- 1Frontiers Science Center for Flexible Electronics (FSCFE), Institute of Flexible Electronics (IFE), Northwestern Polytechnical University, Xi’an, China
- 2MIIT Key Laboratory of Flexible Electronics (KLoFE), Northwestern Polytechnical University, Xi’an, China
- 3Shaanxi Key Laboratory of Flexible Electronics (KLoFE), Northwestern Polytechnical University, Xi’an, China
- 4Institute of Basic and Translational Medicine, Xi’an Medical University, Xi’an, China
- 5School of Chemistry and Chemical Engineering, Shaanxi Normal University, Xi’an, China
- 6Key Laboratory of Flexible Electronics of Zhejiang Province, Ningbo Institute of Northwestern Polytechnical University, Ningbo, China
The atomically thin two-dimensional (2D) transition metal dichalcogenides (TMDCs) have attracted the researcher’s interest in the field of flexible electronics due to their high mobility, tunable bandgaps, and mechanical flexibility. As an emerging technique, laser-assisted direct writing has been used for the synthesis of TMDCs due to its extremely high preparation accuracy, rich light–matter interaction mechanism, dynamic properties, fast preparation speed, and minimal thermal effects. Currently, this technology has been focused on the synthesis of 2D graphene, while there are few literatures that summarize the progress in direct laser writing technology in the synthesis of 2D TMDCs. Therefore, in this mini-review, the synthetic strategies of applying laser to the fabrication of 2D TMDCs have been briefly summarized and discussed, which are divided into top-down and bottom-up methods. The detailed fabrication steps, main characteristics, and mechanism of both methods are discussed. Finally, prospects and further opportunities in the booming field of laser-assisted synthesis of 2D TMDCs are addressed.
1 Introduction
Flexible electronics is a disruptive science and technology based on a high degree of interdisciplinary integration, which provides novel opportunities for the development of the next generation of the information technology revolution and the era of intelligent manufacturing (Zheng et al., 2022; Li et al., 2023). The two-dimensional materials, especially transition metal dichalcogenides have become trending research topics of flexible electronics due to their unique structure, mechanical flexibility, tunable bandgaps, and high mobility (Mak et al., 2010; Fan et al., 2015; Kim et al., 2015; Leong, 2020; Yu et al., 2020; Liu et al., 2021; Wu et al., 2022).
Currently, significant research has been focused on the efficient synthesis of high-quality large-scale 2D TMDCs with simple crafts and low costs, owing to the prerequisite and key factor for their diverse advanced industrial application (Lee et al., 2012; Lei et al., 2013; Mahjouri Samani et al., 2014; Shim et al., 2014; Xia et al., 2014; Xu et al., 2021b). Various synthesis techniques, such as chemical vapor deposition (CVD), metal–organic chemical vapor deposition (MOCVD), and molecular beam epitaxy (MBE), have been used to control the growth of 2D TMDCs on different substrates and show great potential in growing high-quality crystalline 2D TMDCs under high temperature (Yu et al., 2017; Gao et al., 2021). However, patterning TMDCs cannot be achieved in the synthesis process, and an additional patterning process is still required during the device fabrication (Mak et al., 2010). In recent years, direct laser writing technology has been widely used in the synthesis of materials because of the ability to minimize thermal effects, rich light–matter interaction mechanism, kinetic properties, the high fabrication accuracy, and fast preparation speed (Lu et al., 2014; Jung et al., 2019; Park et al., 2020a; Park et al., 2020b; Hu et al., 2020). In addition, maskless and lithography-free properties of laser patterning can enable one-step fabrication of the desired specific patterns, avoiding material contamination while reducing the process flow (Cao et al., 2013; Mohapatra et al., 2020). All preparation methods of synthesizing 2D TMDCs and their advantages and disadvantages are shown in Table 1, which shows the salient features of direct laser writing technology from multiple perspectives.
In this mini-review, we systematically summarize the latest studies on the laser-assisted synthesis of 2D TMDCs. Basically, there are essentially two classes of synthetic strategies: top-down and bottom-up methods. With regard to top-down methods, the advantages and mechanisms of the laser–material interactions are discussed, including laser exfoliation, laser thinning, and laser-driven phase transition. With regard to bottom-up methods, the growth mechanisms are discussed, including direct laser writing, laser heterostructures, and laser doping. Finally, prospects and further opportunities in the laser-assisted synthesis of 2D TMDCs are also addressed.
2 Top-down synthesis methods
Top-down synthesis methods generally require synthesizing the 2D TMDC crystal first, followed by further processing via laser–material interactions to improve the morphology or properties. Specifically, it can be classified into laser stripping, laser thinning, and laser-driven phase transition.
