- Key Laboratory of Functional Molecular Engineering of Guangdong Province, School of Chemistry and Chemical Engineering, South China University of Technology, Guangzhou, China
In comparison with all-carbon parent compounds, the incorporation of Si-element into carboskeletons generally endows the corresponding sila-analogues with unique biological activity and physical-chemical properties. Silacycles have recently shown promising application potential in biological chemistry, pharmaceuticals industry, and material chemistry. Therefore, the development of efficient methodology to assemble versatile silacycles has aroused increasing concerns in the past decades. In this review, recent advances in the synthesis of silacycle-system are briefly summarized, including transition metal-catalytic and photocatalytic strategies by employing arylsilanes, alkylsilane, vinylsilane, hydrosilanes, and alkynylsilanes, etc. as starting materials. Moreover, a clear presentation and understanding of the mechanistic aspects and features of these developed reaction methodologies have been high-lighted.
1 Introduction
Nonmetallic Si-element which constitutes almost 30% of the mass of earth´s crust in silica and silicates exists extensively in our planet. As is well-known that aza-, oxa-, or thia-organic molecules are commonly encountered in many natural products and pharmaceuticals, but sila-organic molecules are not readily available in nature. Compared with all-carbon organic compounds, sila-analogues generally possess unique bioactive and photophysical properties (Förster et al., 2014; Pujals et al., 2006; Lippert et al., 2009) due to that silicon element has larger covalent radius (rSi vs. rC: 111 p.m. vs 67 p.m.) and less electronegativity (χSi vs. χC: 1.74 ev vs. 2.50 ev) different from carbon atom (Allred and Rochow, 1958). For instance, some representative examples of therapeutically potential silacycles are described in Figure 1. However, incorporating Si-element into all-carbon skeletons and heterocyclic systems has not been well-established. The main reason is that extra 3d orbitals of silicon element easily interact with heteroatoms and metal ions to form hypervalent five- or six-coordinated intermediates (Breit et al., 2013; Shen et al., 2017), leading to decomposition of organosilicanes (Akiyama and Imazeki, 1997; Fleming and Winter 1998). Meanwhile, transmetalation between sila-compounds and metal catalysts can often occur to make the silane-based chemical reactions show poor chemoselectivity (Chen et al., 2021; Pawley et al., 2022). Therefore, the development of versatile strategies to access various organosilanes has recently aroused increasing concerns. To date, the scope, mechanism, and applications for intermolecular silylations of alkanes, alkenes, arenes, diazo-compounds, and alkynes, etc., have been widely explored, and the corresponding studies involved transition metal-catalysis and photocatalysis have also been summarized by different research groups (Cheng and Hartwig, 2015; Li et al., 2015; Xu et al., 2015; Van Hoveln et al., 2019; Keipour et al., 2017; Chatgilialoglu et al., 2018; Huang W S et al., 2022). However, no comprehensive review about synthetical strategies of silacycles through C-C bond and C-heteroatom bond formation reactions has been published. This review will focus on the assembly of unique structural silacyclical skeletons through intermolecular and intramolecular coupling-cyclization of alkylsilanes, arylsilanes, alkynylsilanes, vinylsilanes, and hydrosilanes, etc., with different coupling-reagents.
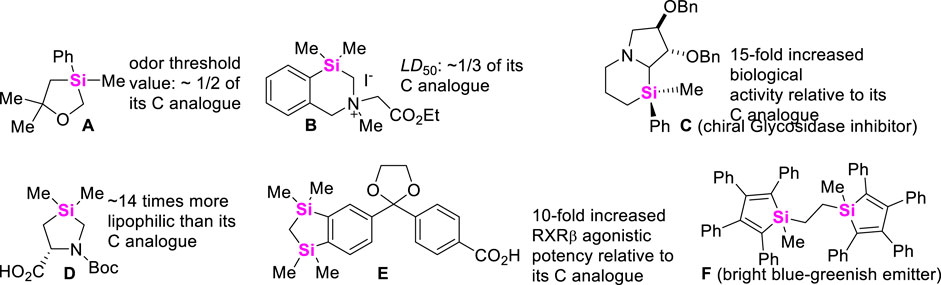
FIGURE 1. The difference in of biological activity and photophysical properties between the representative silacycles and their all-carbon analogues.
2 Synthetic strategy of silacycles via C-Si σ bond functionalization
C-Si σ bond is commonly encountered in many organic sila-molecules. Therefore, the development of C-Si bond cleavage-based transformation will provide a novel platform to assemble silacycles with high step-economy. Generally, C-Si bonds include strained C-Si σ bonds and unstrained C-Si σ bonds. The cleavage of C-Si bonds catalyzed by transition metal is still in its infancy because of the unmet challenges on reactivity, selectivity, and substrate scope.
2.1 Coupling-cyclization by catalytic cleavage of the strained C-Si σ bond activation
The scope of strained C-Si bond cleavage is primarily restricted to the silacycles with a small ring size such as silacyclobutanes (SCBs), in which strain release can provide thermodynamic driving forces. In these regards, Oshima and Yorimitsu early reported a Pd-catalyzed intermolecular cycloaddition of SCBs with enones, in which coupling-cyclization of SCBs with enones under Pd-catalysis conditions could smoothly proceed via formal cycloaddition to yield the corresponding eight-membered ring (Hirano et al., 2008). The reaction was first triggered by the initial oxidative addition (O. A.) of SCBs to Pd (0) catalysts, producing palladasilacyclopentane which was then trapped by a, β-unsaturated ketones (Scheme 1A).
