Phytates as a natural source for health promotion: A critical evaluation of clinical trials
- 1LAQV-REQUIMTE, Department of Chemistry, University of Aveiro, Aveiro, Portugal
- 2Phytotherapy Lab (PhT-Lab), Endocrinology Unit, Department of Medicine (DIMED), University of Padova, Padova, Italy
- 3AIROB, Associazione Italiana per la Ricerca Oncologica di Base, Padova, Italy
- 4Instituto de Ciencias Naturales, Facultad de Medicina Veterinaria y Agronomía, Universidad de Las Américas, Las Américas, Chile
- 5Educational Program, Geography, Environment and Service Sector, Abai Kazakh National Pedagogical University, Almaty, Kazakhstan
- 6Biomedical Research Centre, Al-Farabi Kazakh National University, Almaty, Kazakhstan
- 7Centre for Healthy Living, Department of Nutrition and Dietetics, Faculty of Pharmacy, University of Concepción, Concepción, Chile
- 8Unidad de Desarrollo Tecnológico, UDT, Universidad de Concepción, Concepción, Chile
- 9Department of Pharmacognosy, Faculty of Pharmacy, Sivas Cumhuriyet University, Sivas, Türkiye
- 10Aromatic Plant Research Center, Lehi, UT, United States
- 11Department of Chemistry, University of Alabama in Huntsville, Huntsville, AL, United States
- 12Department of Food Science, Faculty of Food Science and Technology, Universiti Putra Malaysia, Serdang, Selangor, Malaysia
- 13Natural Medicines and Products Research Laboratory, Institute of Bioscience, Universiti Putra Malaysia, Serdang, Selangor, Malaysia
- 14Department of Biochemistry, Faculty of Science, University of Maiduguri, Maiduguri, Borno, Nigeria
- 15Department of Clinical Pharmacy, University of Medicine and Pharmacy of Craiova, Craiova, Romania
- 16Facultad de Medicina, Universidad del Azuay, Cuenca, Ecuador
Phytates are a type of organophosphorus compound produced in terrestrial ecosystems by plants. In plant feeds, phytic acid and its salt form, phytate, account for 60%–80% of total phosphorus. Because phytate is a polyanionic molecule, it can chelate positively charged cations such as calcium, iron, and zinc. Due to its prevalence in vegetal tissues and the fact that people consume plants, phytate was first considered a potential health benefit. This updated review aims to summarize the current data on the results of clinical trials of phytates on human health, highlighting both beneficial and undesirable effects. To obtain these updated data, published papers in electronic databases such as PubMed/MedLine, TRIP database, Wiley, Google Scholar, Baidu, and Scopus were searched. Study results have shown that phytate can have beneficial health effects such as antioxidant, anticancer potential and reduction of pathological calcifications in blood vessels and organs; but also, negative effects by reducing the absorption of minerals important for maintaining the homeostasis of the human body. According to these recent results derived from recent clinical studies, phytates may be a potential natural source for health benefits. To improve clinical efficacy and human health benefits, further dose-response studies are needed to determine effective therapeutic doses and potential interactions with conventional drugs.
1 Introduction
Phytates [chemically known as myoinositol (1,2,3,4,5,6) hexakisphosphate] can be found in numerous plants and their parts, including seeds, nuts, legumes, and cereals (Habtamu Fekadu, 2014; Pujol et al., 2023). Phytates possess the trivial names of inositol hexakisphosphate (IP6, InsP6) or phytic acid, with the last one commonly used. Phytates in plants represent a source of energy and antioxidant capacity (as a phosphate groups donor), but they have a predominant role as storage mineral chelating agents (depot of Cu2+ and Zn2+ cations in particular) due to the presence of negative charges at physiological pH (Barrientos and Murthy, 1996; Persson et al., 1998; Raboy, 2003). In addition, this compound is present outside the plant kingdom and can be found in all organisms (excluding prokaryotes), where, together with its derivatives, it is involved in cell metabolism, homeostasis, and pathological conditions (Bohn et al., 2008; Maffucci and Falasca, 2020; Silva et al., 2021). Phytates were first discovered in 1855 by a German researcher Theodor Hartig who identified circular particles in different plant seeds (Hartig, 1855; Khoo and Wolf, 1970). The chemical structure of phytate was later defined in 1914, as myo-inositol-1,2,3,4,5,6-hexakis dihydrogen phosphate (Anderson, 1914). The resulting insoluble salts denominated as phytates consist of a ring with six carbon atoms esterified with a phosphate group (IP6). Phytases promote IP6 dephosphorylation into smaller inositol phosphates (IP1-IP5) also belonging to the phytates family. Given its abundance in vegetal tissues and the consumption of plants by humans, phytate was early investigated for a possible role in health (Schlemmer et al., 2009). Indeed, already in the 1940s phytate was suggested as an anti-nutrient, due to its capacity to couple with cations and reduce absorption (McCance and Widdowson, 1942; Mc and Walsham, 1948). Subsequently, it has been reported that a large number of phytates in an unbalanced diet could represent an issue, however in a normal diet with low phytate content, phytates can be considered safe and not implicated in nutrient deficiency (Lopez et al., 2002). More recently the interaction between phytates and human diet and health has been revised suggesting numerous potential beneficial effects (Schlemmer et al., 2009). For example, phytate has been implicated in preventing calcifications (Grases and Costa-Bauza, 1999; Fernandez-Palomeque et al., 2015), in diabetes (Omoruyi et al., 2013; Sanchis et al., 2018), in lipid profile (Koba et al., 2003; Yuangklang et al., 2005), in a healthy diet (Prieto et al., 2010), in cancer (Vucenik and Shamsuddin, 2006; Pallem et al., 2020; Markiewicz et al., 2021), and in dental medicine (Parkinson et al., 2018; Liu and Tu, 2021). Non-etheless, a few works reported the potential negative impact of phytate (Kim et al., 2020; Kim et al., 2021), while clinical trials experimenting with phytates in human diseases are poorly represented (Hanson et al., 2006; Hunt and Beiseigel, 2009; Prieto et al., 2010; Sanchis et al., 2018). This review highlights the pros and cons of phytates, with a special emphasis on the therapeutic health beneficial effects in humans.
2 Review methodology
Relevant information on the results of clinical trials of the beneficial effects of phytates on human health was collected by searching scientific databases such as PubMed/MedLine, TRIP database, Wiley, Google Scholar and Scopus using the next MeSH terms: “Humans,” “Kidney Failure,” “Chronic,” “Phytic Acid,” “Renal Dialysis,” “Vascular Calcification,” “Antioxidants/metabolism,” “Apoptosis/drug effects,” “Cell Proliferation/drug effects,” “Dietary Supplements,” “Humans,” “Phytic Acid/pharmacology,” “Calcinosis/drug therapy,” “Calcinosis/pathology,” “Calcium, Crystallization,” “Phytic Acid/therapeutic use.” There were evaluated 70 articles from international publications that included pharmacological studies with identified mechanisms of action and clinical studies of phytates regarding the effects in various chronic diseases. Of the studies reviewed, only papers written in English were selected. Abstracts, short communications, experimental pharmacological studies that did not include mechanisms of action, or that used homeopathic medicines were excluded. We also explored https://clinicaltrials.gov/website to identify clinical trials with phytates. The most relevant data have been summarized in tables and illustrative figures. The chemical formulas have been validated according to PubChem (PubChem, 2022).
3 Bioavailability and pharmacokinetics data
For several decades, phytic acid was considered an anti-nutrient due to its ability to form insoluble salts with cations such as zinc, magnesium, iron, and copper, which could cause a decrease in the bioavailability of these essential elements. In the last 20 years, several studies have shown that this effect is more evident in cases of a diet very rich in phytates, combined with a deficiency in trace elements (Vucenik and Shamsuddin, 2003; Silva and Bracarense, 2016). However, as the bioactive properties of phytate have been elucidated over the years, namely, its antioxidant and antitumor capabilities, the understanding of its digestion and absorption processes, as well as their interactions with the components of the human body, has sparked a lot of interest. Phytate is usually found in high quantities in almonds (0.35%–9.42%) (Harland and Oberleas, 1986), walnuts (0.2%–6.69%) (Chen, 2004), wheat bran (2.1%–7.3%) (Harland and Oberleas, 1986), maize (6.39%) (Kortekangas et al., 2020), rice bran (2.56%–8.7%) (Kortekangas et al., 2020), sorghum 0.57%–3.355% (Kortekangas et al., 2020), kidney beans (0.61%–2.38%) (Lehrfeld, 1994), soybeans (1%–2.22%) (Gupta et al., 2015); and in small quantities in other vegetables and seeds: millet (0.18%–1.67%) (Ravindran et al., 1994), chickpeas (0.28%–1.60%) (Ravindran et al., 1994), lentils (0.27%–1.51%), rye (0.54%–1.46%) (Gupta et al., 2015), barley 0.38%–1.16% (Kortekangas et al., 2020); oat 0.42%–1.16% (Harland and Oberleas, 1986); (Figure 1), albeit it can be destroyed during food processing like soaking and malting.
