High-pressure synthesis and magnetic properties of tetragonal R2BaCuO5 (R = Sm and Eu)
- 1Chemistry and Physics of Materials Unit, Jawaharlal Nehru Centre for Advanced Scientific Research, School of Advanced Materials, Bangalore, India
- 2Deutsches Elektronen-Synchrotron (DESY), Hamburg, Germany
We report the experimental discovery of a new structural phase of well-known orthorhombic R2BaCuO5 (R = Sm and Eu), exhibiting a tetragonal crystal structure with space group
1 Introduction
The oxides of the general formula R2BaMO5 (R = rare-earth, M = 3d-transition metal) form a large family of compounds that crystallize into four different structural types with intriguing magnetic properties (Michel and Raveau, 1982; 1983 St. Schiffler and Müller-Buschaum, 1986; St. Schiffler and Müller-Buschbaum, 1986; 1987a; 1987b; Mevs and Müller-Buschbaum, 1989; Mevs and Müller-Buschbaum, 1989; Müller-Buschbaum and Sonne, 1990; Amador et al., 1990; Salinas-Sánchez, Sáez-Puche, and Alario-Franco, 1990; Salinas-Sánchez et al., 1991; Sáez-Puche et al., 1992; Salinas-Sánchez et al., 1992; García-Matres, Martínez, et al., 1993; De Andrés et al., 1993; Sáez-Puche and Hernández-Velasco, 1994; Hernández-Velasco, Salinas-Sánchez, and Sáez-Puche, 1994). Amongst these, the best known is the green phase R2BaCuO5 (R = Sm-Gd, Dy-Lu, Y) compounds exhibiting the orthorhombic structure with space group
The R2BaCuO5 oxides have two different structures depending on the lanthanide trivalent cation size. For lanthanide ions Sm-Gd, Dy-Lu and Y, the structure is orthorhombic with space group Pnma, called the “green phase”. Whereas a tetragonal structure of cuprates, the so-called “brown phase” is observed for R = La, Pr, and Nd, space group P4/mbm. Table 1 shows a comparison of structural types, magnetic properties, and the ordering temperatures among R2BaCuO5 compounds. It can be noted that previous studies reveal some of the green phase compounds exhibited strong magnetoelectric coupling, mainly due to the interplay of 4f-3d magnetic interactions (Indra et al., 2019; Yanda, Ter-Oganessian, and Sundaresan, 2019; Yanda et al., 2020; 2021). For example, Sm2BaCuO5 demonstrates a linear magnetoelectric effect below the Cu2+ spins ordering temperature, and this coupling is further affected by the Sm3+ spins ordering (Indra et al., 2019; Yanda, Ter-Oganessian, and Sundaresan, 2019). Also, Gd2BaCuO5 is shown to exhibit multiferroic properties (Yanda et al., 2020). Further, Dy2BaCuO5 and Ho2BaCuO5 compounds evidence the linear magnetoelectric effect and field-induced multiferroicity, while Er2BaCuO5 exhibits field-induced electric polarization around 5.1 K above the critical field Hc ∼ 0.9 T, indicating coupling between magnetism and electric polarization (Indra et al., 2019; Yanda et al., 2021). The remaining compounds in the green phase series with R = Eu, Y, Tm, and Lu, however, do not show magnetoelectric coupling (Yanda, 2021).
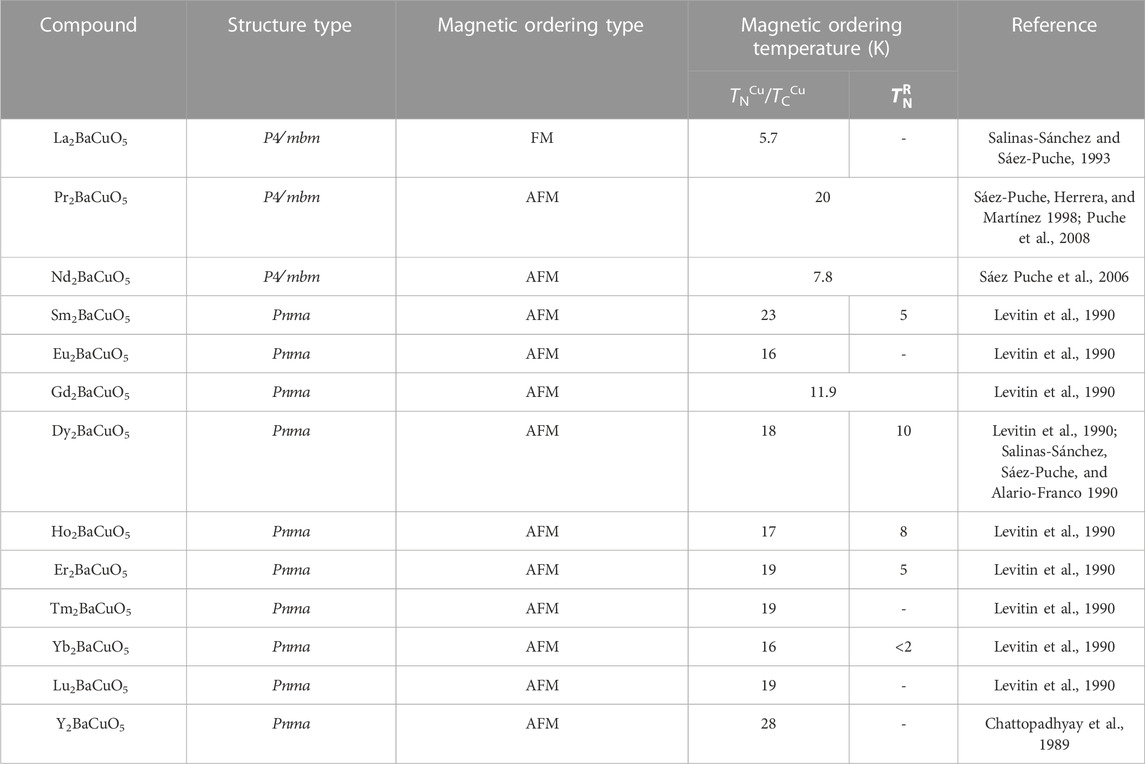
TABLE 1. Comparison of magnetic properties among R2BaCuO5 (R = rare-earth cations) compounds. FM and AFM mean ferromagnetic and antiferromagnetic orderings, respectively.