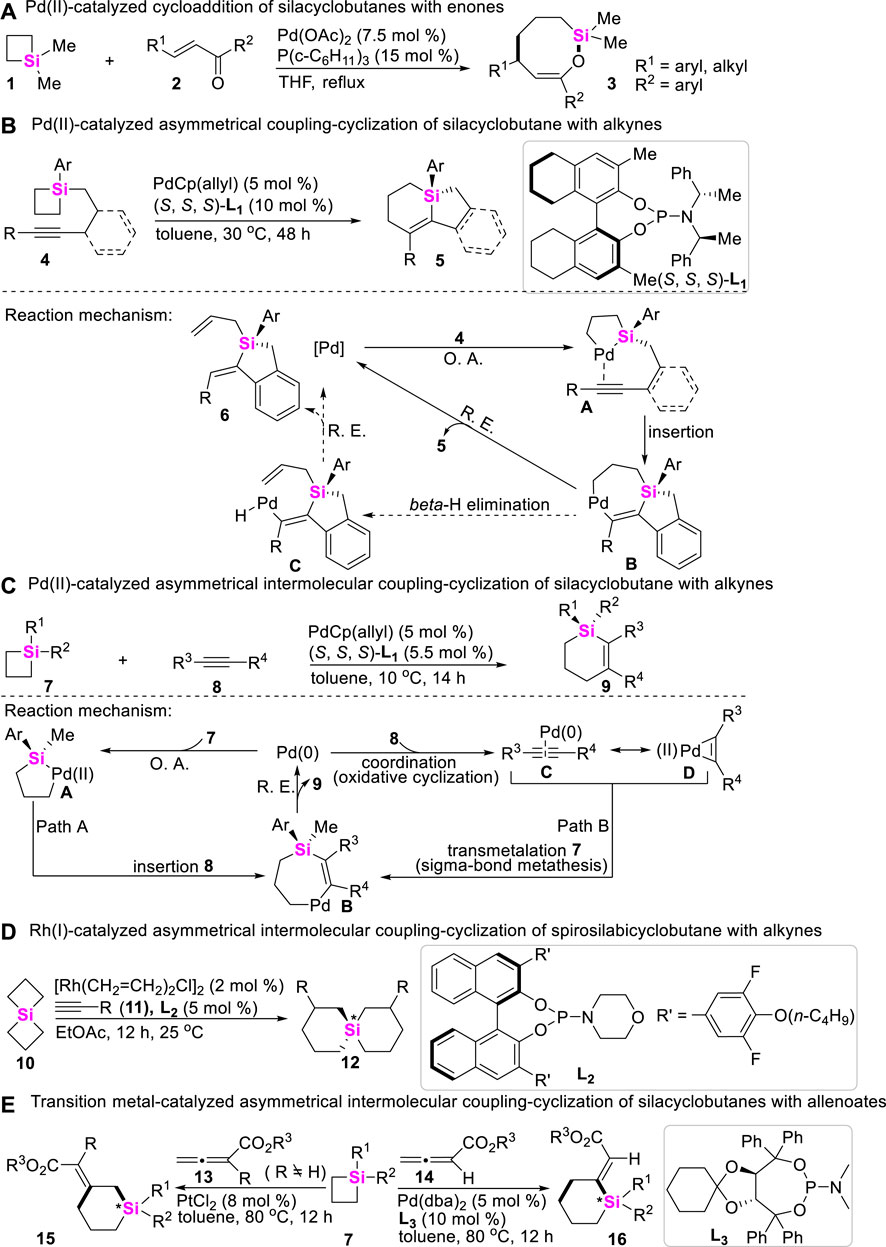
SCHEME 1. Transition metal-catalyzed coupling-cyclization of silacyclobutanes with alkenes, alkynes and allenoates.
Possibly encouraged by Oshima’s work (Hirano et al., 2008), in 2011, Hayashi and Shintani developed a Pd-catalyzed enantioselective desymmetrization of SCBs through an intramolecular coupling-cyclization, in which alkyne-tethered SCBs 4 were used as starting material, and 5,5′,6,6′,7,7′,8,8′-octahydro-1,10-binaphthyl phosphoramidite (L1) was utilized as a chiral ligand (Shintani et al., 2011). This transformation provided an efficient approach to access chiral silacycles 5 featuring tetraorganosilicon stereocenter (Scheme 1B). The overall process for this transformation starts with the oxidative addition of a C-Si bond of silacyclobutane from 4 to Pd (0) catalysts, giving 1-pallada-2-silacyclopentanes A. Intermediates A then undergo an intramolecular insertion of the alkyne to form 1-pallada-4-sila-2-cycloheptenes B, followed by the subsequent reductive elimination (R. E.) to produce compounds 5 along with regeneration of Pd (0)-catalysts. Meanwhile, β-H elimination from intermediate B gives alkenylpalladium hydride species C, and successive reductive elimination results in the formation of byproducts 6.
