- 1School of Chemistry and Materials Science, Ludong University, Yantai, China
- 2School of Energy Materials and Chemical Engineering, Hefei University, Hefei, China
Biomass feedstocks are promising candidates of renewable clean energy. The development and utilization of biological energy is in line with the concept of sustainable development and circular economy. As an important platform chemical, γ-valerolactone (GVL) is often used as green solvent and biofuel additive. Regarding this, the efficient synthesis of GVL from biomass derivative furfural (FF) has attracted wide attention recently, However, suitable catalyst with appropriate acid-base sites is required due to the complex reaction progress. In this Mini Review, the research progress of catalytic synthesis of GVL from furfural by Zr/Hf-based catalysts was reviewed. The different effects of Lewis acid-base and Brønsted acid sites in the catalysts on each steps in the reaction process were discussed firstly. Then the effects of regulation of acid-base sites in the catalysts was also studied. Finally, the advantages and challenges of Zr/Hf-based catalysts in FF converted to GVL system were proposed.
Introduction
Although the exploration and utilization of fossil energy promote the development of human society, it also causes nonnegligible harm to the environment, which makes people focus on available energy to reduce dependence on fossil energy (Roman-Leshkov et al., 2007; Luterbacher et al., 2014; Zhang et al., 2019). Biomass, as the only renewable organic carbon source, has received extensive attention due to its abundance, cheapness, and availability (Li et al., 2014; Zhao et al., 2019; Li H. et al., 2020). A variety of valuable compounds (e.g., xylose, furfural, furfuryl alcohol, levulinic acid and its esters, and γ-valerolactone) can be obtained from biomass (Liu et al., 2015; Li F. et al., 2017; Li H. et al., 2017; Lingaiah, 2018; Li et al., 2019a; Luo et al., 2019). Among them, γ-valerolactone (GVL) has excellent physical and chemical properties such as high boiling point (207°C), low melting point (31°C), and low toxicity (LD50 = 8,800 mg/kg). It can be used as a green organic solvent in a variety of reactions, and has broad application prospects in the organic synthesis, biorefinery, and food industry (Yan et al., 2015; Ye et al., 2020b). In addition, GVL can be further converted into various valerate esters (these have been identified as new generation biofuels), which can be used to synthesize various biomass-based liquid fuels (Yu et al., 2019).
In recent years, the related research on the synthesis of GVL mainly focuses on the direct hydrogenation or catalytic transfer hydrogenation (CTH)with levulinic acid and its esters as substrates. Both noble metal (Ru, Rh, Pt, Pd, Au) catalysts and non-precious metal (Ni, Cu, Co.) catalystshave been used for the hydrogenation of LA to GVL (Yuan et al., 2013; Luo et al., 2014; Molleti et al., 2018). Obregon et al. studied the liquid phase hydrogenation of LA on Ni/Al2O3, after reacting at 250°C and 6.5 MPa H2 pressure for 2 h, The yiled of GVL reached 92%I. (Obregon et al., 2014). Although high yield was achieved by hydrogenation, however, the use of pressur-ized-hydrogen gas is often associated with potential explosion hazard, so the transfer hydrogenation strategy for the synthesis of GVL from LA has been developed. Numerous sup-ported Ru, Pd, Ni, and Cu catalysts were investigated to this reaction (Dutta et al., 2019; Ye et al., 2020b). Fu et al. firstly reported an non-precious skeletal Ni catalyst which could effective catalyze the reaction with i-PrOH as H-donor at room temperature over 9 h (Yang, et al., 2013). In addition, different hydrogen donors such as formic acid, hydrosilicon and alcohol have been exploited for this transformation, compare to other H-donors, the secure, safe and easily operated alcohol not only can act as H-donor, but also can serve as a solvent, furthermore, it can enhance the selec-tivity in the hydrogenation process, too(He et al., 2020a).Compared with levulinic acid, furfural (FF) is more available from biomass feedstocks, so the researchers considered FF directly as a feedstock for GVL production (Bui et al., 2013).
The conversion of FF to GVL requires a series of cascade reactions (Figure 1) such as CTH, etherification, ring-opening, partial hydrogenation, and cyclization reaction (Zhu et al., 2016). Such complex reaction processes require higher performance catalysts. Therefore, it is necessary to fully consider both the structure and acid-base properties of the catalyst to improve the catalyst activity. Since Zr/Hf-based catalysts show excellent catalytic performance in CTH reactions and are more economical than precious metals, more and more researchers applied them to the reaction of converting GVL from FF (Li et al., 2016; Wu et al., 2018; Zhou et al., 2019a; Wang et al., 2019).