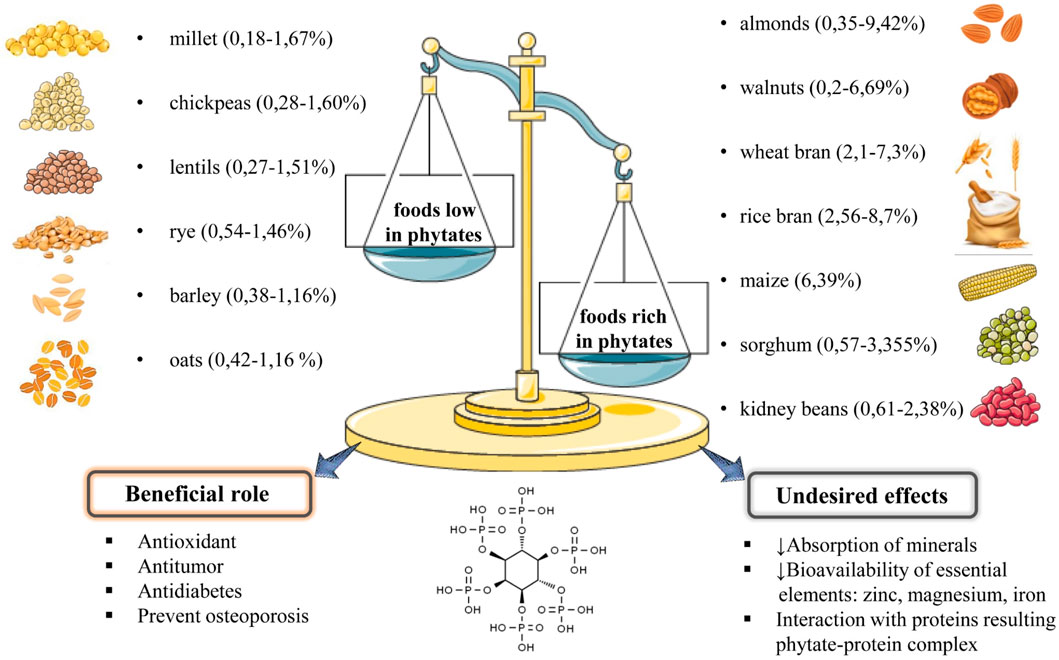
FIGURE 1. Summarized diagram regarding the main sources of phytates, their quantities in foods, beneficial role and undesired effects.
This is usually hydrolyzed to inositol, inositol phosphate derivatives, and orthophosphate (Figure 2) by phytases found in microorganisms or plants. Plant intrinsic phytases, on the other hand, can only be triggered under specific conditions, such as cooking, fermentation, and immersion (Schlemmer et al., 2009). Thus, in general, when an ingested food is high in phytates, this is hydrolyzed mostly in the intestines by microbial phytases (Weremko et al., 1997). Complexed cations and proteins are also released when phytases cleave the phosphate groups from the phytate, enhancing their bioaccessibility.
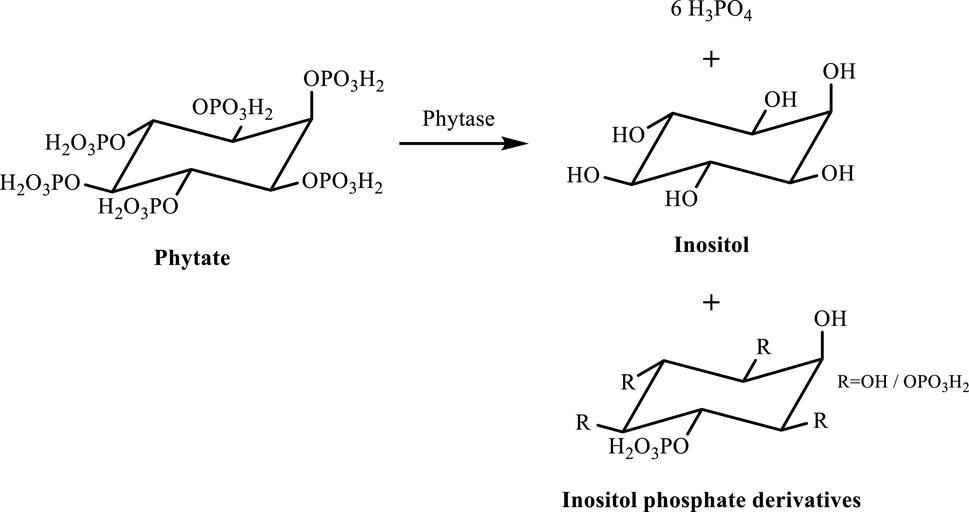
FIGURE 2. Phytate hydrolysis in inositol, inositol phosphate derivatives, and orthophosphate (Lei and Porres, 2003).
In the past, being negatively charged at physiological pH, phytate and its main derivatives were assumed not to permeate the lipid bilayer of plasma membranes, and therefore, their absorption was highly questionable. This paradigm, however, has changed over the years (Nahapetian and Young, 1980), as subsequent studies have suggested the absorption of its derivatives, mainly in the intestines. Among them, Nahapetian and Young (1980) used radioactively labelled 14C of myo-inositol and discovered that this was detected in rats’ urine, blood, and internal organs. Accordingly, Grases et al. (2001) demonstrated that a normal diet rich in phytates, or the adding it supplements to a normal human diet, resulted in increased inositol hexaphosphate (IP6) levels in plasma and excreted in urine. Moreover, in a clinical trial, Grases et al. (2006a) also reported that, independently of stomach conditions, 8 h after intake of 400 mg of phytates followed by a low-phytate diet resulted in an accumulation of more than 400 µg of IP6 in the urine excretion. Note that, according to Dinicola et al. (2017), these compounds are rapidly digested and distributed throughout the body following oral consumption, with their derivatives reaching their peak plasma levels within 4 h. Still, regardless of these data, it is unclear whether phytate derivatives absorption is mediated by pinocytosis, active transporters, or other processes. So far, in a study aimed at investigating the anti-cancer mechanism of IP6, the authors reported that IP6 entered HeLa cells via pinocytosis (Ferry et al., 2002). It is also known that phytates can interact with proteins due to the affinity of phosphate groups for cationic amino acids. This interaction can be harmful, disrupting protein digestion, but it can also protect the human body from harmful effects of specific proteins, such as oxidases and pathogenic proteins and several diseases like diabetes. Lee et al. (2006) found that diabetic KK mice fed with a diet supplemented with 0.5% and 1.0% sodium phytate for 8 weeks ad libitum, induced a decrease in blood glucose levels. Because phytate may bind to some amino acids and inhibit digestive enzymes, the low glycemic response could be related to the delayed digestion of starch (Lee et al., 2006). Affinity competition, pH, pI, ionic strength, and amino acid availability are all variables that can alter the stability of these complexes. These can be manipulated to prevent phytate-protein complex formation (Wang and Guo, 2021).
4 Phytate in clinical trials
Phytate is a natural plant-derived compound, however artificial forms of this compound are also marketed. The effect of natural (plant-derived) and artificial forms has been studied on different diseases, for instance, breast cancer, T2DM, cardiovascular calcification, and BMD (Tables 1, 2; Figure 3). For reviewing clinical trials about phytate, studies published in English found in the ClinicalTrials® and PubMed® databases in the last 5 years have been collected. In a double-blind, randomized, clinical trial, Proietti et al. (2017) investigated the effect of phytate on 20 adult breast cancer patients. The patients were separated into two groups. The treatment group was administered phytate (200 mg) per day and the control group received a gel containing hyaluronic acid (5 g) per day for 6 months. At the end of the 6 months, questionnaires belonging to the “European organization for testing the treatment of cancer (EORTC)” consisting of several questions under different parameters were requested from the patients. Patients’ assessments of the questionnaires showed that phytate administration not only increased the life quality and ability to perform daily life activities but also decreased adverse effects caused by chemotherapy in the treatment group compared to the control group. The level of glycation end-products (AGEs) and glycated hemoglobin (HbA1c) are higher in T2DM patients and the approach for the attenuation in the level of these proteins or ligands could be promising in the management of this disease (Quispe et al., 2022). A cross-over, randomized, clinical trial conducted by Sanchis et al. (2018) reported that a phytate-based diet could be used for this purpose. In the study, 35 adult T2DM patients were divided into phytate-based diet groups and non-phytate-based diet groups. The phytate-based diet group was administered 380 mg of phytate, three times per day for 12 weeks. After that, 12 weeks wash-out period was given to each group. At the end of the wash-out period, groups were switched to the alternate diet for 12 weeks. Observations on the patients were continued for another 12 weeks. The outcome of the study showed that the levels of AGEs and HbA1c were diminished after phytate-based diet intervention compared to the beginning. In another double-blind, randomized, crossover, clinical study, Ikenaga et al. (2020) showed the effect of phytate on serum uric acid (SUA) levels in healthy volunteers. 48 healthy volunteers were chosen and divided into a phytic acid drink (which contains 600 mg of phytic acid) ingested and a control drink (in case, mineral water) ingested groups. Results indicated that the level of SUA was decreased in the phytic acid drink ingested group compared to the control group. The outcome of the study showed that phytic acid has the potential to improve life quality by regulating the SUA level.
