- 1College of Electronic and Optical Engineering & College of Flexible Electronics (Future Technology), Nanjing University of Posts and Telecommunications, Nanjing, China
- 2College of Chemistry and Chemical Engineering, Qingdao University, Qingdao, China
Photocatalysis is a potential strategy to solve energy and environmental problems. The development of new sustainable photocatalysts is a current topic in the field of photocatalysis. ZnIn2S4, a visible light-responsive photocatalyst, has attracted extensive research interest in recent years. Due to its suitable band gap, strong chemical stability, durability, and easy synthesis, it is expected to become a new hot spot in the field of photocatalysis in the near future. This mini-review presents a comprehensive summary of the modulation strategies to effectively improve the photocatalytic activity of ZnIn2S4 such as morphology and structural engineering, defects engineering, doping engineering, and heterojunction engineering. This review aims to provide reference to the proof-of-concept design of highly active ZnIn2S4-based photocatalysts for the enhanced hydrogen evolution reaction.
Introduction
Since 1972, when Fujishima and Honda demonstrated that hydrogen can be produced from water by the photoelectrochemical reaction using a TiO2 photoelectrode, photocatalytic technology has provided a feasible strategy for hydrogen generation. The key to catalytic hydrogen evolution lies in the development and utilization of catalysts (Zou et al., 2019; Wu et al., 2021; Zhitong Wang et al., 2021; Bhavani et al., 2022). For photocatalytic catalysts, hydrogen is produced by reducing hydrogen ions using electrons and protons generated in sunlight. So far, researchers have developed a variety of types of semiconductor photocatalysts, such as oxide-type semiconductor photocatalysts (Zhang et al., 2017; Zhao Zhang et al., 2018; Idris et al., 2020), nitrogen (oxygen) compound-type semiconductor photocatalysts (Shuqu Zhang et al., 2018; Zhu et al., 2022), and sulfide-type semiconductor photocatalysts (Kuang et al., 2016; Ruijie Yang et al., 2021). Metal sulfide has become one of the most important semiconductor materials due to its excellent visible light response, suitable band gap structure, and low cost (Song et al., 2021; Zhitong Wang et al., 2021; Zhou et al., 2022). However, CdS and CdIn2S4 still have some obstacles, such as rapid recombination of photogenic electrons and holes, low specific surface area, and photocorrosion. Therefore, it is necessary to determine an effective method to improve the activity and stability of sulfide semiconductors.
Among the semiconductor photocatalysts currently studied, ZnIn2S4 as one of the ternary metal sulfides has attracted extensive attention due to its narrow band gap, good chemical stability, and strong photoelectric conversion ability. Compared with single metal sulfides (CdS and ZnS etc.), ZnIn2S4 has more excellent photoelectric characteristics, physical and chemical stability, and environmental friendliness and has greater durability in photocatalytic reactions.
Compared with other ternary metal sulfides, ZnIn2S4 is the only AB2X4 series compound with a layered structure, non-toxic, and has a convenient synthesis process; the unique cone structure has a huge surface area for the photocatalytic reaction and also provides plenty of active sites, which makes ZnIn2S4 have greater application value in the field of energy conversion (Jie Wang et al., 2021; Man Wang et al., 2021). However, the high photoexcited charge recombination ratio makes ZnIn2S4 unable to effectively utilize solar energy, and the photocatalytic efficiency is significantly limited (Tu et al., 2018; Chao et al., 2021a; Yijin Wang et al., 2021; Yu et al., 2022). Some potential strategies such as ion doping, morphology regulation, design defects, heterojunction structure design, and loading cocatalyst have been explored (Figure 1).
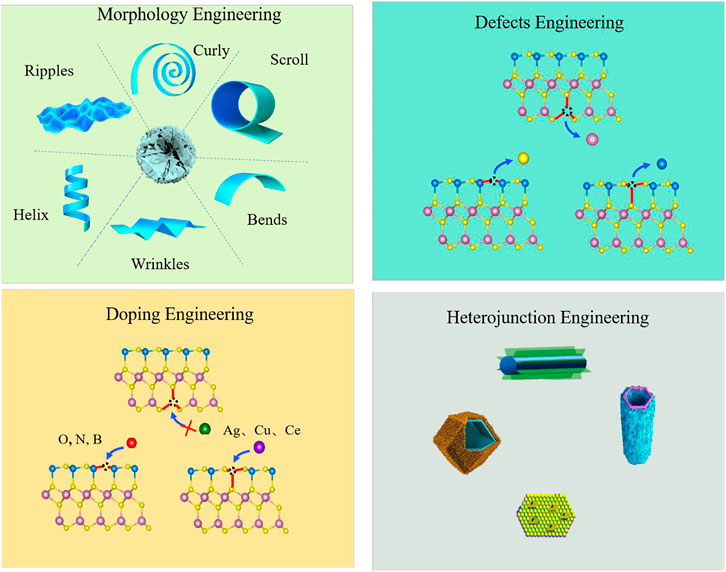
FIGURE 1. Potential strategies of ZnIn2S4-based photocatalysts for the enhanced hydrogen evolution reaction.