Subsequently, Hayashi and Shintani further developed a Pd-catalyzed intermolecular desymmetrization of SCBs with alkynes almost using the same reaction conditions (Shintani et al., 2012), assembling Si-stereogenic 1-sila-2-cyclohexenes. The reaction mechanism possibly proceeds through Path A, in which oxidative addition of a C-Si σ bond of SCBs 7 to Pd (0) gives 1-pallada-2-silacyclopentane A. A species then undergo insertion of alkynes 8 to produce 1-pallada-4-sila-2-cycloheptenes B, the reductive elimination of B leads to the formation of products 9 along with regeneration of Pd (0)-catalysts. Alternatively, coordination of 8 to Pd (0) could precede cleavage of the C-Si σ bond of 7 as shown in Path B. Subsequent transmetalation (or σ-bond metathesis) of 7 can provide the same intermediates B, which eventually give silacycles 9 and Pd (0)-catalysts by reductive elimination (Scheme 1C). Of course, the detailed experiments and DFT calculation about this transformation were further performed by Xu, confirming that Path B (Scheme 1C) is reasonable (Zhang Q W et al., 2016). In 2021, the similar transformation of Rh/Cu-catalyzed coupling-cyclization of SCBs with arylpropiolate-type internal alkynes was also achieved by Xu (Wang X C et al., 2021). In 2022, Song developed a Rh (I)-catalyzed intermolecular asymmetric coupling-cyclization of siprosilacyclobutanes 10 with terminal alkynes 11 to produce spirosilabicyclohexenes 12 with up to 96% ee (Scheme 1D) (Chen et al., 2022). Meanwhile, Song still utilized allenoates 13 and 14 as coupling reagents to react with silacyclobutanes 7 to furnish 2- or 3-(E)-enoate-substituted silacyclohexenes 15 and 16 (up to 80% ee) in the presence of chiral phosphoramidite L3 (Scheme 1E) (Tang et al., 2022).
Besides that a, β-unsaturated ketones and alkynes could be employed to couple with SCBs via C-Si σ bond cleavage, cycloketones, cyclopropenones, arenes, and trisylhydrazones were also demonstrated to be efficient coupling-partners. In this vein, Murakami successively developed Pd-catalyzed intramolecular and intermolecular coupling-strategies of cyclobutanones with SCBs to construct complex structural benzosilacycles through σ-bond exchange process. For the intramolecular coupling-cyclization of cyclobutanone-containing SCBs 17 (Ishida et al., 2014), the key step is involved the oxidative addition of Pd (0) onto Si-C σ bond of silacyclobutane moiety to generate silapalladacycles A (Scheme 2A). On the contrary, for the intermolecular coupling-cyclization of cyclobutanones 19 with SCBs 7 (Okumura et al., 2017), the control experiments confirmed that the oxidative addition of Pd (0) onto C-C bond of cycloketone moiety to generate palladacycle (II) intermediates (A) prefer to occur (Scheme 2B). Based on the similar reaction mechanism, Xu and co-workers developed a Pd (II)-catalyzed [4 + 2] annulation of cyclopropenes with benzosilacyclobutanes to rapidly assemble silabicyclo [4.1.0] heptanes 23 and 25 in which cyclopropenes include gem-difluorocyclopropenes 24 (Scheme 2C) (Wang W et al., 2021; Xu et al., 2022). Meanwhile, Zhao still found Pd (II)- or Ni (II)-catalytical system could rapidly enable the intermolecular coupling-cyclization of silacyclobutanes 26 with cyclopropenones 27 (Scheme 2D) and aryl halides 29, 31, and 33 (Schemes 2E–G), furnishing silacycles 28, 30, 32, and 34 (Zhao et al., 2018a; Qin et al., 2020; Qin et al., 2021; Wang X et al., 2021).
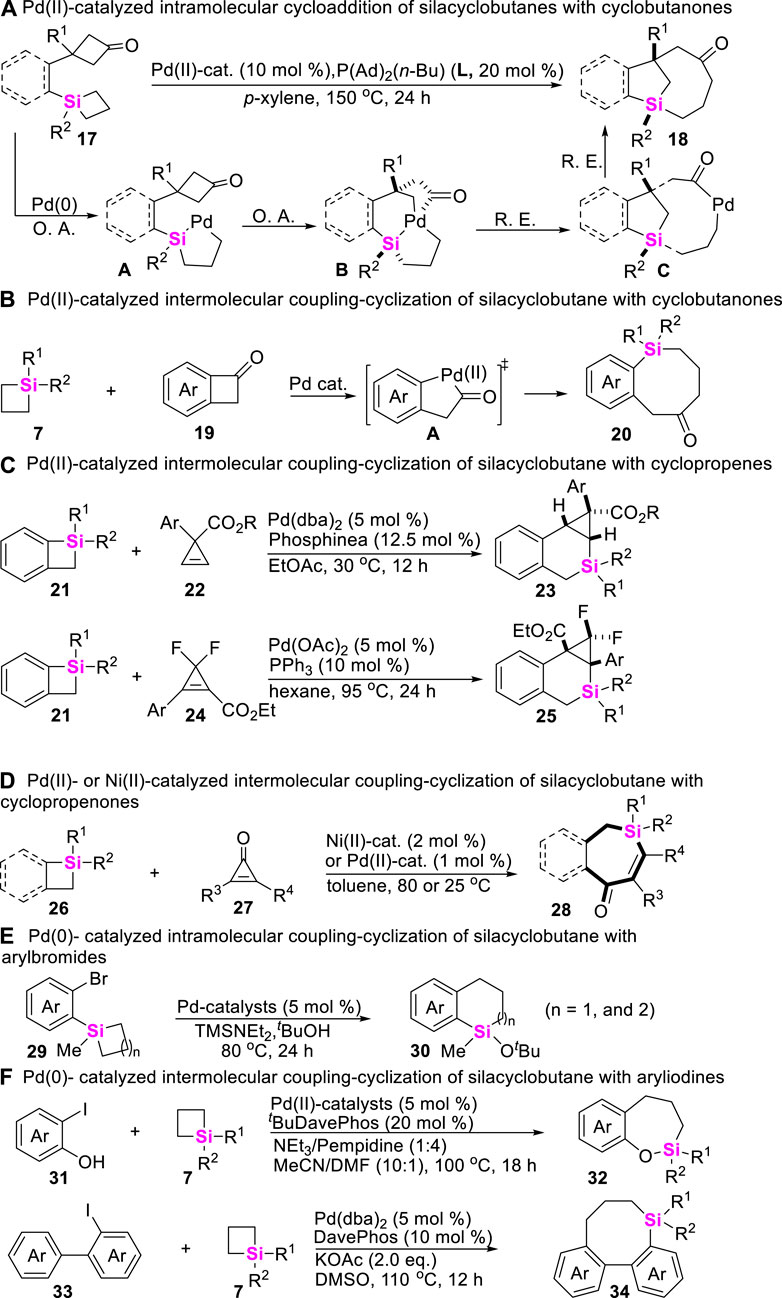
SCHEME 2. Transition metal-catalyzed coupling-cyclization of silacyclobutanes with cycloketones, cyclopropenes, and arylhalides.
