- 1School of Elementary Education, Changsha Normal University, Changsha, China
- 2National and Local Joint Engineering Laboratory for New Petro-Chemical Materials and Fine Utilization of Resources, Key Laboratory of the Assembly and Application of Organic Functional Molecules of Hunan Province, College of Chemistry and Chemical Engineering, Hunan Normal University, Changsha, China
The development of efficient heterogeneous catalytic system to convert plentiful biomass to renewable bio-chemicals is urgent need. Titanate nanotubes-based materials obtained from hydrothermal treatment have been reported as low-cost and efficient catalytic materials in chemical syntheses for bio-based chemicals production with interesting catalytic performance. This mini-review expressly revealed the significance and potential of using titanate nanotubes based material as sustainable and environmentally benign solid catalysts/supports for synthesis of various bio-based chemicals, including glycerol-derived solketal, jet fuel range alkanes precursors, biomass-derived esters, aldehydes, aromatic compounds and so on. From the current knowledge on titanate nanotubes-based material via hydrothermal method here summarized, the future lines of research in the field of catalysis/supports for bio-based chemicals production are outlined.
Introduction
At the present moment, fossil fuels are the primary sources of energy for humankind. However, the use of fossil fuels often associated with the concerns, such as price fluctuation, long-term availability, and growing environmental effects (Brockway et al., 2019). Besides, the quest for global energy and chemicals needs will be in high demand due to the rapidly developments of economic and socioeconomic. Hence, transforming renewable energy into alternative fuels and chemicals is an essential and indispensable pathway (Gielen et al., 2019; Stančin et al., 2020). Biomass is an abundant renewable and cleaner resource, which can be converted into a wide range of various fuel grade molecules and bio-chemicals as alternatives to fossil-derived products (Li et al., 2017; Schutyser et al., 2018; Okolie et al., 2021; Ashokkumar et al., 2022). In this situation, new chemical technology and efficient catalysts to convert plentiful biomass to renewable bio-chemicals is urgent need.
Titanate nanotubes (TNTs), a typical of Ti-based material, have attracted extensive researches due to its novel properties such as chemical stability, large surface area, non-toxicity, and relatively hydrophobic nature, which have shown great potential not only as catalysts but also as supports. A lot of literatures have described the synthesis of titanate nanotubes by various methods such as hydrothermal treatment (Kasuga et al., 1998; Kasuga et al., 1999), template-assisted method (Zhang et al., 2001), and the anodizing of titanium metal (Gong et al., 2001). It is worth noted that TNTs obtained by hydrothermal treatment of TiO2 nanoparticles in the absence of a template and at low temperature (120–150°C), which has received significant attention (Bavykin et al., 2006). In recent years, many articles and reviews have covered the applications of hydrothermally synthesized TNTs materials in photoelectrochemial reactions (Arifin et al., 2021), photocatalytic (Ji et al., 2022), dye-sensitized solar cells (Madurai Ramakrishnan et al., 2020; Souza et al., 2021), adsorbents (Li et al., 2021) and other interesting applications (Yao et al., 2020). In addition, the structural, optical, thermal and morphological properties of TNTs synthesized by conventional hydrothermal method were systematically discussed in other reviews (Ou and Lo, 2007; Muniyappan et al., 2017; Rempel et al., 2021).
Kitano and co-workers found that the TNTs exhibited excellent catalytic performance in Friedel-Crafts alkylation and the 5-hydroxymethylfurfural production (Kitano et al., 2010). This finding may potentially open up new catalytic applications of the titanate nanotubes for organic transformations and biomass conversion. In the past few years, some studies have been reported the use of TNTs in various acid (base) catalyzed organic chemical transformation (Kitano et al., 2013; Wada et al., 2013; Li et al., 2015; Reddy et al., 2015). This mini-review focuses on TNTs based material as solid catalysts/supports with the potential application for the bio-based chemicals production (Figure 1).
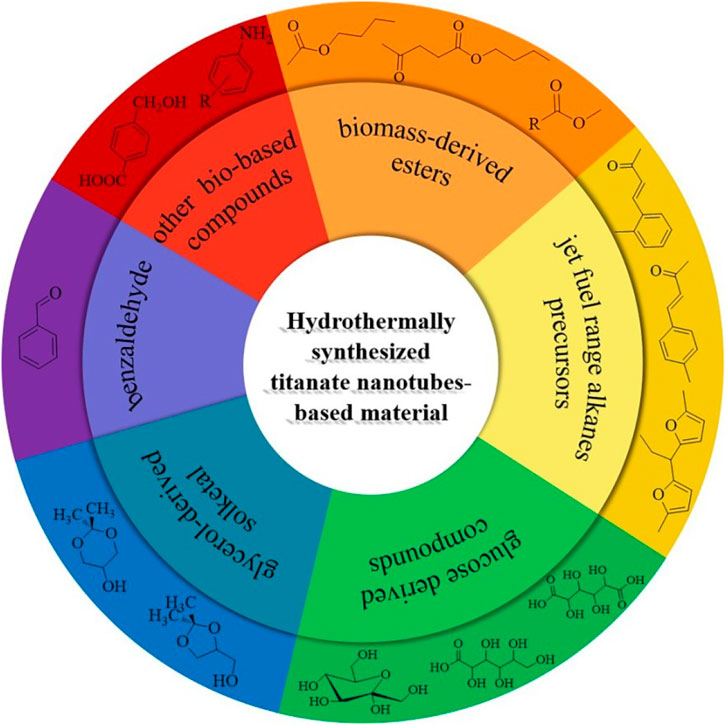
FIGURE 1. The schematic of production of typical biomass derived chemicals over titanate nanotubes-based catalyst.