Optimization of Photocatalytic Hydrogen Evolution Performance of ZnIn2S4
Morphology and Structural Engineering
1. It is well known that precise control of the morphology of semiconductor photocatalysts plays an important role in improving their physical and chemical properties and the performance of photocatalytic systems (Xie et al., 2021; Xuehua Wang et al., 2021; Cheng et al., 2022; Mingming Liu et al., 2022; Wang et al., 2022; Xia Liu et al., 2022). The photocatalytic performance of ZnIn2S4 photocatalyst can be significantly improved by morphological adjustment, which can be attributed to the following four factors: 1) the specific surface area of ZnIn2S4 can be increased; 2) it promotes mass transfer and light capture; 3) it is beneficial to expose more active sites on the surface that can participate in redox reactions; 4) it shortens the migration distance and accelerates the migration speed of photogenerated carriers. Therefore, researchers have explored different morphologies of ZnIn2S4 as a photocatalyst to improve its photocatalytic performance, including nanosheets, nanoflowers, nanowires, nanorods, nanorings, and nanotubes (Guan et al., 2018; Xu et al., 2021).
Doping Engineering
Element doping can extend the scope of light absorption, add catalytic sites of a photocatalyst, and adjust the hydrogen adsorption and desorption characteristics (Ida et al., 2018; Quan Zhang et al., 2021; Hou et al., 2022). By introducing donor/acceptor energy levels into the doped ions in semiconductors, the concentration and energy distribution of carriers near the conduction band/valence band edge can be adjusted to improve the electron transition behavior. Therefore, with the rapid development of research on photocatalyst modification of ZnIn2S4, many researchers are committed to introducing cations or anions into ZnIn2S4.
Cationic doping caused Fermi levels to pass through the conduction band, giving the material metallic properties, thus improving the conductivity and photocarrier migration ability. For example, Qiu et al. (Pan et al., 2021; Qiu et al., 2021; Shi et al., 2022) introduced nickel ions into the ZnIn2S4 lattice by the solvothermal method and prepared Ni-doped ZnIn2S4 nanosheets with few layers. The photocatalytic activity of ZnIn2S4 nanosheets was about seven times higher than that of pure ZnIn2S4 nanosheets. Theoretical calculations show that Ni ions are preferentially embedded in the zinc rather than indium sites in tetrahedrons, which induced a narrower band gap, higher electronic conductivity, and more charge carriers that can participate in the hydrogen evolution process. More importantly, Ni dopants can subtly change the electronic structure of the S site and achieve the optimal free energy of hydrogen adsorption on Ni by fine-tuning the S−H bond energy. Therefore, the Ni doping in ZnIn2S4 nanoparticles can prolong the lifetime of the photoexcited charge and then enhance the activity of photocatalytic hydrogen evolution. Therefore, the doping of metal cations in the photocatalyst can improve the light absorption range and contribute to the enhancement of photocatalytic activity. On the contrary, anion (O, N, and P, etc.) doping can regulate the valence band to promote the migration of holes and adjust the conduction band to enhance the reduction ability of photogenerated electrons (Goswami et al., 2021; Shuqu Zhang et al., 2021).
Defects Engineering
Defects engineering is applied to photocatalysts to improve the separation efficiency of photocarriers. The introduced defects can be used as a center to capture photocarriers and prevent their recombination, thus improving charge separation and exposing more active sites. Vacancies in photocatalysts are typical point defects, which play an important role in improving photocatalytic performance due to their regulation of physicochemical and photoelectrochemical properties such as photocarrier migration, light absorption, surface acidity and alkalinity, surface active sites, adsorption properties, solubility properties, and electronic structure. In recent years, with the increasing interest in ZnIn2S4 photocatalysts, the studies on vacancy engineering based on ZnIn2S4 (sulfur, zinc, and indium vacancies) are also increasing (Lee et al., 2019; Pengfei Wang et al., 2019).
Yu Liu et al. (2022) proposed preparing ZnIn2S4 microspheres with S vacancy defects through solvothermal and low-temperature hydrogenation reduction strategies. Due to the formation of S vacancy defects on the surface, the band gap of ZnIn2S4 microspheres was reduced to 2.38 eV, which has good visible light response activity. Experimental results and density functional theory calculations showed that the surface S vacancy caused by the surface field potential difference promotes the spatial separation of electrons and holes, thus improving the performance of the photocatalyst and greatly deepening the surface defects engineering understanding of how to affect the separation of light raw charge and to find other efficient and stable metal sulfide photocatalysts which provides a new train of thought. Tai and Zhou, (2021) used reactive ion etching to generate Zn vacancies in ZnIn2S4 particles. With the increase of Zn vacancy concentration, the band gap of ZnIn2S4 decreases from 2.17 to 2.06 eV. Under the optimum Zn vacancy concentration, the photocatalytic hydrogen evolution rate of ZnIn2S4 is 2.7 times higher than that of pure ZnIn2S4, and the photocatalytic process of ZnIn2S4 is stable without any degradation through cyclic experiments, showing good stability. The existence of Zn vacancies reduces the charge carrier transfer resistance, improves the charge separation rate, and prolongs the emission decay life (He et al., 2019).