2.1 Laser exfoliation
The unique characteristics of the laser, such as ultra-high-peak power density and short pulse duration, far exceed conventional heating methods, such as electric or gas heating, thus making it possible to exfoliate monolayers of two-dimensional materials. The van der Waals interaction between the layers of TMDC materials can be broken by the laser, which enables the efficient and controllable preparation of TMDCs with a specific number of layers in a relatively short time. In addition, this technique has now been used to exfoliate a variety of 2D TMDC materials such as MoS2, MoSe2, and WS2 under different conditions (Schuffenhauer et al., 2005; Hu et al., 2006; An et al., 2018; Gao et al., 2019; Zhai et al., 2021; Zuo et al., 2021; Kimiagar and Abrinaei, 2023).
TMDCs are layered materials that form an MX2 crystal structure composed of strongly covalently bonded X-M-X sandwiches, and the X-M-X layers are held together by weak van der Waals forces (An et al., 2018). Zhai et al. (2021) created a method for exfoliating 2H-phase MoS2 (2H-MoS2), that is, both efficient and free from contaminants, and also the exfoliation process can be directly observed by optical microscopy (Figure 1A). Compared to other exfoliation techniques, this method is significantly faster and easier. The thickness of the irradiated region underwent a reduction approximately from 100 nm to 5 nm, and the area of the peeled region is also much larger than the diameter of the laser spot used. They believed that the light illumination and water medium are essential for the laser exfoliation of 2D TMDCs. When 2H-MoS2 layers are exposed to laser irradiation, the rapid vaporization of water molecules between the layers results in the exfoliation of MoS2 sheets, and the exfoliation process can be controlled by applying a bias voltage. The related research results indicated that water molecules could be incorporated into the interlayer spaces of 2H-MoS2 sheets (Levita and Righi, 2017; Ma et al., 2018). The phase transition may accompany the exfoliation process. Gao et al. (2019) achieved a one-step exfoliating bulk 2H-MoS2 into 2H and 1T MoS2 nanosheets using pulsed laser irradiation. Figure 1B shows a conceptual model of this experimental mechanism, suggesting that the 2H-phase MoS2 can be reversed into 1T MoS2 with the assistance of the chemically doped Fe3+ ions. In addition, the capability of the protic solvent also provides the proton to the reaction environment and plays a crucial role in triggering the phase transition.
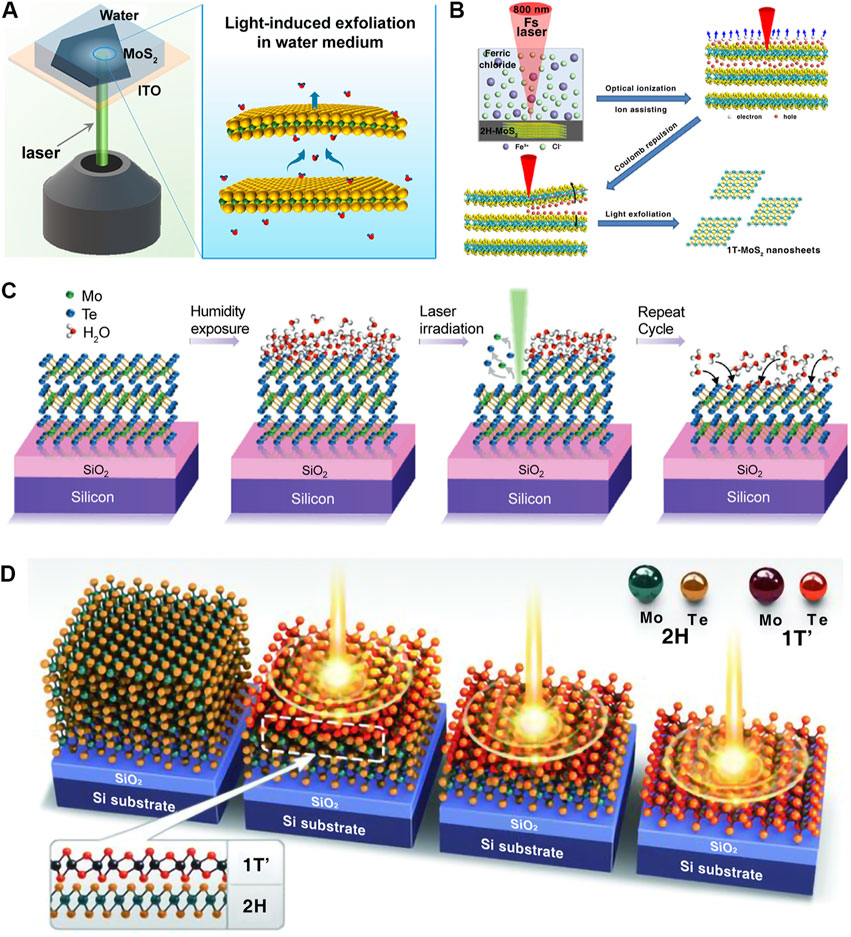
FIGURE 1. Top-down synthesis methods. (A) Schematic of the laser exfoliation device for 2H-MoS2 flakes in aqueous media (Zhai et al., 2021) (Copyright 2021, American Chemical Society). (B) Schematic of the preparation of MoS2 nanosheets through femtosecond laser exfoliation (Zuo et al., 2021) (Copyright 2021, American Chemical Society). (C) Schematic of the mechanism for laser thinning of MoTe2 (Nagareddy et al., 2018) (Copyright 2018, Wiley-VCH). (D) Schematic of the laser-driven phase patterning process of MoTe2 from 2H to 1T′ (Cho et al., 2015) (Copyright 2015, American Association for the Advancement of Science).