Recently, three new members of the R2BaZnO5 for R = Pr, Sm, and Eu were synthesized by a high-pressure and high-temperature method indicating polymorphism for Sm- and Eu- crystallizing in both
2 Experimental section
Polycrystalline samples of orthorhombic R2BaCuO5 (R = Sm and Eu) were prepared as starting materials by the conventional solid-state synthesis procedure starting from the stoichiometric mixture of high-purity R2O3, BaCO3, and CuO. The reactants were ground, followed by thermal treatments at 1,000°C in the air for 12 h with some intermediate grindings to homogenize the reaction products. Two different high-pressure machines were used to prepare and study the tetragonal phases. In the first step, the samples were treated with a pressure of 4.5 GPa and a temperature of 1,000°C in a laboratory-based cubic multi-anvil high-pressure apparatus. This transformed the R2BaCuO5 (R = Sm and Eu) to the tetragonal phase, which was characterized by powder x-ray diffraction at the powder diffraction and total scattering beamline P02.1 (PETRA III at DESY, Hamburg, Germany), with the wavelength of 0.20735 Å (Dippel et al., 2015). The sample detector distance (SDD) for XRD experiments was set to ∼1,000 mm. In the second step, in situ energy-dispersive x-ray diffraction spectra (EDXRD) at different pressure and temperature conditions were collected for orthorhombic Sm2BaCuO5. This experiment was performed in a Hall-type six-ram LVP (mavo press LPQ6 1,500–100; Max Voggenreiter GmbH, Germany) installed at the P61B beamline at DESY, Hamburg (Farla et al., 2022) using DESY standard 14/7 high-pressure assembly. Cr2O3-doped MgO octahedron was used as a pressure transmitting medium along with a tubular-resistive graphite heater. The pressure exerted at different press loads on the sample was calculated from in situ EDXRD data (using a MgO pressure marker) and temperature values were deduced from the Power-Temperature relationship (at the corresponding press load). The recovered sample was then characterized by a PANalytical Empyrean alpha-1 diffractometer using monochromatized Cu Kα1 radiation (λ = 1.5406 Å). The XRD pattern of all the samples was qualitatively analyzed by the Rietveld method using the program FULLPROF suite (Rodríguez-Carvajal, 1993). EDXRD profiles were analyzed using the PDIndexer software package provided by Seto Y (Seto et al., 2010; Seto, 2012). DC magnetization measurements were performed using a superconducting quantum interference device (SQUID) magnetometer (MPMS, Quantum Design). Specific heat capacity (
3 Results and discussion
3.1 Crystal structure
Analysis of the room temperature XRD pattern of ambient pressure R2BaCuO5 (R = Sm and Eu) precursor, prepared by conventional solid-state synthesis, confirms an orthorhombic phase (space group
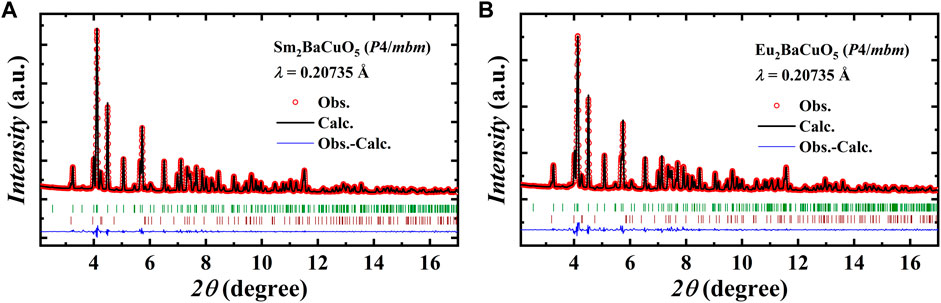
FIGURE 1. Rietveld refined synchrotron XRD pattern collected at RT of (A). Sm2BaCuO5 and (B). Eu2BaCuO5 in tetragonal phase, respectively. The second row of vertical tick marks indicates the secondary phase R2CuO4 (R = Sm and Eu).