In the sandwich ZnIn2S4 stacking structure, Zn or S atoms exposed to the surface are easily desorbed, resulting in Zn or S defects. However, due to steric hindrance, the atoms located in the middle layer of a sandwich structure are difficult to be removed, so it is difficult to form in-layer defects. Zn or S defects promote the directional migration of photogenerated electrons but have little effect on hole regulation. Luan et al, (2022) successfully prepared ultra-thin ZnIn2S4 nanosheets with an abundant [InS]6 intermediate layer and perfect [InS]4 and [ZnS]4 surface layer by controlling the crystal growth of ZnIn2S4 with the rapid heating and hydrothermal method. The in vacancy induces the redistribution of orbitals near the maximum value of the valence band, separates the oxidation and reduction sites on both sides of the ultra-thin ZnIn2S4 nanosheet with in vacancy, and increases the density of states between the valence band and the conduction band. The electrons around indium vacancy are delocalized, which is conducive to the interlayer charge transfer and improves the conductivity of the ZnIn2S4 nanosheet.
Heterojunction Engineering
In order to overcome the inherent shortcomings of the high electron–hole recombination rate and low utilization rate of a single unmodified semiconductor photocatalyst, the construction of heterojunction by coupling two semiconductor materials is generally considered to be an effective strategy (Yang et al., 2020; Liu et al., 2021; Xu et al., 2022). The construction of heterojunction with a suitable band position will form a potential gradient between the heterogeneous interfaces to promote the separation and transfer of photocarriers and can also enhance the optical capture performance (Zhao et al., 2019; Mu et al., 2020; Zhang et al., 2020; Zuo et al., 2020). According to the band orientation and carrier transfer path, the structure of heterojunction photocatalyst can be divided into many types, including Type−I (transboundary state photocatalyst), Type−II (alternate state photocatalyst), Z-scheme (alternate state photocatalyst), and Mott−Schottky type (Yang et al., 2018; Li et al., 2019; Hu et al., 2020). In recent years, various heterostructures based on ZnIn2S4 have been successfully constructed, and their photocatalytic properties in energy and environmental applications have been studied (Zhenfei Yang et al., 2021; Chen et al., 2022).
The Type 1 heterojunction is a kind of semiconductor heterojunction in which the valence band and conduction band of one semiconductor are located between the valence band and conduction band of the other one. Under the irradiation of incident light, the conduction band to the electronic from high to low conduction band direction and the hole from low to high with direction, in the process of a photocatalytic oxidation–reduction reaction, will be two semiconductor materials to bring to the lower conduction band and a high price (Chao et al., 2021b; Longlu Wang et al., 2021). Different from Type−I heterojunction, Type−II heterojunction is formed by a staggered conduction band and valence band of two semiconductor materials (Jiang et al., 2019; Yan et al., 2019). The movement direction of charge carriers and redox reaction sites of Type−I heterojunction is the same as that of Type−I heterojunction. Due to structural differences, Type−II heterojunction can effectively promote the separation of photogenerated carriers and inhibit their recombination, and the energy conversion efficiency is significantly improved (Zizhong Zhang et al., 2018; Zhao et al., 2021). Although Type−II heterojunction photocatalysts exhibit good photocatalytic performance, such high photocatalytic performance sacrifices the redox ability of charge carriers, so the reduced driving force may not smoothly drive the specific photocatalytic reaction. Due to the well matching of the electronic band structure of the two semiconductor materials, the Z-scheme heterojunction keeps the electrons at a more negative potential and the holes at a corrected potential, resulting in a strong redox ability (Sabbah et al., 2022; Su et al., 2022).
Perspectives
This review presents a comprehensive summary of the modulation strategies to effectively improve the photocatalytic activity of ZnIn2S4 such as morphology and structural engineering, defects engineering, doping engineering, and heterojunction engineering. Although a large number of promising results have been achieved in photocatalytic HER for ZnIn2S4, there are still many untapped areas to be investigated to realize their full potential. Branched flower-like nanostructures with atomically thin petals are usually obtained by liquid-phase synthesis. This morphology is very favorable for catalysis because it maximizes exposure of active sites, rather than planar stacking like 2D nanosheets. The next key challenge lies in understanding the nucleation stage in the liquid phase so that the platelet topography can be controlled reliably. Further work should be able to develop more precise crystal growth methods and gain full control of defects/doping elements/functional groups to identify, quantify, and ultimately develop active sites. At the same time, complementary advanced characterization techniques, such as nanoscale STM, XAFS, HAADF-STEM, positron annihilation spectroscopy, and ultrafast transient absorption spectroscopy, also need to be developed in parallel to probe the reaction kinetics at the atomic or molecular scale. With the synergistic development of robust and novel ultrathin 2D materials, catalytic hydrogen evolution technology is expected to achieve greater breakthroughs (Hu et al., 2013; Bingqing Wang et al., 2019).
Author Contributions
MT and WY wrote the manuscript. FZ collected references. XL and LW supervised the whole work. All the authors approved this manuscript.