Meanwhile, the more challenging Rh-catalyzed intramolecular coupling-cyclization of SCBs with Csp2-H bonds has recently been achieved to make π-conjugated siloles with good regioselectivities by He (Zhang J et al., 2016). As shown in Scheme 3A, this transformation undergoes sequential C-Si bond and aryl Csp2-H bond activation process, and the catalytic cycle involves a rarely endocyclic β-hydride elimination of five-membered metallacycles A, which after reductive elimination gave rise to a Si-RhI species B that is capable of C-H activation. Apart from the Rh-catalysts, Zhao further developed a Ni (0)-catalyzed asymmetric intramolecular coupling-cyclization of alkene and SCBs to make enantioenriched silicon-stereogenic benzosiloles by utilizing ortho-vinylaryl silacyclobutanes 37 as substrates (Zhang et al., 2021). Two distinct pathways of this transformation are proposed. Path A begins with the coordination of the nickel (0) catalyst with alkene moiety to generate η2–coordinated complex A or B, then followed by an alkoxide-promoted transmetalation, β-hydride elimination, and reductive elimination to produce the desired benzosilacycles 38. In the contrast, Path B involves the oxidative addition of C-Si bond on SCB and sequential intramolecular insertion of an alkene moiety (Scheme 3B).
More recently, Wang reported a highly effcient Pd (II)-catalyzed carbene insertion into C-Si bonds of SCBs 7 to deliver silacyclopentanes 40 with excellent enantioselectivity by using trisylhydrazones 39 as carbenoid precursors (Huo et al., 2021). This reaction features with wide substrate scope and high tolerance of functional groups. Mechanistic studies including DFT calculations suggest a catalytic cycle involving oxidative addition of Pd to strained C-Si bonds, carbenoid migratory insertion, and reductive elimination (Scheme 4). Moreover, the roles of the chiral ligand L5 in controlling the reaction enantioselectivity are also elucidated.
2.2 Coupling-cyclization by catalytic cleavage of the unstrained Si-C σ bond activation
In comparison with the strained C-Si bonds, unstrained C-Si σ bonds possess higher thermodynamic stability. Therefore, the inert C-Si bond cleavage generally requires the use of stoichiometric amounts of either organomagnesium (van Klink et al., 2002) or organolithium reagents (Yu et al., 2008) and harsh reaction conditions. Nevertheless, transition metal-catalyzed C-Si σ bond activation provides an alternative mild approach to enable C-Si σ bond cleavage. In these regards, Chatani first reported an Rh (I)-catalyzed benzosilole synthesis in 2009 through the coupling-cyclization of 2-silylphenylboronic acids with alkynes (Tobisu et al., 2009). The corresponding mechanism starts from the formation of arylrhodium intermediates A, generated by the transmetalation of arylboronic acids 41 to rhodium hydroxide Rh (I)OH, and adds across alkynes 8 to form the vinylrhodium intermediates B, which subsequently undergo oxidative addition at a trimethylsilyl group to afford intermediates C, producing benzosilole products 42 and methyl-rhodium D through reductive elimination. Meanwhile, protonolysis of D regenerates the catalytically active Rh (I)OH (Scheme 5A). By using the similar strategy, Xi, He, Ogoshi, and Zhao successively developed Pd-, Rh-, and Ni-catalyzed coupling-cyclization of unstrained C-Si σ bonds with alkynes (Schemes 5B, 5C), aldehydes (Scheme 5D), and alkenes (Scheme 5E), providing various synthetic methods to construct complex structural benzosiloles 45 (Liang et al., 2012) and 47 (Zhang et al., 2014), benzoxasiloles 49 (Hoshimoto et al., 2014), and spiro-silacycles 51 (Shi et al., 2022).
3 Synthetic strategy of silacycles via Si-H σ bond functionalization
Hydrosilanes belong to readily accessible organosilanes which possess versatile reactivity. So, the cross-coupling of hydrosilanes with alkanes, arenes, alkenes, and alkynes could provide an alternative strategy to form C-Si bonds. Among them, transition metal-catalyzed C-H/Si-H coupling and hydrosilylation of unsaturated hydrocarbons represent two main chemical transformations to assemble silacycles. Meanwhile, hydrosilanes including dihydrosilanes and monohydrosilanes are also the most common precursors of chiral tetraorganosilicons, and the development of synthetic methodology for chiral silacycles which are derived from hydrosilanes, has become more challenging.