Derived atomic positions and other structural parameters of both Sm2BaCuO5 and Eu2BaCuO5 in the tetragonal
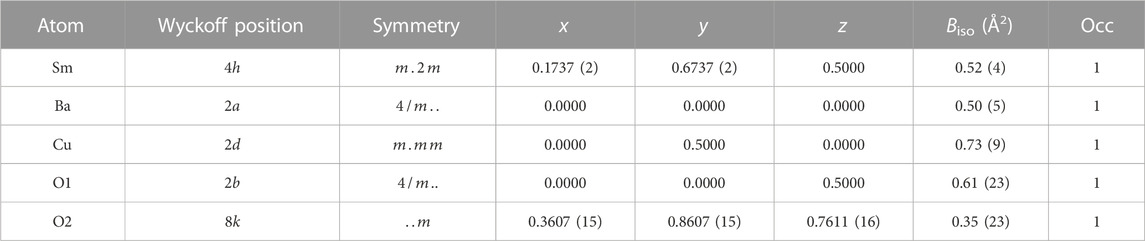
TABLE 2. Structural parameters obtained from Rietveld refinement of room temperature powder synchrotron XRD pattern of Sm2BaCuO5 in space group
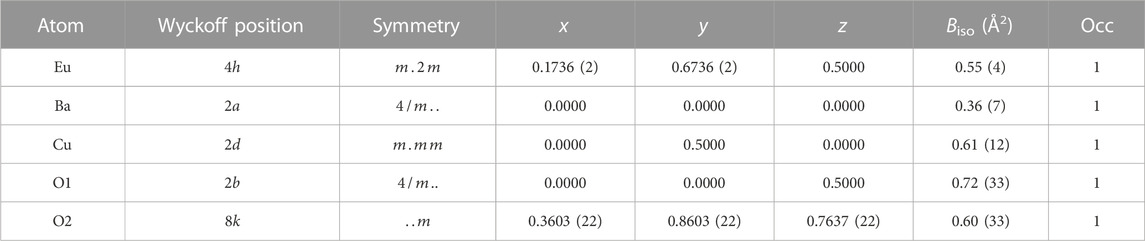
TABLE 3. Structural parameters obtained from Rietveld analysis of room temperature powder synchrotron XRD pattern of Eu2BaCuO5 in space group
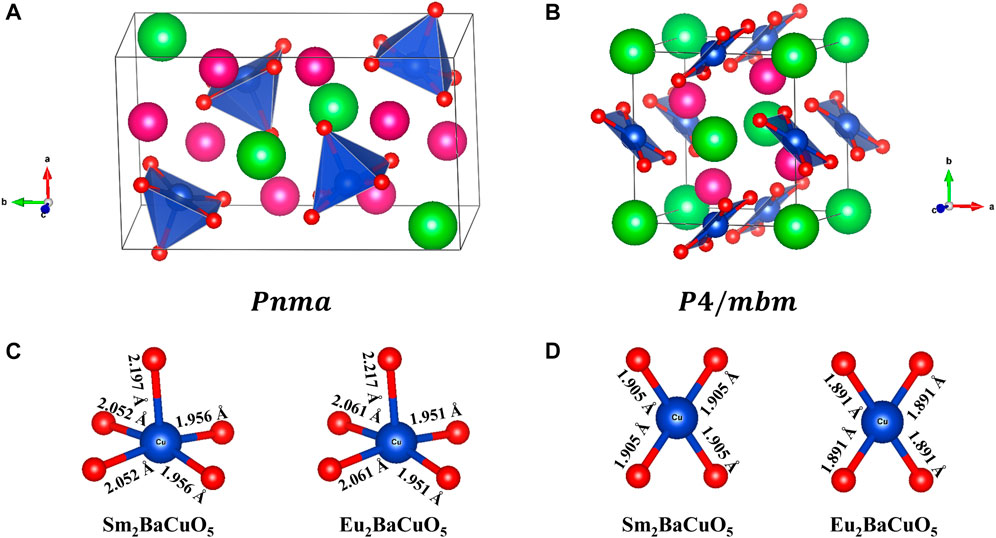
FIGURE 2. (A, B). Refined crystal structure of R2BaCuO5 (R = Sm and Eu) and (C, D). refined bond lengths in orthorhombic phase and tetragonal phase, respectively. (Pink: Rare-earth; Green: Barium; Blue: Copper; Red: Oxygen).
Further, the bond lengths for the copper polyhedra in both forms are shown in Figures 2C, D. From this, it is clear that the square pyramid CuO5 shows strong distortion compared to square planar CuO4, which has zero distortion because of its regular shape. The distortion parameter obtained for CuO5 calculated with the formula
are 1.9 × 10−3 and 2.3 × 10−3, for Sm- and Eu- samples, respectively, where
To investigate this high-pressure structural evolution, Sm2BaCuO5 in the orthorhombic phase was probed using energy dispersive x-ray diffraction (EDXRD), using a synchrotron white beam when the sample was exposed to different P-T conditions. The diffracted x-rays are energy analyzed at a fixed angle of 3.0230° in a continuous energy range of 30–160 keV. The obtained EDXRD spectra of Sm2BaCuO5 under ambient conditions are shown in Figure 3A, and the spectrum is consistent with the orthorhombic phase, indexed by the PDIndexer software. The sample was then subjected to pressure, and the corresponding in situ XRD patterns are shown in Supplementary Figure S2A. Upon compression, we observed the broadening of all reflections, and we could only detect 3–4 major broad reflections above a pressure of 1 GPa. These can be related to pressure-induced effects (amorphization or disorder state) on the sample. This effect remains consistent throughout the pressure range of 1–10.9 GPa, along with the pressure effect (peak shift). The swift change of spectra above 1 GPa also suggests the possible reorganization or disordering happens at a very early stage of the compression. On heating, reflections are getting sharper (a sign of order/recrystallization) again. The corresponding in situ spectra obtained at 10.9 GPa, and various temperatures are shown in Supplementary Figure S2B. It shows that the tetragonal phase appears around 720°C at 10.9 GPa. This also suggests that the orthorhombic phase transforms into disordered/amorphous state upon compression, then directly converts to a tetragonal phase upon heating. The tetragonal phase obtained at 10.9 GPa and 823°C could be retained after the quenching and recovered after decompression. The corresponding spectra are shown in Figure 3B. The sample was then crushed and measured by the laboratory angle-resolved x-ray diffraction to perform a conventional Rietveld refinement. The obtained structural parameters are enlisted in Table 4, which agrees well with the phase obtained at 4.5 GPa and 1,000°C in our high-pressure apparatus. It can be noted that at 10.9 GPa, the transition is completed within a few minutes and well below 1,000°C. We speculate that the observed phase transformation of Sm2BaCuO5 from orthorhombic to a tetragonal structure may be possible just above 1 GPa and at temperatures above 1,000°C. It is also to be noted that the tetragonal phase is stable till 10.9 GPa, supported by in situ experiments.