2.2 Laser thinning
Laser thinning techniques have provided a direct and site-specific method for removing layers and obtaining “on-demand” 2D TMDCs (Castellanos Gomez et al., 2012; Lu et al., 2014; Sunamura et al., 2016; Kim et al., 2017; Park et al., 2017; Gong et al., 2018; Nagareddy et al., 2018; Rho et al., 2019; Tran-Khac et al., 2019; Wang et al., 2020; Kang et al., 2021). Unlike the traditional gas or plasma etching thinning method, laser thinning shows high accuracy on thinned layers, flexible programmable patterning mode, and high thinning efficiency. Nagareddy et al. (2018) demonstrated an efficient laser thinning method, which was constructed by the Raman spectroscopy and coupled with an atomic force microscope. The experimental setup was placed in an airtight environment with strictly controlled humidity. Using ultra-low laser power, the thickness of MoTe2 film can be controlled layer by layer from multilayer to monolayer, as shown in Figure 1C. In addition, the thickness reduction shows the linear correlation between the thickness reduction and the number of scans. The laser thinning process is achieved through the sublimation of the top layer, mainly caused by the conversion of light absorbed by the material into heat energy (Lu et al., 2014). The heat generated by the conversion of absorbed laser into energy is difficult to dissipate through the substrate due to the poor coupling between TMDC thin layers that are mediated by van der Waals forces (Su et al., 2021). Thus, until the laser power is increased, the bottom layer remains in close contact with the SiO2/Si substrate, acting as a heat sink to prevent removal.
2.3 Laser driven phase
The exploration and manipulation of a novel phase of matter is a primary pursuit for materials research. The emergence of atomically 2D TMDCs has enabled the examination of diffusive, displacive, and quantum phase transitions. Cho et al. (2015) demonstrated a novel method of localized polymorph engineering and realized the laser-induced phase transition of MoTe2. The multilayer 2H-MoTe2 flake with about 30 layers was obtained by mechanical exfoliation. The schematic diagram of the phase transition mechanism is illustrated in Figure 1D. Under laser irradiation, the thickness of the irradiated region in 2H-MoTe2 decreased by a few layers and transformed to 1T′ phase. During the irradiation process, the 1T′ MoTe2 layer remained due to the heat sink effect of the SiO2 substrate. It should be noticed that the phase transition is irreversible from 1T′ to 2H phase even under the higher energy or intensity of the laser. The driving force for the one-way phase transition is caused by the irreversible Te vacancy created under laser irradiation. Compared with conventional methods such as heat treatment, strain engineering, charge transfer, and plasma irradiation, laser-induced phase transition engineering allows for specific phase transition sites and patterned processing without impurities during the phase transition (Chen Z. R. et al., 2019).
3 Bottom-up synthesis methods
Unlike the top-down synthesis method, the bottom-up synthesis method is more efficient and cost-effective in the synthesis of 2D TMDC materials because it does not require the preparation of precursors using other methods. With the assistance of a laser, large-area patterned TMDCs, heterostructure, and atom doping can be realized.
3.1 Laser directed synthesis
Direct laser writing technology can directly pattern 2D TMDC materials without mask and lithography, reducing the process flow of device fabrication and the risk of chemical contamination. Generally, the fiber laser (1.06 μm), carbon dioxide laser (10.6 μm), and femtosecond laser (780 nm) have been adopted for the synthesis of 2D TMDCs (Hu et al., 2018). Xu et al. (2021a) reported a method for the efficient synthesis of wafer-scale MoS2 using a direct laser writing technique, and the workflow is shown in Figure 2A. With heating by a 1.06 μm commercial fiber laser, the MoS2 can be programmable with text, lines, patterns, and matrices in a few minutes. The laser interacts with the precursor, and the reaction temperature facilitates the thermal decomposition of (NH4)2MoS4 into MoS2. Apart from the thermal decomposition, the photochemical reaction induced by two-photon absorption can also be adopted for synthesizing 2D TMDCs. As shown in Figure 2B, the femtosecond direct laser writing was carried out to synthesize MoS2 under an objective lens. Molybdenum acetylacetonate and carbon disulfide were adopted as the molybdenum and sulfur sources. The two-photon absorption occurs in the focal point, synthesizing MoS2 from the molybdenum metal complex via a photochemical reaction. With the assistance of a femtosecond laser, a minimal line width of approximately 780 nm can be achieved.