Biomass Derived Chemicals Production
Production of Glycerol-Derived Solketal
The acetalization reaction has been widely studied because they are important and efficient processes to convert aldehydes or ketones into high-valued compounds. The glycerol-derived solketal as oxygenated compound may be incorporate into additive for standard diesel fuel as well as decrease the emission of hydrocarbons and particulate species (Talebian-Kiakalaieh et al., 2018). De Carvalho et al. reported the acetalization of glycerol using the protonated titanate nanotubes as a solid catalyst (de Carvalho et al., 2017). The synthesis time of TNTs and the role of structure on the catalytic performance were systematically investigated. The best performance towards glycerol conversion was achieved by the TNTs synthesized at 72 h with high textural, morphological and acidity properties. The glycerol conversion was 44.4% and the selectivity toward the desired products (solketal and acetal) was observed to be more than 98% at 50°C and an acetone/glycerol molar ratio of 1. Decoration of transition and rare earth metal nanoparticles on titanate nanotubes has a considerable effect on the number of Brønsted and Lewis acidity sites (Camposeco et al., 2016; Wada et al., 2016). These researches demonstrated that various metals (Ag, Au, Ce, Fe, Mn, Pd, Pt, V, W and Nb) obviously improve the Brønsted acidity of TNTs except for La; some metals such as Pt, Mn and W could enhance Lewis acidity of TNTs; the addition of transition metals (V, Mn, W and Fe) increased remarkably the total acidity of TNTs. In another study, TNTs incorporated with metals (Pt, Co, Ni) were synthesized and applied in acetalization of glycerol with acetone (Gomes et al., 2018). Interestingly, the Pt-containing titanate nanotubes (Pt-TNT) catalyst has high catalytic activity, affording 46.7% of glycerol conversion with 10% selectivity to solketal, whereas the Co and Ni-incorporated titanate nanotubes exhibited relativity low catalytic activity (glycerol conversion <5%). Structural properties of various titanate nanotubes-based acid catalysts are listed Table 1. The suitable tuning of pore-structure and proper surface acidity of Pt-TNT were contributed to the resulting in a stable solid for this reaction (Gomes et al., 2018). It is noteworthy that leaching of Co and Ni species is reported as the main mechanism for the catalyst deactivation for Co-containing titanate nanotubes and Ni-containing titanate nanotubes. This demonstrated that the catalytic activity and recyclability of TNTs could be tuned by incorporation of a suitable metal.
Jet Fuel Range Alkanes Precursors Synthesis
Aldol condensation approach is the very important for synthesis of high-quality bio-fuels (He et al., 2021). Protonated titanate nanostructures used as solid acid catalysts exhibited the excellent catalytic activity in the condensation reaction between various benzaldehyde derivatives and cyclohexanone (Sluban et al., 2017). A higher conversion rate was obtained over TNTs as compared to titanate nanoribbons, demonstrating the beneficial role of nanotube morphology. The catalyst showed a remarkable stability since no significant decrease in the catalytic activity in five cycles. In addition, protonated titanate nanotubes did not require any activation prior to the reaction. Recently, protonated titanate nanotubes catalyst displayed much higher activity for acid-catalyzed aldol condensation of methyl benzaldehyde and acetone, two platform compounds obtained from lignocelluloses (Timothy et al., 2020). Around 76% yield of jet fuel precursors, namely 4-(o-tolyl)but-3-en-2-one, was obtained under the optimum reaction conditions. After the hydrodeoxygenation (HDO) of jet fuel precursors in cyclohexane under mild conditions (403K, 5MPa, 2 h), high yields ( ∼ 90%) of dicycloalkanes were achieved. They discovered the catalytic activity of protonated titanate nanotubes was higher than TiO2 P25 and titanate nanowire under the same conditions. It is suggested that the special nanotube morphology, bigger surface area, higher acid site amount and acid strength could be considered as the reasons for the good catalytic performance of protonated titanate nanotube. It is also found that the protonated titanate nanotubes catalyst was stable and could be repeatedly used for five runs without significant deactivation.
The protonated titanate nanotubes as a good solid catalyst was applied for the hydroxyalkylation/alkylation (HAA) of 2-methylfuran (2-MF) with n-butanal from lignocelluloses to synthesize diesel and jet fuel range alkanes precursors. Compared to other inorganic solid acids such as SO42-/ZrO2, ZrP and H-ZSM-5, protonated titanate nanotubes has higher catalytic activity, giving 77% yield of HAA product under mild reaction conditions (Li et al., 2015). The protonated titanate nanotubes was also effective for the catalytic HAA of 2-MF with other lignocellulosic carbonyl compounds, such as furfural, acetone and mesityl oxide. The outstanding catalytic performance of protonated titanate nanotubes for the HAA of 2-MF and n-butanal can be explained by the following reasons: 1) the protonated titanate nanotubes has higher specific surface area, which is beneficial for the adsorption of reactants, 2) the transformation of commercial TiO2 P25 to protonated titanate nanotubes leads to the higher acidity (the amount of acid sites and the generation of strong acid sites), and 3) the generation of Brönsted acid sites may be beneficial to the HAA reaction of 2-MF and n-butanal.