3.1 Intramolecular coupling-cyclization of Si-H bonds with alkanes
As is well-known, Csp3-H bond functionalization is one of the most useful and versatile strategies for constructing organic molecules. In comparison with Csp-H bond and Csp2-H bond, the reactivity of alkyl Csp3-H bond is inerter. Therefore, examples of Csp3-H/Si-H coupling reactions are very rare. The pioneer studies on transition metal-catalyzed Csp3-H/Si-H bond coupling reaction were mainly performed by Hartwig, but most of these transformations suffered from high reaction temperatures (135°C ∼ 200 C) (Tsukada and Hartwig, 2005). In 2010, Takai and co-workers reported Rh (I)-catalyzed Csp2-H/Si-H coupling reaction to produce the mixture of silafluorene 53 and dibenzo [b, d]saline 54 by using ortho-arylphenylsilanes including 33 as starting materials (Scheme 6A) (Ureshino et al., 2010). Subsequently, they further found that increasing the reaction temperature could chemoselectively enable Csp3-H/Si-H coupling under the same catalytical system, giving dibenzo [b, d]silane 56 as a sole intermolecular coupling-cyclization product by using ortho-alkylphenylsilanes as starting materials (Kuninobu et al., 2013a). The proposed mechanism was involved in the oxidative addition of the Si-H bond to Rh (I)-catalysts, then undergoing Si-H bond activation and σ-bond metathesis to form cyclorhodiumates B and C, respectively. Subsequently, reductive elimination of intermediate B or C produces benzosilacycle 56 and regenerates the Rh (I)-catalyst (Scheme 6B). To our satisfaction, Takai continued to optimize these reaction parameters by utilizing phosphorus ligand and 3,3-dimethyl-1-butene as additional additives, significantly lowering the reaction temperatures from 180°C to 50 C (Murai et al., 2015).
Possibly encouraged by Takai’s work, Huang further explored the synthetic approach to access 1,3-sila-heterocycles through methoxyl or aminomethyl Csp3-H/Si-H coupling of arylalkylhydrosilanes 57 (Fang et al., 2017), and found that PCP Pincer-Ru(II)- and PCP Pincer-Ir (III)-catalyzed intramolecular cyclization could rapidly assemble 1,3-sila-heterocycles 58 via intermolecular σ-bond metathesis process (Scheme 6C). As for the more challenging Csp3-H/Si-H coupling of trialkylhydrosilanes 59, Gevorgyan developed an Ir(I)-catalyzed and pyridine-chelation-assisted Csp3-H silylation strategy of an unactivated C (sp3)–H bonds to produce silolanes 60 with good to excellent yields (Ghavtadze et al., 2014), in which different linear alkyl chains were well-tolerated in this reaction conditions (Scheme 6D).
Although chiral Si-atoms are not naturally occurring, these organosilanes show great application potential, especially in the field of life sciences and material chemistry. To date, the approach to access chiral sila-compounds through Csp3-H/Si-H coupling reaction is very limited. Albeit Takai ever achieved an asymmetric Csp3-H silylation toward silicon-stereogenic center, the corresponding enantiomeric excess (ee) values of spirosilabiindanes did not exceed 40% (Murai et al., 2015). Recently, He utilized Rh (I)/chiral Josiphos-catalytic system, successively realized intramolecular asymmetric Csp3-H/Si-H coupling-reaction of dihydrosilanes, constructing silicon-stereogenic dihydrobenzosiloles (Scheme 7A) (Yang et al., 2020) and dihydrodibenzosilines (Scheme 7B) (Guo et al., 2021) with excellent enantioselectivity (up to 97% ee values).
3.2 Intramolecular coupling-cyclization of Si-H bonds with arenes
Silicon-containing π-conjugated molecules can be utilized in the areas of electro- and photo-luminescence, the intramolecular coupling-cyclization of aryl Csp2-H bonds with Si-H bonds has therefore been widely explored to furnish silaarenes. Usually, the present synthetic methods to access benzosilacycles are mainly focused on the set-up of different reaction systems, including arylsilanes and catalysts. The reaction mechanism was generally involved in the oxidative addition of low-valent metal ions including Rh (I) and Ir (I) to Si-H bond of hydrosilanes 66, followed by the sequential aryl Csp2-H bond activation and reductive elimination process to afford diverse silaarenes 67 (Scheme 8A). The earlier study about transition metal-catalyzed aryl Csp2-H/Si-H coupling-reaction was reported by Takai in 2010 (Ureshino et al., 2010). Takai and co-workers utilized RhCl(PPh3)3 as catalysts, and employed biarylmonohydrosilanes as substrates to rapidly assemble silafluorenes through Rh (I)-catalyzed double activation of Si-H and C-H bonds with dehydrogenation. Since then, Hartwig (Li et al., 2014), Shi (Su et al., 2017), Zhao (Zhao et al., 2018b), and Xu (Lin et al., 2017) also realized Ir (I)-catalyzed intramolecular dehydrogenation-coupling of aryl Csp2-H bonds with monohydrosilanes 68, 70, and 72, producing azo-silacycles 69 (Scheme 8B), oxa-silacycles 71 (Scheme 8C) and cyclic disiloxanes 73 (Scheme 8D), respectively.
Of course, Takai still found Rh (I)-salts possess excellent catalytical activity to allow for dehydrogenation-coupling between aryl Csp2-H bonds and dihydrosilanes. For example, in 2013, Takai and coworkers developed an intramolecular asymmetric coupling-cyclization of bis (biphenyl)bihydrosilanes 74 in the presence of {[RhCl(cod)]2}] and chiral (R)-binap (Kuninobu et al., 2013b), providing spirosilabifluorenes 75 in 73%–95% yields (Scheme 9A). Encouraged by this work, W. He (Scheme 9B) and C. He (Scheme 9C) further realized the construction of stereogenic silicon benzosilacycles by using aryldihydrosilanes 76 and vinyldihydrosilanes 78 as starting material in the presence of Rh (I)-catalysts and chiral diphosphine ligands, excellent enantioselectivities of chiral benzosilacompounds 77 and 79 were up to >99% ee (Ma et al., 2021; Yuan et al., 2021).