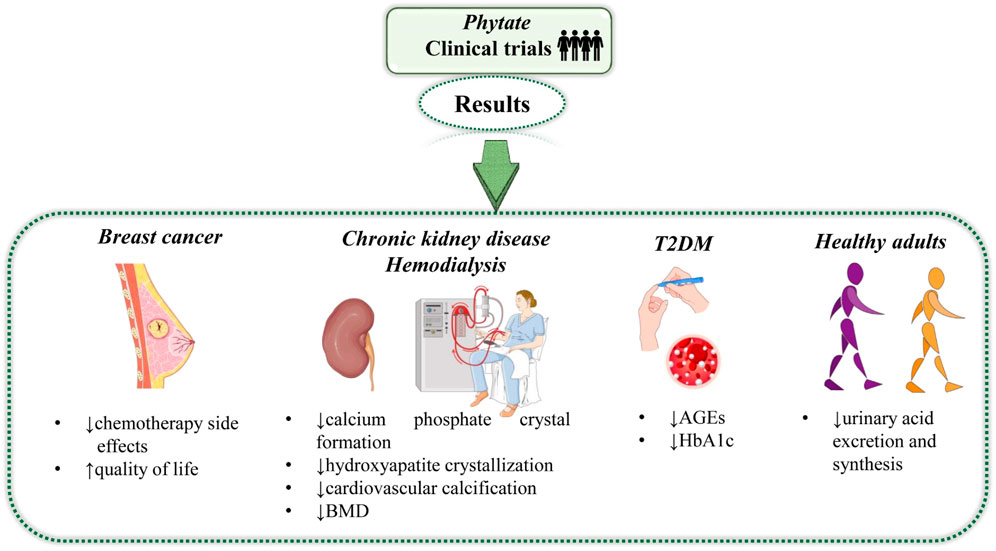
FIGURE 3. Summarized diagram with the most relevant results of natural and artificial forms of phytate. Abbreviations and symbols: ↓decrease, ↑increase, AGEs, glycation end-products and HbA1c, glycated hemoglobin.
Mainly two different artificial forms of phytate SNF472 and Fe-Pa-HCP have been extensively studied on cardiovascular calcification levels of hemodialysis patients or patients with renal disease at different stages. A randomized, double-blind, placebo-controlled clinical study by Perelló et al. (2018) investigated the effect of SNF72 on cardiovascular calcification. In the study, the effect of SNF472 was initially analyzed on 16 healthy volunteers with different doses (0.5, 5, 9, 12 mg/kg) for 4-h infusions. Pharmacokinetics (PK) analysis showed that in maximum SNF472 concentration measured in plasma (Cmax) and area under the curve (AUC) parameters increased in a dose-dependent manner but not significantly. Additionally, treatment-emergent adverse events (TEAEs) were observed during the treatment period. At the end of PK and TEAE analyses, the effective dose was determined at the Dose Escalation Safety Committee (DESC) meeting as 9 mg/kg. The chosen effective dose was applied to eight hemodialysis (HD) patients. Patients were assigned into two groups. One group was infused with 9 mg/kg of SNF472 and the other with a placebo for 4 h during their dialysis session. The drugs were infused into the patients two times per week for 4 weeks. Results of the study revealed that calcium phosphate crystal formation which is associated with cardiovascular calcification was significantly lower in the SNF472 administered group compared to the placebo control. Another randomized, double-blind, placebo-controlled, clinical trial with different doses of SNF472 was also conducted by the same research group to test the effect of SNF472 on HD patients and its inhibitory effect on HAP crystallization. SNF472 with several doses (1, 3, 5, 12.5, and 20 mg/kg) were administered by patients three times a week. At the end of the treatment period, pharmacokinetics analyses in the study showed no significant difference in the Cmax and AUC parameters. Additionally, SNF472 administration did not cause any adverse effects on HD patients. To determine the effect of SNF472 on cardiovascular calcification in HD patients the effective dose was selected as 10 mg/kg by DESC. Then, HD patients were administered the determined effective dose of SNF472 or placebo three times per week for 4 weeks. At the end of 4 weeks, the level of HAP crystallization was decreased in the SNF472-administered group compared to the placebo-controlled (Sanchis et al., 2018). A clinical trial performed by Raggi et al. (2020) examined the effect of SNF472 on cardiovascular calcification in patients with end-stage renal disease. In this randomized, placebo-controlled, double-blind, phase 2b study, 274 patients aged 18–80 years were selected and divided into three groups: placebo, SNF472 (300 mg), and SNF472 (600 mg) for 52 weeks. According to the study, SNF472 was administered to the patients three times a week after hemodialysis. The results showed that aortic valve progression and coronary artery calcification were less in the SNF472 (600 mg) group compared to the placebo-controlled group. The same research group also designed the CaLIPSO (Cal for calcium and ipso meaning the item itself) trial which is a multicenter, double-blind, placebo-controlled, randomized clinical study to evaluate the effect of SNF472 on hemodialysis patients with cardiovascular calcification in the coronary arteries, aorta and aortic valve. In the study, the calcium levels of the patients were initially screened for 28 days. At the end of the screening period, 274 patients were chosen depending on their Agatston coronary artery calcium (CAC) score (101–399) and randomized in a 1:1:1 ratio to administer placebo, SNF472 300 mg or SNF472 600 mg three times per week for 52 weeks. At the end of 52 weeks, the CAC score of the HD patients was significantly reduced in the SNF472-treated groups as compared to the placebo-controlled group (Bellasi et al., 2021). In general, HD patients have a low BMD, which could be associated with cardiovascular calcification (Orlic et al., 2010; Kim et al., 2021). Therefore, any dietary intervention that increases BMD could be a promising approach for the management of this problem in HD patients. Regarding this, in a further study by the same group, Bushinsky et al. (2021) investigated the effect of the SNF472 on BMD in HD patients with Agatston CAC score (101–399) which were tested with a CaLIPSO trial. The design of this study was the same as the previous one. The outcome of this study showed that SNF472 treatment did not increase the total hip and femoral neck BMD in HD patients. The total-hip BMD was significantly reduced in the 600-mg SNF472-treated group compared with the placebo control. Overall, these studies indicated that SNF472 was effective in reducing cardiovascular calcification, however, it did not have an effect against BMD in HD patients. Recently, the effects of phytate on iron absorption have been investigated by using three different ferrous derivatives. In a controlled, single-blind, randomized crossover trial, 23 adult healthy women were administered the above-mentioned derivatives over 33 days. The iron absorption of the substances was investigated with two different enhancers, cornmeal, and bouillon. According to the randomly allocated groups, two different fortifiers, namely, bouillon, and cornmeal were administered to the patients, as well as Fe-Pa-HCP (ferric iron complexed with phytic acid and hydrolyzed corn protein), FePP (ferric pyrophosphate) and FeSO4 (ferrous sulfate). Especially, Fe-Pa-Hcp given together with bouillon showed a promising effect on the patient with iron deficiency (Dold et al., 2020).
Overall, all these studies showed that both natural and artificial forms of the phytate have a promising effect on healthy volunteers, breast cancer, hemodialysis patients and cardiovascular calcification. None or minor adverse effects were reported in the aforementioned clinical trials. However, we should note that all clinical trials have some limitations such as a low number of patients, short intervention time, etc. So, further clinical trials were needed to deeply understand the positive or negative effects of phytate administration.