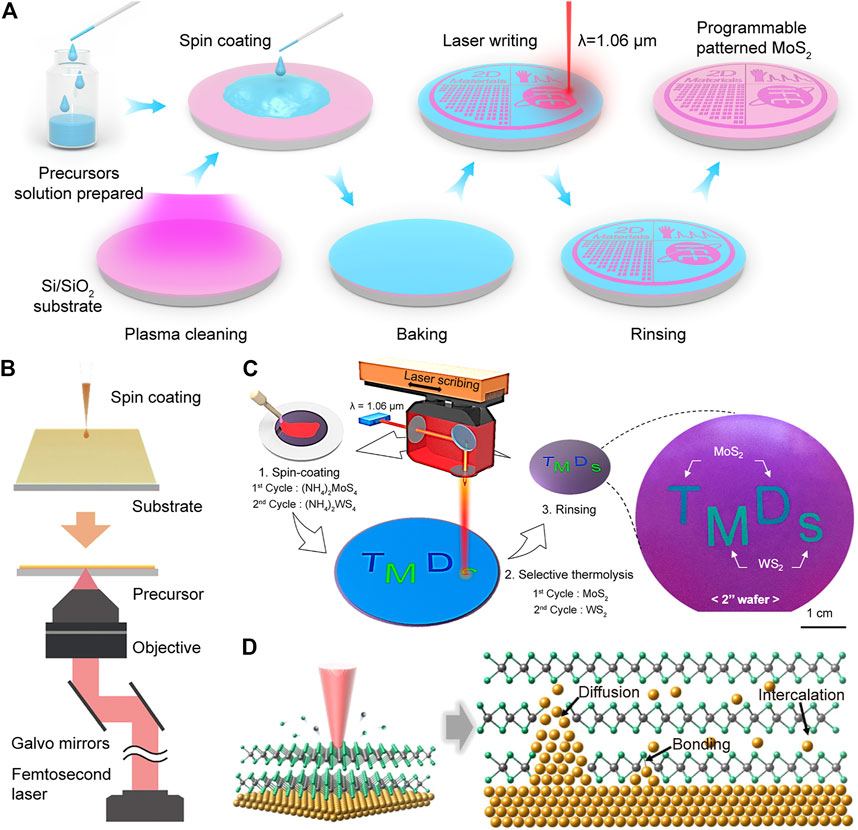
FIGURE 2. Bottom-up synthesis methods. (A) Flow diagram of direct laser writing, a method for the laser-directed synthesis of MoS2 on the SiO2/Si wafer (Xu et al., 2021a) (Copyright 2021, Elsevier). (B) Schematic of MoS2 synthesized by femtosecond laser (Xu et al., 2022) (Copyright 2022, American Chemical Society). (C) Schematic of the layer synthesis of MoS2–WS2 heterostructure (Park et al., 2020a) (Copyright 2020, American Chemical Society). (D) Schematic of Au-doped MoS2 (Huo et al., 2021) (Copyright 2021, American Chemical Society).
3.2 Laser heterostructure
The direct laser writing technique allows the fabrication of single 2D TMDCs and the direct preparation of 2D TMDC heterostructures. Compared with the transfer method, the laser heterostructure process can be programmable patterned, avoiding material damage and pollution (Li et al., 2020). In addition, the synthesis efficiency of heterostructures is greatly improved compared with CVD. Park et al. (2020a) successfully prepared WS2–MoS2 heterostructures vertically by two-step laser scribing, as shown in Figure 2C. The MoS2 layer was first synthesized by the thermal decomposition of (NH4)2MoS4 when the temperature increased over 700°C under laser irradiation. While for the heterostructures, due to the different optical absorption coefficients, the MoS2 layer shows a minor temperature increase, and only the (NH4)2WS4 layer absorbed the laser energy and selectively decomposed into WS2. Thus, the selective growth process by laser effectively produces layer-by-layer 2D TMDC heterostructures in a programmable pattern.
3.3 Laser doping
The impurity atom can modulate the physical and chemical properties of semiconductors such as electrical and optical. A small amount of elemental doping could affect the energy bands enhancing semiconductor conductivity. However, doping elements in 2D TMDCs are relatively difficult and generate more defects in the 2D TMDCs. In addition, the weak interaction will result in unstable interfaces due to the interaction between doped elements and 2D TMDCs (Mak et al., 2010). Laser provides a simple way for 2D TMDC doping, which is promising for future electronic applications (Chen J. et al., 2019). One way to achieve laser doping in 2D TMDCs is by dissolving the doping substance in the precursor, which results in doping during formation (Hu et al., 2020). In addition, doping of elements in preprepared 2D TMDCs can also be accomplished by introducing gas molecules or solids containing the desired doping atoms (Afaneh et al., 2018; Rho et al., 2019; Huo et al., 2021). For example, Huo et al. (2021) reported an Au-doped MoS2 process by doping a solid source, as shown in Figure 2D. MoS2 was transferred to the Au electrode, and with femtosecond laser irradiation, the Au atom can be intercalated and diffused into MoS2. The interface diffusion and chemical bonding of Au reduced the Schottky barrier of the metal–semiconductor interfaces, which could enhance the performance of the devices.