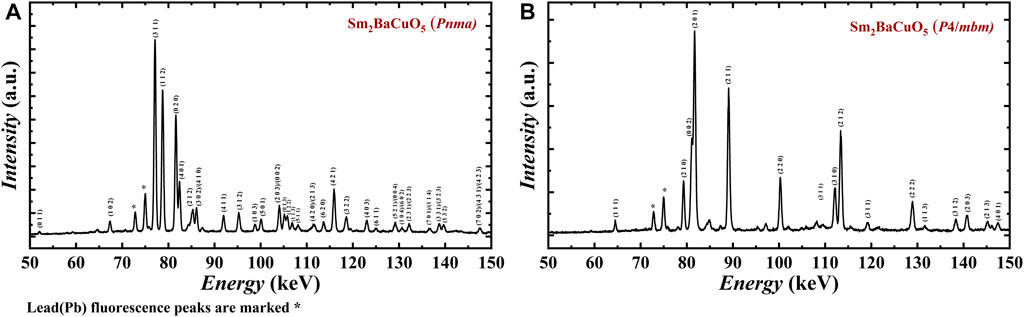
FIGURE 3. Energy-dispersive X-ray diffraction profiles of Sm2BaCuO5 analyzed at a fixed angle of 3.0230° at (A) ambient pressure and temperature, (B) recovered sample.
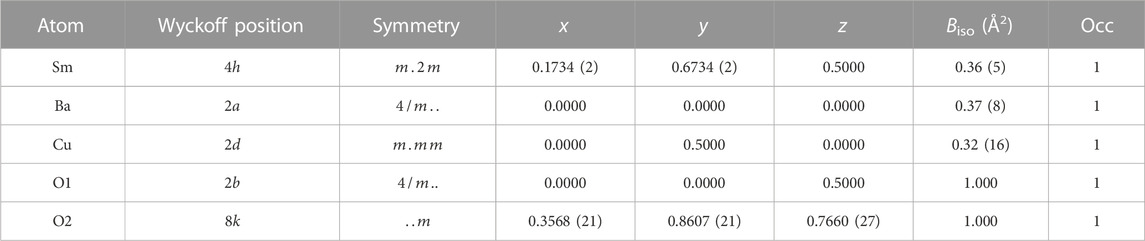
TABLE 4. Structural parameters obtained from Rietveld refinement of room temperature powder XRD pattern of Sm2BaCuO5 (10.9 GPa and 823
For physical property measurements, to avoid confusion for the readers, we have now named the tetragonal structured Sm2BaCuO5 and Eu2BaCuO5 synthesized at 4.5 GPa and 1,000°C in a cubic multi-anvil high-pressure apparatus installed at our lab as SBCO_HP1, EBCO_HP and the recovered Sm2BaCuO5 sample obtained at 10.9 GPa and 823°C as SBCO_HP2 in an LVP installed at the P61B beamline at DESY.
3.2 Magnetic properties
The temperature dependence of dc magnetic susceptibility
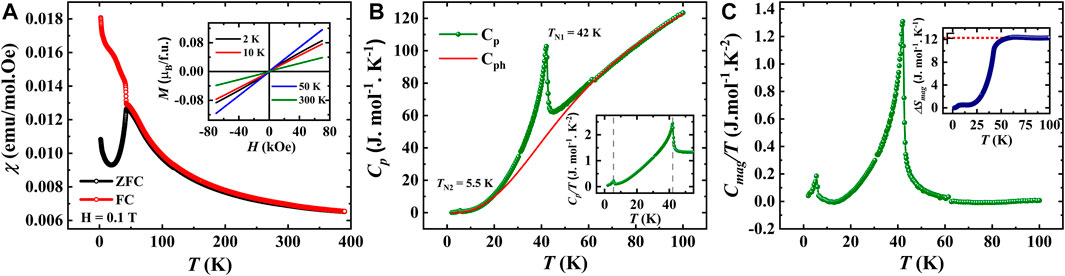
FIGURE 4. (A). DC magnetization as a function of temperature of SBCO_HP1 (Inset shows isothermal magnetization curves), (B). Temperature-dependent specific heat capacity measured in zero magnetic fields fitted with Debye-Einstein model (Inset shows Cp/T vs. T), (C). Magnetic contribution for specific heat as a function of temperature (Inset shows a change in magnetic entropy as a function of temperature).