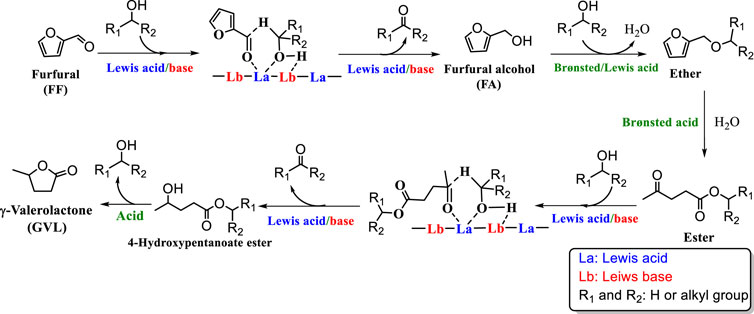
FIGURE 1. Possible reaction mechanism for the cascade conversion of biomass-derived furfural (FF) to γ-valerolactone (GVL).
At present, some excellent reviews are related to the synthesis of GVL, but most of these reviews focus on the synthesis of GVL with levulinic acid and its esters as the substrate (Dutta et al., 2019; Ye et al., 2020b). In this mini-review, the latest progress in the design of high-performance Zr/Hf-based catalysts for GVL production from FF. Some variables affecting the design of Zr/Hf-based catalysts such as the regulation of active sites of catalysts and the physical and chemical properties of catalysts were summarized. In addition, the reaction parameters in regulating conversion efficiency was discussed, providing insights for the development of efficient, economic, and sustainable catalytic systems that would be important for future research.
Effects of Catalyst Properties on the Synthesis of GVL From FF
In the system of FF for the synthesis of GVL, Zr/Hf-based catalysts showed good performance, as shown in Table 1. Zhu et al. first used Au/ZrO2 (providing Lewis acid-base sites) with ZSM-5 (providing Brønsted acid sites) to catalyze the conversion of FF to GVL (Zhu et al., 2016). The experimental results showed that when Au/ZrO2 was used as the catalyst, FF was almost completely converted to furfuryl alcohol (FA) (99.0% yield) rather than GVL. Similarly, no GVL was detected when only ZSM-5 was used as the catalyst. These results showed that the presence of both Lewis acid-base and Brønsted acid sites in the catalyst was necessary to successfully catalyze the conversion of FF to GVL. Rojas-Buzo et al. found that the prepared Hf-MOF-808 catalyst could successfully catalyze the CTH reaction of FF to FA and levulinic acid to obtain GVL, but could not directly catalyze the synthesis of GVL from FF(Rojas-Buzo et al., 2018). However, when the Hf-MOF-808/Al-β zeolite (containing Brønsted acid sites) combined catalyst was applied to this reaction, a good 75% yield of GVL was obtained at 120°C for 48 h. This result strongly shows that Brønsted acid is crucial to the ring-opening process involved in the conversion of FA to levulinate in this reaction process. Although combined catalyst system could improve the reaction yield, the catalyst preparation process becomes complicated and the production cost increases. To simplify the preparation process of the catalyst and increase reaction yield of GVL, the exploration of bifunctional catalysts containing both Lewis and Brønsted acid sites has attracted more and more attention. Bui et al. first used the physical mixture of Zr-Beta and mesoporous Al-MFI zeolite as Lewis acid and Brønsted acid catalysts to convert FF into GVL in one-pot (Bui et al., 2013). Later, Iglesias et al. synthesized a bifunctional catalyst containing both Lewis acid and Brønsted acid by loading ZrO2 on SBA-15 zeolite (Iglesias et al., 2018). The catalyst can control the strength of Lewis acid and Brønsted acid by changing the number of ZrO2 layers. Kinetic studies showed that the strength of Lewis acid in the catalyst had an important influence on the distribution of products. Strong Lewis acid sites promote etherification and isomerization of FA rather than MPV reduction. Srinivasa Rao et al. used the impregnation method to load different proportions of ZrO2 and phosphotungstic acid (TPA) on β-zeolite to further study the effect of Lewis/Brønsted acid content in the catalyst on the yield of GVL (Srinivasa Rao et al., 2021). The experimental results show that more Brønsted acid sites and fewer Lewis acid sites in the catalyst are more conducive to the production of levulinic acid ester rather than GVL. Therefore, the key to obtain high yield GVL is to control the Lewis acid-base and Brønsted acid sites with appropriate strength and number of bifunctional catalysts. Very recently, Tan et al. synthesised a variety of novel coordination organophosphate–Hf polymers from vinylphosphonic acid (VPA), p-toluenesulfonic acid (p-TSA), and HfCl. Specifically, VPA–Hf(1 : 1.5)-0.5 with an appropriate L/B acid ratio of 5.3 and was found to exhibit superior performance in the one-step conversion of furfural (FF) to γ-valerolactone (GVL) in a high yield of 81.0%, with a turnover frequency of 5.0 h−1. (Tan et al., 2022).