4 Conclusion and outlook
This mini-review summarized the top-down and bottom-up methods for the laser synthesis of 2D TMDCs. Compared with the traditional methods, the laser synthesis methods have the advantages of flexibility, convenience, efficiency, and controllability. Laser synthesis technology can directly produce large-area, high-quality TMDCs, alloys, and heterostructure, which is a promising sign for future industrial-scale manufacturing.
Laser synthesis of 2D TMDCs is an emerging field, and some issues need to be further addressed, especially for the laser–reactive matter interaction. In addition, the synthesis of sulfides in the atmospheric environment is the focus of attention, while the atmosphere protection and hydrogen participation would be focused while synthesizing selenide and telluride. In addition, recent works on laser synthesis of 2D TMDCs constructed on the rigid substrate (such as SiO2/Si) or liquid environments and direct laser synthesis on flexible substrates (such as polyimide, polydimethylsiloxane, polyethylene terephthalate, and polyethylene naphthalene) have not been realized yet, which would offer another chance for realizing applications in flexible electronics.
Author contributions
HW: investigation, methodology, visualization, and writing—original draft. MX: investigation, methodology, writing—original draft, writing—review and editing, visualization, and funding acquisition. HJ: investigation, writing—original draft, writing—review and editing, and visualization. TH: investigation, methodology, and conceptualization. WL: methodology, writing—review and editing, and funding acquisition. LZ: methodology, conceptualization, writing—review and editing, and funding acquisition. XW: conceptualization, methodology, writing—review and editing, supervision, project administration, and funding acquisition.
Funding
The authors gratefully acknowledge financial support from the National Key Research and Development Program of China (2020YFB2008501), the National Natural Science Foundation of China (11904289), the Key Research and Development Program of Shaanxi Province (2020ZDLGY04-08 and 2020GXLH-Z-027), the Natural Science Foundation of Shaanxi Province (2023-JC-YB-495, 2022JQ-659, and 2022JQ-552), the Natural Science Foundation of Ningbo (202003N4003), the Fundamental Research Funds for the Central Universities (3102019PY004, 31020190QD010, and 3102019JC004), the start-up funds from Northwestern Polytechnical University, and the Open Research Fund of the State Key Laboratory of Organic Electronics and Information Displays.
Conflict of interest
The authors declare that the research was conducted in the absence of any commercial or financial relationships that could be construed as a potential conflict of interest.
Publisher’s note
All claims expressed in this article are solely those of the authors and do not necessarily represent those of their affiliated organizations, or those of the publisher, the editors, and the reviewers. Any product that may be evaluated in this article, or claim that may be made by its manufacturer, is not guaranteed or endorsed by the publisher.
References
Afaneh, T., Sahoo, P. K., Nobrega, I. A. P., Xin, Y., and Gutiérrez, H. R. (2018). Laser-assisted chemical modification of monolayer transition metal dichalcogenides. Adv. Funct. Mater. 28 (37), 1802949. doi:10.1002/adfm.201802949
An, S. J., Kim, Y. H., Lee, C., Park, D. Y., and Jeong, M. S. (2018). Exfoliation of transition metal dichalcogenides by a high-power femtosecond laser. Sci. Rep. 8 (1), 12957. doi:10.1038/s41598-018-31374-w
Cao, L. J., Yang, S. B., Gao, W., Liu, Z., Gong, Y. J., Ma, L. L., et al. (2013). Direct laser-patterned micro-supercapacitors from paintable MoS2 films. Small 9 (17), 2905–2910. doi:10.1002/smll.201203164
Castellanos Gomez, A., Barkelid, M., Goossens, A. M., Calado, V. E., van der Zant, H. S. J., and Steele, G. A. (2012). Laser-thinning of MoS2: On demand generation of a single-layer semiconductor. Nano Lett. 12 (6), 3187–3192. doi:10.1021/nl301164v
