 2491–2499. doi:10.1021/acsnano.5b07333
Mashtalir, O., Naguib, M., Mochalin, V. N., Dall’Agnese, Y., Heon, M., Barsoum, M. W., et al. (2013). Intercalation and Delamination of Layered Carbides and Carbonitrides. Nat. Commun. 4, 1716. doi:10.1038/ncomms2664
Matsumoto, K., Ueno, K., Hirotani, J., Ohno, Y., and Omachi, H. (2020). Fabrication of Carbon Nanotube Thin Films for Flexible Transistors by Using a Cross‐Linked Amine Polymer. Chem. Eur. J. 26, 6118–6121. doi:10.1002/chem.202000228
Naguib, M., Come, J., Dyatkin, B., Presser, V., Taberna, P.-L., Simon, P., et al. (2012). MXene: a Promising Transition Metal Carbide Anode for Lithium-Ion Batteries. Electrochemistry Commun. 16, 61–64. doi:10.1016/j.elecom.2012.01.002
Naguib, M., Kurtoglu, M., Presser, V., Lu, J., Niu, J., Heon, M., et al. (2011). Two-Dimensional Nanocrystals Produced by Exfoliation of Ti3AlC2. Adv. Mater. 23, 4248–4253. doi:10.1002/adma.201102306
Naguib, M., Mochalin, V. N., Barsoum, M. W., and Gogotsi, Y. (2014). 25th Anniversary Article: MXenes: a New Family of Two-Dimensional Materials. Adv. Mater. 26, 992–1005. doi:10.1002/adma.201304138
Naguib, M., Unocic, R. R., Armstrong, B. L., and Nanda, J. (2015). Large-scale Delamination of Multi-Layers Transition Metal Carbides and Carbonitrides "MXenes". Dalton Trans. 44, 9353–9358. doi:10.1039/c5dt01247c
Natu, V., Clites, M., Pomerantseva, E., and Barsoum, M. W. (2018). Mesoporous MXene Powders Synthesized by Acid Induced Crumpling and Their Use as Na-Ion Battery Anodes. Mater. Res. Lett. 6, 230–235. doi:10.1080/21663831.2018.1434249
Omachi, H., Inoue, T., Hatao, S., Shinohara, H., Criado, A., Yoshikawa, H., et al. (2020). Concise, Single‐Step Synthesis of Sulfur‐Enriched Graphene: Immobilization of Molecular Clusters and Battery Applications. Angew. Chem. Int. Ed. 59, 7836–7841. doi:10.1002/anie.201913578
Sarycheva, A., and Gogotsi, Y. (2020). Raman Spectroscopy Analysis of the Structure and Surface Chemistry of Ti3C2Tx MXene. Chem. Mater. 32, 3480–3488. doi:10.1021/acs.chemmater.0c00359
Seh, Z. W., Fredrickson, K. D., Anasori, B., Kibsgaard, J., Strickler, A. L., Lukatskaya, M. R., et al. (2016). Two-dimensional Molybdenum Carbide (MXene) as an Efficient Electrocatalyst for Hydrogen Evolution. ACS Energ. Lett. 1, 589–594. doi:10.1021/acsenergylett.6b00247
Verger, L., Xu, C., Natu, V., Cheng, H.-M., Ren, W., and Barsoum, M. W. (2019). Overview of the Synthesis of MXenes and Other Ultrathin 2D Transition Metal Carbides and Nitrides. Curr. Opin. Solid State. Mater. Sci. 23, 149–163. doi:10.1016/j.cossms.2019.02.001
Wang, X., Kajiyama, S., Iinuma, H., Hosono, E., Oro, S., Moriguchi, I., et al. (2015). Pseudocapacitance of MXene Nanosheets for High-Power Sodium-Ion Hybrid Capacitors. Nat. Commun. 6, 6544. doi:10.1038/ncomms7544
Wang, Y., Xu, Y., Hu, M., Ling, H., and Zhu, X. (2020). MXenes: Focus on Optical and Electronic Properties and Corresponding Applications. Nanophotonics 9, 1601–1620. doi:10.1515/nanoph-2019-0556
Xuan, J., Wang, Z., Chen, Y., Liang, D., Cheng, L., Yang, X., et al. (2016). Organic‐Base‐Driven Intercalation and Delamination for the Production of Functionalized Titanium Carbide Nanosheets with Superior Photothermal Therapeutic Performance. Angew. Chem. Int. Ed. 55, 14569–14574. doi:10.1002/anie.201606643
Yang, S., Zhang, P., Wang, F., Ricciardulli, A. G., Lohe, M. R., Blom, P. W. M., et al. (2018). Fluoride‐Free Synthesis of Two‐Dimensional Titanium Carbide (MXene) Using A Binary Aqueous System. Angew. Chem. Int. Ed. 57, 15491–15495. doi:10.1002/anie.201809662
Yun, T., Kim, J.-S., Shim, J., Choi, D. S., Lee, K. E., Koo, S. H., et al. (2017). Ultrafast Interfacial Self-Assembly of 2D Transition Metal Dichalcogenides Monolayer Films and Their Vertical and In-Plane Heterostructures. ACS Appl. Mater. Inter. 9, 1021–1028. doi:10.1021/acsami.6b11365
Zhou, B., Li, Z., Li, Y., Liu, X., Ma, J., Feng, Y., et al. (2021). Flexible Hydrophobic 2D Ti3C2Tx-Based Transparent Conductive Film with Multifunctional Self-Cleaning, Electromagnetic Interference Shielding and Joule Heating Capacities. Composites Sci. Tech. 201, 108531. doi:10.1016/j.compscitech.2020.108531
Keywords: MXene, exfoliation, tetramethylammonium base, Al etching, transparent conductive film
Citation: Saita E, Iwata M, Shibata Y, Matsunaga Y, Suizu R, Awaga K, Hirotani J and Omachi H (2022) Exfoliation of Al-Residual Multilayer MXene Using Tetramethylammonium Bases for Conductive Film Applications. Front. Chem. 10:841313. doi: 10.3389/fchem.2022.841313
Received: 22 December 2021; Accepted: 23 February 2022;
Published: 21 March 2022.
Edited by:
Pawel Gluchowski, Włodzimierz Trzebiatowski Institute of Low Temperature and Structure Research (PAS), PolandReviewed by:
Asif Shahzad, Kyungpook National University, South KoreaAnna Zielińska-Jurek, Gdansk University of Technology, Poland
Copyright © 2022 Saita, Iwata, Shibata, Matsunaga, Suizu, Awaga, Hirotani and Omachi. This is an open-access article distributed under the terms of the Creative Commons Attribution License (CC BY). The use, distribution or reproduction in other forums is permitted, provided the original author(s) and the copyright owner(s) are credited and that the original publication in this journal is cited, in accordance with accepted academic practice. No use, distribution or reproduction is permitted which does not comply with these terms.
*Correspondence: Jun Hirotani, hirotani.jun.7v@kyoto-u.ac.jp; Haruka Omachi, omachi@chem.nagoya-u.ac.jp
‡Present address: Jun Hirotani, Department of Micro Engineering, Graduate School of Engineering, Kyoto University, Kyoto, Japan
†These authors have contributed equally to this work and share first authorship