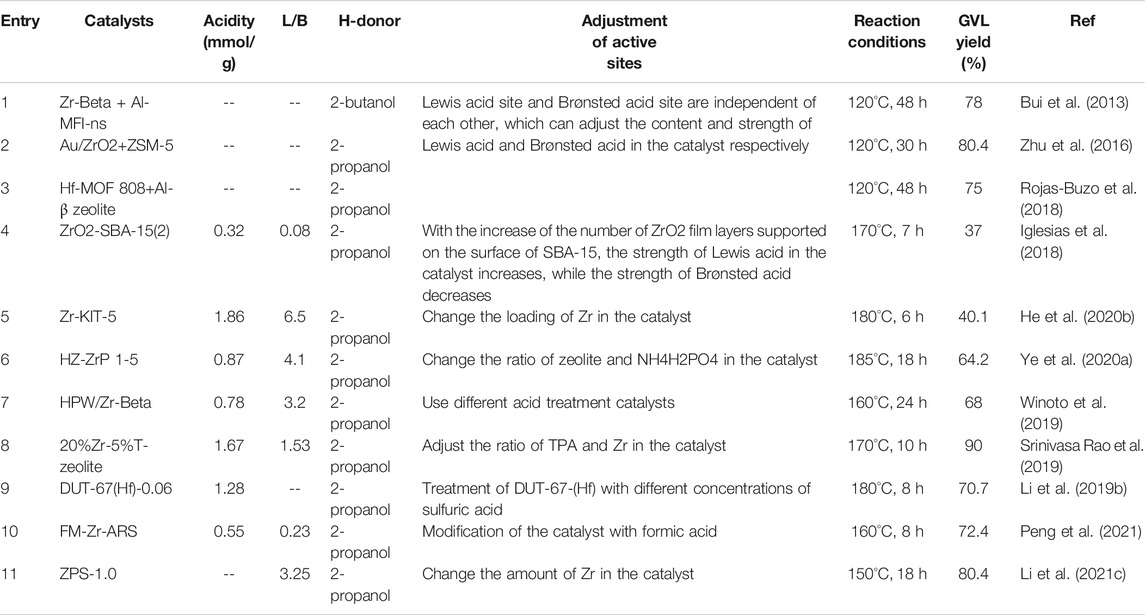
TABLE 1. Catalytic production of γ-valerolactone (GVL) from furfural (FF)over Zr/Hf-based catalysts.
Zeolite with a complex microporous structure has an open framework with regular pore size and appropriate size, which is conducive to mass transfer and is easy to adjust acidity (Wang et al., 2017; Wang et al., 2020; Peng et al., 2020; Chai et al., 2021). Since zeolite has these unique advantages, the existing catalysts for FF conversion to GVL are mostly prepared with various zeolites as supporter. These catalysts mainly change the content of Lewis acid sites in the catalysts by changing the metal loading, and different kinds and concentrations of acids are used to control the content of Brønsted acid in the catalysts (Srinivasa Rao et al., 2019; Winoto et al., 2019; He et al., 2020a; Ye et al., 2020a). He et al. adjusted the content of Lewis/Brønsted acid in the catalyst by adding different amounts of ZrOCl2·8H2O(He et al., 2020b). The more Zr is loaded in the catalyst, the higher the molar ratio of Lewis acid to Brønsted acid is. NH3-TPD results showed that with the increase of Zr loading in the catalyst, the total number of acid sites in the catalyst increased gradually. But excessive Zr loading will produce zirconia clusters, which will reduce the activity of the catalyst. Li et al. treated the catalyst by soaking DUT-67 (Hf) in different concentrations of sulfuric acid solution to change the content of Brønsted acid (Li W. et al., 2019). The results showed that with the increase of sulfuric acid concentration, the total content of acid sites in the catalyst increased continuously, but excessive Brønsted acid in the catalyst would lead to side reactions, which decreased the yield of GVL. SrinivasaRao et al. loaded phosphotungstic acid (TPA) and ZrO2 with different contents inside and outside the pores of SBA-15, respectively (Srinivasa Rao et al., 2021). Under the premise of keeping the total Lewis acid content in the catalyst unchanged, the molar ratio of Lewis acid to Brønsted acid in the catalyst was adjusted by controlling the amount of ZrO2 and TPA. The catalyst showed excellent catalytic activity, and the yield of GVL reached 90% at 170°C for 10 h.
