Visible-Light-Promoted Transition-Metal-Free Construction of 3-Perfluoroalkylated Thioflavones
- 1Collaborative Innovation Centre of Henan Province for Green Manufacturing of Fine Chemicals, Key Laboratory of Green Chemical Media and Reactions, Ministry of Education, Henan Engineering Research Centre of Chiral Hydroxyl Pharmaceutical, Henan Engineering Laboratory of Chemical Pharmaceutical and Biomedical Materials, School of Chemistry and Chemical Engineering, Henan Normal University, Xinxiang, China
- 2Green Catalysis Center, College of Chemistry, Zhengzhou University, Zhengzhou, China
A visible-light-promoted transition-metal-free perfluoroalkylation/cyclization reaction was developed with 9-mesityl-10-methylacridinium perchlorate (Acr+-Mes·ClO4−) as the photocatalyst, by which various perfluoroalkyl-substituted heterocycles including thioflavones, oxindoles, and quinoline-2,4(1H,3H)-diones were prepared at room temperature. Moreover, the potential of this sustainable method is demonstrated by the excellent in vitro anti-lymphoma and cervical carcinoma activity of the novel 3-perfluoroalkylated thioflavone 3m.
Introduction
Thioflavone is a privileged scaffold that is ubiquitous in natural products, bioactive molecules, and functional materials (Dong et al., 2018). The derivatives of thioflavones have been found to exhibit intriguing biological activities, such as anticancer, (Wang et al., 1996), and anti-malarial (Razdan et al., 1978). Consequently, the construction of thioflavones with various substituents has attracted considerable attention (Kumar and Bodas, 2001; Pan et al., 2018; Sangeetha and Sekar, 2019; Yang et al., 2020; Zheng et al., 2020; Wang W. et al., 2021; Lee Jae, 2021). The perfluoroalkyl group, especially trifluoromethyl group, is one of the most prominent substituents in medicinal chemistry, which is essential for more than 70 approved drugs (Schiesser et al., 2020). It may be attributed to the fact that perfluoroalkyl group can remarkablely improve the pharmacokinetics properties, lipophilicity and target inhibitory of the parent compounds (Müller et al., 2007; Tang et al., 2015; Tang et al., 2017a; Tang et al., 2017b; Wang et al., 2019; Ma et al., 2021a; Chen et al., 2022). In this context, developing the method to access perfluoroalkyl containing thioflavone is of great significance. However, there are currently no available reports to deliver this fragment.
Recently, methylthiolated alkynone is used as a unique starting material to access thioflavones containing diverse substitutes by the radical-initiated cyclization (Zhou et al., 2006; Alcaide et al., 2017). For instance, Song and coworkers developed a highly efficient approach to synthesizing phosphoryl-, acyl-, and sulfenyl-containing thioflavones from methylthiolated alkynones (Xu et al., 2019). Huang’s group realized the reaction of AgSCF3 with methylthiolated alkynones for the synthesis of 3-trifluoromethylthiolated thioflavones with (NH4)2S2O8 as the oxidant at 80°C (Wang L. et al., 2021). Du and Zhao et al reported the synthesis of selenyl/sulfenyl thioflavones with phenyliodine(III) bis(trifluoroacetate) (PIFA) as an oxidant (Ai et al., 2020). Recently, Ye and Wu’s group realized an Ir-photocatalyzed radical relay reaction of methylthiolated alkynones and potassium metabisulfite in the presence of sodium methylsulfinate (Liu et al., 2022). With the radical-initiated cyclization of methylthiolated alkynone, our group has developed some protocols to access the phosphorylated, (Liu et al., 2020), acylated, (Zhu et al., 2021), sulfonylated, (Feng et al., 2020; Jiang et al., 2020), and thiocyanated thioflavones (Zeng et al., 2021). Nevertheless, the synthesis of thioflavones bearing perfluoroalkyl-substituents is rarely reported. Considering the critical roles of F-containing groups in the development of bioactive molecules, we herein disclosed that perfluoroalkyl-containing thioflavones could be accessed through photocatalytic cascade perfluoroalkylation/cyclization reactions in the presence of sodium perfluoroalkanesulfinates (RfSO2Na) as the perfluoroalkyl source (Rf = CF3, C2F5, C4F9, C6F13, C8F17) and 9-mesityl-10-methylacridinium perchlorate (Acr+-Mes·ClO4−) as a transition-metal-free photocatalyst (Scheme 1). More importantly, the synthesized compounds exhibited excellent in vitro antitumor properties, which indicated that the unique protocol could be used to deliver novel antitumor hit compounds.