Further, to investigate the antiferromagnetic transitions, heat capacity measurements have been carried out under zero applied magnetic field. The λ-type transitions at 42 and 5.5 K, as displayed in Figure 4B, confirm two long-range magnetic orderings. However, neutron diffraction studies are required to verify the Cu2+ and/or Sm3+ ion orderings. In order to assess the magnetic entropy changes, the phononic contribution to the specific heat has been estimated by fitting the heat capacity using the Debye-Einstein model, as shown in Figure 4B. The magnetic specific heat is obtained by subtracting Cph from Cp, and its temperature variation is shown in Figure 4C. The magnetic entropy is further obtained by integrating the magnetic specific heat Cmag/T, which yields the magnetic entropy changes 12.3 J mol-1K−1. The obtained entropy is 35% of the theoretically predicted value of
The temperature-dependent magnetic susceptibility
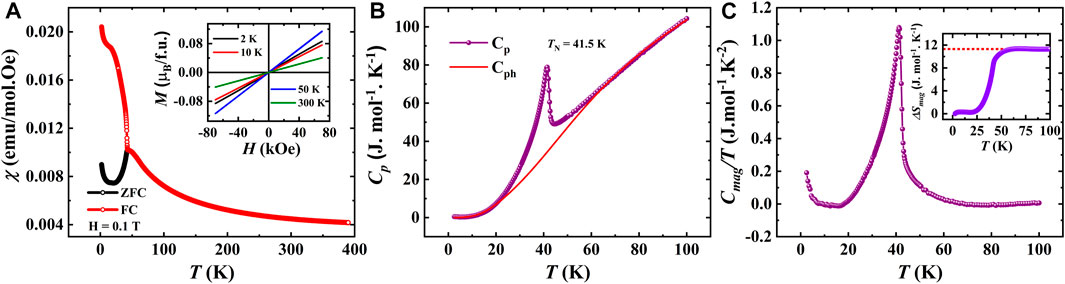
FIGURE 5. (A). Temperature dependent dc susceptibility measured under 0.1 T for SBCO_HP2 (Inset shows M(H) curves measured at different temperatures), (B). Temperature dependent specific heat measured under zero magnetic field and its fit with Debye-Einstein model, (C). Magnetic contribution for specific heat as a function of temperature (Inset shows a change in magnetic entropy as a function of temperature).
Figure 6A shows the temperature evolution of the dc magnetic susceptibility measured under a magnetic field of 0.1 T for EBCO_HP. The susceptibility increases with decreasing temperature without any signature of magnetic anomaly, indicating the paramagnetic behavior of this compound. This behavior is supported by the heat capacity data, which exhibits no anomalies in the temperature range of 2–100 K, as displayed in Figure 6B. Generally, for Eu3+-containing compounds, Eu3+ ions do not contribute to the magnetism and typically
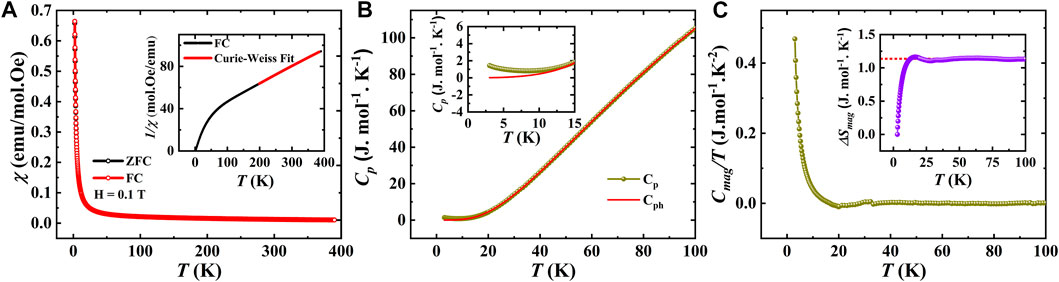
FIGURE 6. (A). Temperature dependence of the magnetization measured under 0.1 T for EBCO_HP (Inset shows Curie-Weiss fit of inverse susceptibility), (B). Temperature-dependent heat capacity measured at 0 T (Inset shows the zoomed view in the low-temperature region). (C). Magnetic specific heat against temperature (Inset shows magnetic entropy as a function of temperature).
To investigate further the magnetic properties of this material, magnetic field dependence studies were performed. Figure 7A shows the field dependence at various temperatures for positive and negative fields up to 7 T. Interestingly, the magnetization curve recorded at 2 K shows a nonlinear behavior with a maximum magnetization of 1.5
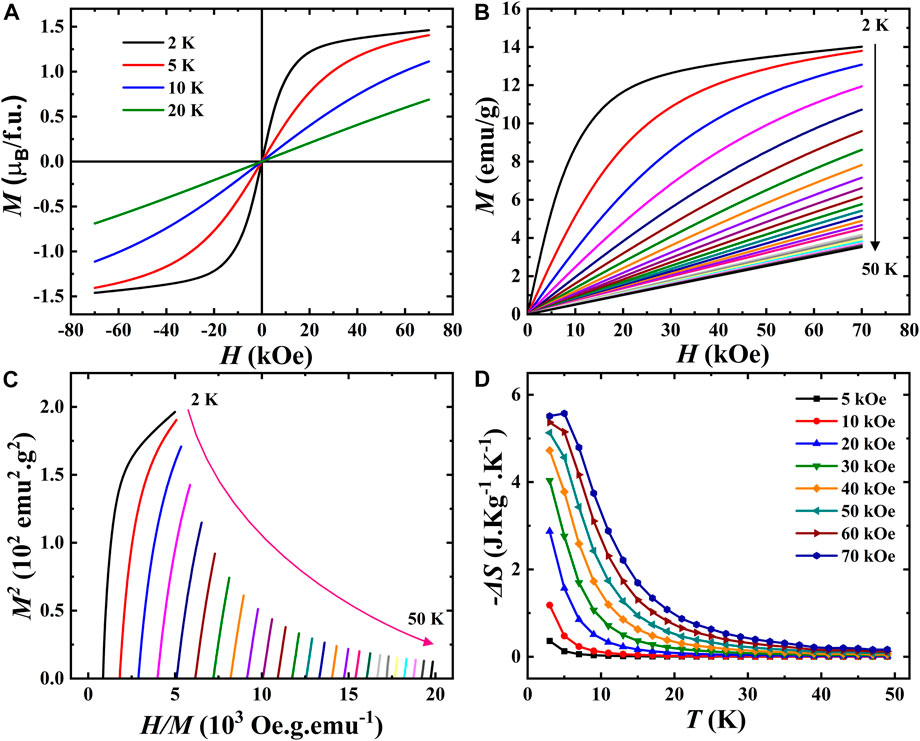
FIGURE 7. (A). Isothermal magnetization against the magnetic field recorded at different temperatures for EBCO_HP. (B). M(H) curves from 2–50 K measured from 0 to 7 T. (C). Arrott plots. (D). Temperature-dependent magnetic entropy change (
According to classical thermodynamic theory, the change in magnetic entropy (
The entropy variation as a function of temperature under different magnetic fields is represented in Figure 7D as a function of temperature. The magnetic entropy decreases with increasing temperature, similar to the magnetic susceptibility variation in Figure 7B. We have found that the maximum change in entropy corresponding to a magnetic field variation of 70 kOe at 3 K is about 5.6 J kg-1K−1. Overall, these results indicate Eu2BaCuO5 in the tetragonal phase is a good candidate for magnetocaloric materials. Therefore, the different magnetic behaviors of R2BaCuO5 (R = rare-earth cations) can be explained based on the Cu-O-R-O-Cu interaction pathway, which apparently can control magnetic interactions.