Funding
This work was financially supported by the Natural Science Foundation of China (51902101), the Youth Natural Science Foundation of Hunan Province (2021JJ540044), the Natural Science Foundation of Jiangsu Province (BK20201381), and the Science Foundation of Nanjing University of Posts and Telecommunications (NY219144).
Conflict of Interest
The authors declare that the research was conducted in the absence of any commercial or financial relationships that could be construed as a potential conflict of interest.
Publisher’s Note
All claims expressed in this article are solely those of the authors and do not necessarily represent those of their affiliated organizations or those of the publisher, the editors, and the reviewers. Any product that may be evaluated in this article, or claim that may be made by its manufacturer, is not guaranteed or endorsed by the publisher.
References
Bhavani, P., Praveen Kumar, D., Hussain, M., Aminabhavi, T. M., and Park, Y.-K. (2022). Eco-friendly Rice Husk Derived Biochar as a Highly Efficient Noble Metal-free Cocatalyst for High Production of H2 Using Solar Light Irradiation. Chem. Eng. J. 434, 134743. doi:10.1016/j.cej.2022.134743
Bingqing Wang, B., Ding, Y., Deng, Z., and Li, Z. (2019). Rational Design of Ternary NiS/CQDs/ZnIn2S4 Nanocomposites as Efficient Noble-metal-free Photocatalyst for Hydrogen Evolution under Visible Light. Chin. J. Catal. 40 (3), 335–342. doi:10.1016/s1872-2067(18)63159-6
Chao, Y., Zhang, W., Zhou, P., Chen, H., Lu, S., Li, M., et al. (2021a). An In-Situ NH4+-etched Strategy for Anchoring Atomic Mo Site on ZnIn2S4 Hierarchical Nanotubes for Superior Hydrogen Photocatalysis. Sci. China Chem. 64, 1716–1722. doi:10.1007/s11426-021-1063-2
Chao, Y., Zhou, P., Lai, J., Zhang, W., Yang, H., Lu, S., et al. (2021b). Ni 1− X Co X Se 2 -C/ZnIn 2 S 4 Hybrid Nanocages with Strong 2D/2D Hetero‐Interface Interaction Enable Efficient H 2 ‐Releasing Photocatalysis. Adv. Funct. Mat. 31, 2100923. doi:10.1002/adfm.202100923
Chen, J., Tang, Y., Wang, S., Xie, L., Chang, C., Cheng, X., et al. (2022). Ingeniously Designed Ni-Mo-S/ZnIn2S4 Composite for Multi-Photocatalytic Reaction Systems. Chin. Chem. Lett. 33 (3), 1468–1474. doi:10.1016/j.cclet.2021.08.103
Cheng, X., Wang, L., Xie, L., Sun, C., Zhao, W., Liu, X., et al. (2022). Defect-driven Selective Oxidation of MoS2 Nanosheets with Photothermal Effect for Photo-Catalytic Hydrogen Evolution Reaction. Chem. Eng. J. 439, 135757. doi:10.1016/j.cej.2022.135757
Goswami, T., Yadav, D. K., Bhatt, H., Kaur, G., Shukla, A., Babu, K. J., et al. (2021). Defect-Mediated Slow Carrier Recombination and Broad Photoluminescence in Non-metal-doped ZnIn2S4 Nanosheets for Enhanced Photocatalytic Activity. J. Phys. Chem. Lett. 12, 5000–5008. doi:10.1021/acs.jpclett.1c01203
Guan, Z., Xu, Z., Li, Q., Wang, P., Li, G., and Yang, J. (2018). AgIn5S8 Nanoparticles Anchored on 2D Layered ZnIn2S4 to Form 0D/2D Heterojunction for Enhanced Visible-Light Photocatalytic Hydrogen Evolution. Appl. Catal. B Environ. 227, 512–518. doi:10.1016/j.apcatb.2018.01.068
He, Y., Rao, H., Song, K., Li, J., Yu, Y., Lou, Y., et al. (2019). 3D Hierarchical ZnIn 2 S 4 Nanosheets with Rich Zn Vacancies Boosting Photocatalytic CO 2 Reduction. Adv. Funct. Mat. 29 (45), 1905153. doi:10.1002/adfm.201905153
Hou, Z., Sun, Z., Cui, C., Zhu, D., Yang, Y., and Zhang, T. (2022). Ru Coordinated ZnIn 2 S 4 Triggers Local Lattice‐Strain Engineering to Endow High‐Efficiency Electrocatalyst for Advanced Zn‐Air Batteries. Adv. Funct. Mater. 32, 2110572. doi:10.1002/adfm.202110572