Results and Discussion
We chose methylthiolated alkynone (1a) and CF3SO2Na (2a) as model substrates to investigate the perfluoroalkylation/cyclization reaction in CH3CN under 18 W blue LEDs irradiation at 35°C. Fortunately, when Acr+-Mes·ClO4− was used as the photocatalyst and HCl as the acid additive, 1a and 2a could be converted into the corresponding trifluoromethylated thioflavone 3a in 37% yield (Table 1, entry 1). Evaluation of the different photocatalysts showed that Acr+-Mes·ClO4− was the best photocatalyst for this process (Table 1, entries 2-4). Different acidic additives were screened to further improve the efficiency of this transformation (Table 1, entries 5-8). The experimental results indicated that trifluoroacetic acid (TFA) showed the highest reactivity, affording 3a in 44% yield (Table 1, entry 7). To further improve the yield, a range of solvents, including DCM, DCE, CHCl3, 1,4-dioxane, DMF, DMSO, EtOH, H2O, and acetonitrile aqueous were evaluated (Table 1, entries 9-20). The mixed solvent CH3CN/H2O (v/v = 10:1) was found to be the optimal solvent system, and the desired product 3a could be obtained in 56% yield (Table 1, entry 19). Furthermore, increasing the amount of 2a to 3 equiv afforded the highest yield (Table 1, entry 21). The control experiments confirmed the photochemical nature of this transformation, as no product was observed in the absence of photocatalyst or visible light (Table 1, entries 22-23). The reaction efficiency was decreased to 35% in the absence of TFA, which indicated that the acid plays an important role in promoting the transformation (Table 1, entry 24). Taken together, the optimal reaction conditions were established as follows: 1a (0.2 mmol), 2a (3 equiv), Acr+-Mes·ClO4− (5 mol%) as catalyst, TFA (1 equiv) as additive, CH3CN/H2O (v/v = 10:1) as solvent, at 35°C under the irradiation of blue LEDs (λmax = 460 nm) for 5 h.
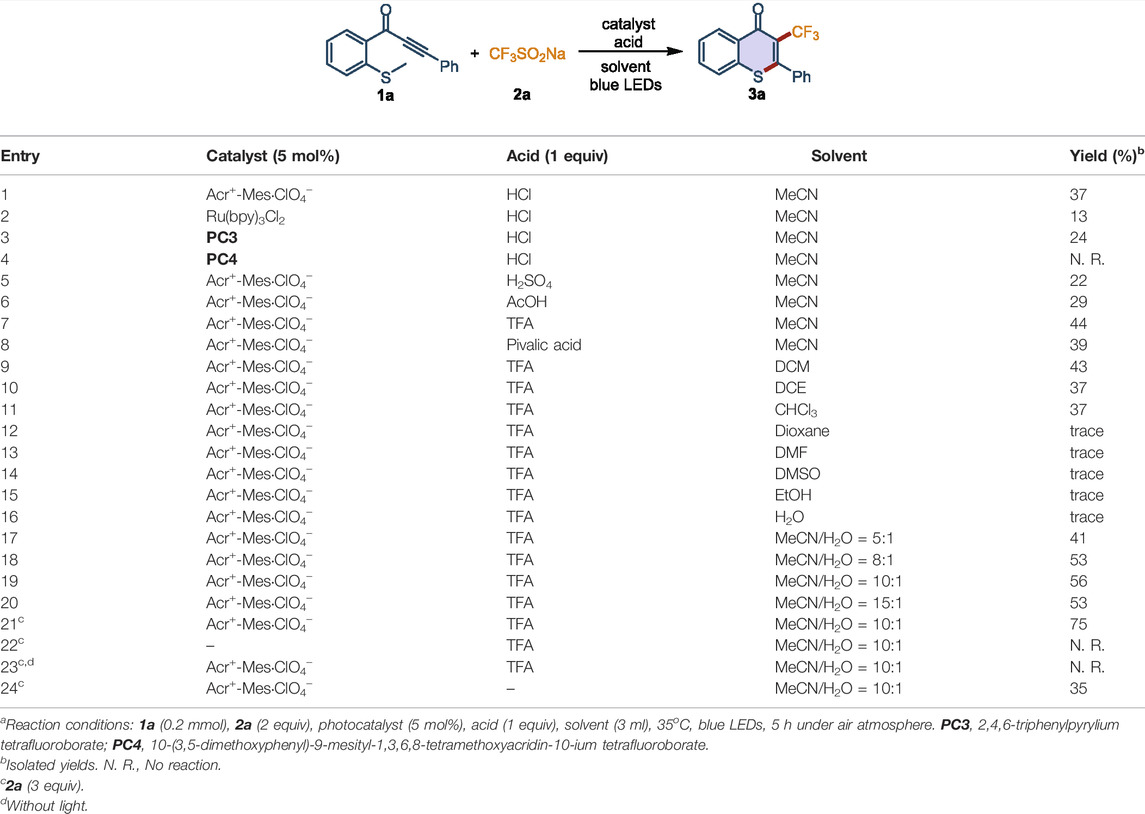
TABLE 1. Optimization of reaction conditionsa.