4 Conclusion
We have successfully synthesized the HP-HT polymorph of the orthorhombic R2BaCuO5 (R = Sm and Eu) and studied their structural and magnetic properties. In summary, we can say that “A new form is born” under HP-HT. It is clear from the above investigations that R2BaCuO5 (R = Sm and Eu) exhibits polymorphism under pressure. These materials undergo an irreversible structural phase transition from orthorhombic (SG:
Data availability statement
The original contributions presented in the study are included in the article/Supplementary Material, further inquiries can be directed to the corresponding author.
Author contributions
SM: conceptualization, synthesis, data curation, software visualization, formal analysis, writing-original draft. PY: conceptualization. SB: synthesis, data curation. ME: data curation. AS: project administration, supervision, conceptualization, reviewing, writing-reviewing and editing, funding acquisition. All authors contributed to the article and approved the submitted version.
Acknowledgments
Authors express their thanks to the Sheikh Saqr Laboratory (SSL) and International Centre for Materials Science (ICMS) at Jawaharlal Nehru Centre for Advanced Scientific Research (JNCASR), Bangalore, for various measurement facilities. SM would like to acknowledge Ms. Pavitra Shanbhag for discussing in situ experiments. SM acknowledges JNCASR for providing a research fellowship (JNC/S0692). AS would like to acknowledge the financial support from the Science and Engineering Board (SERB sanction No. CRG/2018/000520), Department of Science and Technology (DST), Government of India. The authors acknowledge DESY (Hamburg, Germany), a member of the Helmholtz Association HGF, for the provision of experimental facilities. Parts of this research were carried out at the large volume press (LVP) beamline P61B, powder diffraction and total scattering beamline P02.1 at PETRA III, DESY.
Conflict of interest
The authors declare that the research was conducted in the absence of any commercial or financial relationships that could be construed as a potential conflict of interest.
Publisher’s note
All claims expressed in this article are solely those of the authors and do not necessarily represent those of their affiliated organizations, or those of the publisher, the editors and the reviewers. Any product that may be evaluated in this article, or claim that may be made by its manufacturer, is not guaranteed or endorsed by the publisher.
Supplementary material
The Supplementary Material for this article can be found online at: https://www.frontiersin.org/articles/10.3389/fchem.2023.1166475/full#supplementary-material
References
Amador, J., Gutiérrez-Puebla, E., Monge, M. A., Rasines, I., Ruz-Valero, C., Fernandez, F., et al. (1990). Evidence of low-dimensional antiferromagnetic ordering and crystal structure in the R2BaNiO5 (R=Y,Er) oxides. Phys. Rev. B 42 (13), 7918–7924. doi:10.1103/PhysRevB.42.7918
Andrés, A. De, S. Taboada, Martínez, J. L. A. Salinas, J. Hernández, and Sáez-Puche, R. (1993). Optical phonons in R2BaMO5 oxides with M=Co, Ni, Cu, and R=a rare earth. Phys. Rev. B 47 (22), 14898–14904. doi:10.1103/PhysRevB.47.14898
Banerjee, B. K. (1964). On a generalised approach to first and second order magnetic transitions. Phys. Lett. 12 (1), 16–17. doi:10.1016/0031-9163(64)91158-8
Chattopadhyay, T., J BrownKöbler, P. U., and Wilhelm, M. (1989). Evidence for the antiferromagnetic ordering of the green phase Y2BaCuO5. Europhys. Lett. (EPL) 8 (7), 685–689. doi:10.1209/0295-5075/8/7/018
Dippel, Ann Christin, Liermann, Hanns Peter, Walter, Peter, Schulte-Schrepping, Horst, Seeck, Oliver H., and Franz, Hermann (2015). Beamline P02.1 at PETRA III for high-resolution and high-energy powder diffraction. J. Synchrotron Radiat. 22, 675–687. doi:10.1107/S1600577515002222
Farla, Robert, Bhat, Shrikant, Sonntag, Stefan, Chanyshev, Artem, Ma, Shuailing, Ishii, Takayuki, et al. (2022). Extreme conditions research using the large-volume press at the P61B endstation, PETRA III. J. Synchrotron Radiat. 29, 409–423. doi:10.1107/S1600577522001047