Chen, J., Wang, Q. Y., Sheng, Y. C., Cao, G. Q., Yang, P., Shan, Y. B., et al. (2019a). High-performance WSe2 photodetector based on a laser-induced p–n junction. ACS Appl. Mater. Interfaces 11 (46), 43330–43336. doi:10.1021/acsami.9b13948
Chen, Z. R., Nan, H. Y., Liu, Z., Wang, X. Y., Gu, X. F., and Xiao, S. Q. (2019b). Effect of thermal conductivity of substrate on laser-induced phase transition of MoTe2. J. Raman Spectrosc. 50 (5), 755–761. doi:10.1002/jrs.5559
Cho, S., Kim, S., Kim, J. H., Zhao, J., Seok, J., Keum, D. H., et al. (2015). Phase patterning for ohmic homojunction contact in MoTe2. Science 349 (6248), 625–628. doi:10.1126/science.aab3175
Fan, X. B., Xu, P. T., Zhou, D. K., Sun, Y. F., Li, Y. C., Nguyen, M. A. T., et al. (2015). Fast and efficient preparation of exfoliated 2H MoS2 nanosheets by sonication-assisted lithium intercalation and infrared laser-induced 1T to 2H phase reversion. Nano Lett. 15 (9), 5956–5960. doi:10.1021/acs.nanolett.5b02091
Gao, Q. G., Zhang, C. F., Yang, K. Q., Pan, X. J., Zhang, Z., Yang, J. J., et al. (2021). High-performance CVD bilayer MoS2 radio frequency transistors and gigahertz mixers for flexible nanoelectronics. Micromachines 12 (4), 451. doi:10.3390/mi12040451
Gao, Z. W., Liu, M. J., Zheng, W. R., Zhang, X. D., and Lee, L. Y. S. (2019). Surface engineering of MoS2 via laser-induced exfoliation in protic solvents. Small 15 (44), 1903791. doi:10.1002/smll.201903791
Gong, L. L., Zhang, Q., Wang, L. J., Wu, J. F., Han, C., Lei, B., et al. (2018). Emergence of photoluminescence on bulk MoS2 by laser thinning and gold particle decoration. Nano Res. 11 (9), 4574–4586. doi:10.1007/s12274-018-2037-5
Hu, J. J., Zabinski, J. S., Sanders, J. H., Bultman, J. E., and Voevodin, A. A. (2006). Pulsed laser syntheses of layer-structured WS2 nanomaterials in water. J. Phys. Chem. B 110 (18), 8914–8916. doi:10.1021/jp0611471
Hu, Y. S., Zeng, X. B., Ren, T. T., Wu, S. X., Wang, W. Z., Zeng, Y., et al. (2018). Preparation of controllable-thickness 1T@2H-MoS2 thin films by pulsed laser-induced synthesis and the selective separation of the 1T phase. J. Mater. Chem. C 6 (43), 11651–11658. doi:10.1039/C8TC03815E
Hu, Y. S., Zeng, X. B., Ren, T. T., Xiao, Y. H., Zeng, Y., Wang, W. Z., et al. (2020). One-step growth of centimeter-scale doped multilayer MoS2 films by pulsed laser-induced synthesis. J. Mater. Chem. C 8 (20), 6900–6905. doi:10.1039/C9TC06908A
Huo, J. P., Xiao, Y., Sun, T. M., Zou, G. S., Shen, D. Z., Feng, B., et al. (2021). Femtosecond laser irradiation-mediated MoS2–metal contact engineering for high-performance field-effect transistors and photodetectors. ACS Appl. Mater. Interfaces 13 (45), 54246–54257. doi:10.1021/acsami.1c12685
Jung, J., Lee, J., Kim, Y., Bark, H., and Lee, C. (2019). Ultrafast and low-temperature synthesis of patternable MoS2 using laser irradiation. J. Phys. D Appl. Phys. 52 (18), 18LT01. doi:10.1088/1361-6463/ab0724
Kang, S., Won, D., Yang, H., Lin, C. H., Ku, C. S., Chiang, C. Y., et al. (2021). Phase-controllable laser thinning in MoTe2. Appl. Surf. Sci. 563, 150282. doi:10.1016/j.apsusc.2021.150282
Kim, S. J., Choi, K., Lee, B., Kim, Y., and Hong, B. H. (2015). Materials for flexible, stretchable electronics: Graphene and 2D materials. Annu. Rev. Mater. Res. 45 (1), 63–84. doi:10.1146/annurev-matsci-070214-020901
Kim, S., Kim, J. H., Kim, D., Hwang, G., Baik, J., Yang, H., et al. (2017). Post-patterning of an electronic homojunction in atomically thin monoclinic MoTe2. 2D Mater. 4 (2), 024004. doi:10.1088/2053-1583/aa5b0e
Kimiagar, S., and Abrinaei, F. (2023). Laser-assisted hydrothermal synthesis of MoS2 nanosheets under different laser energies and potential application in nonlinear optics. Optik 272, 170305. doi:10.1016/j.ijleo.2022.170305
Lee, Y. H., Zhang, X. Q., Zhang, W., Chang, M. T., Lin, C. T., Chang, K. D., et al. (2012). Synthesis of large-area MoS2 atomic layers with chemical vapor deposition. Adv. Mater. 24 (17), 2320–2325. doi:10.1002/adma.201104798