With the optimal conditions in hand, we further explored the scope and generality of this cascade perfluoroalkylation/cyclization reaction. Firstly, the reactivities of electron-rich or electron-deficient methylthiolated alkynones were investigated. As depicted in Scheme 2, the electron-donating groups (4-Me, 4-Et, 4-tBu, 4-MeO, 3-Me) were well tolerant, giving the desired products 3b-3f in 55–78% yields. For the substrates with electron-withdrawing groups, we found that both halogen substitutions (4-F, 4-Cl, 4-Br, 3-F, 2-Br) and CF3 group were compatible with this transformation, and the corresponding products 3g-3l were obtained in moderate to good yields. Moreover, the methylthiolated alkynone containing a strong electron-withdrawing substituent (4-CN) worked well under the standard conditions (3m). The pyridine or naphthalene analogs (1n-1o) were also found to be tolerant to the optimized condition. To our delight, the substrate containing alkyl group instead of aryl ring is well tolerant, affording the desired product 3p in 63% yield. Moreover, the structure of 3a was unambiguously confirmed by X-ray crystallographic analysis.
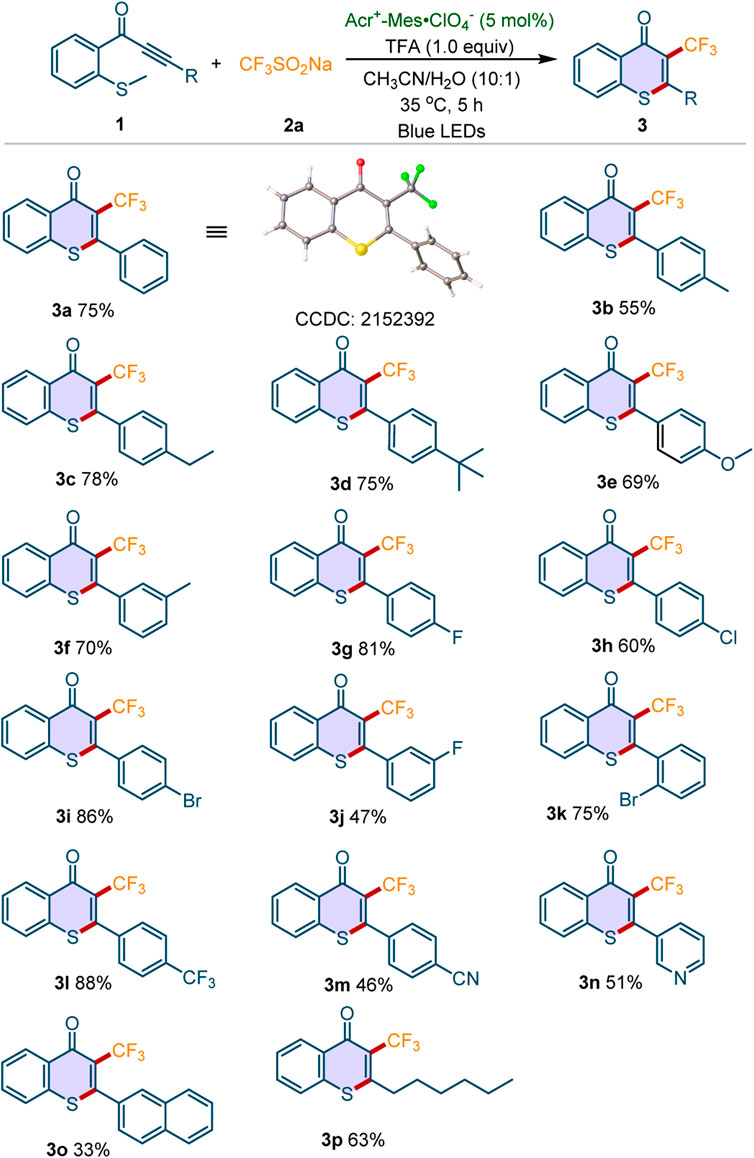
Scheme 2. Scope of methylthiolated alkynones. Reaction conditions: 1 (0.2 mmol), 2a (3 equiv), Acr+-Mes·ClO4− (5 mol%), TFA (1 equiv), CH3CN/H2O (10:1, 3 ml), 35oC, blue LEDs, 5 h under air atmosphere. Isolated yields were given.