In addition to using zeolite as a carrier, bifunctional materials prepared with ligands base on biomass derivatives are also applied to the conversion of FF to GVL. Using alizarin red S (ARS) as the ligand, Peng et al. synthesized FM-Zr-ARS catalyst by a simple hydrothermal method (Peng et al., 2021). The sulfonic acid group contained in ARS can acted as Brønsted acid for the ring-opening reaction, however, it cannot effectively regulate the relative content of different active sites in the catalyst. As a key step in the conversion of FF to GVL, CTH reaction is generally completed through a six-membered ring transition state. Lewis acid sites are usually used to activate H on the aldehyde group and C connected with the alcohol hydroxyl group. Lewis base sites are mainly used to activate the alcohol hydroxyl group, making H easier to remove. Finally, the transfer hydrogenation process is completed through the six-membered ring transition state (Li et al., 2018; Zhou et al., 2019b; Li et al., 2019c). Jarinya et al. found that Hf-UiO-66 has lower activation energy (13.5 kcal/molvs 14.9 kcal/mol) than Zr-UiO-66 based on density functional theory (DFT)(Sittiwong et al., 2021). It is due toHf having stronger Lewis acidity, Hf has better performance than Zr in CTH reaction under the same preparation conditions (Luo et al., 2014; Xie et al., 2016; Injongkol et al., 2017; Li X. et al., 2020). Tan et al. prepared a new coordination organic phosphate-Hf polymer VPA-Hf(1:1.5)-0.5, which showed good activity for one-pot cascade conversion of FF to GVL. By controlling the ratio of vinyl phosphoric acid, p-toluenesulfonic acid and HfCl4, the content of Lewis acid sites and B acid sites can be accurately adjusted, and the E factor value (0.19) shows that the conversion process mediated by the catalyst is ecologically friendly.
Effect of Reaction Parameters
The reaction can be carried out under mild conditions (120°C) when combined catalysts were used (Table 1). For bifunctional catalysts containing both Lewis acid and Brønsted acid, although the preparation of the catalyst is simpler and the cost is reduced, a higher reaction temperature (150–180°C) is often required to ensure the sufficient progress of the reaction. This may due to the independent active sites can also effectively reduce the adverse effects of steric hindrance in the reaction process, so the reaction can be carried out under mild conditions. However, the disadvantages such as excessively long reaction time and more tedious catalyst preparation process cannot be ignored. The key CTH reactions in the reaction process are completed by MPV reduction reaction, and more green and safe alcohols are usually used as H-donors to avoid the use of dangerous high-pressure H2and corrosive formic acid. In general, the β-H of secondary alcohols is easier to be removed from the transition state, so the hydrogen supply capacity of secondary alcohols is stronger than that of primary alcohols (Elsayed et al., 2020; Li J. et al., 2021). However, the steric hindrance of secondary alcohols will gradually increase with the extension of the carbon chain, and excessive steric hindrance is not conducive to the formation of stable transition states, thereby reducing the hydrogen supply capacity (Li M. et al., 2021; Li W. et al., 2021). Therefore, due to the small steric hindrance, 2-propanol was used as the H-donor to prepare GVL in most cases. In addition, the reusability of the catalyst is also an important aspect to evaluate the catalytic system. However, humus is usually formed during the reaction, which not only affects the carbon balance of the reaction system but also reduces the activity of the catalyst during recycling. Usually, calcination can remove the humus attached to the catalyst and restore the activity of the catalyst (Iglesias et al., 2018; Ye et al., 2020a; Tang et al., 2021). In addition, the catalyst may also have active site leaching during recycling, and it needs to be treated with acid before being put into the next recycling (Li W. et al., 2019).
Conclusion and Outlook
GVL is an important biomass derivative, which can be used as green solvents and biofuels. Highly efficient cascade conversion of FF to GVL presents great challenges due to complex reaction processes and high requirements for catalyst performance. In this mini-review, the influence of the catalyst preparation process on catalyst activity was reviewed, and the reaction parameters such as temperature and H- donor were also discussed. The acid-base properties of the catalyst have a great influence on its catalytic performance. The Lewis acid-base sites in the catalyst are mainly used to catalyze the CTH reaction, and the crucial ring-opening reaction needs to be carried out in the presence of Brønsted acid sites. There is no doubt that higher acid content in the catalyst can provide more active sites, but the imbalance of Lewis acid and Brønsted acid ratio can easily lead to undesirable side reactions. It may lead to carbon imbalance and GVL yield reduction, while the formation of humus attached to the catalyst will reduce the reusability of the catalyst.
Renewable biomass-based carbonaceous support catalysts have great potential for the green synthesis of GVL. Organic hybrid materials have proved to have good activity for CTH reaction, but the Brønsted acid sites are usually not sufficient to catalyze the ring-opening reaction. Therefore, how to improve the strength of Brønsted acid while ensuring the stability of the catalyst structure is the challenge that must be overcome for its application for FF synthesis to GVL. In addition, the accurate control of the strength and content of each active site in the catalyst can better control the reaction process, which is crucial to improving the yield of GVL. Due to the strong Lewis acidity of Zr/Hf materials, Zr/Hf-Based Catalysts showed high performance in the reaction of convert FF to GVL. However, most of the exiting catylic system still suffered from high temperature as well as not so excellent yield, so it is still a challegen to design novel and effecient catalyst for this reaction.
Author Contributions
WS and AL organized and original draft the manuscript; HL and XW contributed to reviewing and supervising part of the manuscript.