Preparation of Biomass-Derived Esters
Some researchers have suggested that the sodium titanate nanotubes is an effcient heterogenous base catalyst in the transesterification reactions, which is the most common route for biodiesel production (Hernández-Hipólito et al., 2014). Recently, the sodium titanates were used as catalysts in the transesterification of pure and cooked oils into biodiesel (Zaki et al., 2019). The biodiesel yield was found to be 95.9% at 80°C for 2 h; the authors discovered that the catalyst showed high activity for cooked oil conversion, with yields of 96.0, 96.0, and 93.58% for the first, second, and third uses of oil, respectively. The authors found that the transesterification reaction preferentially proceeded via dual-site Langmuir-Hinshelwood mechanism with the aid of the Density Functional Theory (DFT), Monte Carlo (MC) simulation, and molecular dynamics simulation. Furthermore, the transesterification reaction kinetics followed a pseudo-first-order kinetics model. Simiarlly, the sodium titanate catalysts were prepared by sol–gel hydrothermal method, and the synthesis parameters of sodium titanates on the catalysts activity in soybean oil conversion to biodiesel were discussed using a factorial design (Machorro López et al., 2021). Combing the characterization results and catalytic results, the authors pointed out that trititanate was the most efficient in the conversion of soybean oil to biodiesel, achieving around 80% conversion. Doping metal ions on sodium titanate nanotubes may be an important strategy to improve the catalytic acitivity. For example, sodium titanate nanotubes doped with potassium proved as a efficient catalyst for transesterification of soybean oil with methanol (Hernández-Hipólito et al., 2015; Martínez-Klimova et al., 2016). Recently, the promoting role of sodium carbonate addition to sodium titanate nanotubes were reported (Martínez-Klimov et al., 2020). Incorporation of Na2CO3 (3–10 wt%) to sodium titanate nanotubes can increase the amount of strong basic sites in the catalysts. A synergetic effect between Na2CO3 and sodium titanate nanotubes was proposed for the increase in the amount of strong basic sites, resulting in an excellent catalytic performance in transformation of triglycerides to methyl esters (97% yield). Interestingly, in other important studies, lipase immobilized onto the sodium titanate nanotubes have recently been employed in the fatty acid methyl esters production (Nady et al., 2020; El-Kady et al., 2021). The immobilized lipase gave a high fatty acid methyl esters yield of 83.5% at short time of 90 min and showed the enhanced recycling stability for ten consecutive cycles.
Esterification is the most common reaction for biomass conversion and high-valued chemicals production (Zhang et al., 2019). Xu et al. found the catalytic performance of the titanate nanotubes is significantly higher than titanate nanosheets and layered H2TiO7 in esterification of acetic acid with n-butanol (Xu et al., 2020). The authors proposed that the surface acid characteristics and confinement effect were responsible for the high catalytic activity of titanate nanotubes. This clearly reveals that the microstructure is important to the catalytic activity. The finite amount of catalytic sites on the TNTs, however, would hamper in practical applications. It is noteworthy that TNTs prepared by hydrothermal method with abundant hydroxyl groups. Thus, the potential to modify TiO2 nanotubes to incorporate organosulfonic acid groups open new perspectives for their use as solid acid catalysts in a variety of reactions. Our groups reported various titanate nanotubes-bonded organosulfonic acid catalysts for the esterification of biomass-derived levulinic acid with n-butyl alcohol (Zhou et al., 2018; Zhou et al., 2019; Zhou et al., 2022a). Up to 98.9% yield of n-butyl levulinate was obtained under the optimal reaction conditions. In these hybrid catalysts the acid sites are covalently linked on titanate nanoutbes, therefore, they showed an excellent reusability with a slight decrease in several runs. On the other hand, the incorporation of organic groups on the TNTs can tune surface hydrophobicity property. Recently, in order to recycle heterogeneous acid catalysts from the reaction mixture, a new solid acid catalyst Fe3O4@TNTs-SO3H was successfully synthesized and applied to esterification of renewable levulinic acid to fuel additive n-butyl levulinate (Mao et al., 2020). This catalyst was demonstrated to show high catalytic activity, affording n-butyl levulinate with a yield of 94.6% under optimum conditions; the catalyst could be reused for 6 times. It is believed that titanate nanotubes can be rationally designed via post-synthesis strategy to prepare solid acid catalysts with excellent performance.
The alcoholysis process has been reported as a highly reactive method for conversion of lignocellulose to valuable chemicals (Zhu et al., 2017). Sulfonic acid functionalized TiO2 nanotubes were prepared by the sulphonation reaction of hydrothermally synthesized Titanate nanotubes using chlorosulfonic acid as the sulfating agent in our recent work (Zhou et al., 2022b). About 79.9% yield of n-butyl levulinate was achieved in the alcoholysis of the furfuryl alcohol with n-butanol under mild conditions. In addition, the catalysts showed a stable catalytic performance after four consecutive cycles. The covalently linked –SO3H groups on the TNTs surface was responsible for the stability of catalyst.
Synthesis of Glucose Derived Compounds
Kumar and co-workers have recently reported a sodium titanate nanotubes as a potential Lewis base catalyst for large-scale demonstration of glucose isomerization to fructose in aqueous media (Kumar et al., 2018). In this work, the glucose conversion could be reached with 31.26% fructose yield and 65.26% selectivity under relatively lower operating conditions for 15 min or less. They found that the presence of large basic sites in sodium titanate nanotubes was contribute to the higher glucose conversion. Additionally, the catalyst could be effciently recycled and regenerated by a simple NaOH treatment. On the contray, protonated titanate nanotubes was reported as solid acid catalyst for conversion of glucose into HMF via isomerization and dehydration process, giving the moderate yield of HMF (Kitano et al., 2010). Recently, protonated titanate nanotubes exhibit relatively high catalytic performance for isomerizaiton of alpha pinene, an inexpensive and important essential oil which is widely used in the synthesis of various fine chemicals (Huang et al., 2020). Hence, it is believed that the protonated titanate nanotubes/sodium titanate nanotubes can be uesed as acid or base catalyst in different types of isomerization reactions for bio-based chemicals production.
More importantly, TNTs are regarded as an attractive support material because they exhibit large surface area, high surface hydroxyl density, high ion-exchange capacity and the good stability. Recently, the catalytic performance of Au-Pd nanoparticles prepared by colloidal synthesis and immobilised on titanate nanotubes in the selective oxidation of glucose to gluconic and glucaric acids has been studied by Khawaji et al. under relatively mild conditions (Khawaji et al., 2019). They found that Au-rich catalysts favored deep oxidation to glucaric acid while Pd-rich catalysts displayed the formation of gluconic acid. It is suggested that the bimetallic composition of Au and Pd on TNTs could be tuned to enhance the production of either gluconic acid or glucaric acid.