Subsequently, the scope of sodium perfluoroalkanesulfinates 2 was examined. As shown in Scheme 3, a variety of sodium perfluoroalkanesulfinates were well tolerated in this protocol. For example, CF3CF2SO2Na, CF3(CF2)3SO2Na, CF3(CF2)5SO2Na and CF3(CF2)7SO2Na reacted well with 1a, furnishing the perfluoroalkyl-substituted thioflavones 3q-3t in moderate to good yields. Meanwhile, the sodium perfluoroalkanesulfinates reacted well with methylthiolated alkynones 1 bearing different substituents (Me, Et, OMe, F), affording the desired products 3u-3y in 41–84% yields. Notably, all the synthesized 3-perfluoroalkylated thioflavones are new compounds.
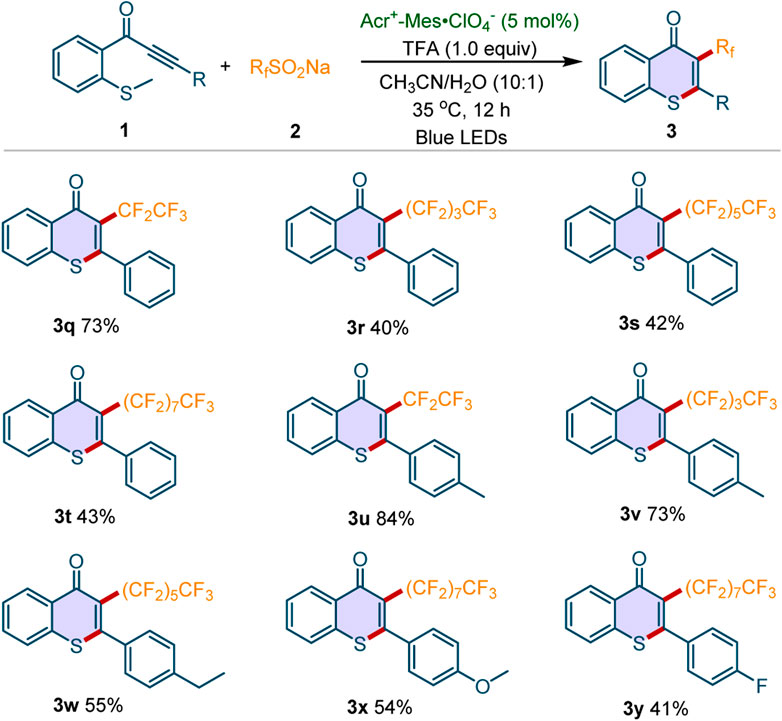
Scheme 3. Scope of sodium perfluoroalkanesulfinates. Reaction conditions: 1 (0.2 mmol), 2a (3 equiv), Acr+-Mes·ClO4− (5 mol%), TFA (1 equiv), CH3CN/H2O (v/v = 10:1, 3 mL), 35oC, blue LEDs, 12 h under air atmosphere. Isolated yields were given.
To evaluate the applicability of this perfluoroalkylation/cyclization reaction in the pharmaceutical industry, it was scaled up to 4 mmol under standard conditions. Delightfully, the desired product 3a was obtained in 64% yield (Scheme 4A), which indicates that the transformation may be used in drug development. Inspired by the successful usage of this photocatalytic system in the synthesis of perfluoroalkylated thioflavones, we then applied the sustainable system in the construction of other perfluoroalkylated heterocycles under the standard conditions (Scheme 4B). The substrates N-(4-chlorophenyl)-N-methylmethacrylamide 4 and N-(2-cyanophenyl)-N-methylmethacrylamide 6 could be converted into the corresponding trifluoromethylated oxindole 5 and trifluoromethylated quinoline-2,4(1H,3H)-dione 7 in 58 and 88% yields, respectively.