Funding
The study was funded by National Natural Science Foundation of China (No. 21806070), Natural Science Foundation of Shandong Province, China (No. ZR2018PB017) and University Natural Science Research Project of Anhui Province (No. KJ2019A0829 and KJ2019A0832).
Conflict of Interest
The authors declare that the research was conducted in the absence of any commercial or financial relationships that could be construed as a potential conflict of interest.
Publisher’s Note
All claims expressed in this article are solely those of the authors and do not necessarily represent those of their affiliated organizations, or those of the publisher, the editors and the reviewers. Any product that may be evaluated in this article, or claim that may be made by its manufacturer, is not guaranteed or endorsed by the publisher.
References
Bui, L., Luo, H., Gunther, W. R., and Román-Leshkov, Y. (2013). Domino Reaction Catalyzed by Zeolites with Brønsted and Lewis Acid Sites for the Production of γ-Valerolactone from Furfural. Angew. Chem. Int. Ed. 52, 8022–8025. doi:10.1002/anie.201302575
Chai, Y., Dai, W., Wu, G., Guan, N., and Li, L. (2021). Confinement in a Zeolite and Zeolite Catalysis. Acc. Chem. Res. 54, 2894–2904. doi:10.1021/acs.accounts.1c00274
Dutta, S., Yu, I. K. M., Tsang, D. C. W., Ng, Y. H., Ok, Y. S., Sherwood, J., et al. (2019). Green Synthesis of Gamma-Valerolactone (GVL) through Hydrogenation of Biomass-Derived Levulinic Acid Using Non-noble Metal Catalysts: A Critical Review. Chem. Eng. J. 372, 992–1006. doi:10.1016/j.cej.2019.04.199
Elsayed, I., Jackson, M. A., and Hassan, E. B. (2020). Hydrogen-Free Catalytic Reduction of Biomass-Derived 5-Hydroxymethylfurfural into 2,5-Bis(hydroxymethyl)furan Using Copper-Iron Oxides Bimetallic Nanocatalyst. ACS Sustain. Chem. Eng. 8, 1774–1785. doi:10.1021/acssuschemeng.9b05575
He, J., Li, H., Xu, Y., and Yang, S. (2020a). Dual Acidic Mesoporous KIT Silicates Enable One-Pot Production of γ-valerolactone from Biomass Derivatives via cascade Reactions. Renew. Energ. 146, 359–370. doi:10.1016/j.renene.2019.06.105
He, J., Xu, Y., Yu, Z., Li, H., Zhao, W., Wu, H., et al. (2020b). ZrOCl2 as a Bifunctional and In Situ Precursor Material for Catalytic Hydrogen Transfer of Bio-Based Carboxides. Sustain. Energ. Fuels 4, 3102–3114. doi:10.1039/c9se01313j
Iglesias, J., Melero, J. A., Morales, G., Paniagua, M., Hernández, B., Osatiashtiani, A., et al. (2018). ZrO2-SBA-15 Catalysts for the One-Pot cascade Synthesis of GVL from Furfural. Catal. Sci. Technol. 8, 4485–4493. doi:10.1039/c8cy01121d
Injongkol, Y., Maihom, T., Treesukul, P., Sirijaraensre, J., Boekfa, B., and Limtrakul, J. (2017). Theoretical Study on the Reaction Mechanism of Hydrogenation of Furfural to Furfuryl Alcohol on Lewis Acidic BEA Zeolites: Effects of Defect Structure and Tetravalent Metals Substitution. Phys. Chem. Chem. Phys. 19, 24042–24048. doi:10.1039/c7cp04229a
Li, F., France, L. J., Cai, Z., Li, Y., Liu, S., Lou, H., et al. (2017a). Catalytic Transfer Hydrogenation of Butyl Levulinate to γ-valerolactone over Zirconium Phosphates with Adjustable Lewis and Brønsted Acid Sites. Appl. Catal. B: Environ. 214, 67–77. doi:10.1016/j.apcatb.2017.05.013
Li, H., Bhadury, P. S., Riisager, A., and Yang, S. (2014). One-pot Transformation of Polysaccharides via Multi-Catalytic Processes. Catal. Sci. Technol. 4, 4138–4168. doi:10.1039/c4cy00711e
Li, H., Guo, H., Fang, Z., Aida, T. M., and Smith, R. L. (2020a). Cycloamination Strategies for Renewable N-Heterocycles. Green. Chem. 22, 582–611. doi:10.1039/C9GC03655E
Li, H., Guo, H., Su, Y., Hiraga, Y., Fang, Z., Hensen, E. J. M., et al. (2019a). N-formyl-stabilizing Quasi-Catalytic Species Afford Rapid and Selective Solvent-free Amination of Biomass-Derived Feedstocks. Nat. Commun. 10, 699. doi:10.1038/s41467-019-08577-4