Lei, S. D., Ge, L. H., Liu, Z., Najmaei, S., Shi, G., You, G., et al. (2013). Synthesis and photoresponse of large GaSe atomic layers. Nano Lett. 13 (6), 2777–2781. doi:10.1021/nl4010089
Leong, W. S. (2020). Versatile strategy for making 2D materials. Nature 577 (7791), 477–478. doi:10.1038/d41586-020-00094-5
Levita, G., and Righi, M. C. (2017). Effects of water intercalation and tribochemistry on MoS2 lubricity: An ab initio molecular dynamics investigation. ChemPhysChem 18 (11), 1475–1480. doi:10.1002/cphc.201601143
Li, J., Yang, X. D., Liu, Y., Huang, B. L., Wu, R. X., Zhang, Z. W., et al. (2020). General synthesis of two-dimensional van der Waals heterostructure arrays. Nature 579 (7799), 368–374. doi:10.1038/s41586-020-2098-y
Li, W. W., Xu, M. Z., Gao, J. W., Zhang, X. S., Huang, H., Zhao, R. Q., et al. (2023). Large-scale ultra-robust MoS2 patterns directly synthesized on polymer substrate for flexible sensing electronics. Adv. Mater. 35 (8), 2207447. doi:10.1002/adma.202207447
Liu, Y., Duan, X. D., Shin, H. J., Park, S., Huang, Y., and Duan, X. F. (2021). Promises and prospects of two-dimensional transistors. Nature 591 (7848), 43–53. doi:10.1038/s41586-021-03339-z
Lu, J. P., Lu, J. H., Liu, H. W., Liu, B., Chan, K. X., Lin, J. D., et al. (2014). Improved photoelectrical properties of MoS2 films after laser micromachining. ACS Nano 8 (6), 6334–6343. doi:10.1021/nn501821z
Ma, H., Shen, Z. G., and Ben, S. (2018). Understanding the exfoliation and dispersion of MoS2 nanosheets in pure water. J. Colloid Interface Sci. 517, 204–212. doi:10.1016/j.jcis.2017.11.013
Mahjouri Samani, M., Tian, M. K., Wang, K., Boulesbaa, A., Rouleau, C. M., Puretzky, A. A., et al. (2014). Digital transfer growth of patterned 2D metal chalcogenides by confined nanoparticle evaporation. ACS Nano 8 (11), 11567–11575. doi:10.1021/nn5048124
Mak, K. F., Lee, C., Hone, J., Shan, J., and Heinz, T. F. (2010). Atomically thin MoS2: A new direct-gap semiconductor. Phys. Rev. Lett. 105 (13), 136805. doi:10.1103/PhysRevLett.105.136805
Mohapatra, P. K., Ranganathan, K., and Ismach, A. (2020). Selective area growth and transfer of high optical quality MoS2 layers. Adv. Mater. Interfaces 7 (24), 2001549. doi:10.1002/admi.202001549
Nagareddy, V. K., Octon, T. J., Townsend, N. J., Russo, S., Craciun, M. F., and Wright, C. D. (2018). Humidity-controlled ultralow power layer-by-layer thinning, nanopatterning and bandgap engineering of MoTe2. Adv. Funct. Mater. 28 (52), 1804434. doi:10.1002/adfm.201804434
Park, J., Kim, M. S., Cha, E., Kim, J., and Choi, W. (2017). Synthesis of uniform single layer WS2 for tunable photoluminescence. Sci. Rep. 7 (1), 16121. doi:10.1038/s41598-017-16251-2
Park, S., Lee, A., Choi, K. H., Hyeong, S. K., Bae, S., Hong, J. M., et al. (2020a). Layer-selective synthesis of MoS2 and WS2 structures under ambient conditions for customized electronics. ACS Nano 14 (7), 8485–8494. doi:10.1021/acsnano.0c02745
Park, S., Park, J., Kim, Y. g., Bae, S., Kim, T. W., Park, K. I., et al. (2020b). Laser-directed synthesis of strain-induced crumpled MoS2 structure for enhanced triboelectrification toward haptic sensors. Nano Energy 78, 105266. doi:10.1016/j.nanoen.2020.105266
Rho, Y., Pei, J., Wang, L., Su, Z., Eliceiri, M., and Grigoropoulos, C. P. (2019). Site-selective atomic layer precision thinning of MoS2 via laser-assisted anisotropic chemical etching. ACS Appl. Mater. Interfaces 11 (42), 39385–39393. doi:10.1021/acsami.9b14306
Schuffenhauer, C., Parkinson, B. A., Jin-Phillipp, N. Y., Joly-Pottuz, L., Martin, J.-M., Popovitz-Biro, R., et al. (2005). Synthesis of fullerene-like tantalum disulfide nanoparticles by a gas-phase reaction and laser ablation. Small 1 (11), 1100–1109. doi:10.1002/smll.200500133
Shim, G. W., Yoo, K., Seo, S. B., Shin, J., Jung, D. Y., Kang, I. S., et al. (2014). Large-area single-layer MoSe2 and its van der Waals heterostructures. ACS Nano 8 (7), 6655–6662. doi:10.1021/nn405685j