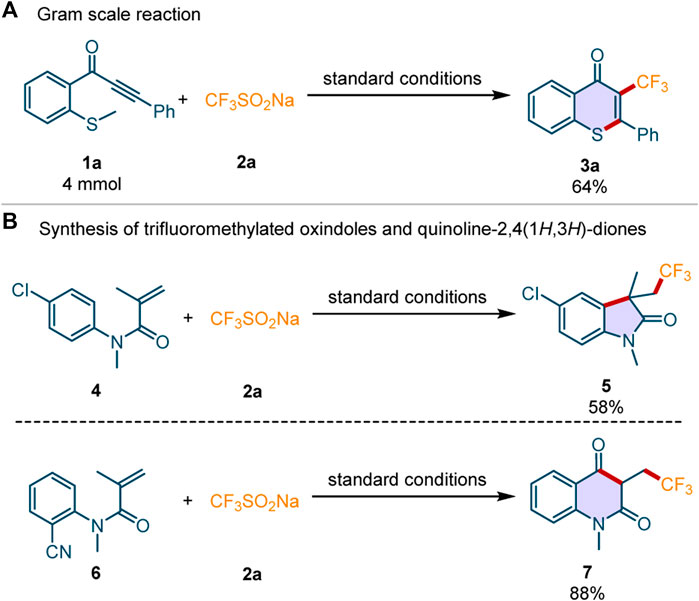
Scheme 4. The gram-scale synthesis and the application of this perfluoroalkylation/cyclization reactions.
To explore the reaction mechanism, the control experiment and the Stern–Volmer fluorescence quenching experiments were performed (Scheme 5). The addition of radical quencher, 2,2,6,6-tetramethylpiperidinyl-1-oxyl (TEMPO), to the standard conditions completely prevented the reaction (Scheme 5A). It indicated that a radical pathway may be involved in this photocatalytic transformation. We conducted the Stern–Volmer fluorescence quenching experiment by mixing the photocatalyst Acr+-Mes·ClO4− (PC) with methylthiolated alkynone 1a and CF3SO2Na 2a, respectively. The results were depicted in Scheme 5B. The luminescence effect was obviously quenched by the addition of 1a, while it is hardly changed by the addition of 2a. Moreover, a strong linear relationship was observed between I0/I and the concentration of 1a, indicating that 1a could act as an available quencher of the excited state of the photocatalyst (for details see the Supplementary Material).
Based on the above experimental results and the previous reports, (Neogi et al., 2020; Ma et al., 2021b; Yang et al., 2021; Ma et al., 2022; Shen et al., 2022; Zhu et al., 2022), we proposed a plausible reaction mechanism for this photocatalyzed perfluoroalkylation/cyclization reaction (Scheme 6). Under the visible light irradiation, Acr+-Mes was activated into the excited state Acr+-Mes*, which then oxidized the substrate 1 to afford the radical cation 1•+ and the radical anion [Acr+-Mes]•-. CF3SO2Na 2a was in situ converted into CF3SO2H in the presence of the acid TFA. Then the radical cation 1•+ reacted with CF3SO2H via a single-electron transfer (SET) process to generate the CF3 radical and regenerate the substrate 1. The desired product 3 was afforded by the addition of CF3 radical to the triple bond of 1 and a subsequent intramolecular demethylation cyclization. On the other hand, the [Acr+-Mes]•- could be oxidized by the O2 in the air to regenerate the ground state of the photocatalyst and complete the photoredox cycle.
To highlight this sustainable method in drug development, we evaluated the in vitro antitumor activity of the novel 3-perfluoroalkylated thioflavones. As shown in Scheme 7, compound 3m exhibited better antitumor activities against Ramos cell and Hela cell than that of broad-spectrum antitumor drug 5-fluorouracil (5-FU), which indicated that our compound has the potential to treat human B cell lymphoma and human cervical carcinoma. Because tumor resistance to chemotherapeutic drugs is a significant issue in the clinical treatment of carcinoma, the development of novel chemical entries, such as the 3-perfluoroalkylated thioflavones, is of great value.
Conclusion
In summary, we have developed a visible-light-induced perfluoroalkylation/cyclization of methylthiolated alkynones for the mild and rapid construction of 3-perfluoroalkylated thioflavones. It has been demonstrated that this radical involved strategy is tolerant of a variety of functional groups and could be applied to the construction of other perfluoroalkylated heterocycles, such as oxindoles and quinoline-2.4(1H,3H)-diones. Moreover, compound 3m exhibited robust antitumor activity, which provides a novel chemical entry for the clinical treatment of human lymphoma and cervical carcinoma. The structural modification of these novel compounds is currently underway in our laboratory.
Data Availability Statement
The original contributions presented in the study are included in the article/Supplementary Material, further inquiries can be directed to the corresponding authors.
Author Contributions
All authors listed have made a substantial, direct, and intellectual contribution to the work and approved it for publication.
Conflict of Interest
The authors declare that the research was conducted in the absence of any commercial or financial relationships that could be construed as a potential conflict of interest.