5 Discussion and therapeutic perspective
It is already established that phytate levels found in mammals are strongly dependent on dietary intake (oral or topical), rather than on endogenous synthesis. Besides being a naturally occurring antioxidant, dietary phytates have been claimed to prevent kidney stone formation, protect against diabetes mellitus, caries, atherosclerosis and coronary heart disease, as well as to fight the progression of a wide range of cancers (Abdulwaliyu et al., 2019; Bloot et al., 2021; Kumar et al., 2021) (Figures 3, 4).
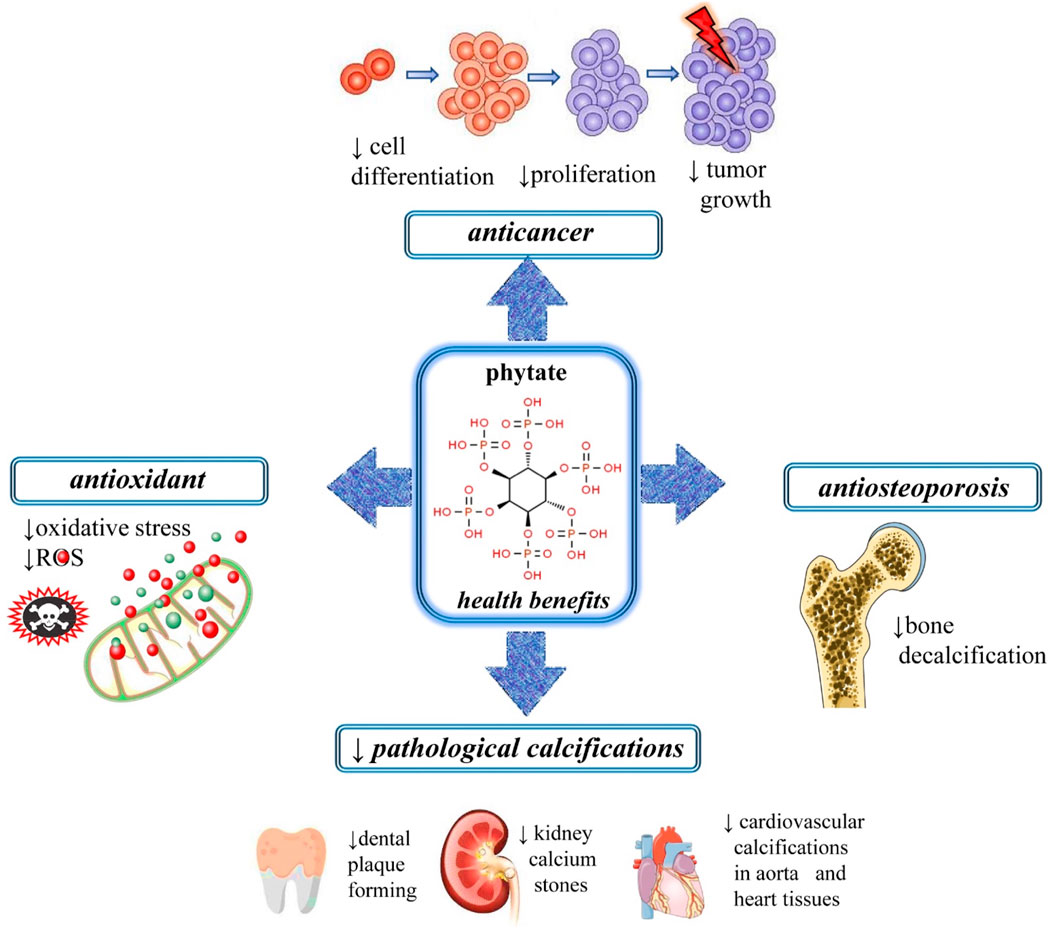
FIGURE 4. Illustrative figure with the most important therapeutic applications of phytates. Abbreviations and symbols: ↑increase, ↓decrease, ROS, reactive oxygen species.
5.1 Phytate as antioxidant
Oxidative stress occurs as a result of an imbalance between free radicals and antioxidants found in our body (Calina et al., 2016; Salehi et al., 2019; Sharifi-Rad et al., 2021a; Sharifi-Rad et al., 2021c). Free radicals can be formed from dioxygen molecules, which contain unpaired electrons (Calina et al., 2016; Islam et al., 2021; Alshehri et al., 2022; Ozkan et al., 2023). It is precisely because of the unpaired electrons that oxygen molecules react easily with other molecules (Tsoukalas et al., 2019a; Tsoukalas et al., 2019b; Salehi et al., 2019; Sharifi-Rad et al., 2021b; Salehi et al., 2021). This results in chemical chain reactions (called oxidation reactions) that can have beneficial or harmful consequences (Calina et al., 2020; Mititelu et al., 2020; Islam et al., 2021; Amin et al., 2022). Due to their chelating ability and antioxidant activity many compounds can act as regulators of heme iron excess, avoiding oxygen-initiated free radical formation and, ultimately, preventing damaging Fenton reactions (Nussbaum et al., 2017; Buga et al., 2019; Calina et al., 2020; Bhuia et al., 2023). Furthermore, inositol hexaphosphate (IP6) also proved to be effective in reducing the occurrence of metal-catalyzed protein glycation, such as advanced glycation end products (AGEs), which may trigger diabetes-related diseases (López-Moreno et al., 2022). The antioxidant capacity of phytates was first demonstrated by Sanchis et al. (2018), in chemical models, as well as in a randomized crossover study of 33 patients with type II diabetes mellitus (T2DM). Notably, the authors concluded that the dietary supplementation with IP6 for 3 months resulted in lowering the levels of circulating AGEs (∼25%) and glycated hemoglobin (3.8%) in the patients (Sanchis et al., 2018; Omoruyi et al., 2020).
5.2 Protective effect of phytate against pathological calcifications
Like other polyphosphates, phytates are considered to be powerful inhibitors of calcium salt crystallization in urine and soft tissues. Moreover, they show high-affinity binding to the calcium of hydroxyapatite (HAP) crystals through chemisorption, avoiding both crystallization and redissolution processes. Besides protecting against pathological calcifications (e.g., dental calculi, renal calculi and cardiovascular calcifications), they also present beneficial effects in the treatment of the bone decalcification process occurring during osteoporosis (López-González et al., 2008; Sanchis et al., 2021). The benefit of IP6 on bone decalcification associated with osteoporosis was demonstrated by Lopez-Gonzalez et al. (2019), in a study performed with 143 women through monitoring of bone mineral density (BMD) in the lumbar column and the neck of the femur, and questionnaires regarding osteoporosis risk factors. Regardless of the limitation of the volunteer group (inclusion only of women with a maximum of 5 years since menopause), the authors could conclude that the phytate consumption by postmenopausal women reduced their risks of hip and major osteoporotic fractures, being the positive effect more pronounced in women with risk factors of osteoporosis associated (Lopez-Gonzalez et al., 2019). The ability of IP6 in inhibiting brushite and HAP crystallization was demonstrated by Grases et al. (2009) in a study performed with 29 healthy dental plaque-forming volunteers. The subjects rinsed their mouths for 1 min, twice each day, with mouthwash solution containing phytate (0.142% as a percentage by weight) and zinc, having concluded that the treatment with zinc phytate significantly inhibited the development of tartar formation (about 70%) (Grases et al., 2009). Several studies put in evidence the ability of phytate to inhibit oxalate monohydrate formation and calcium phosphate crystals (Grases and Costa-Bauzá, 1999). The potential of IP6 in the treatment of renal calculi was also demonstrated (Grases and Costa-Bauza, 2019). As an example, in the work of Curhan et al. (2004), the authors concluded that rats fed with an AIN-76A diet (purified rodent diet containing no phytate) developed mineral deposits at the kidney corticomedullary junction, whereas a complete absence was observed in a group fed with AIN-76A + 1% IP6. In a prospective study including 96,245 females, aged 25–42, the regular ingestion of dietary IP6 was shown to significantly reduce the risk of calcium stones (Curhan et al., 2004). The women included in the study had no history of kidney stones and were followed for an 8-year period, and their diets were accessed with self-administered food frequency questionnaires. Regarding the preventive effects on cardiovascular calcifications, Grases et al. 2008, Grases et al. 2006b) reported that the oral supplementation of Wistar rats with a balanced diet (UAR-A04) containing phytate, for 76 weeks, reduced calcium deposition in the aorta and heart tissues. A retrospective cross-sectional study performed by Sanchis et al. (2016) referring to 69 chronic kidney disease patients, demonstrated that the adequate consumption of phytate could efficiently prevent abdominal aortic calcification. The phytate consumption levels were inferred from 10 food-frequency questionnaires validated on the same day of the 2-h urine analysis. In another study; Fernández-Palomeque et al. (2015) evaluated the relationship between physiological levels of urinary phytate and heart valve calcification, in a population of 188 elderly subjects (mean age: 68 years). The valve calcification of the subjects was measured by echocardiography and urinary phytate was also assessed. In addition, blood samples were also collected as part of each medical history to ascertain the existence of concomitant diseases, cardiovascular risk factors, medication usage and food habits. The obtained results clearly showed an inverse correlation between urinary phytate content and mitral annulus calcification, thus suggesting that the consumption of phytate-rich food may help to prevent cardiovascular calcification evolution (Fernández-Palomeque et al. (2015). The effects of oral supplementation with different nutrients (magnesium, zinc, iron, vitamin K) including phytate were also recently assessed in patients with calcific aortic valve stenosis but the results of the study, despite promise (Vucenik, 2019), have not yet been fully disclosed (Donato et al., 2021).