Su, B. W., Zhang, X. L., Xin, W., Guo, H. W., Zhang, Y. Z., Liu, Z. B., et al. (2021). Laser-assisted two dimensional material electronic and optoelectronic devices. J. Mater. Chem. C 9 (8), 2599–2619. doi:10.1039/D0TC05540A
Sunamura, K., Page, T. R., Yoshida, K., Yano, T. a., and Hayamizu, Y. (2016). Laser-induced electrochemical thinning of MoS2. J. Mater. Chem. C 4 (15), 3268–3273. doi:10.1039/C5TC04409J
Tran-Khac, B. C., White, R. M., DelRio, F. W., and Chung, K. H. (2019). Layer-by-layer thinning of MoS2 via laser irradiation. Nanotechnology 30 (27), 275302. doi:10.1088/1361-6528/ab11ad
Wang, M. M., Li, D. W., Liu, K., Guo, Q. T., Wang, S. M., and Li, X. (2020). Nonlinear optical imaging, precise layer thinning, and phase engineering in MoTe2 with femtosecond laser. ACS Nano 14 (9), 11169–11177. doi:10.1021/acsnano.0c02649
Wu, R. X., Tao, Q. Y., Li, J., Li, W., Chen, Y., Lu, Z. Y., et al. (2022). Bilayer tungsten diselenide transistors with on-state currents exceeding 1.5 milliamperes per micrometre. Nat. Electron. 5 (8), 497–504. doi:10.1038/s41928-022-00800-3
Xia, J., Huang, X., Liu, L. Z., Wang, M., Wang, L., Huang, B., et al. (2014). CVD synthesis of large-area, highly crystalline MoSe2 atomic layers on diverse substrates and application to photodetectors. Nanoscale 6 (15), 8949–8955. doi:10.1039/C4NR02311K
Xu, M. Z., Gao, J. W., Song, J. C., Wang, H. X., Zheng, L., Wei, Y., et al. (2021a). Programmable patterned MoS2 film by direct laser writing for health-related signals monitoring. iScience 24 (11), 103313. doi:10.1016/j.isci.2021.103313
Xu, M. Z., Tang, B. J., Lu, Y. H., Zhu, C., Lu, Q. B., Zhu, C., et al. (2021b). Machine learning driven synthesis of few-layered WTe2 with geometrical control. J. Am. Chem. Soc. 143 (43), 18103–18113. doi:10.1021/jacs.1c06786
Xu, Y. N., Jiao, B. Z., Wang, Y. C., Xue, S. Y., Gao, H., Yu, K. W., et al. (2022). Rapid in-situ synthesis and patterning of edge-unsaturated MoS2 by femtosecond laser-induced photo-chemical reaction. ACS Appl. Mater. Interfaces 14 (4), 5558–5566. doi:10.1021/acsami.1c20293
Yu, H., Liao, M., Zhao, W., Liu, G., Zhou, X. J., Wei, Z., et al. (2017). Wafer-scale growth and transfer of highly-oriented monolayer MoS2 continuous films. ACS Nano 11 (12), 12001–12007. doi:10.1021/acsnano.7b03819
Yu, J. D., Wang, L., Hao, Z. B., Luo, Y., Sun, C. Z., Wang, J., et al. (2020). Van der Waals epitaxy of III-nitride semiconductors based on 2D materials for flexible applications. Adv. Mater. 32 (15), 1903407. doi:10.1002/adma.201903407
Zhai, X. T., Zhang, R. X., Sheng, H. X., Wang, J., Zhu, Y. M., Lu, Z. C., et al. (2021). Direct observation of the light-induced exfoliation of molybdenum disulfide sheets in water medium. ACS Nano 15 (3), 5661–5670. doi:10.1021/acsnano.1c00838
Zheng, L., Wang, X. W., Jiang, H. J., Xu, M. Z., Huang, W., and Liu, Z. (2022). Recent progress of flexible electronics by 2D transition metal dichalcogenides. Nano Res. 15 (3), 2413–2432. doi:10.1007/s12274-021-3779-z
Keywords: laser, two-dimensional materials, transition metal dichalcogenides, synthesis methods, mechanism
Citation: Wang H, Xu M, Ji H, He T, Li W, Zheng L and Wang X (2023) Laser-assisted synthesis of two-dimensional transition metal dichalcogenides: a mini review. Front. Chem. 11:1195640. doi: 10.3389/fchem.2023.1195640
Received: 28 March 2023; Accepted: 10 April 2023;
Published: 25 April 2023.
Edited by:
Tao Wei, University of Erlangen Nuremberg, GermanyReviewed by:
Muqing Chen, University of Science and Technology of China, ChinaCopyright © 2023 Wang, Xu, Ji, He, Li, Zheng and Wang. This is an open-access article distributed under the terms of the Creative Commons Attribution License (CC BY). The use, distribution or reproduction in other forums is permitted, provided the original author(s) and the copyright owner(s) are credited and that the original publication in this journal is cited, in accordance with accepted academic practice. No use, distribution or reproduction is permitted which does not comply with these terms.
*Correspondence: Xuewen Wang, aWFteHd3YW5nQG53cHUuZWR1LmNu
†These authors have contributed equally to this work