Selective Oxidation of Benzyl Alcohol to Benzaldehyde
The selective oxidation is potentially key reaction in the biomass conversion and value-added chemicals production (Nasrollahzadeh et al., 2020). The selective oxidation of benzyl alcohol, a typical biomass derivative, to corresponding carbonyl compounds has been received much attention. A highly active Au-Pd on titanate nanotubes (Au-Pd/Ti-NT) catalyst has been produced by using colloidal synthesis and immobilisation on sodium-free Ti-nanotubes (Khawaji and Chadwick, 2017). The catalyst has markedly superior catalytic activity (turn over frequency>19 ,000 h−1) for the selective oxidation of benzyl alcohol compared with similar catalysts reported in the literature such as Au-Pd catalysts supported on Ti-NTs prepared by adsorption as well as conventional Au-Pd/TiO2 prepared by impregnation. The authors claimed that the superior catalytic activity of the catalyst is attributed to the high metal dispersion on the external surfaces of titanate nanotubes, the narrow particle size distribution, and the high degree of Au-Pd mixed alloying. Moreover, the effect of the catalyst preparation method on the selective oxidation catalytic activity of Au-Pd supported on titanate nanotubes (Au-Pd/Ti-NT) was further investigated (Khawaji and Chadwick, 2019). The most active Au-Pd/Ti-NT catalyst for the selective oxidation of benzyl alcohol is shown to be that prepared using colloidal synthesis and immobilization with PVA as a stabilizer, which has markedly superior catalytic activity compared to catalysts prepared by deposition-precipitation, adsorption, and dry impregnation methods. Therefore, it is very importance to select a synthesis method to obtain optimal catalytic performance. Besides, the morphology and physiochemical properties of the support were also found to play a crucial role for catalytic oxidation activity, selectivity, and stability (Khawaji and Chadwick, 2018; Khawaji and Chadwick, 2020). Furthermore, exploring the utilization of TNTs-based material as a photocatalyst for selective oxidation of benzyl alcohol will be desirable under ambient conditions (Yang et al., 2016).
Production of Other Bio-Based Chemicals
Hydrogenation reactions are considered as valuable and key technologies in biomass conversion processes (Li et al., 2019). Titanate nanotubes supported Pd was applied to hydrogenation of 4-carboxy-benzaldehyde, displaying a better catalytic performance than the commercial Pd/C catalyst (Liu et al., 2018). Meanwhile, Torres’ group reported the selective hydrogenation of nitrobenzenes over gold nanoparticles supported on titania nanotubes in liquid phase at room temperature (Torres et al., 2018). It was found that the selectivity towards p-substituted anilines reached 90% for all substrates in their study. Recently, other nobel metals, such Pt and Pd, confined on titanate nanotubes also performed well for the hydrogenation of nitroarenes and other substituted-nitroarenes (Shanmugaraj et al., 2022). These works indicated that the TNTs has the hollow tubular structure, the abundant –OH groups and strong metal–support interaction which renders them excellent supports for preparing TNT-supported catalysts for hydrogenation of various compounds to high-value chemicals.
Transforming of CO2 conversion into hydrocarbons recently has received significant attention (Díaz de León et al., 2019). A ternary hybrid catalyst, poly (ethyleneimine)-tethered Ir complex catalyst immobilized in titanate nanotubes were applied to hydrogenation of CO2 to formic acid under the relatively mild conditions (Kuwahara et al., 2017). Kuwahara et al. stated that the ability of TNTs to efficiently capture CO2 and to stabilize PEI, where Na+-type TNTs with higher basic property provides more productive effect, which are responsible for the high catalytic performances. Recently, the catalytic activity of rhodium supported on titanate nanotubes was evaluated by in situ infrared study in the synthesis of formic acid via CO2 hydrogenation (Ruiz-García et al., 2019). For this catalyst a turn over frequency (TOF) of 7.2 × 10−2 h−1 was obtained at 90°C and atmospheric pressure. Furthermore, the authors provided the evidence of active surface species bonded to support sites and to rhodium sites via in-situ studies. Besides, photocataytic CO2 conversion to hydrocarbon fuel using TiO2 based material is another important strategy (Razzaq and In, 2019).
Conclusion
In summary, titanate nanotubes-based heterogeneous catalyst prepared via hydrothermal method have been critically outlined and discussed in this mini-review as a promising catalyst/support for bio-based chemicals production. Titanate nanotubes can be modified by a variety of metal or non-metal dopants or be functionalized by organic surface modification to increase the acid and/or base properties of titanate nanotubes, thereby enhancing the catalytic activity and selectivity. Their very interesting properties make them promising catalysts for use in various reactions, such as acetalization/condensation, hydroxyalkylation/alkylation, transesterification, esterification, alcoholysis and isomerization for production of biomass derived chemicals. The described titanate nanotubes-based heterogeneous catalyst with different catalytic properties can be utilized as low-cost, efficient, sustainable and versatile materials. Enlightened by the hollow tubular structure, confinement effect and strong metal–support interaction, much works on the rational design of multifunctional catalyst for selective oxidation and hydrogenation reaction are ongoing. Although these catalysts have some advantages such as simple separation, and recycling, it would be highly desirable to keep the catalysts intrinsic characteristics that will enhance the catalytic stability of titanate nanotubes-based material under extreme environment. Therefore, except for traditional catalyst characterization, a combination of in situ catalytic studies and theoretical calculations and simulations are also helpful to provide valuable information toward the structure-activity relationships of titanate nanotubes-based catalysts. This review is also expected to act as a key reference to researchers for developing advanced titanate nanotubes-based catalysts in large scale applications for bio-based chemicals production with resulting in significant developments.
Author Contributions
SZ: Conceptualization, writing-original draft preparation, project administration. LW: Investigation. JB: Conceptualization. XL: Project administration, supervision. MeL: Conceptualization. MiL: Conceptualization. KH: Conceptualization.
Funding
This work was supported by the Scientific Research Fund of Hunan Provincial Education Department (Grant Nos. 19A035, 20B364) and Science and Technology Planning Project of Hunan Province (2018TP1017).