García-Matres, E., Martínez, J. L., Rodríguez-Carvajal, J., Alonso, J. A., Salinas-Sánchez, A., and Sáez-Puche, R. (1993a). Structural characterization and polymorphism of R2BaNiO5 (R = Nd, Gd, Dy, Y, Ho, Er, Tm, Yb) studied by neutron diffraction. J. Solid State Chem. 103 (2), 322–333. doi:10.1006/jssc.1993.1107
García-Matres, E., Rodríguez-Carvajal, J., Martinez, J. L., Alonso, J. A., Salinas-Sánchez, A., and Sáez-Puche, R. (1993b). Polymorphism in the oxides R2BaNiO5 (R=Tm, Yb, Lu). Solid State Ionics 63 (65), 915–921. doi:10.1016/0167-2738(93)90215-O
Hernández-Velasco, J., and Sáez-Puche, R. (1993). Dimorphism and magnetic properties of Dy2BaCoO5. J. Alloys Compd. 198 (1–2), 63–67. doi:10.1016/0925-8388(93)90146-E
Hernández-Velasco, J., Salinas-Sánchez, A., and Sáez-Puche, R. (1994). Antiferromagnetic ordering and structural characterization of the Brown colored R2BaCoO5 oxides (R = rare earth). J. Solid State Chem. 110 (2), 321–329. doi:10.1006/jssc.1994.1175
Indra, A., Mukherjee, S., Majumdar, S., Gutowski, O., Zimmermann, M. V., and Giri, S. (2019). High-temperature ferroelectric order and magnetoelectric coupling driven by the magnetic field cooling effect in R2BaCuO5 (R=Er,Dy,Sm). Phys. Rev. B 100 (1), 14413. doi:10.1103/PhysRevB.100.014413
Ishii, Yuto, Chen, Jie, Yoshida, Hiroyuki K., Oda, Migaku, Christianson, Andrew D., and Yamaura, Kazunari (2020). High-pressure synthesis, crystal structure, and magnetic properties of the shastry-sutherland-lattice oxides BaLn2ZnO5 (Ln = Pr, Sm, Eu). J. Solid State Chem. 289, 121489. doi:10.1016/j.jssc.2020.121489
Levitin, R. Z., Mill, B. V., Moshchalkov, V. V., Samarin, N. A., Snegirev, V. V., and Zoubkova, J. (1990). Two magnetic transitions and metamagnetism in the R2BaCuO5 (R = Sm, Eu, Gd, Dy, Ho, Er, Tm, Yb) compounds. J. Magnetism Magnetic Mater. 90 (91), 536–540. doi:10.1016/S0304-8853(10)80196-9
Mevs, H., and Müller-Buschbaum, H. K. (1989b). Neue oxometallate vom BaCuSm2O6-typ: BaCoHo2O5, BaCoYb2O5 und vom BaNiLn2O5-typ: BaCoEr2O5. Z. Für Anorg. Und Allg. Chem. 574 (1), 172–176. doi:10.1002/zaac.655740119
Mevs, H., and Müller-Buschbaum, H. K. (1989a). Ein wechsel des strukturtyps in den oxiden BaCoGd2O5, BaCoDy2O5 und BaCoY2O5. ZAAC - J. Inorg. General Chem. 573 (1), 128–132. doi:10.1002/zaac.19895730113
Michel, C., and Raveau, B. (1982). Les oxydes A2BaCuO5 (A = Y, Sm, Eu, Gd, Dy, Ho, Er, Yb). J. Solid State Chem. 43 (1), 73–80. doi:10.1016/0022-4596(82)90216-X
Michel, C., and Raveau, B. (1983). Ln2BaZnO5 and Ln2BaZn1-xCuxO5: A series of zinc oxides with zinc in a pyramidal coordination. J. Solid State Chem. 49 (2), 150–156. doi:10.1016/0022-4596(83)90108-1
Müller-Buschbaum, H. K., and Sonne, P. (1990). BaZnNd2O5: Eine neue struktur bei oxometallaten BaMLn2O5. J. Less-Common Metals 167 (1), 193–198. doi:10.1016/0022-5088(90)90305-4
Puche, R. Sáez, E., Fernández-Díaz, M. T., and Cascales, C. (2008). Magnetic characterization of Pr2BaCuO5. J. Phys. Condens. Matter 20 (4), 045210. doi:10.1088/0953-8984/20/04/045210
Rodríguez-Carvajal, J. (1993). Recent advances in magnetic structure determination by neutron powder diffraction. Phys. B Phys. Condens. Matter 192 (1–2), 55–69. doi:10.1016/0921-4526(93)90108-I
Sáez Puche, R., Climent, E., Jiménez-Melero, E., Romero De Paz, J., Martínez, J. L., and Fernández-Díaz, M. T. (2006). Neutron powder diffraction study on Nd2BaCuO5 oxide. J. Alloys Compd. 408 (412), 613–617. doi:10.1016/j.jallcom.2004.12.060
Sáez-Puche, R., Coronado, J. M., Martín-Llorente, J. M., and Rasines, I. (1992). Antiferromagnetism in the R2BaNiO5 oxides (R = Nd, Eu, Dy, Ho). Mater. Chem. Phys. 31 (1–2), 151–154. doi:10.1016/0254-0584(92)90168-8
Sáez-Puche, R., and Hernández-Velasco, J. (1994). Structural relationships and magnetic behaviour in R2BaMO5 oxides (R = rare earth; M = Co, Ni and Cu). Adv. Mater. Res. 1 (2), 65–82. doi:10.4028/www.scientific.net/amr.1-2.65
Sáez-Puche, R., Herrera, S. R., and Martínez, J. L. (1998). Synthesis and magnetic behaviour of the novel Pr2BaCuO5 oxide. J. Alloys Compd. 269 (1–2), 57–59. doi:10.1016/S0925-8388(98)00163-7