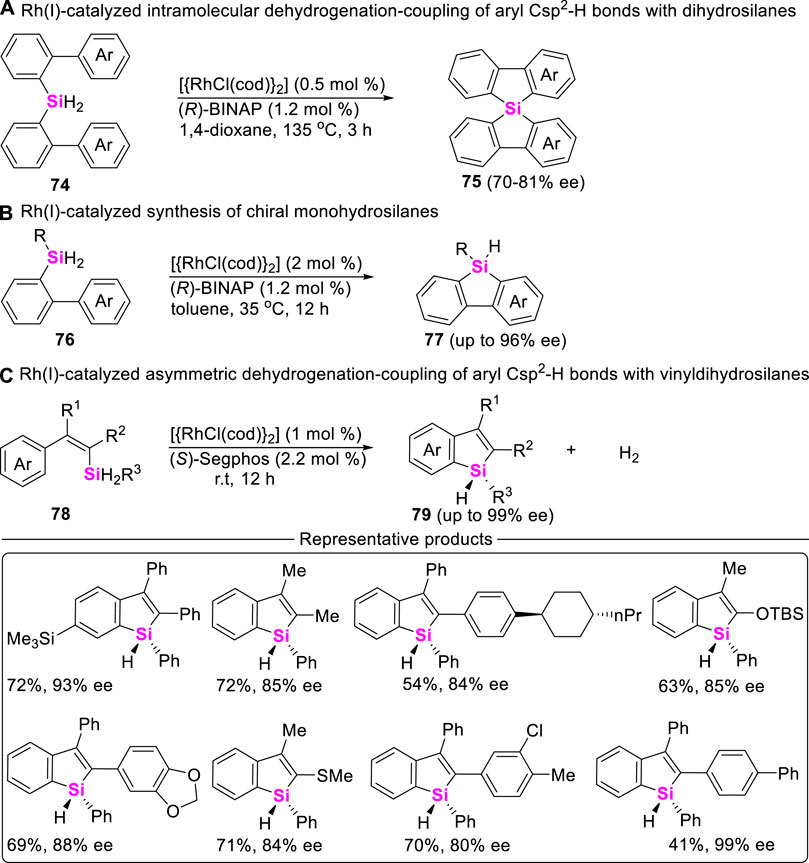
SCHEME 9. Rh-catalyzed intramolecular asymmetric dehydrogenation-coupling of aryl Csp2-H bonds with dihydrosilanes.
Besides that transition metal Rh (I)- and Ir (I)-catalysts could efficiently enhance the cross-coupling of aryl Csp3-H bonds with hydrosilanes, Studer and Li also found that oxidants-promoted aryl Csp2-H bond/Si-H coupling could easily occur to deliver benzosilacycles 80 in good reaction conversions (Scheme 10). This transformation is generally involved in the homolysis of oxidants such as DTBP and TBHP to produce alkoxyl radicals, which then abstracted the H atom from monohydrosilanes to form Si-centered radicals. Finally, the radical-cyclization between Si-centered radicals and arenes gave 9-silafluorene skeletons (Leifert and Studer, 2015; Xu et al., 2015).
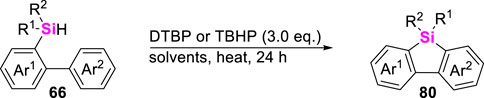
SCHEME 10. Oxidant-promoted intramolecular dehydrogenation-coupling of aryl Csp2-H bonds with hydrosilanes.
3.3 Intramolecular coupling-cyclization of Si-H bonds with alkenes and alkynes
Despite the rich history of the synthesis of silacycles, the method of intramolecular coupling-cyclization of hydrosilanes with alkenes or alkynes has been significantly lacking. To date, only Nakamura in 2008 reported a Me3SnLi-promoted intramolecular coupling-cyclization of hydrosilanes with alkynes to produce benzosiloles (Ilies et al., 2008), in which nucleophilic addition of Et3SnLi to alkynes was involved (Scheme 11A). Subsequently, Nakamura further found that strong base KH could also promote the same transformation by using (2-alkynylphenyl)monohydrosilanes as substrates (Scheme 11A) (Ilies et al., 2009). By utilizing the similar substrates, Xu realized a Rh-catalyzed dynamic kinetic asymmetric intramolecular hydrosilylation with “silicon-centered” racemic hydrosilanes in the presence of chiral ligand SiMOS-Phos, providing silicon-stereogenic benzosiloles in excellent ee values (Scheme 11B) (Tang et al., 2020; Zeng et al., 2022). By the way, the Pt (0)-catalyzed intermolecular hydrosilylation of OH-containing acetylenes 89 with dihydrosilanes 90 was also achieved in 2018 by Xu to allow for assembling silyloxycycles and cyclic siloxanes 91 (Scheme 11C) (Long et al., 2018). In 2020, the transition metal-catalysis strategy has been gradually developed by Wang to assemble benzosilacycles through intramolecular coupling-cyclization of monohydrosilanes with alkenes. The intramolecular coupling-cyclization of bis-(alkenyl)dihydrosilanes (Chang et al., 2020) and mono-(alkene)dihydrosilanes (Huang Y H et al., 2022) could smoothly proceed in the presence of Rh (I)-catalysts and chiral ligands, producing spirosilabiindanes (Scheme 11D) and monohydro-benzosilacycles (Scheme 11E) with good to excellent ee values, respectively.