Conflict of Interest
The authors declare that the research was conducted in the absence of any commercial or financial relationships that could be construed as a potential conflict of interest.
Publisher’s Note
All claims expressed in this article are solely those of the authors and do not necessarily represent those of their affiliated organizations, or those of the publisher, the editors and the reviewers. Any product that may be evaluated in this article, or claim that may be made by its manufacturer, is not guaranteed or endorsed by the publisher.
References
Arifin, K., Yunus, R. M., Minggu, L. J., and Kassim, M. B. (2021). Improvement of TiO2 Nanotubes for Photoelectrochemical Water Splitting: Review. Int. J. Hydrogen Energy 46, 4998–5024. doi:10.1016/j.ijhydene.2020.11.063
Ashokkumar, V., Venkatkarthick, R., Jayashree, S., Chuetor, S., Dharmaraj, S., Kumar, G., et al. (2022). Recent Advances in Lignocellulosic Biomass for Biofuels and Value-Added Bioproducts - a Critical Review. Bioresour. Technol. 344, 126195. doi:10.1016/j.biortech.2021.126195
Bavykin, D. V., Friedrich, J. M., and Walsh, F. C. (2006). Protonated Titanates and TiO2 Nanostructured Materials: Synthesis, Properties, and Applications. Adv. Mat. 18, 2807–2824. doi:10.1002/adma.200502696
Brockway, P. E., Owen, A., Brand-Correa, L. I., and Hardt, L. (2019). Estimation of Global Final-Stage Energy-Return-On-Investment for Fossil Fuels with Comparison to Renewable Energy Sources. Nat. Energy 4, 612–621. doi:10.1038/s41560-019-0425-z
Camposeco, R., Castillo, S., Mejia-Centeno, I., Navarrete, J., and Rodriguez-Gonzalez, V. (2016). Behavior of Lewis and Brönsted Surface Acidity Featured by Ag, Au, Ce, La, Fe, Mn, Pd, Pt, V and W Decorated on Protonated Titanate Nanotubes. Microporous Mesoporous Mater. 236, 235–243. doi:10.1016/j.micromeso.2016.08.033
Coelho, D. C., Oliveira, A. C., Filho, J. M., Oliveira, A. C., Lucredio, A. F., Assaf, E. M., et al. (2016). Effect of the Active Metal on the Catalytic Activity of the Titanate Nanotubes for Dry Reforming of Methane. Chem. Eng. J. 290, 438–453. doi:10.1016/j.cej.2016.01.051
de Carvalho, D. C., Oliveira, A. C., Ferreira, O. P., Filho, J. M., Tehuacanero-Cuapa, S., and Oliveira, A. C. (2017). Titanate Nanotubes as Acid Catalysts for Acetalization of Glycerol with Acetone: Influence of the Synthesis Time and the Role of Structure on the Catalytic Performance. Chem. Eng. J. 313, 1454–1467. doi:10.1016/j.cej.2016.11.047
Díaz de León, J. N., Rodríguez, J. R., Rojas, J., Esqueda-Barrón, Y., Cardenas, L., Ramesh Kumar, C., et al. (2019). New Insight on the Formation of Sodium Titanates 1D Nanostructures and its Application on CO2 Hydrogenation. Front. Chem. 7, 750. doi:10.3389/fchem.2019.00750
El-Kady, K., Raslan, M., and Zaki, A. H. (2021). Effect of Different TiO2 Morphologies on the Activity of Immobilized Lipase for Biodiesel Production. ACS Omega 6, 35484–35493. doi:10.1021/acsomega.1c04942
Gielen, D., Boshell, F., Saygin, D., Bazilian, M. D., Wagner, N., and Gorini, R. (2019). The Role of Renewable Energy in the Global Energy Transformation. Energy Strategy Rev. 24, 38–50. doi:10.1016/j.esr.2019.01.006
Gomes, I. S., de Carvalho, D. C., Oliveira, A. C., Rodríguez-Castellón, E., Tehuacanero-Cuapa, S., Freire, P. T. C., et al. (2018). On the Reasons for Deactivation of Titanate Nanotubes with Metals Catalysts in the Acetalization of Glycerol with Acetone. Chem. Eng. J. 334, 1927–1942. doi:10.1016/j.cej.2017.11.112
Gong, D., Grimes, C. A., Varghese, O. K., Hu, W., Singh, R. S., Chen, Z., et al. (2001). Titanium Oxide Nanotube Arrays Prepared by Anodic Oxidation. J. Mat. Res. 16, 3331–3334. doi:10.1557/jmr.2001.0457
He, J., Qiang, Q., Liu, S., Song, K., Zhou, X., Guo, J., et al. (2021). Upgrading of Biomass-Derived Furanic Compounds into High-Quality Fuels Involving Aldol Condensation Strategy. Fuel 306, 121765. doi:10.1016/j.fuel.2021.121765
Hernández-Hipólito, P., García-Castillejos, M., Martínez-Klimova, E., Juárez-Flores, N., Gómez-Cortés, A., and Klimova, T. E. (2014). Biodiesel Production with Nanotubular Sodium Titanate as a Catalyst. Catal. Today 220-222, 4–11. doi:10.1016/j.cattod.2013.09.003
Hernández-Hipólito, P., Juárez-Flores, N., Martínez-Klimova, E., Gómez-Cortés, A., Bokhimi, X., Escobar-Alarcón, L., et al. (2015). Novel Heterogeneous Basic Catalysts for Biodiesel Production: Sodium Titanate Nanotubes Doped with Potassium. Catal. Today 250, 187–196. doi:10.1016/j.cattod.2014.03.025