Hu, P., Pramana, S. S., Cao, S., Ngaw, C. K., Lin, J., Loo, S. C. J., et al. (2013). Ion-induced Synthesis of Uniform Single-Crystalline Sulphide-Based Quaternary-Alloy Hexagonal Nanorings for Highly Efficient Photocatalytic Hydrogen Evolution. Adv. Mat. 25, 2567–2572. doi:10.1002/adma.201204545
Hu, J., Chen, C., Zheng, Y., Zhang, G., Guo, C., and Li, C. M. (2020). Spatially Separating Redox Centers on Z‐Scheme ZnIn 2 S 4 /BiVO 4 Hierarchical Heterostructure for Highly Efficient Photocatalytic Hydrogen Evolution. Small 16 (37), 2002988. doi:10.1002/smll.202002988
Ida, S., Sato, K., Nagata, T., Hagiwara, H., Watanabe, M., Kim, N., et al. (2018). A Cocatalyst that Stabilizes a Hydride Intermediate during Photocatalytic Hydrogen Evolution over a Rhodium-Doped TiO2 Nanosheet. Angew. Chem. Int. Ed. 57, 9073–9077. doi:10.1002/anie.201803214
Idris, A. M., Liu, T., Hussain Shah, J., Han, H., and Li, C. (2020). Sr2CoTaO6 Double Perovskite Oxide as a Novel Visible-Light-Absorbing Bifunctional Photocatalyst for Photocatalytic Oxygen and Hydrogen Evolution Reactions. ACS Sustain. Chem. Eng. 8 (37), 14190–14197. doi:10.1021/acssuschemeng.0c05237
Jiang, R., Wu, D., Lu, G., Yan, Z., and Liu, J. (2019). Modified 2D-2D ZnIn2S4/BiOCl van der Waals heterojunctions with CQDs: Accelerated charge transfer and enhanced photocatalytic activity under vis- and NIR-light. Chemosphere 227, 82–92. doi:10.1016/j.chemosphere.2019.04.038
Jie Wang, J., Sun, S., Zhou, R., Li, Y., He, Z., Ding, H., et al. (2021). A Review: Synthesis, Modification and Photocatalytic Applications of ZnIn2S4. J. Mater. Sci. Technol. 78, 1–19. doi:10.1016/j.jmst.2020.09.045
Kuang, P. Y., Zheng, P. X., Liu, Z. Q., Lei, J. L., Wu, H., Li, N., et al. (2016). Embedding Au Quantum Dots in Rimous Cadmium Sulfide Nanospheres for Enhanced Photocatalytic Hydrogen Evolution. Small 12, 6735–6744. doi:10.1002/smll.201602870
Lee, J., Kim, H., Lee, T., Jang, W., Lee, K. H., and Soon, A. (2019). Revisiting Polytypism in Hexagonal Ternary Sulfide ZnIn2S4 for Photocatalytic Hydrogen Production within the Z-Scheme. Chem. Mat. 31 (21), 9148–9155. doi:10.1021/acs.chemmater.9b03539
Li, Z., Wang, X., Tian, W., Meng, A., and Yang, L. (2019). CoNi Bimetal Cocatalyst Modifying a Hierarchical ZnIn2S4 Nanosheet-Based Microsphere Noble-Metal-Free Photocatalyst for Efficient Visible-Light-Driven Photocatalytic Hydrogen Production. ACS Sustain. Chem. Eng. 7 (24), 20190–20201. doi:10.1021/acssuschemeng.9b06430
Liu, T., Yang, K., Gong, H., and Jin, Z. (2021). Visible-light Driven S-Scheme Mn0.2Cd0.8S/CoTiO3 Heterojunction for Photocatalytic Hydrogen Evolution. Renew. Energy 173, 389–400. doi:10.1016/j.renene.2021.03.146
Longlu Wang, L., Xie, L., Zhao, W., Liu, S., and Zhao, Q. (2021). Oxygen-facilitated Dynamic Active-Site Generation on Strained MoS2 during Photo-Catalytic Hydrogen Evolution. Chem. Eng. J. 405, 127028. doi:10.1016/j.cej.2020.127028
Luan, Q., Xue, X., Li, R., Gu, L., Dong, W., Zhou, D., et al. (2022). Boosting Photocatalytic Hydrogen Evolution: Orbital Redistribution of Ultrathin ZnIn2S4 Nanosheets via Atomic Defects. Appl. Catal. B Environ. 305, 121007. doi:10.1016/j.apcatb.2021.121007
Man Wang, M., Zhang, G., Guan, Z., Yang, J., and Li, Q. (2021). Spatially Separating Redox Centers and Photothermal Effect Synergistically Boosting the Photocatalytic Hydrogen Evolution of ZnIn 2 S 4 Nanosheets. Small 17, 2006952. doi:10.1002/smll.202006952
Mingming Liu, M., Li, H., Liu, S., Wang, L., Xie, L., Zhuang, Z., et al. (2022). Tailoring Activation Sites of Metastable Distorted 1T′-phase MoS2 by Ni Doping for Enhanced Hydrogen Evolution. Nano Res., 524–530. doi:10.1007/s12274-022-4267-9
Mu, F., Cai, Q., Hu, H., Wang, J., Wang, Y., Zhou, S., et al. (2020). Construction of 3D Hierarchical Microarchitectures of Z-Scheme UiO-66-(COOH)2/ZnIn2S4 Hybrid Decorated with Non-noble MoS2 Cocatalyst: A Highly Efficient Photocatalyst for Hydrogen Evolution and Cr(VI) Reduction. Chem. Eng. J. 384, 123352. doi:10.1016/j.cej.2019.123352