Li, H., He, J., Riisager, A., Saravanamurugan, S., Song, B., and Yang, S. (2016). Acid-Base Bifunctional Zirconium N-Alkyltriphosphate Nanohybrid for Hydrogen Transfer of Biomass-Derived Carboxides. ACS Catal. 6, 7722–7727. doi:10.1021/acscatal.6b02431
Li, H., Li, Y., Fang, Z., and Smith, R. L. (2019c). Efficient Catalytic Transfer Hydrogenation of Biomass-Based Furfural to Furfuryl Alcohol with Recycable Hf-Phenylphosphonate Nanohybrids. Catal. Today 319, 84–92. doi:10.1016/j.cattod.2018.04.056
Li, H., Yang, T., and Fang, Z. (2018). Biomass-derived Mesoporous Hf-Containing Hybrid for Efficient Meerwein-Ponndorf-Verley Reduction at Low Temperatures. Appl. Catal. B: Environ. 227, 79–89. doi:10.1016/j.apcatb.2018.01.017
Li, H., Zhao, W., and Fang, Z. (2017b). Hydrophobic Pd Nanocatalysts for One-Pot and High-Yield Production of Liquid Furanic Biofuels at Low Temperatures. Appl. Catal. B: Environ. 215, 18–27. doi:10.1016/j.apcatb.2017.05.039
Li, J., Zhao, S., Li, Z., Liu, D., Chi, Y., and Hu, C. (2021a). Efficient Conversion of Biomass-Derived Levulinic Acid to γ-Valerolactone over Polyoxometalate@Zr-Based Metal-Organic Frameworks: The Synergistic Effect of Bro̷nsted and Lewis Acidic Sites. Inorg. Chem. 60, 7785–7793. doi:10.1021/acs.inorgchem.1c00185
Li, M., Liu, Y., Lin, X., Tan, J., Yang, S., and Li, H. (2021b). One-step Upgrading of Bio-Based Furfural to γ-valerolactone via HfCl4-Mediated Bifunctional Catalysis. RSC Adv. 11, 35415–35424. doi:10.1039/d1ra05637a
Li, W., Cai, Z., Li, H., Shen, Y., Zhu, Y., Li, H., et al. (2019b). Hf-based Metal Organic Frameworks as Bifunctional Catalysts for the One-Pot Conversion of Furfural to γ-valerolactone. Mol. Catal. 472, 17–26. doi:10.1016/j.mcat.2019.04.010
Li, W., Li, M., Liu, H., Jia, W., Yu, X., Wang, S., et al. (2021c). Domino Transformation of Furfural to γ-valerolactone over SAPO-34 Zeolite Supported Zirconium Phosphate Catalysts with Tunable Lewis and Brønsted Acid Sites. Mol. Catal. 506, 111538. doi:10.1016/j.mcat.2021.111538
Li, X., Du, Z., Wu, Y., Zhen, Y., Shao, R., Li, B., et al. (2020b). A Novel Hafnium-Graphite Oxide Catalyst for the Meerwein-Ponndorf-Verley Reaction and the Activation Effect of the Solvent. RSC Adv. 10, 9985–9995. doi:10.1039/c9ra10795a
Lingaiah, N. (2018). One Pot Selective Transformation of Biomass Derived Chemicals towards Alkyl Levulinates over Titanium Exchanged Heteropoly Tungstate Catalysts. Catal. Today 309, 269–275. doi:10.1016/j.cattod.2017.05.040
Liu, J., Li, H., Liu, Y.-C., Lu, Y.-M., He, J., Liu, X.-F., et al. (2015). Catalytic Conversion of Glucose to 5-hydroxymethylfurfural over Nano-Sized Mesoporous Al2O3-B2o3 Solid Acids. Catal. Commun. 62, 19–23. doi:10.1016/j.catcom.2015.01.008
Luo, H. Y., Consoli, D. F., Gunther, W. R., and Román-Leshkov, Y. (2014). Investigation of the Reaction Kinetics of Isolated Lewis Acid Sites in Beta Zeolites for the Meerwein-Ponndorf-Verley Reduction of Methyl Levulinate to γ-valerolactone. J. Catal. 320, 198–207. doi:10.1016/j.jcat.2014.10.010
Luo, Y., Li, Z., Li, X., Liu, X., Fan, J., Clark, J. H., et al. (2019). The Production of Furfural Directly from Hemicellulose in Lignocellulosic Biomass: A Review. Catal. Today 319, 14–24. doi:10.1016/j.cattod.2018.06.042
Luterbacher, J. S., Rand, J. M., Alonso, D. M., Han, J., Youngquist, J. T., Maravelias, C. T., et al. (2014). Nonenzymatic Sugar Production from Biomass Using Biomass-Derived γ-Valerolactone. Science 343, 277–280. doi:10.1126/science.1246748