Huang, G., Zhou, S., Liu, J., Su, S., and Yin, D. (2020). Highly-selective Solvent-free Catalytic Isomerization of α-pinene to Camphene over Reusable Titanate Nanotubes. RSC Adv. 10, 10606–10611. doi:10.1039/d0ra01093f
Ji, H., Ni, J., Zhao, D., and Liu, W. (2022). Application of Titanate Nanotubes for Photocatalytic Decontamination in Water: Challenges and Prospects. ACS Est. Eng. doi:10.1021/acsestengg.1c00451
Kasuga, T., Hiramatsu, M., Hoson, A., Sekino, T., and Niihara, K. (1998). Formation of Titanium Oxide Nanotube. Langmuir 14, 3160–3163. doi:10.1021/la9713816
Kasuga, T., Hiramatsu, M., Hoson, A., Sekino, T., and Niihara, K. (1999). Titania Nanotubes Prepared by Chemical Processing. Adv. Mat. 11, 1307–1311. doi:10.1002/(sici)1521-4095(199910)11:15<1307::aid-adma1307>3.0.co;2-h
Khawaji, M., and Chadwick, D. (2017). Au-Pd Bimetallic Nanoparticles Immobilised on Titanate Nanotubes: A Highly Active Catalyst for Selective Oxidation. ChemCatChem 9, 4353–4363. doi:10.1002/cctc.201700851
Khawaji, M., and Chadwick, D. (2018). Au–Pd NPs Immobilised on Nanostructured Ceria and Titania: Impact of Support Morphology on the Catalytic Activity for Selective Oxidation. Catal. Sci. Technol. 8, 2529–2539. doi:10.1039/c7cy02329d
Khawaji, M., and Chadwick, D. (2019). Selective Catalytic Oxidation over Au-Pd/titanate Nanotubes and the Influence of the Catalyst Preparation Method on the Activity. Catal. Today 334, 122–130. doi:10.1016/j.cattod.2018.11.080
Khawaji, M., and Chadwick, D. (2020). Selective Oxidation Using Au-Pd Catalysts: Role of the Support in the Stabilization of Colloidal Au-Pd NPs. Catal. Today 348, 203–211. doi:10.1016/j.cattod.2019.08.036
Khawaji, M., Zhang, Y., Loh, M., Graça, I., Ware, E., and Chadwick, D. (2019). Composition Dependent Selectivity of Bimetallic Au-Pd NPs Immobilised on Titanate Nanotubes in Catalytic Oxidation of Glucose. Appl. Catal. B Environ. 256, 117799. doi:10.1016/j.apcatb.2019.117799
Kitano, M., Nakajima, K., Kondo, J. N., Hayashi, S., and Hara, M. (2010). Protonated Titanate Nanotubes as Solid Acid Catalyst. J. Am. Chem. Soc. 132, 6622–6623. doi:10.1021/ja100435w
Kitano, M., Wada, E., Nakajima, K., Hayashi, S., Miyazaki, S., Kobayashi, H., et al. (2013). Protonated Titanate Nanotubes with Lewis and Brønsted Acidity: Relationship between Nanotube Structure and Catalytic Activity. Chem. Mat. 25, 385–393. doi:10.1021/cm303324b
Kumar, S., Nepak, D., Kansal, S. K., and Elumalai, S. (2018). Expeditious Isomerization of Glucose to Fructose in Aqueous Media over Sodium Titanate Nanotubes. RSC Adv. 8, 30106–30114. doi:10.1039/c8ra04353a
Kuwahara, Y., Fujie, Y., and Yamashita, H. (2017). Poly(ethyleneimine)-tethered Ir Complex Catalyst Immobilized in Titanate Nanotubes for Hydrogenation of CO2 to Formic Acid. ChemCatChem 9, 1906–1914. doi:10.1002/cctc.201700508
Li, H., Huang, Y., Liu, J., and Duan, H. (2021). Hydrothermally Synthesized Titanate Nanomaterials for the Removal of Heavy Metals and Radionuclides from Water: A Review. Chemosphere 282, 131046. doi:10.1016/j.chemosphere.2021.131046
Li, H., Li, Y., Fang, Z., and Smith, R. L. (2019). Efficient Catalytic Transfer Hydrogenation of Biomass-Based Furfural to Furfuryl Alcohol with Recycable Hf-Phenylphosphonate Nanohybrids. Catal. Today 319, 84–92. doi:10.1016/j.cattod.2018.04.056
Li, H., Zhao, W., and Fang, Z. (2017). Hydrophobic Pd Nanocatalysts for One-Pot and High-Yield Production of Liquid Furanic Biofuels at Low Temperatures. Appl. Catal. B Environ. 215, 18–27. doi:10.1016/j.apcatb.2017.05.039
Li, S., Li, N., Li, G., Li, L., Wang, A., Cong, Y., et al. (2015). Protonated Titanate Nanotubes as a Highly Active Catalyst for the Synthesis of Renewable Diesel and Jet Fuel Range Alkanes. Appl. Catal. B Environ. 170-171, 124–134. doi:10.1016/j.apcatb.2015.01.022
Liu, J., Du, W., Li, Z., and Yang, A. (2018). Preparation of TiO2 Nanotube Supported Pd for the Hydrogenation of 4-Carboxy-Benzaldehyde. Catal. Lett. 148, 2472–2479. doi:10.1007/s10562-018-2469-2
Machorro López, J. J., Lázaro, A. L., Rodriguez‐Valadez, F. J., and Espejel‐Ayala, F. (2021). Synthesis of Sodium Titanate Catalysts Using a Factorial Design for Biodiesel Production. Environ. Prog. Sustain. Energy 40, e13475. doi:10.1002/ep.13475
Madurai Ramakrishnan, V., Muthukumarasamy, N., Balraju, P., Pitchaiya, S., Velauthapillai, D., and Pugazhendhi, A. (2020). Transformation of TiO2 Nanoparticles to Nanotubes by Simple Solvothermal Route and its Performance as Dye-Sensitized Solar Cell (DSSC) Photoanode. Int. J. Hydrogen Energy 45, 15441–15452. doi:10.1016/j.ijhydene.2020.04.021