Pan, J., Zhang, G., Guan, Z., Zhao, Q., Li, G., Yang, J., et al. (2021). Anchoring Ni Single Atoms on Sulfur-Vacancy-Enriched ZnIn2S4 Nanosheets for Boosting Photocatalytic Hydrogen Evolution. J. Energy Chem. 58, 408–414. doi:10.1016/j.jechem.2020.10.030
Pengfei Wang, P., Shen, Z., Xia, Y., Wang, H., Zheng, L., Xi, W., et al. (2019). Atomic Insights for Optimum and Excess Doping in Photocatalysis: A Case Study of Few-Layer Cu-ZnIn2 S4. Adv. Funct. Mat. 29 (3), 1807013. doi:10.1002/adfm.201807013
Qiu, B., Huang, P., Lian, C., Ma, Y., Xing, M., Liu, H., et al. (2021). Realization of All-In-One Hydrogen-Evolving Photocatalysts via Selective Atomic Substitution. Appl. Catal. B Environ. 298, 120518. doi:10.1016/j.apcatb.2021.120518
Quan Zhang, Q., Gu, H., Wang, X., Li, L., Zhang, J., Zhang, H., et al. (2021). Robust Hollow Tubular ZnIn2S4 Modified with Embedded Metal-Organic-Framework-Layers: Extraordinarily High Photocatalytic Hydrogen Evolution Activity under Simulated and Real Sunlight Irradiation. Appl. Catal. B Environ. 298, 120632. doi:10.1016/j.apcatb.2021.120632
Ruijie Yang, R., Mei, L., Fan, Y., Zhang, Q., Zhu, R., Amal, R., et al. (2021). ZnIn 2 S 4 ‐Based Photocatalysts for Energy and Environmental Applications. Small Methods 5 (10), 2100887. doi:10.1002/smtd.202100887
Sabbah, A., Shown, I., Qorbani, M., Fu, F.-Y., Lin, T.-Y., Wu, H.-L., et al. (2022). Boosting Photocatalytic CO2 Reduction in a ZnS/ZnIn2S4 Heterostructure through Strain-Induced Direct Z-Scheme and a Mechanistic Study of Molecular CO2 Interaction Thereon. Nano Energy 93, 106809. doi:10.1016/j.nanoen.2021.106809
Shi, X., Dai, C., Wang, X., Hu, J., Zhang, J., Zheng, L., et al. (2022). Protruding Pt Single-Sites on Hexagonal ZnIn2S4 to Accelerate Photocatalytic Hydrogen Evolution. Nat. Commun. 13, 1–10. doi:10.1038/s41467-022-28995-1
Shuqu Zhang, S., Liu, X., Liu, C., Luo, S., Wang, L., Cai, T., et al. (2018). MoS2 Quantum Dot Growth Induced by S Vacancies in a ZnIn2S4 Monolayer: Atomic-Level Heterostructure for Photocatalytic Hydrogen Production. ACS Nano 12 (1), 751–758. doi:10.1021/acsnano.7b07974
Shuqu Zhang, S., Zhang, Z., Si, Y., Li, B., Deng, F., Yang, L., et al. (2021). Gradient Hydrogen Migration Modulated with Self-Adapting S Vacancy in Copper-Doped ZnIn2S4 Nanosheet for Photocatalytic Hydrogen Evolution. ACS Nano 15 (9), 15238–15248. doi:10.1021/acsnano.1c05834
Song, Y., Zhang, J., Dong, X., and Li, H. (2021). A Review and Recent Developments in Full‐Spectrum Photocatalysis Using ZnIn 2 S 4 ‐Based Photocatalysts. Energy Tech. 9, 2100033. doi:10.1002/ente.202100033
Su, T., Men, C., Chen, L., Chu, B., Luo, X., Ji, H., et al. (2022). Sulfur Vacancy and Ti 3 C 2 T X Cocatalyst Synergistically Boosting Interfacial Charge Transfer in 2D/2D Ti 3 C 2 T X /ZnIn 2 S 4 Heterostructure for Enhanced Photocatalytic Hydrogen Evolution. Adv. Sci. 9, 2103715. doi:10.1002/advs.202103715
Tai, L., and Zhou, Y. (2021). Creating Zinc Vacancy within 3D Hierarchical ZnIn2S4 Particles for Boosted Photocatalytic Performance towards H2 Evolution Reaction. Ceram. Int. 47, 32218–32225. doi:10.1016/j.ceramint.2021.08.115
Tu, X., Lu, J., Li, M., Su, Y., Yin, G., and He, D. (2018). Hierarchically ZnIn2S4 Nanosheet-Constructed Microwire Arrays: Template-free Synthesis and Excellent Photocatalytic Performances. Nanoscale 10, 4735–4744. doi:10.1039/c7nr09413b
Wang, S., Wang, L., Xie, L., Zhao, W., Liu, X., Zhuang, Z., et al. (2022). Dislocation-strained MoS2 Nanosheets for High-Efficiency Hydrogen Evolution Reaction. Nano Res. 15, 4996–5003. doi:10.1007/s12274-022-4158-0