5.3 Anticancer potential
The onset of cancer is due to the uncontrolled multiplication and mutation of body cells that eventually form a tumor (Tsoukalas et al., 2019a; Ianoși et al., 2019; Jain et al., 2021; Salehi et al., 2021; Motyka et al., 2023). Several pharmacological experiments demonstrated the anticancer effects of phytate. Distinct studies support the hypothesis that IP6 reduces cell proliferation, and induces apoptosis and differentiation of malignant cells with reversion to normal phenotype, affecting several critical molecular targets (Vucenik, 2019). A broad spectrum of laboratory data has been accumulated over more than 30 years since the pioneering assays reported by Shamsuddin and Ullah, 1989, 1988), who reported the great antineoplastic potential of IP6 in rat models involving cancer of the large intestine, treated with Na-IP6 drinking water. In addition to confirming IP6 as a preeminent broad-spectrum antineoplastic agent, additional experiments also demonstrated the preventive and therapeutic potential of IP6 against cancers of different cells and different tissue systems. For example, de Lima et al. (2015) demonstrated the cytotoxic effects of a phytic acid nickel complex, on human acute leukemia Jurkat T cells. The dephosphorylated metabolites (IP4 and IP5) of phytate also presented anticancer activity and remarkably proved to be more potent in inducing apoptosis of HeLa cells than phytate (Ferry et al., 2002). Phytate anticancer effects were also assessed against several other cancer cell lines: breast and prostate cancer; cervical cancer; HepG2 hepatoma; rhabdomyosarcoma, for which it was demonstrated the efficient inhibitory effect of IP6 in human malignant cell growth in a dose and time-dependent manner (Vucenik and Shamsuddin, 2006). Other pharmacological experiments also put in evidence of phytate antineoplastic properties not only for colon cancer but also for breast carcinoma, skin papillomas, liver cancer or metastatic fibrosarcoma. The animal experiments, in agreement with in-vivo assays, highlighted a significant decrease in the number and size of tumors. Furthermore, the synergistic anticancer activity of phytate when combined with inositol was also observed (Vucenik and Shamsuddin, 2006; Vucenik, 2019). Encouraging results come also from pilot clinical studies in advanced colorectal cancer with multiple liver and lung metastases. IP6 was given to 22 patients given as an adjuvant to chemotherapy and an overall reduction of tumor growth was noticed. Furthermore, in some cases a regression of the neoplastic lesions was also detected (Vucenik, 2019). The use of IP6 in combination with chemotherapy was shown to reduce side effects, allowing the patients to perform their daily activities (Proietti et al., 2017). A case reported by Khurana et al. (2019) on a patient with metastatic melanoma, who refused the conventional chemotherapy treatment to be treated only with IP6 + inositol supplement, achieved complete remission of cancer and remained in remission 3 years later (Khurana et al., 2019).
6 Conclusion
In this review, we have compiled information on the pharmacological potential, bioavailability, clinical trials, and advantages and disadvantages of phytates, with a focus on therapeutic health effects in humans. The role of phytates in human health has been described as a dual problem since their discovery. On one hand, studies have shown that phytates can reduce the bioavailability of essential minerals for maintaining the homeostasis of the human body; on the other hand, they have proven beneficial effects for human health, such as antioxidant, the reduction of side effects of chemotherapy or the significant reduction of hydroxyapatite crystallization in hemodialysis patients. Future research in preclinical studies is needed to elucidate more pharmacological mechanisms of action of phytates as well as adverse effects and potential drug interactions. Translational pharmacological studies to determine the exact therapeutic dose effective in humans are also needed.
Author contributions
Conceptualization and design were performed by JS-R; investigation, data curation, and writing were performed by SP, RR, RP, EP-O, AS, AY, ES, and BM; review and editing were performed by SC, MM, AA, DC, WS, and JS-R; validation, supervision SC, MM, AA, DC, WS, and JS-R; All the authors contributed equally, read and approved the final manuscript. All authors contribute equally, and have read and agreed to the published version of the manuscript.
Acknowledgments
MM wants to thank ANID CENTROS BASALES ACE210012.
Conflict of interest
The authors declare that the research was conducted in the absence of any commercial or financial relationships that could be construed as a potential conflict of interest.
Publisher’s note
All claims expressed in this article are solely those of the authors and do not necessarily represent those of their affiliated organizations, or those of the publisher, the editors and the reviewers. Any product that may be evaluated in this article, or claim that may be made by its manufacturer, is not guaranteed or endorsed by the publisher.
References
Abdulwaliyu, I., Arekemase, S. O., Adudu, J. A., Batari, M. L., Egbule, M. N., and Okoduwa, S. I. R. (2019). Investigation of the medicinal significance of phytic acid as an indispensable anti-nutrient in diseases. Clin. Nutr. Exp. 28, 42–61. doi:10.1016/j.yclnex.2019.10.002
Alshehri, M. M., Quispe, C., Herrera-Bravo, J., Sharifi-Rad, J., Tutuncu, S., Aydar, E. F., et al. (2022). A review of recent studies on the antioxidant and anti-infectious properties of Senna plants. Oxid. Med. Cell Longev. 2022, 1–38. doi:10.1155/2022/6025900
Amin, R., Thalluri, C., Docea, A. O., Sharifi-Rad, J., and Calina, D. (2022). Therapeutic potential of cranberry for kidney health and diseases. eFood 3, e33. doi:10.1002/efd2.33
Anderson, R. J. (1914). A contribution to the Chemistry of phytin. J. Biol. Chem. 17, 171–190. doi:10.1016/s0021-9258(18)88416-2
Barrientos, L. G., and Murthy, P. P. (1996). Conformational studies of myo-inositol phosphates. Carbohydr. Res. 296, 39–54. doi:10.1016/s0008-6215(96)00250-9
Bellasi, A., Raggi, P., Bover, J., Bushinsky, D. A., Chertow, G. M., Ketteler, M., et al. (2021). Trial design and baseline characteristics of CaLIPSO: A randomized, double-blind placebo-controlled trial of SNF472 in patients receiving haemodialysis with cardiovascular calcification. Clin. Kidney J. 14, 366–374. doi:10.1093/ckj/sfz144
Bhuia, M. S., Rahaman, M. M., Islam, T., Bappi, M. H., Sikder, M. I., Hossain, K. N., et al. (2023). Neurobiological effects of gallic acid: Current perspectives. Chin. Med. 18, 27. doi:10.1186/s13020-023-00735-7
Bloot, A. P. M., Kalschne, D. L., Amaral, J. A. S., Baraldi, I. J., and Canan, C. (2021). A review of phytic acid sources, obtention, and applications. Food Rev. Int. 39, 73–92. doi:10.1080/87559129.2021.1906697
Bohn, L., Meyer, A. S., and Rasmussen, S. K. (2008). Phytate: Impact on environment and human nutrition. A challenge for molecular breeding. J. Zhejiang Univ. Sci. B 9, 165–191. doi:10.1631/jzus.b0710640
Buga, A. M., Docea, A. O., Albu, C., Malin, R. D., Branisteanu, D. E., Ianosi, G., et al. (2019). Molecular and cellular stratagem of brain metastases associated with melanoma. Oncol. Lett. 17, 4170–4175. doi:10.3892/ol.2019.9933
Bushinsky, D. A., Raggi, P., Bover, J., Ketteler, M., Bellasi, A., Rodriguez, M., et al. (2021). Effects of myo-inositol hexaphosphate (SNF472) on bone mineral density in patients receiving hemodialysis: An analysis of the randomized, placebo-controlled CaLIPSO study. Clin. J. Am. Soc. Nephrol. 16, 736–745. doi:10.2215/cjn.16931020
Calina, D., Buga, A. M., Mitroi, M., Buha, A., Caruntu, C., Scheau, C., et al. (2020). The treatment of cognitive, behavioural and motor impairments from brain injury and neurodegenerative diseases through cannabinoid system modulation-evidence from in vivo studies. J. Clin. Med. 9, 2395. doi:10.3390/jcm9082395
Calina, D., Rosu, L., Rosu, A. F., Ianosi, G., Ianosi, S., Zlatian, O., et al. (2016). Etiological diagnosis and pharmacotherapeutic management of parapneumonic pleurisy. Farmacia 64, 946–952.