Salinas-Sánchez, A., García-Muñoz, J. L., Rodríguez-Carvajal, J., Sáez-Puche, R., and Martinez, J. L. (1992). Structural characterization of R2BaCuO5 (R = Y, Lu, Yb, Tm, Er, Ho, Dy, Gd, Eu and Sm) oxides by X-ray and neutron diffraction. J. Solid State Chem. 100 (2), 201–211. doi:10.1016/0022-4596(92)90094-C
Salinas-Sánchez, A., Sáez-Puche, R., and Alario-Franco, M. A. (1990). Evidence of antiferromagnetic order in the green phases R2BaCuO5 (R = Dy, Y, and Yb). J. Solid State Chem. 89 (2), 361–365. doi:10.1016/0022-4596(90)90277-5
Salinas-Sánchez, A., Sáez-Puche, R., Rodríguez-Carvajal, J., and Martínez, J. L. (1991). Structural characterization of R2BaNiO5 (R=Tm and Yb): Polymorphism for R=Tm. Solid State Commun. 78 (6), 481–488. doi:10.1016/0038-1098(91)90361-X
Salinas-Sánchez, A., and Sáez-Puche, R. (1993). Structural characterization and magnetic properties of LaRBaCuO5 oxides (R=rare earth). Solid State Ionics 63–65 (8), 927–931. doi:10.1016/0167-2738(93)90217-Q
Schiffler, St., and Müller-Buschaum, H. K. (1986a). Ein neuer strukturtyp zur formel ABLn2O5 zur kenntnis von BaNiNd2O5. Z. Für Anorg. Und Allg. Chem. 532 (1), 10–16. doi:10.1002/zaac.19865320103
Schiffler, St., and Müller-Buschbaum, H. K. (1987a). Neue verbindungen zum BaNiNd2O5-typ: BaNiLn2O5 (Ln=Sm, Gd, Ho, Er, Tm). Monatsh. Für Chem. Chem. Mon. 118 (6–7), 741–747. doi:10.1007/BF00809224
Schiffler, St., and Müller-Buschbaum, H. K. (1987b). Neue verbindungen zum BaPtNd2O5-typ: BaMLn2O5 (M = Pt, ln ≡ Sm, Gd; M = Pd, ln = Pr, Tb). J. Less Common Metals 128 (February), 117–123. doi:10.1016/0022-5088(87)90197-4
Schiffler, St., and Müller-Buschbaum, H. K. (1986b). BaNiYb2O5 – eine verbindung, die nicht im BaNiLn2O5-typ kristallisiert mit einem beitrag über BaCuSm2O5. Z. Für Anorg. Und Allg. Chem. 540 (9–10), 243–250. doi:10.1002/zaac.19865400927
Seto, Yusuke, Nishio-Hamane, Daisuke, Nagai, Takaya, and Sata, Nagayoshi (2010). Development of a software suite on X-ray diffraction experiments. Rev. High Press. Sci. Technology/Koatsuryoku No Kagaku Gijutsu 20 (3), 269–276. doi:10.4131/jshpreview.20.269
Seto, Yusuke (2012). Whole pattern fitting for two-dimensional diffraction patterns from polycrystalline materials. Rev. High Press. Sci. Technol. 22 (2), 144–152. doi:10.4131/jshpreview.22.144
Yanda, Premakumar (2021). Magnetoelectric Multiferroic Prop. Green Phases R2BaCuO5 Aeschynites RFeWO6 R= Rare Earth. http://lib.jncasr.ac.in:8080/jspui/handle/123456789/3229.
Yanda, Premakumar, Golosovsky, I. V., Mirebeau, I., Ter-Oganessian, N. V, Rodríguez-Carvajal, J., and Sundaresan, A. (2020). Interplay of 4f-3d interactions and spin-induced ferroelectricity in the green phase Gd2BaCuO5. Phys. Rev. Res. 2 (2), 1–7. doi:10.1103/PhysRevResearch.2.023271
Yanda, P., Orlandi, F., Manuel, P., Boudjada, N., Rodriguez-Carvajal, J., and Sundaresan, A. (2021). Magnetic-field-induced ferroelectric states in centrosymmetric R2BaCuO5 (R = Dy and Ho). Phys. Rev. B 104 (14), 144401. doi:10.1103/PhysRevB.104.144401
Keywords: high-pressure synthesis, polymorphism, antiferromagnetism, magnetocaloric effect, paramagnetism
Citation: Mishra S, Yanda P, Bhat S, Etter M and Sundaresan A (2023) High-pressure synthesis and magnetic properties of tetragonal R2BaCuO5 (R = Sm and Eu). Front. Chem. 11:1166475. doi: 10.3389/fchem.2023.1166475
Received: 15 February 2023; Accepted: 23 May 2023;
Published: 09 June 2023.
Edited by:
Yashonath Subramnian, Indian Institute of Science (IISc), IndiaReviewed by:
Md Motin Seikh, Visva-Bharati University, IndiaSabine Wurmehl, Leibniz Institute for Solid State and Materials Research Dresden (IFW Dresden), Germany
Copyright © 2023 Mishra, Yanda, Bhat, Etter and Sundaresan. This is an open-access article distributed under the terms of the Creative Commons Attribution License (CC BY). The use, distribution or reproduction in other forums is permitted, provided the original author(s) and the copyright owner(s) are credited and that the original publication in this journal is cited, in accordance with accepted academic practice. No use, distribution or reproduction is permitted which does not comply with these terms.
*Correspondence: A. Sundaresan, sundaresan@jncasr.ac.in