Mao, F.-F., Zhao, W., Tao, D.-J., and Liu, X. (2020). Highly Efficient Conversion of Renewable Levulinic Acid to N-Butyl Levulinate Catalyzed by Sulfonated Magnetic Titanium Dioxide Nanotubes. Catal. Lett. 150, 2709–2715. doi:10.1007/s10562-020-03177-0
Martínez-Klimov, M. E., Ramírez-Vidal, P., Roquero Tejeda, P., and Klimova, T. E. (2020). Synergy between Sodium Carbonate and Sodium Titanate Nanotubes in the Transesterification of Soybean Oil with Methanol. Catal. Today 353, 119–125. doi:10.1016/j.cattod.2019.08.027
Martínez-Klimova, E., Hernández-Hipólito, P., and Klimova, T. E. (2016). Biodiesel Production with Nanotubular Sodium Titanate Doped with Potassium as a Catalyst. MRS Adv. 1, 415–420. doi:10.1557/adv.2015.52
Muniyappan, S., Solaiyammal, T., Sudhakar, K., Karthigeyan, A., and Murugakoothan, P. (2017). Conventional Hydrothermal Synthesis of Titanate Nanotubes: Systematic Discussions on Structural, Optical, Thermal and Morphological Properties. Mod. Electron. Mater. 3, 174–178. doi:10.1016/j.moem.2017.10.002
Nady, D., Zaki, A. H., Raslan, M., and Hozayen, W. (2020). Enhancement of Microbial Lipase Activity via Immobilization over Sodium Titanate Nanotubes for Fatty Acid Methyl Esters Production. Int. J. Biol. Macromol. 146, 1169–1179. doi:10.1016/j.ijbiomac.2019.09.240
Nasrollahzadeh, M., Shafiei, N., Nezafat, Z., Soheili Bidgoli, N. S., and Soleimani, F. (2020). Recent Progresses in the Application of Cellulose, Starch, Alginate, Gum, Pectin, Chitin and Chitosan Based (Nano)catalysts in Sustainable and Selective Oxidation Reactions: A Review. Carbohydr. Polym. 241, 116353. doi:10.1016/j.carbpol.2020.116353
Okolie, J. A., Mukherjee, A., Nanda, S., Dalai, A. K., and Kozinski, J. A. (2021). Next‐generation Biofuels and Platform Biochemicals from Lignocellulosic Biomass. Int. J. Energy Res. 45, 14145–14169. doi:10.1002/er.6697
Ou, H., and Lo, S. (2007). Review of Titania Nanotubes Synthesized via the Hydrothermal Treatment: Fabrication, Modification, and Application. Sep. Purif. Technol. 58, 179–191. doi:10.1016/j.seppur.2007.07.017
Razzaq, A., and In, S.-I. (2019). TiO2 Based Nanostructures for Photocatalytic CO2 Conversion to Valuable Chemicals. Micromachines 10, 326. doi:10.3390/mi10050326
Reddy, B. R. P., Reddy, P. V. G., and Reddy, B. N. (2015). Efficient Solvent Free Synthesis of Tertiary α-aminophosphonates Using H2Ti3O7nanotubes as a Reusable Solid-Acid Catalyst. New J. Chem. 39, 9605–9610. doi:10.1039/c5nj01914a
Rempel, A. A., Valeeva, A. A., Vokhmintsev, A. S., and Weinstein, I. A. (2021). Titanium Dioxide Nanotubes: Synthesis, Structure, Properties and Applications. Russ. Chem. Rev. 90, 1397–1414. doi:10.1070/rcr4991
Ruiz‐García, J. R., Fierro‐Gonzalez, J. C., Handy, B. E., Hinojosa‐Reyes, L., De Haro Del Río, D. A., Lucio‐Ortiz, C. J., et al. (2019). An In Situ Infrared Study of CO2 Hydrogenation to Formic Acid by Using Rhodium Supported on Titanate Nanotubes as Catalysts. ChemistrySelect 4, 4206–4216. doi:10.1002/slct.201900361
Schutyser, W., Renders, T., Van den Bosch, S., Koelewijn, S.-F., Beckham, G. T., and Sels, B. F. (2018). Chemicals from Lignin: An Interplay of Lignocellulose Fractionation, Depolymerisation, and Upgrading. Chem. Soc. Rev. 47, 852–908. doi:10.1039/c7cs00566k
Shanmugaraj, K., Bustamante, T. M., de León, J. N. D., Aepuru, R., Mangalaraja, R. V., Torres, C. C., et al. (2022). Noble Metal Nanoparticles Supported on Titanate Nanotubes as Catalysts for Selective Hydrogenation of Nitroarenes. Catal. Today 392-393, 93–104. doi:10.1016/j.cattod.2021.09.003
Sluban, M., Cojocaru, B., Parvulescu, V. I., Iskra, J., Cerc Korošec, R., and Umek, P. (2017). Protonated Titanate Nanotubes as Solid Acid Catalyst for Aldol Condensation. J. Catal. 346, 161–169. doi:10.1016/j.jcat.2016.12.015
Souza, A. P. S., Almeida, A. F. L., Freire, F. N. A., Nunes, V. F., and de Lima, F. M. Titanates Nanotubes and Nanoribbons Applied in Dye-Sensitized Solar Cells, R. F. d. Nascimento, V. d. O. S. Neto, P. B. A. Fechine, and P. d. T. C. Freire, in Nanomaterials and Nanotechnology. Materials Horizons: From Nature to Nanomaterials, Springer Singapore, Singapore, 2021, pp. 339–373.doi:10.1007/978-981-33-6056-3_11
Stančin, H., Mikulčić, H., Wang, X., and Duić, N. (2020). A Review on Alternative Fuels in Future Energy System. Renew. Sustain. Energy Rev. 128, 109927. doi:10.1016/j.rser.2020.109927