Publisher’s Note
All claims expressed in this article are solely those of the authors and do not necessarily represent those of their affiliated organizations, or those of the publisher, the editors and the reviewers. Any product that may be evaluated in this article, or claim that may be made by its manufacturer, is not guaranteed or endorsed by the publisher.
Acknowledgments
We would like to thank the Large Instrument Sharing System for the support of structure confirmation. We acknowledge the financial support from the National Natural Science Foundation of China (82003585, 21971224), the Postgraduate Education Reform Project of Henan Province (2019SJGLX008Y, 2019SJGLX034Y), the Postgraduate Education Reform and Quality Improvement Project of Henan Province (YJS2021AL079), and the Technical innovation Team of Henan Normal University (2022TD03).
Supplementary Material
The Supplementary Material for this article can be found online at: https://www.frontiersin.org/articles/10.3389/fchem.2022.953978/full#supplementary-material
References
Ai, Z., Xiao, J., Li, Y., Guo, B., Du, Y., and Zhao, K. (2020). Metal-free Synthesis of 3-chalcogenyl Chromones from Alkynyl Aryl Ketones and Diorganyl Diselenides/disulfides Mediated by PIFA. Org. Chem. Front. 7, 3935–3940. doi:10.1039/d0qo01175d
Alcaide, B., Almendros, P., Busto, E., Herrera, F., Lázaro-Milla, C., and Luna, A. (2017). Photopromoted Entry to Benzothiophenes, Benzoselenophenes, 3H-Indoles, Isocoumarins, Benzosultams, and (Thio)flavones by Gold-Catalyzed Arylative Heterocyclization of Alkynes. Adv. Synth. Catal. 359, 2640–2652. doi:10.1002/adsc.201700427
Chen, N., Lei, J., Wang, Z., Liu, Y., Sun, K., and Tang, S. (2022). Construction of Fluoro-Containing Heterocycles Mediated by Free Radicals. Chin. J. Org. Chem. 42, 1061–1084. doi:10.6023/cjoc202109033
Dong, J., Zhang, Q., Meng, Q., Wang, Z., Li, S., and Cui, J. (2018). The Chemistry and Biological Effects of Thioflavones. Mini Rev. Med. Chem. 18, 1714–1732. doi:10.2174/1389557518666180515145633
Feng, Z.-W., Li, J., Jiang, Y.-Q., Tian, Y., Xu, G.-Q., Shi, X., et al. (2020). Transition-metal-free Sulfonylations of Methylthiolated Alkynones to Synthesize 3-sulfonylated Thioflavones. New J. Chem. 44, 14786–14790. doi:10.1039/d0nj03386c
Jiang, Y. Q., Li, J., Feng, Z. W., Xu, G. Q., Shi, X., Ding, Q. J., et al. (2020). Ethylene Glycol: A Green Solvent for Visible Light‐Promoted Aerobic Transition Metal‐Free Cascade Sulfonation/Cyclization Reaction. Adv. Synth. Catal. 362, 2609–2614. doi:10.1002/adsc.202000233
Kumar, P., and Bodas, M. S. (2001). A New Synthesis of 4H-1-Benzothiopyran-4-Ones Using (Trimethylsilyl)methylenetriphenylphosphorane. Tetrahedron 57, 9755–9758. doi:10.1016/s0040-4020(01)00977-2
Lee Jae, I. (2021). Novel Synthesis of Thioflavones and Their Pyridyl Analogs from 2-Mercaptobenzoic(nicotinic) Acid. J. Korean Phys. Soc. 65, 166–169. doi:10.5012/JKCS.2021.65.2.166
Liu, X. C., Chen, X. L., Liu, Y., Sun, K., Peng, Y. Y., Qu, L. B., et al. (2020). Visible‐Light‐Induced Metal‐Free Synthesis of 2‐Phosphorylated Thioflavones in Water. ChemSusChem 13, 298–303. doi:10.1002/cssc.201902817
Liu, Y., Zhang, X., Lv, J., Zhang, C., Chang, X., Ye, S., et al. (2022). A Photocatalytic Radical Relay Reaction of 2-methylthiolated Phenylalkynones and Potassium Metabisulfite. Org. Chem. Front. 9, 450–455. doi:10.1039/d1qo01658j