Wu, Y., Yao, S., Lv, G., Wang, Y., Zhang, H., Liao, P., et al. (2021). Construction of P-N Junctions in Single-Unit-Cell ZnIn2S4 Nanosheet Arrays toward Promoted Photoelectrochemical Performance. J. Catal. 401, 262–270. doi:10.1016/j.jcat.2021.08.009
Xia Liu, X., Hou, Y., Tang, M., and Wang, L. (2022). Atom Elimination Strategy for MoS2 Nanosheets to Enhance Photocatalytic Hydrogen Evolution. Chin. Chem. Lett. doi.doi:10.1016/j.cclet.2022.05.003
Xie, L., Wang, L., Zhao, W., Liu, S., Huang, W., and Zhao, Q. (2021). WS2 Moiré Superlattices Derived from Mechanical Flexibility for Hydrogen Evolution Reaction. Nat. Commun. 12 (1), 5070. doi:10.1038/s41467-021-25381-1
Xu, W., Gao, W., Meng, L., Tian, W., and Li, L. (2021). Incorporation of Sulfate Anions and Sulfur Vacancies in ZnIn 2 S 4 Photoanode for Enhanced Photoelectrochemical Water Splitting. Adv. Energy Mat. 11 (26), 2101181. doi:10.1002/aenm.202101181
Xu, Y., Yan, A., Jiang, L., Huang, F., Hu, D., Duan, G., et al. (2022). MoS2/HCSs/ZnIn2S4 Nanocomposites with Enhanced Charge Transport and Photocatalytic Hydrogen Evolution Performance. J. Alloys Compd. 895, 162504. doi:10.1016/j.jallcom.2021.162504
Xuehua Wang, X., Wang, X., Huang, J., Li, S., Meng, A., and Li, Z. (2021). Interfacial Chemical Bond and Internal Electric Field Modulated Z-Scheme Sv-ZnIn2S4/MoSe2 Photocatalyst for Efficient Hydrogen Evolution. Nat. Commun. 12, 1–11. doi:10.1038/s41467-021-24511-z
Yan, A., Shi, X., Huang, F., Fujitsuka, M., and Majima, T. (2019). Efficient Photocatalytic H2 Evolution Using NiS/ZnIn2S4 Heterostructures with Enhanced Charge Separation and Interfacial Charge Transfer. Appl. Catal. B Environ. 250, 163–170. doi:10.1016/j.apcatb.2019.02.075
Yang, G., Ding, H., Chen, D., Feng, J., Hao, Q., and Zhu, Y. (2018). Construction of Urchin-like ZnIn2S4-Au-TiO2 Heterostructure with Enhanced Activity for Photocatalytic Hydrogen Evolution. Appl. Catal. B Environ. 234, 260–267. doi:10.1016/j.apcatb.2018.04.038
Yang, Z., Shao, L., Wang, L., Xia, X., Liu, Y., Cheng, S., et al. (2020). Boosted Photogenerated Carriers Separation in Z-Scheme Cu3P/ZnIn2S4 Heterojunction Photocatalyst for Highly Efficient H2 Evolution under Visible Light. Int. J. Hydrogen Energy 45 (28), 14334–14346. doi:10.1016/j.ijhydene.2020.03.139
Yijin Wang, Y., Huang, W., Guo, S., Xin, X., Zhang, Y., Guo, P., et al. (2021). Sulfur‐Deficient ZnIn 2 S 4 /Oxygen‐Deficient WO 3 Hybrids with Carbon Layer Bridges as a Novel Photothermal/Photocatalytic Integrated System for Z‐Scheme Overall Water Splitting. Adv. Energy Mat. 11, 2102452. doi:10.1002/aenm.202102452
Yu Liu, Y., Li, Z., Xie, Y., Tao, Y., Wu, J., Wang, S., et al. (2022). Surface Domain Potential Difference-Mediated Efficient Charge Separation on a Defective ZnIn2S4 Microsphere Photocatalyst. Mater. Today Chem. 23, 100714. doi:10.1016/j.mtchem.2021.100714
Yu, M., Lv, X., Mahmoud Idris, A., Li, S., Lin, J., Lin, H., et al. (2022). Upconversion Nanoparticles Coupled with Hierarchical ZnIn2S4 Nanorods as a Near-Infrared Responsive Photocatalyst for Photocatalytic CO2 Reduction. J. Colloid Interface Sci. 612, 782–791. doi:10.1016/j.jcis.2021.12.197
Zhang, S., Wang, L., Liu, C., Luo, J., Crittenden, J., Liu, X., et al. (2017). Photocatalytic Wastewater Purification with Simultaneous Hydrogen Production Using MoS 2 QD-Decorated Hierarchical Assembly of ZnIn 2 S 4 on Reduced Graphene Oxide Photocatalyst. Water Res. 121, 11–19. doi:10.1016/j.watres.2017.05.013
Zhang, G., Chen, D., Li, N., Xu, Q., Li, H., He, J., et al. (2020). Construction of Hierarchical Hollow Co 9 S 8 /ZnIn 2 S 4 Tubular Heterostructures for Highly Efficient Solar Energy Conversion and Environmental Remediation. Angew. Chem. 132, 8332–8338. doi:10.1002/ange.202000503