Molleti, J., Tiwari, M. S., and Yadav, G. D. (2018). Novel Synthesis of Ru/OMS Catalyst by Solvent-free Method: Selective Hydrogenation of Levulinic Acid to γ-valerolactone in Aqueous Medium and Kinetic Modelling. Chem. Eng. J. 334, 2488–2499. doi:10.1016/j.cej.2017.11.125
Obregón, I., Corro, E., Izquierdo, U., Requies, J., and Arias, P. L. (2014). Levulinic Acid Hydrogenolysis on Al2O3-Based Ni-Cu Bimetallic Catalysts. Chin. J. Catal. 35, 656–662. doi:10.1016/S1872-2067(14)60051-6
Peng, P., Gao, X.-H., Yan, Z.-F., and Mintova, S. (2020). Diffusion and Catalyst Efficiency in Hierarchical Zeolite Catalysts. Natl. Sci. Rev. 7, 1726–1742. doi:10.1093/nsr/nwaa184
Peng, Q., Wang, H., Xia, Y., and Liu, X. (2021). One-pot Conversion of Furfural to Gamma-Valerolactone in the Presence of Multifunctional Zirconium Alizarin Red S Hybrid. Appl. Catal. A: Gen. 621, 118203. doi:10.1016/j.apcata.2021.118203
Rojas-Buzo, S., García-García, P., and Corma, A. (2018). Catalytic Transfer Hydrogenation of Biomass-Derived Carbonyls over Hafnium-Based Metal-Organic Frameworks. ChemSusChem 11, 432–438. doi:10.1002/cssc.201701708
Román-Leshkov, Y., Barrett, C. J., Liu, Z. Y., and Dumesic, J. A. (2007). Production of Dimethylfuran for Liquid Fuels from Biomass-Derived Carbohydrates. Nature 447, 982–985. doi:10.1038/nature05923
Sittiwong, J., Boonmark, S., Nunthakitgoson, W., Maihom, T., Wattanakit, C., and Limtrakul, J. (2021). Density Functional Investigation of the Conversion of Furfural to Furfuryl Alcohol by Reaction with I-Propanol over UiO-66 Metal-Organic Framework. Inorg. Chem. 60, 4860–4868. doi:10.1021/acs.inorgchem.0c03764
Srinivasa Rao, B., Krishna Kumari, P., Koley, P., Tardio, J., and Lingaiah, N. (2019). One Pot Selective Conversion of Furfural to γ-valerolactone over Zirconia Containing Heteropoly Tungstate Supported on β-zeolite Catalyst. Mol. Catal. 466, 52–59. doi:10.1016/j.mcat.2018.12.024
Srinivasa Rao, B., Yogita, D., Dhana Lakshmi, P. K., and Lingaiah, N. (2021). Influence of Metal Oxide and Heteropoly Tungstate Location in Mesoporous Silica towards Catalytic Transfer Hydrogenation of Furfural to γ-valerolactone. Sustain. Energ. Fuels 5, 3719–3728. doi:10.1039/d1se00340b
Tan, J., Liu, Y., Li, M., Li, H., and Yang, S. (2022). One-step Catalytic Upgrading of Bio-Based Furfural to γ-valerolactone Actuated by Coordination Organophosphate-Hf Polymers. Sustain. Energ. Fuels 6, 484–501. doi:10.1039/D1SE01476E
Tang, B., Li, S., Song, W.-C., Li, Y., and Yang, E.-C. (2021). One-pot Transformation of Furfural into γ-valerolactone Catalyzed by a Hierarchical Hf-Al-USY Zeolite with Balanced Lewis and Brønsted Acid Sites. Sustain. Energ. Fuels 5, 4724–4735. doi:10.1039/d1se00942g
Wang, T., Hu, A., Xu, G., Liu, C., Wang, H., and Xia, Y. (2019). Porous Zr-Thiophenedicarboxylate Hybrid for Catalytic Transfer Hydrogenation of Bio-Based Furfural to Furfuryl Alcohol. Catal. Lett. 149, 1845–1855. doi:10.1007/s10562-019-02748-0
Wang, Y., Wu, Q., Meng, X., and Xiao, F.-S. (2017). Insights into the Organotemplate-free Synthesis of Zeolite Catalysts. Engineering 3, 567–574. doi:10.1016/j.eng.2017.03.029
Winoto, H. P., Fikri, Z. A., Ha, J.-M., Park, Y.-K., Lee, H., Suh, D. J., et al. (2019). Heteropolyacid Supported on Zr-Beta Zeolite as an Active Catalyst for One-Pot Transformation of Furfural to γ-valerolactone. Appl. Catal. B: Environ. 241, 588–597. doi:10.1016/j.apcatb.2018.09.031
Wu, W., Li, Y., Li, H., Zhao, W., and Yang, S. (2018). Acid–Base Bifunctional Hf Nanohybrids Enable High Selectivity in the Catalytic Conversion of Ethyl Levulinate to γ-Valerolactone. Catalysts 8, 264–278. doi:10.3390/catal8070264
Xie, C., Song, J., Zhou, B., Hu, J., Zhang, Z., Zhang, P., et al. (2016). Porous Hafnium Phosphonate: Novel Heterogeneous Catalyst for Conversion of Levulinic Acid and Esters into γ-Valerolactone. ACS Sustain. Chem. Eng. 4, 6231–6236. doi:10.1021/acssuschemeng.6b02230