Chen, Q. (2004). Determination of phytic acid and inositol pentakisphosphates in foods by high-performance ion chromatography. J. Agric. Food Chem. 52, 4604–4613. doi:10.1021/jf035294x
Curhan, G. C., Willett, W. C., Knight, E. L., and Stampfer, M. J. (2004). Dietary factors and the risk of incident kidney stones in younger women: Nurses' Health Study II. Arch. Intern Med. 164, 885–891. doi:10.1001/archinte.164.8.885
De Lima, E. M., Kanunfre, C. C., De Andrade, L. F., Granato, D., and Rosso, N. D. (2015). Cytotoxic effect of inositol hexaphosphate and its Ni(II) complex on human acute leukemia Jurkat T cells. Toxicol Vitro 29, 2081–2088. doi:10.1016/j.tiv.2015.08.018
Dinicola, S., Minini, M., Unfer, V., Verna, R., Cucina, A., and Bizzarri, M. (2017). Nutritional and acquired deficiencies in inositol bioavailability. Correlations with metabolic disorders. Int. J. Mol. Sci. 18, 2187. doi:10.3390/ijms18102187
Dold, S., Zimmermann, M. B., Jeroense, F., Zeder, C., Habeych, E., Galaffu, N., et al. (2020). Iron bioavailability from bouillon fortified with a novel ferric phytate compound: A stable iron isotope study in healthy women (part II). Sci. Rep. 10, 5339. doi:10.1038/s41598-020-62307-1
Donato, M., Faggin, E., Cinetto, F., Felice, C., Lupo, M. G., Ferri, N., et al. (2021). The emerging role of nutraceuticals in cardiovascular calcification: Evidence from preclinical and clinical studies. Nutrients 13, 2603. doi:10.3390/nu13082603
Fernandez-Palomeque, C., Grau, A., Perello, J., Sanchis, P., Isern, B., Prieto, R. M., et al. (2015). Relationship between urinary level of phytate and valvular calcification in an elderly population: A cross-sectional study. PLoS One 10, e0136560. doi:10.1371/journal.pone.0136560
Ferry, S., Matsuda, M., Yoshida, H., and Hirata, M. (2002). Inositol hexakisphosphate blocks tumor cell growth by activating apoptotic machinery as well as by inhibiting the Akt/NFkappaB-mediated cell survival pathway. Carcinogenesis 23, 2031–2041. doi:10.1093/carcin/23.12.2031
Grases, F., and Costa-Bauza, A. (2019). Key aspects of myo-inositol hexaphosphate (phytate) and pathological calcifications. Molecules 24, 4434. doi:10.3390/molecules24244434
Grases, F., Costa-Bauza, A., Perelló, J., Isern, B., Vucenik, I., Valiente, M., et al. (2006a). Influence of concomitant food intake on the excretion of orally administered myo-inositol hexaphosphate in humans. J. Med. Food 9, 72–76. doi:10.1089/jmf.2006.9.72
Grases, F., and Costa-Bauza, A. (1999). Phytate (IP6) is a powerful agent for preventing calcifications in biological fluids: Usefulness in renal lithiasis treatment. Anticancer Res. 19, 3717–3722.
Grases, F., and Costa-Bauzá, A. (1999). Phytate (IP6) is a powerful agent for preventing calcifications in biological fluids: Usefulness in renal lithiasis treatment. Anticancer Res. 19, 3717–3722.
Grases, F., Perelló, J., Sanchis, P., Isern, B., Prieto, R. M., Costa-Bauzá, A., et al. (2009). Anticalculus effect of a triclosan mouthwash containing phytate: A double-blind, randomized, three-period crossover trial. J. Periodontal Res. 44, 616–621. doi:10.1111/j.1600-0765.2008.01168.x
Grases, F., Sanchis, P., Perello, J., Isern, B., Prieto, R. M., Fernandez-Palomeque, C., et al. (2006b). Phytate (Myo-inositol hexakisphosphate) inhibits cardiovascular calcifications in rats. Front. Biosci. 11, 136–142. doi:10.2741/1786
Grases, F., Sanchis, P., Perello, J., Isern, B., Prieto, R. M., Fernandez-Palomeque, C., et al. (2008). Phytate reduces age-related cardiovascular calcification. Front. Biosci. 13, 7115–7122. doi:10.2741/3214
Grases, F., Simonet, B. M., Vucenik, I., Prieto, R. M., Costa-Bauzá, A., March, J. G., et al. (2001). Absorption and excretion of orally administered inositol hexaphosphate (IP(6) or phytate) in humans. Biofactors 15, 53–61. doi:10.1002/biof.5520150105
Gupta, R. K., Gangoliya, S. S., and Singh, N. K. (2015). Reduction of phytic acid and enhancement of bioavailable micronutrients in food grains. J. Food Sci. Technol. 52, 676–684. doi:10.1007/s13197-013-0978-y
Habtamu Fekadu, G. (2014). Potential health benefits and adverse effects associated with phytate in foods: A review. Glob. J. Med. Res. 14, 23–31.
Hanson, L. N., Engelman, H. M., Alekel, D. L., Schalinske, K. L., Kohut, M. L., and Reddy, M. B. (2006). Effects of soy isoflavones and phytate on homocysteine, C-reactive protein, and iron status in postmenopausal women. Am. J. Clin. Nutr. 84, 774–780. doi:10.1093/ajcn/84.4.774
Harland, B. F., Oberleas, D., Ellis, R., Gelroth, J., Gordon, D., Phillips, K., et al. (1986). Anion-exchange method for determination of phytate in foods: Collaborative study. J. Assoc. Off. Anal. Chem. 69, 667–670. doi:10.1093/jaoac/69.4.667
Hunt, J. R., and Beiseigel, J. M. (2009). Dietary calcium does not exacerbate phytate inhibition of zinc absorption by women from conventional diets. Am. J. Clin. Nutr. 89, 839–843. doi:10.3945/ajcn.2008.27175
Ianoși, S. L., Batani, A., Ilie, M. A., Tampa, M., Georgescu, S. R., Zurac, S., et al. (2019). Non-invasive imaging techniques for the in vivo diagnosis of Bowen's disease: Three case reports. Oncol. Lett. 17, 4094–4101. doi:10.3892/ol.2019.10079
Ikenaga, T., Noguchi, H., Kakumoto, K., Kohda, N., Tsukikawa, H., Matsuguma, K., et al. (2020). Effect of phytic acid on postprandial serum uric acid level in healthy volunteers: A randomized, double-blind, crossover study. Nucleosides Nucleotides Nucleic Acids 39, 504–517. doi:10.1080/15257770.2019.1656337
Islam, M. S., Quispe, C., Hossain, R., Islam, M. T., Al-Harrasi, A., Al-Rawahi, A., et al. (2021). Neuropharmacological effects of quercetin: A literature-based review. Front. Pharmacol. 12, 665031. doi:10.3389/fphar.2021.665031
Jain, D., Chaudhary, P., Varshney, N., Bin Razzak, K. S., Verma, D., Zahra, T. R. K., et al. 2021. Tobacco smoking and liver cancer risk: Potential avenues for carcinogenesis. J. Oncol., 2021, 1, 11. doi:10.1155/2021/5905357
Khoo, U., and Wolf, M. J. (1970). Origin and development of protein granules in maize endosperm. Am. J. Bot. 57, 1042–1050. doi:10.1002/j.1537-2197.1970.tb09907.x
Khurana, S., Baldeo, C., and Joseph, R. W. (2019). Inositol hexaphosphate plus inositol induced complete remission in stage IV melanoma: A case report. Melanoma Res. 29, 322–324. doi:10.1097/cmr.0000000000000577
Kim, H. J., Jung, Y. S., Jung, Y. J., Kim, O. H., and Oh, B. C. (2021). High-phytate diets increase amyloid beta deposition and apoptotic neuronal cell death in a rat model. Nutrients 13, 4370. doi:10.3390/nu13124370
Kim, O. H., Booth, C. J., Choi, H. S., Lee, J., Kang, J., Hur, J., et al. (2020). High-phytate/low-calcium diet is a risk factor for crystal nephropathies, renal phosphate wasting, and bone loss. Elife 9, e52709. doi:10.7554/elife.52709