4 Synthetic strategy of silacycles via coupling-cyclization of vinylsilanes
Although vinylsilanes have been widely used in the Hiyama cross-coupling reaction to make C-C bonds with the release of the silyl group, transition metal-catalyzed Mizoroki-Heck reaction of vinylsilanes with aryl halides can form new C-C bonds at the β-position of vinylsilane and keep silyl moiety untouched. Therefore, the development of intramolecular Mizoroki-Heck coupling-cyclization of vinylsilanes may provide an efficient approach to access silacycles. Unfortunately, the examples involving vinylsilane which participated in the Mizoroki-Heck reaction are rarely reported. To date, only Xi and Teen successively reported Pd-catalyzed intramolecular coupling-cyclization of vinylsilanes with aryl Csp2-X bonds (X = Br and I) to assemble benzosilacycles (Scheme 12A) (Teng and Keese, 1999; Ouyang et al., 2012).
Aryl migration via Smiles rearrangement is a powerful tool for the synthesis of polycyclic arenes. However, the modes of radical Smiles rearrangement are very limited. More recently, Zeng reported a novel photo-catalyzed cycloaromatization of ortho-alkynylaryl vinylsilanes 99 with arylsulfonyl azides 100 for delivering naphthyl-fused benzosiloles 101, various ortho-alkynylaryl vinylsilanes including ortho-alkynylaryl allylsilanes and arylsulfonyl azides are well-allowed for this reaction system (Chen et al., 2021). The corresponding reaction mechanism features a unique combination of cascade S-N/C-S bond cleavages and α-silyl radical Smiles rearrangement, in which silyl hyperconjugation effect (the so-called β-effect) plays a key role in controlling the regioselective coupling-cyclization (Scheme 12B). Post-synthetic applications indicate that these silaarenes show promising potential in luminescent materials.
5 Synthetic strategy of silacycles via coupling-cyclization of alkynylsilanes
Alkynylsilanes belong to versatile synthons, possessing characteristic reactivity. Therefore, the development of alkynylsilane-based coupling-cyclization has aroused increasing concerns in the assembly of silacycles. In these regards, Nozaki utilized silicon-containing diynes 102 as substrates, explored the reactivity of Rh-catalyzed intramolecular alkynylsilylation of alkynes to produce enyne-functionalized dibenzosilacycles 103 (Shintani et al., 2015), this transformation was involved in sequential oxidative-addition, syn-insertion and reductive elimination process (Scheme 13A). Subsequently, Nozaki continued to design and synthesize prochiral triynes 104 and developed an Rh-catalyzed asymmetric [2 + 2 + 2] cycloaddition of silicon-containing prochiral triynes with isocyanates to afford silicon-stereogenic silicon-bridged arylpyridinones 105 (Shintani et al., 2016), high yields and enantioselectivities have been achieved by employing an axially chiral monophosphine ligand (Scheme 13B). Meanwhile, transition metal-catalyzed intramolecular coupling-cyclization of alkynylsilanes with aryl Csp2-X bonds (X = Br, I, etc.,) could also provide an alternated approach to access silacyclies. Possibly encouraged by the research work from Teen (Teng and Keese, 1999) and Ouyang (Ouyang et al., 2012) groups, Donnard utilized ortho-alkynylsilylalkyl aryl halides as substrates, realized the Pd (II)-catalyzed of coupling-cyclization of alkynylsilanes 96 with aryl boronic acids (Scheme 13C), rapidly assembling vinylation benzosiloles 106 (Wagner et al., 2017). More recently, Zeng developed an efficient Pd/Rh- cooperatively catalyzed arylalkynylation of ortho-alkynylsilyalkylaryl halides 107 with a-alkynylalcohols 108, merging an alkynylidene moiety into benzosilacycle 109 (Chen et al., 2021). The corresponding mechanistic investigations demonstrated that the relay trimetallic transmetalation played a pivotal role in governing this transformation (Scheme 13D).
On the other hand, alkynylsilanes can also undergo 1,2-silicon migration under transition metal-catalytic systems (Kanno et al., 2016). Employing these reaction characteristics, Tanaka reported an Rh(I)/biphep complex catalyzeed cycloisomerization of 2-(alkynylsilyl-ethynyl)phenols 110, leading to the formation of alkynylmethylidene-benzoxasiloles 111 through concomitant silicon and carbon migration (Namba et al., 2017). This novel cycloisomerization possibly proceeds via the formation of Rh-vinylidenes through 1,2-silicon migration, followed by 1,3-carbon (alkyne) migration via the formation of hypervalent silicon centers (Scheme 13E).
6 Synthetic strategies of silacycles via Si-Si σ bond activation of disilanes
The relatively weak Si-Si σ bond (ca. 54 kcal/mol) (Sanderson, 1983) suggests that disilanes could be converted into Si-Mn + 2-Si species via low-valent metal-based oxidative addition. Thus, the addition of intermetallic Si-Mn + 2-Si σ-bonds to alkynes can provide an efficient route for the preparation of silacycles. In these regards, the first example was evidenced by Sakurai et al., 1975, that a strained cyclic disilane 112 can react with activated alkynes 8 in the presence of Pd(II)-catalysts to provide disilyla-cycloheptenes 113 (Scheme 14A). Encouraged by this pioneering work, different disilanes were successively designed and synthesized to react with alkynyl moieties via intramolecular coupling-cyclization. For examples, Ito and Matsuda reported Pd-catalyzed intramolecular syn bis-silylation of alkyl/alkyl internal alkynes (Ito et al., 1991; Ahmad et al., 2017), providing straightforward access to a large set of syn-disilylated olefins 115 and 117 (Scheme 14B). In 2012, Matsuda and co-workers (Matsuda and Ichioka, 2012) utilized Rh (I)-catalysts to enable an intramolecular bis-silylation of aryl/aryl internal alkynes 118 into silylbenzosilanes 119 (Scheme 14C). Of course, apart from the coupling-cyclization of disilanes with alkynes, Pd-catalyzed intramolecular σ-bond metathesis between disilanes with cyclobutanones was also investigated by Murakami (Ishida et al., 2012) to furnish an acylsilane-tethered silaindane skeletons 121 (Scheme 14D).