Ma, C.-H., Ji, Y., Zhao, J., He, X., Zhang, S.-T., Jiang, Y.-Q., et al. (2022). Transition-metal-free Three-Component Acetalation-Pyridylation of Alkenes via Photoredox Catalysis. Chin. J. Catal. 43, 571–583. doi:10.1016/s1872-2067(21)63917-7
Ma, C., Feng, Z., Li, J., Zhang, D., Li, W., Jiang, Y., et al. (2021b). Photocatalytic Transition-metal-free Direct 3-alkylation of 2-Aryl-2h-Indazoles in Dimethyl Carbonate. Org. Chem. Front. 8, 3286–3291. doi:10.1039/d1qo00064k
Ma, C., Li, Q., Zhao, M., Fan, G., Zhao, J., Zhang, D., et al. (2021a). Discovery of 1-Amino-1h-Imidazole-5-Carboxamide Derivatives as Highly Selective, Covalent Bruton's Tyrosine Kinase (BTK) Inhibitors. J. Med. Chem. 64, 16242–16270. doi:10.1021/acs.jmedchem.1c01559
Müller, K., Faeh, C., and Diederich, F. (2007). Fluorine in Pharmaceuticals: Looking beyond Intuition. Science 317, 1881–1886. doi:10.1126/science.1131943
Neogi, S., Ghosh, A. K., Majhi, K., Samanta, S., Kibriya, G., and Hajra, A. (2020). Organophotoredox-Catalyzed Direct C-H Amination of 2H-Indazoles with Amines. Org. Lett. 22, 5605–5609. doi:10.1021/acs.orglett.0c01973
Pan, G.-F., Zhu, X.-Q., Guo, R.-L., Gao, Y.-R., and Wang, Y.-Q. (2018). Synthesis of Enones and Enals via Dehydrogenation of Saturated Ketones and Aldehydes. Adv. Synth. Catal. 360, 4774–4783. doi:10.1002/adsc.201801058
Razdan, R. K., Bruni, R. J., Mehta, A. C., Weinhardt, K. K., and Papanastassiou, Z. B. (1978). A New Class of Antimalarial Drugs: Derivatives of Benzothiopyrans. J. Med. Chem. 21, 643–649. doi:10.1021/jm00205a010
Sangeetha, S., and Sekar, G. (2019). Copper-Catalyzed One-Pot Synthesis of 2-Arylthiochromenones: An In Situ Recycle of Waste Byproduct as Useful Reagent. Org. Lett. 21, 75–79. doi:10.1021/acs.orglett.8b03508
Schiesser, S., Chepliaka, H., Kollback, J., Quennesson, T., Czechtizky, W., and Cox, R. J. (2020). N-trifluoromethyl Amines and Azoles: An Underexplored Functional Group in the Medicinal Chemist's Toolbox. J. Med. Chem. 63, 13076–13089. doi:10.1021/acs.jmedchem.0c01457
Shen, J., Xu, J., He, L., Liang, C., and Li, W. (2022). Application of Langlois’ Reagent (NaSO2CF3) in C-H Functionalisation. Chin. Chem. Lett. 33, 1227–1235. doi:10.1016/j.cclet.2021.09.005
Tang, S., Deng, Y.-L., Li, J., Wang, W.-X., Ding, G.-L., Wang, M.-W., et al. (2015). Synthesis of Perfluorinated Isoquinolinediones through Visible-Light-Induced Cyclization of Alkenes. J. Org. Chem. 80, 12599–12605. doi:10.1021/acs.joc.5b01803
Tang, S., Yuan, L., Deng, Y.-L., Li, Z.-Z., Wang, L.-N., Huang, G.-X., et al. (2017b). Visible-light-induced Perfluoroalkylation/arylmigration/desulfonylation Cascades of Conjugated Tosyl Amides. Tetrahedron Lett. 58, 329–332. doi:10.1016/j.tetlet.2016.12.027
Tang, S., Yuan, L., Li, Z.-Z., Peng, Z.-Y., Deng, Y.-L., Wang, L.-N., et al. (2017a). Visible-light-induced Dearomative Spirocyclization of N -benzylacrylamides toward Perfluorinated Azaspirocyclic Cyclohexadienones. Tetrahedron Lett. 58, 2127–2130. doi:10.1016/j.tetlet.2017.04.055
Wang, H.-K., Bastow, K. F., Cosentino, L. M., and Lee, K.-H. (1996). Antitumor Agents. 166. Synthesis and Biological Evaluation of 5,6,7,8-Substituted-2-Phenylthiochromen-4-Ones. J. Med. Chem. 39, 1975–1980. doi:10.1021/jm960008c
Wang, L., Wang, H., Meng, W., Xu, X.-H., and Huang, Y. (2021). Facile Syntheses of 3-trifluoromethylthio Substituted Thioflavones and Benzothiophenes via the Radical Cyclization. Chin. Chem. Lett. 32, 389–392. doi:10.1016/j.cclet.2020.02.040