Zhao, C., Zhang, Y., Jiang, H., Chen, J., Liu, Y., Liang, Q., et al. (2019). Combined Effects of Octahedron NH2-UiO-66 and Flowerlike ZnIn2S4 Microspheres for Photocatalytic Dye Degradation and Hydrogen Evolution under Visible Light. J. Phys. Chem. C 123, 18037–18049. doi:10.1021/acs.jpcc.9b03807
Zhao, S., Liang, Q., Gao, W., Zhou, M., Yao, C., Xu, S., et al. (2021). In Situ Growth of ZnIn2S4 on MOF-Derived Ni-Fe LDH to Construct Ternary-Shelled Nanotubes for Efficient Photocatalytic Hydrogen Evolution. Inorg. Chem. 60, 9762–9772. doi:10.1021/acs.inorgchem.1c01064
Zhao Zhang, Z., Lu, L., Lv, Z., Chen, Y., Jin, H., Hou, S., et al. (2018). Porous Carbon Nitride with Defect Mediated Interfacial Oxidation for Improving Visible Light Photocatalytic Hydrogen Evolution. Appl. Catal. B Environ. 232, 384–390. doi:10.1016/j.apcatb.2018.03.086
Zhenfei Yang, Z., Xia, X., Yang, W., wang, L., and Liu, Y. (2021). Photothermal Effect and Continuous Hot Electrons Injection Synergistically Induced Enhanced Molecular Oxygen Activation for Efficient Selective Oxidation of Benzyl Alcohol over Plasmonic W18O49/ZnIn2S4 Photocatalyst. Appl. Catal. B Environ. 299, 120675. doi:10.1016/j.apcatb.2021.120675
Zhitong Wang, Z., Qi, R., Liu, D., Zhao, X., Huang, L., Chen, S., et al. (2021). Exfoliated Ultrathin ZnIn 2 S 4 Nanosheets with Abundant Zinc Vacancies for Enhanced CO 2 Electroreduction to Formate. ChemSusChem 14, 852–859. doi:10.1002/cssc.202002785
Zhou, D., Xue, X., Wang, X., Luan, Q., Li, A., Zhang, L., et al. (2022). Ni, in Co-doped ZnIn2S4 for Efficient Hydrogen Evolution: Modulating Charge Flow and Balancing H Adsorption/desorption. Appl. Catal. B Environ. 310, 121337. doi:10.1016/j.apcatb.2022.121337
Zhu, Y., Wang, L., Liu, Y., Shao, L., and Xia, X. (2019). In-situ Hydrogenation Engineering of ZnIn2S4 for Promoted Visible-Light Water Splitting. Appl. Catal. B Environ. 241, 483–490. doi:10.1016/j.apcatb.2018.09.062
Zizhong Zhang, Z., Huang, L., Zhang, J., Wang, F., Xie, Y., Shang, X., et al. (2018). In Situ constructing Interfacial Contact MoS2/ZnIn2S4 Heterostructure for Enhancing Solar Photocatalytic Hydrogen Evolution. Appl. Catal. B Environ. 233, 112–119. doi:10.1016/j.apcatb.2018.04.006
Zou, J.-P., Chen, Y., Liu, S.-S., Xing, Q.-J., Dong, W.-H., Luo, X.-B., et al. (2019). Electrochemical Oxidation and Advanced Oxidation Processes Using a 3D Hexagonal Co3O4 Array Anode for 4-nitrophenol Decomposition Coupled with Simultaneous CO2 Conversion to Liquid Fuels via a Flower-like CuO Cathode. Water Res. 150, 330–339. doi:10.1016/j.watres.2018.11.077
Keywords: ZnIn2S4, morphology and structural engineering, defects engineering, doping engineering, heterojunction engineering
Citation: Tang M, Yin W, Zhang F, Liu X and Wang L (2022) The Potential Strategies of ZnIn2S4-Based Photocatalysts for the Enhanced Hydrogen Evolution Reaction. Front. Chem. 10:959414. doi: 10.3389/fchem.2022.959414
Received: 10 June 2022; Accepted: 14 June 2022;
Published: 12 July 2022.
Edited by:
Yue Li, Henan Institute of Engineering, ChinaCopyright © 2022 Tang, Yin, Zhang, Liu and Wang. This is an open-access article distributed under the terms of the Creative Commons Attribution License (CC BY). The use, distribution or reproduction in other forums is permitted, provided the original author(s) and the copyright owner(s) are credited and that the original publication in this journal is cited, in accordance with accepted academic practice. No use, distribution or reproduction is permitted which does not comply with these terms.
*Correspondence: Xia Liu, bGl1eDkxOEAxNjMuY29t; Longlu Wang, d2FuZ2xvbmdsdUBuanVwdC5lZHUuY24=