Koba, K., Liu, J. W., Bobik, E., Mills, D. E., Sugano, M., and Huang, Y. S. (2003). Effect of phytate in soy protein on the serum and liver cholesterol levels and liver fatty acid profile in rats. Biosci. Biotechnol. Biochem. 67, 15–22. doi:10.1271/bbb.67.15
Kortekangas, A., Silventoinen, P., Nordlund, E., and Ercili-Cura, D. (2020). Phytase treatment of a protein-enriched rice bran fraction improves heat-induced gelation properties at alkaline conditions. Food Hydrocoll. 105, 105787. doi:10.1016/j.foodhyd.2020.105787
Kumar, A., Singh, B., Raigond, P., Sahu, C., Mishra, U. N., Sharma, S., et al. (2021). Phytic acid: Blessing in disguise, a prime compound required for both plant and human nutrition. Food Res. Int. 142, 110193. doi:10.1016/j.foodres.2021.110193
Lee, S.-H., Park, H.-J., Chun, H.-K., Cho, S.-Y., Cho, S.-M., and Lillehoj, H. S. (2006). Dietary phytic acid lowers the blood glucose level in diabetic KK mice. Nutr. Res. 26, 474–479. doi:10.1016/j.nutres.2006.06.017
Lehrfeld, J. (1994). HPLC separation and quantitation of phytic acid and some inositol phosphates in foods: Problems and solutions. J. Agric. Food Chem. 42, 2726–2731. doi:10.1021/jf00048a015
Lei, X. G., and Porres, J. M. (2003). Phytase enzymology, applications, and biotechnology. Biotechnol. Lett. 25, 1787–1794. doi:10.1023/a:1026224101580
Liu, H., and Tu, J. (2021). Reduction of extrinsic tooth stain by a toothpaste containing 10% high cleaning silica, 0.5% sodium phytate and 0.5% sodium pyrophosphate: An 8-week randomised clinical trial. BMC Oral Health 21, 113. doi:10.1186/s12903-021-01484-5
Lopez, H. W., Leenhardt, F., Coudray, C., and Remesy, C. (2002). Minerals and phytic acid interactions: Is it a real problem for human nutrition? Int. J. Food Sci. Technol. 37, 727–739. doi:10.1046/j.1365-2621.2002.00618.x
López-González, A. A., Grases, F., Roca, P., Mari, B., Vicente-Herrero, M. T., and Costa-Bauzá, A. (2008). Phytate (myo-inositol hexaphosphate) and risk factors for osteoporosis. J. Med. Food 11, 747–752. doi:10.1089/jmf.2008.0087
Lopez-Gonzalez, A. A. L., Grases, F., Mari, B., Tomas-Salva, M., and Rodriguez, A. (2019). Urinary phytate concentration and risk of fracture determined by the FRAX index in a group of postmenopausal women. Turk J. Med. Sci. 49, 458–463. doi:10.3906/sag-1806-117
López-Moreno, M., Garcés-Rimón, M., and Miguel, M. (2022). Antinutrients: Lectins, goitrogens, phytates and oxalates, friends or foe? J. Funct. Foods 89, 104938. doi:10.1016/j.jff.2022.104938
Maffucci, T., and Falasca, M. (2020). Signalling properties of inositol polyphosphates. Molecules 25, 5281. doi:10.3390/molecules25225281
Markiewicz, L. H., Ogrodowczyk, A. M., Wiczkowski, W., and Wroblewska, B. (2021). Phytate and butyrate differently influence the proliferation, apoptosis and survival pathways in human cancer and healthy colonocytes. Nutrients 13, 1887. doi:10.3390/nu13061887
Mc, C. R., and Walsham, C. M. (1948). The digestibility and absorption of the calories, proteins, purines, fat and calcium in wholemeal wheaten bread. Br. J. Nutr. 2, 26–41. doi:10.1079/bjn19480006
Mccance, R. A., and Widdowson, E. M. (1942). Mineral metabolism of healthy adults on white and Brown bread dietaries. J. Physiol. 101, 44–85. doi:10.1113/jphysiol.1942.sp003967
Mititelu, R. R., Padureanu, R., Bacanoiu, M., Padureanu, V., Docea, A. O., Calina, D., et al. (2020). Inflammatory and oxidative stress markers-mirror tools in rheumatoid arthritis. Biomedicines 8, 125. doi:10.3390/biomedicines8050125
Motyka, S., Jafernik, K., Ekiert, H., Sharifi-Rad, J., Calina, D., Al-Omari, B., et al. (2023). Podophyllotoxin and its derivatives: Potential anticancer agents of natural origin in cancer chemotherapy. Biomed. Pharmacother. 158, 114145. doi:10.1016/j.biopha.2022.114145
Nahapetian, A., and Young, V. R. (1980). Metabolism of 14C-phytate in rats: Effect of low and high dietary calcium intakes. J. Nutr. 110, 1458–1472. doi:10.1093/jn/110.7.1458
Nussbaum, L., Hogea, L. M., Calina, D., Andreescu, N., Gradinaru, R., Stefanescu, R., et al. (2017). Modern treatment approaches in psychoses. Pharmacogenetic, neuroimagistic and clinical implications. Farmacia 65, 75–81.
Omoruyi, F. O., Budiaman, A., Eng, Y., Olumese, F. E., Hoesel, J. L., Ejilemele, A., et al. 2013. The potential benefits and adverse effects of phytic Acid supplement in streptozotocin-induced diabetic rats. Adv. Pharmacol. Sci., 2013, 1–7. doi:10.1155/2013/172494
Omoruyi, F. O., Stennett, D., Foster, S., and Dilworth, L. (2020). New Frontiers for the use of IP6 and inositol combination in treating diabetes mellitus: A review. Molecules 25, 1720. doi:10.3390/molecules25071720
Ozkan, G., Günal-Köroğlu, D., Karadag, A., Capanoglu, E., Cardoso, S. M., Al-Omari, B., et al. (2023). A mechanistic updated overview on lycopene as potential anticancer agent. Biomed. Pharmacother. 161, 114428. doi:10.1016/j.biopha.2023.114428
Pallem, P., Bodiga, S., and Bodiga, V. L. (2020). Dietary phytate lowers K-ras mutational frequency, decreases DNA-adduct and hydroxyl radical formation in azoxymethane-induced colon cancer. Iran. J. Basic Med. Sci. 23, 20–29. doi:10.22038/IJBMS.2019.34374.8161
Parkinson, C. R., Burnett, G. R., Creeth, J. E., Lynch, R. J. M., Budhawant, C., Lippert, F., et al. (2018). Effect of phytate and zinc ions on fluoride toothpaste efficacy using an in situ caries model. J. Dent. 73, 24–31. doi:10.1016/j.jdent.2018.03.013
Perelló, J., Joubert, P. H., Ferrer, M. D., Canals, A. Z., Sinha, S., and Salcedo, C. (2018). First-time-in-human randomized clinical trial in healthy volunteers and haemodialysis patients with SNF472, a novel inhibitor of vascular calcification. Br. J. Clin. Pharmacol. 84, 2867–2876. doi:10.1111/bcp.13752
Persson, H., Turk, M., Nyman, M., and Sandberg, A. S. (1998). Binding of Cu2+, Zn2+ and Cd2+ to inositol tri-tetra-penta, and hexaphosphates. J. Agric. Food Chem. 46, 4464–3200. doi:10.1021/jf9810362
Prieto, R. M., Fiol, M., Perello, J., Estruch, R., Ros, E., Sanchis, P., et al. (2010). Effects of Mediterranean diets with low and high proportions of phytate-rich foods on the urinary phytate excretion. Eur. J. Nutr. 49, 321–326. doi:10.1007/s00394-009-0087-x
Proietti, S., Pasta, V., Cucina, A., Aragona, C., Palombi, E., Vucenik, I., et al. (2017). Inositol hexaphosphate (InsP6) as an effective topical treatment for patients receiving adjuvant chemotherapy after breast surgery. Eur. Rev. Med. Pharmacol. Sci. 21, 43–50.
PUBCHEM. 2022. PubChem [Online]. Available: https://pubchem.ncbi.nlm.nih.gov/.