Talebian-Kiakalaieh, A., Amin, N. A. S., Najaafi, N., and Tarighi, S. (2018). A Review on the Catalytic Acetalization of Bio-Renewable Glycerol to Fuel Additives. Front. Chem. 6, 573. doi:10.3389/fchem.2018.00573
Timothy, A. A., Han, F., Li, G., Xu, J., Wang, A., Cong, Y., et al. (2020). Synthesis of Jet Fuel Range High-Density Dicycloalkanes with Methyl Benzaldehyde and Acetone. Sustain. Energy Fuels 4, 5560–5567. doi:10.1039/d0se01110j
Torres, C. C., Jiménez, V. A., Campos, C. H., Alderete, J. B., Dinamarca, R., Bustamente, T. M., et al. (2018). Gold Catalysts Supported on TiO 2 -nanotubes for the Selective Hydrogenation of P -substituted Nitrobenzenes. Mol. Catal. 447, 21–27. doi:10.1016/j.mcat.2017.12.039
Wada, E., Kitano, M., Nakajima, K., and Hara, M. (2013). Effect of Preparation Conditions on the Structural and Acid Catalytic Properties of Protonated Titanate Nanotubes. J. Mat. Chem. A 1, 12768–12774. doi:10.1039/c3ta13015k
Wada, E., Kitano, M., Yamamoto, K., Nakajima, K., Hayashi, S., and Hara, M. (2016). Synthesis of Niobium-Doped Titanate Nanotubes as Solid Acid Catalysts. Catal. Sci. Technol. 6, 4832–4839. doi:10.1039/c6cy00044d
Xu, C., He, J., Zhang, W., Cui, H., Zhu, J., and Hu, L. (2020). Effect of Microstructures on the Acidity and Catalytic Performance for H 2 Ti 3 O 7 Nanomaterials. ChemistrySelect 5, 10081–10089. doi:10.1002/slct.202002739
Yang, J., Shen, X., Wei, J., Zhang, L., Zhao, D., and Yao, B. (2016). Selective Oxidation of Alcohols on Hydrogen Titanate Nanotubes under Visible Light Irradiation: Relationship between Nanostructure and Catalytic Activity. Catal. Sci. Technol. 6, 7604–7614. doi:10.1039/c6cy01345g
Yao, Y., Zuo, M., Shao, P., Huang, X., Li, J., Duan, Y., et al. (2020). Oxidative Desulfurization of 4,6-dimethyldibenzothiophene over Short Titanate Nanotubes: A Non-classical Shape Selective Catalysis. J. Porous Mat. 27, 331–338. doi:10.1007/s10934-019-00809-8
Zaki, A. H., Naeim, A. A., and El-Dek, S. I. (2019). Sodium Titanate Nanotubes for Efficient Transesterification of Oils into Biodiesel. Environ. Sci. Pollut. Res. 26, 36388–36400. doi:10.1007/s11356-019-06602-z
Zhang, H., Li, H., Hu, Y., Venkateswara Rao, K. T., Xu, C., and Yang, S. (2019). Advances in Production of Bio-Based Ester Fuels with Heterogeneous Bifunctional Catalysts. Renew. Sustain. Energy Rev. 114, 109296. doi:10.1016/j.rser.2019.109296
Zhang, M., Bando, Y., and Wada, K. (2001). Sol-gel Template Preparation of TiO2 Nanotubes and Nanorods. J. Mat. Sci. Lett. 20, 167–170. doi:10.1023/a:1006739713220
Zhou, S., Lei, M., Bai, J., Liu, X., Wu, L., Long, M., et al. (2022a). Titania Nanotubes-Bonded Sulfamic Acid as an Efficient Heterogeneous Catalyst for the Synthesis of N-Butyl Levulinate. Front. Chem. 10, 894965. doi:10.3389/fchem.2022.894965
Zhou, S., Jiang, D., Liu, X., Chen, Y., and Yin, D. (2018). Titanate Nanotubes-Bonded Organosulfonic Acid as Solid Acid Catalyst for Synthesis of Butyl Levulinate. RSC Adv. 8, 3657–3662. doi:10.1039/c7ra12994g
Zhou, S., Lai, J., Liu, X., Huang, G., You, G., Xu, Q., et al. (2022b). Selective Conversion of Biomass-Derived Furfuryl Alcohol into N-Butyl Levulinate over Sulfonic Acid Functionalized TiO2 Nanotubes. Green Energy & Environ. 7, 257–265. doi:10.1016/j.gee.2020.09.009
Zhou, S., Liu, X., Lai, J., Zheng, M., Liu, W., Xu, Q., et al. (2019). Covalently Linked Organo-Sulfonic Acid Modified Titanate Nanotube Hybrid Nanostructures for the Catalytic Esterification of Levulinic Acid with N-Butyl Alcohol. Chem. Eng. J. 361, 571–577. doi:10.1016/j.cej.2018.12.111
Keywords: titanate nanotubes, bio-based chemicals, catalytic reaction, supports, solid acid (base) catalyst
Citation: Zhou S, Wu L, Bai J, Liu X, Lei M, Long M and Huang K (2022) Titanate Nanotubes-Based Heterogeneous Catalyst for Efficient Production of Biomass Derived Chemicals. Front. Chem. 10:939289. doi: 10.3389/fchem.2022.939289
Received: 09 May 2022; Accepted: 23 May 2022;
Published: 06 June 2022.
Edited by:
Hu Li, Guizhou University, ChinaCopyright © 2022 Zhou, Wu, Bai, Liu, Lei, Long and Huang. This is an open-access article distributed under the terms of the Creative Commons Attribution License (CC BY). The use, distribution or reproduction in other forums is permitted, provided the original author(s) and the copyright owner(s) are credited and that the original publication in this journal is cited, in accordance with accepted academic practice. No use, distribution or reproduction is permitted which does not comply with these terms.
*Correspondence: Shuolin Zhou, c2x6aG91MTk4OUAxNjMuY29t; Xianxiang Liu, bHh4QGh1bm51LmVkdS5jbg==