Wang, S.-W., Yu, J., Zhou, Q.-Y., Chen, S.-Y., Xu, Z.-H., and Tang, S. (2019). Visible-Light-Induced Atom Transfer Radical Addition and Cyclization of Perfluoroalkyl Halides with 1,n-Enynes. ACS Sustain. Chem. Eng. 7, 10154–10162. doi:10.1021/acssuschemeng.9b02178
Wang, W., Bao, Z.-P., Qi, X., and Wu, X.-F. (2021). Nickel-Catalyzed One-Pot Carbonylative Synthesis of 2-Mono- and 2,3-Disubstituted Thiochromenones from 2-Bromobenzenesulfonyl Chlorides and Alkynes. Org. Lett. 23, 6589–6593. doi:10.1021/acs.orglett.1c02442
Xu, J., Zhang, F., Zhang, S., Zhang, L., Yu, X., Yan, J., et al. (2019). Radical Promoted C(sp2)-S Formation and C(sp3)-S Bond Cleavage: Access to 2-Substituted Thiochromones. Org. Lett. 21, 1112–1115. doi:10.1021/acs.orglett.9b00023
Yang, M., Han, H., Jiang, H., Ye, S., Fan, X., and Wu, J. (2021). Photoinduced Reaction of Potassium Alkyltrifluoroborates, Sulfur Dioxide and Para-Quinone Methides via Radical 1,6-addition. Chin. Chem. Lett. 32, 3535–3538. doi:10.1016/j.cclet.2021.05.007
Yang, W., Zhang, M., Chen, W., Yang, X., and Feng, J. (2020). Recent Progress in the Synthesis of Sulfur-Containing Heterocycles Using Sulfur Atom as Radical Acceptors. Chin. J. Org. Chem. 40, 4060–4070. doi:10.6023/cjoc202005039
Zeng, F.-L., Zhu, H.-L., Chen, X.-L., Qu, L.-B., and Yu, B. (2021). Visible Light-Induced Recyclable G-C3n4 Catalyzed Thiocyanation of C(sp2)-H Bonds in Sustainable Solvents. Green Chem. 23, 3677–3682. doi:10.1039/d1gc00938a
Zheng, X., Zhong, T., Zhang, L., Chen, J., Chen, Z., Jiang, X., et al. (2020). Radical-Triggered Cyclization of Methylthio-Substituted Alkynones: Synthesis of Diverse 3-Alkylthiochromones. Eur. J. Org. Chem. 2020, 4534–4541. doi:10.1002/ejoc.202000663
Zhou, C., Dubrovsky, A. V., and Larock, R. C. (2006). Diversity-Oriented Synthesis of 3-Iodochromones and Heteroatom Analogues via ICl-Induced Cyclization. J. Org. Chem. 71, 1626–1632. doi:10.1021/jo0523722
Zhu, H.-L., Zeng, F.-L., Chen, X.-L., Sun, K., Li, H.-C., Yuan, X.-Y., et al. (2021). Acyl Radicals from α-Keto Acids: Metal-free Visible-Light-Promoted Acylation of Heterocycles. Org. Lett. 23, 2976–2980. doi:10.1021/acs.orglett.1c00655
Keywords: photocatalysis, perfluoroalkylation, cyclization, thioflavone, antitumor
Citation: Ma C, Meng H, He X, Jiang Y and Yu B (2022) Visible-Light-Promoted Transition-Metal-Free Construction of 3-Perfluoroalkylated Thioflavones. Front. Chem. 10:953978. doi: 10.3389/fchem.2022.953978
Received: 26 May 2022; Accepted: 15 June 2022;
Published: 13 July 2022.
Edited by:
Zhiyuan Chen, Jiangxi Normal University, ChinaReviewed by:
Indubhusan Deb, Indian Institute of Chemical Biology (CSIR), IndiaYong Luo, Sun Yat-sen University, China
Copyright © 2022 Ma, Meng, He, Jiang and Yu. This is an open-access article distributed under the terms of the Creative Commons Attribution License (CC BY). The use, distribution or reproduction in other forums is permitted, provided the original author(s) and the copyright owner(s) are credited and that the original publication in this journal is cited, in accordance with accepted academic practice. No use, distribution or reproduction is permitted which does not comply with these terms.
*Correspondence: Yuqin Jiang, jiangyuqin@htu.edu.cn; Bing Yu, bingyu@zzu.edu.cn