Pujol, A., Sanchis, P., Grases, F., and Masmiquel, L. (2023). Phytate intake, health and disease: “Let thy food Be thy medicine and medicine Be thy Food&rdquo. Antioxidants 12, 146. doi:10.3390/antiox12010146
Quispe, C., Herrera-Bravo, J., Javed, Z., Khan, K., Raza, S., Gulsunoglu-Konuskan, Z., et al. (2022). Therapeutic applications of curcumin in diabetes: A review and perspective. Biomed. Res. Int. 2022, 1–14. doi:10.1155/2022/1375892
Raboy, V. (2003). myo-Inositol-1,2,3,4,5,6-hexakisphosphate. Phytochemistry 64, 1033–1043. doi:10.1016/s0031-9422(03)00446-1
Raggi, P., Bellasi, A., Bushinsky, D., Bover, J., Rodriguez, M., Ketteler, M., et al. (2020). Slowing progression of cardiovascular calcification with SNF472 in patients on hemodialysis: Results of a randomized phase 2b study. Circulation 141, 728–739. doi:10.1161/circulationaha.119.044195
Ravindran, V., Ravindran, G., and Sivalogan, S. (1994). Total and phytate phosphorus contents of various foods and feedstuffs of plant origin. Food Chem. 50, 133–136. doi:10.1016/0308-8146(94)90109-0
Salcedo, C., Joubert, P. H., Ferrer, M. D., Canals, A. Z., Maduell, F., Torregrosa, V., et al. (2019). A phase 1b randomized, placebo-controlled clinical trial with SNF472 in haemodialysis patients. Br. J. Clin. Pharmacol. 85, 796–806. doi:10.1111/bcp.13863
Salehi, B., Prakash Mishra, A., Nigam, M., Karazhan, N., Shukla, I., Kiełtyka-Dadasiewicz, A., et al. (2021). Ficus plants: State of the art from a phytochemical, pharmacological, and toxicological perspective. Phytotherapy Res. 35, 1187–1217. doi:10.1002/ptr.6884
Salehi, B., Sestito, S., Rapposelli, S., Peron, G., Calina, D., Sharifi-Rad, M., et al. (2019). Epibatidine: A promising natural alkaloid in health. Biomolecules 9, 6. doi:10.3390/biom9010006
Sanchis, P., Buades, J. M., Berga, F., Gelabert, M. M., Molina, M., Íñigo, M. V., et al. (2016). Protective effect of myo-inositol hexaphosphate (phytate) on abdominal aortic calcification in patients with chronic kidney disease. J. Ren. Nutr. 26, 226–236. doi:10.1053/j.jrn.2016.01.010
Sanchis, P., Lopez-Gonzalez, A. A., Costa-Bauza, A., Busquets-Cortes, C., Riutord, P., Calvo, P., et al. (2021). Understanding the protective effect of phytate in bone decalcification related-diseases. Nutrients 13, 2859. doi:10.3390/nu13082859
Sanchis, P., Rivera, R., Berga, F., Fortuny, R., Adrover, M., Costa-Bauza, A., et al. (2018). Phytate decreases formation of advanced glycation end-products in patients with type II diabetes: Randomized crossover trial. Sci. Rep. 8, 9619. doi:10.1038/s41598-018-27853-9
Schlemmer, U., Frolich, W., Prieto, R. M., and Grases, F. (2009). Phytate in foods and significance for humans: Food sources, intake, processing, bioavailability, protective role and analysis. Mol. Nutr. Food Res. 53, S330–S375. doi:10.1002/mnfr.200900099
Shamsuddin, A. M., Elsayed, A. M., and Ullah, A. (1988). Suppression of large intestinal cancer in F344 rats by inositol hexaphosphate. Carcinogenesis 9, 577–580. doi:10.1093/carcin/9.4.577
Shamsuddin, A. M., and Ullah, A. (1989). Inositol hexaphosphate inhibits large intestinal cancer in F344 rats 5 months after induction by azoxymethane. Carcinogenesis 10, 625–626. doi:10.1093/carcin/10.3.625
Sharifi-Rad, J., Dey, A., Koirala, N., Shaheen, S., El Omari, N., Salehi, B., et al. (2021a). Cinnamomum species: Bridging phytochemistry knowledge, pharmacological properties and toxicological safety for health benefits. Front. Pharmacol. 12, 600139. doi:10.3389/fphar.2021.600139
Sharifi-Rad, J., Quispe, C., Herrera-Bravo, J., Martorell, M., Sharopov, F., Tumer, T. B., et al. (2021b). A pharmacological perspective on plant-derived bioactive molecules for epilepsy. Neurochem. Res. 46, 2205–2225. doi:10.1007/s11064-021-03376-0
Sharifi-Rad, J., Quispe, C., Imran, M., Rauf, A., Nadeem, M., Gondal, T. A., et al. (2021c). Genistein: An integrative overview of its mode of action, pharmacological properties, and health benefits. Oxidative Med. Cell. Longev. 2021, 1–36. doi:10.1155/2021/3268136
Silva, E. O., and Bracarense, A. P. (2016). Phytic acid: From antinutritional to multiple protection factor of organic systems. J. Food Sci. 81, R1357–R1362. doi:10.1111/1750-3841.13320
Silva, V. M., Putti, F. F., White, P. J., and Reis, A. R. D. (2021). Phytic acid accumulation in plants: Biosynthesis pathway regulation and role in human diet. Plant Physiol. Biochem. 164, 132–146. doi:10.1016/j.plaphy.2021.04.035
Tsoukalas, D., Fragkiadaki, P., Docea, A. O., Alegakis, A. K., Sarandi, E., Vakonaki, E., et al. (2019a). Association of nutraceutical supplements with longer telomere length. Int. J. Mol. Med. 44, 218–226. doi:10.3892/ijmm.2019.4191
Tsoukalas, D., Fragoulakis, V., Sarandi, E., Docea, A. O., Papakonstaninou, E., Tsilimidos, G., et al. (2019b). Targeted metabolomic analysis of serum fatty acids for the prediction of autoimmune diseases. Front. Mol. Biosci. 6, 120. doi:10.3389/fmolb.2019.00120
Vucenik, I. (2019). Anticancer properties of inositol hexaphosphate and inositol: An overview. J. Nutr. Sci. Vitaminol. (Tokyo) 65, S18–s22. doi:10.3177/jnsv.65.s18
Vucenik, I., and Shamsuddin, A. M. (2003). Cancer inhibition by inositol hexaphosphate (IP6) and inositol: From laboratory to clinic. J. Nutr. 133, 3778s–3784s. doi:10.1093/jn/133.11.3778s
Vucenik, I., and Shamsuddin, A. M. (2006). Protection against cancer by dietary IP6 and inositol. Nutr. Cancer 55, 109–125. doi:10.1207/s15327914nc5502_1
Wang, R., and Guo, S. (2021). Phytic acid and its interactions: Contributions to protein functionality, food processing, and safety. Compr. Rev. Food Sci. Food Saf. 20, 2081–2105. doi:10.1111/1541-4337.12714
Weremko, D., Fandrejewski, H., Zebrowska, T., Han, I. K., Kim, J. H., and Cho, W. T. (1997). Bioavailability of phosphorus in feeds of plant origin for pigs - review. Asian-Australasian J. Animal Sci. 10, 551–566. doi:10.5713/ajas.1997.551
Keywords: phytate, nutrients, health benefits, clinical trials, organophosphorus compound
Citation: Pires SMG, Reis RS, Cardoso SM, Pezzani R, Paredes-Osses E, Seilkhan A, Ydyrys A, Martorell M, Sönmez Gürer E, Setzer WN, Abdull Razis AF, Modu B, Calina D and Sharifi-Rad J (2023) Phytates as a natural source for health promotion: A critical evaluation of clinical trials. Front. Chem. 11:1174109. doi: 10.3389/fchem.2023.1174109
Received: 25 February 2023; Accepted: 03 April 2023;
Published: 14 April 2023.
Edited by:
Huilian Huang, Jiangxi University of Traditional Chinese Medicine, ChinaReviewed by:
Carla Pereira, Centro de Investigação de Montanha (CIMO), PortugalMaharshi Bhaswant, Tohoku University, Japan
Guangtong Chen, Nantong University, China
Copyright © 2023 Pires, Reis, Cardoso, Pezzani, Paredes-Osses, Seilkhan, Ydyrys, Martorell, Sönmez Gürer, Setzer, Abdull Razis, Modu, Calina and Sharifi-Rad. This is an open-access article distributed under the terms of the Creative Commons Attribution License (CC BY). The use, distribution or reproduction in other forums is permitted, provided the original author(s) and the copyright owner(s) are credited and that the original publication in this journal is cited, in accordance with accepted academic practice. No use, distribution or reproduction is permitted which does not comply with these terms.
*Correspondence: Susana M. Cardoso, susanacardoso@ua.pt; Miquel Martorell, mmartorell@udec.cl; Ahmad Faizal Abdull Razis, madfaizal@upm.edu.my; Daniela Calina, calinadaniela@gmail.com; Javad Sharifi-Rad, javad.sharifirad@gmail.com