Yan, K., Yang, Y., Chai, J., and Lu, Y. (2015). Catalytic Reactions of Gamma-Valerolactone: A Platform to Fuels and Value-Added Chemicals. Appl. Catal. B: Environ. 179, 292–304. doi:10.1016/j.apcatb.2015.04.030
Yang, Z., Huang, Y.-B., Guo, Q.-X., and Fu, Y. (2013). RANEY Ni Catalyzed Transfer Hydrogenation of Levulinate Esters to γ-valerolactone at Room Temperature. Chem. Commun. 49, 5328–5330. doi:10.1039/c3cc40980e
Ye, L., Han, Y., Bai, H., and Lu, X. (2020a). HZ-ZrP Catalysts with Adjustable Ratio of Brønsted and Lewis Acids for the One-Pot Value-Added Conversion of Biomass-Derived Furfural. ACS Sustain. Chem. Eng. 8, 7403–7413. doi:10.1021/acssuschemeng.0c01259
Ye, L., Han, Y., Feng, J., and Lu, X. (2020b). A Review about GVL Production from Lignocellulose: Focusing on the Full Components Utilization. Ind. Crops Prod. 144, 112031. doi:10.1016/j.indcrop.2019.112031
Yu, Z., Lu, X., Liu, C., Han, Y., and Ji, N. (2019). Synthesis of γ-valerolactone from Different Biomass-Derived Feedstocks: Recent Advances on Reaction Mechanisms and Catalytic Systems. Renew. Sustain. Energ. Rev. 112, 140–157. doi:10.1016/j.rser.2019.05.039
Yuan, J., Li, S.-S., Yu, L., Liu, Y.-M., Cao, Y., He, H.-Y., et al. (2013). Copper-based Catalysts for the Efficient Conversion of Carbohydrate Biomass into γ-valerolactone in the Absence of Externally Added Hydrogen. Energy Environ. Sci 6, 3308–3313. doi:10.1039/c3ee40857d
Zhang, H., Li, H., Hu, Y., Venkateswara Rao, K. T., Xu, C., and Yang, S. (2019). Advances in Production of Bio-Based Ester Fuels with Heterogeneous Bifunctional Catalysts. Renew. Sustain. Energ. Rev. 114, 109296. doi:10.1016/j.rser.2019.109296
Zhao, W., Chi, X., Li, H., He, J., Long, J., Xu, Y., et al. (2019). Eco-friendly Acetylcholine-Carboxylate Bio-Ionic Liquids for Controllable N-Methylation and N-Formylation Using Ambient CO2 at Low Temperatures. Green. Chem. 21, 567–577. doi:10.1039/c8gc03549k
Zhou, S., Dai, F., Chen, Y., Dang, C., Zhang, C., Liu, D., et al. (2019a). Sustainable Hydrothermal Self-Assembly of Hafnium-Lignosulfonate Nanohybrids for Highly Efficient Reductive Upgrading of 5-hydroxymethylfurfural. Green. Chem. 21, 1421–1431. doi:10.1039/c8gc03710h
Zhou, S., Dai, F., Xiang, Z., Song, T., Liu, D., Lu, F., et al. (2019b). Zirconium-lignosulfonate Polyphenolic Polymer for Highly Efficient Hydrogen Transfer of Biomass-Derived Oxygenates under Mild Conditions. Appl. Catal. B: Environ. 248, 31–43. doi:10.1016/j.apcatb.2019.02.011
Keywords: biomass, furfural, γ-valerolactone, Zr/Hf-based catalysts, active site regulation
Citation: Sun W, Li H, Wang X and Liu A (2022) Cascade Upgrading of Biomass-Derived Furfural to γ-Valerolactone Over Zr/Hf-Based Catalysts. Front. Chem. 10:863674. doi: 10.3389/fchem.2022.863674
Received: 27 January 2022; Accepted: 15 February 2022;
Published: 07 March 2022.
Edited by:
Hu Li, Guizhou University, ChinaReviewed by:
Jian He, Jishou University, ChinaZehui Zhang, South-Central University for Nationalities, China
Copyright © 2022 Sun, Li, Wang and Liu. This is an open-access article distributed under the terms of the Creative Commons Attribution License (CC BY). The use, distribution or reproduction in other forums is permitted, provided the original author(s) and the copyright owner(s) are credited and that the original publication in this journal is cited, in accordance with accepted academic practice. No use, distribution or reproduction is permitted which does not comply with these terms.
*Correspondence: Wenjuan Sun, c3Vud2VuanVhbkBsZHUuZWR1LmNu; Anqiu Liu, bGl1YXFAaGZ1dS5lZHUuY24=