7 Synthetic strategy of silacycles via cross-coupling of 1,3-dienes with dichrolosilanes and intramolecular ring-closing methathesis of diallylsilanes
Silacyclopentenes belong to versatile precursors of silacycles. In 2001, Kozmin reported an Mg-mediated cyclosilylation of butadiene 122 with dichrolodiphenylsilane 123 to produce silacyclopentene 124 (Scheme 15A) (Liu and Kozmin, 2001). Recently, Tomooka and Igawa developed an alternative approach to access silacyclopentenes 124 through successive diallylation of dichrolosilanes 123 and ring-closing methathesis of diallylsilanes 125 (Scheme 15B). These silacyclopentenes 124 could be further oxidized to furnish epoxide 126 by 3-chloro-peroxybenzoic acid (MCPBA), followed by post-modification to afford chiral silacyclopentenols 127 and other multi-functrionalized silacycles (Scheme 15C), featuring with interesting biological activity (Igawa et al., 2016; Igawa et al., 2017). By the way, it should be noted that dichrolosilanes could also easily react with 1,5-dialcohols 128 under base conditions to produce dioxasilanes 129 (Scheme 15D) (Bai et al., 2017).
8 Synthetic strategy of silacycles via cross-coupling of halomethylsilanes with unsaturated hydrocarbons
To date, besides that these organosilicon compounds including silacyclobutanes, aryl/alkylsilanes, hydrosilanes, halosilanes, alkynylsilanes, vinylsilanes, allylsilanes and disilanes which have been successfully employed to couple with different coupling-reagents to make various silacycle skeletons, other novel silyl sources have also aroused wide concerns. In this regard, Gevorgyan designed and made halomethylsilyl ether-tethered alkenes 130, and found that these halomethylsilanes can undergo intramolecular coupling-cyclization under Pd (II)-catalysis system to afford allylic silyloxycycles 131 via Heck reaction (Scheme 16A) (Parasram et al., 2014). Two years later, Song developed photo-catalyzed intramolecular coupling-cyclization of iodomethylsilanes with alkynes to produce 5-exo-cyclization products (Lin et al., 2016), in which alkyl-substituted internal alkynes lead to Z-benzosilolines 132, aryl-substituted internal alkynes result in E-benzosiloline 134 (Scheme 16B). More recently, Song still further found that 3-silaazetidines can be easily prepared in situ from diverse air-stable precursors (RSO2NHCH2SiR2 CH2Cl 135) (Scheme 16C), and this silyl source could easily undergo an intermolecular coupling-cyclization with terminal alkynes 2 in the presence of Pd (II)-catalysts, producing 3-silatetrahydropyridines 136 and diverse silaazacycle derivatives (Wang X et al., 2021).
9 Conclusion and perspectives
In summary, the methods for rapid assembly of silacycles are valuable to the fields of synthetic chemistry, material science, and biological chemistry. Therefore, the discovery of new reagents and new synthetic methodologies plays an important role in the development of organosilane chemistry. To date, the coupling-reaction between hydrosilanes with unsaturated hydrocarbons, alkanes, and arenes has been well-established; meanwhile, the studies on C-Si σ bond activation- and Si-Si σ bond activation-based coupling-cyclization have also obtained significant progress. By contrast, silylenoid-involved coupling-cyclization is very rarely reported. Although several silylenoid precursors such as di-tert-butyldiazidosilanes (Welsh et al., 1988), diamidodichlorosilanes (Denk et al., 1994), and cyclohexene-derived silacyclopropanes (Driver et al., 2002) have been reported, their applications in the construction of silacycles are very limited. Thus, a major goal for the future focus of this field is the development of silylenoid-based new organic reactions, which will be believed to provide a versatile strategy to access more complex structural silacycles.
Author contributions
FC: investigation and editing; LL: investigation; WZ: supervision, writing—review. All authors contributed to the article and approved the submitted version.
Funding
This work is supported by the NSFC (No. 22271100), KARDPGP (No. 2020B010188001), GBABRF (No. 2023A1515010070), and CPSF (No. 2021M701243) for financial support.
Conflict of interest
The authors declare that the research was conducted in the absence of any commercial or financial relationships that could be construed as a potential conflict of interest.
Publisher’s note
All claims expressed in this article are solely those of the authors and do not necessarily represent those of their affiliated organizations, or those of the publisher, the editors and the reviewers. Any product that may be evaluated in this article, or claim that may be made by its manufacturer, is not guaranteed or endorsed by the publisher.
References
Ahmad, M., Gaumont, A. C., Durandetti, M., and Maddaluno, J. (2017). Direct syn addition of two silicon atoms to a C≡C triple bond by Si-Si bond activation: Access to reactive disilylated olefins. Angew. Chem. Int. Ed. 56, 2504–2508. doi:10.1002/ange.201611719
Akiyama, T., and Imazeki, S. (1997). Base promoted preparation of alkenylsilanols from allylsilanes. Chem. Lett. 10, 1077–1078. doi:10.1246/cl.1997.1077
Allred, A. L., and Rochow, E. G. (1958). A scale of electronegativity based on electrostatic force. J. Inorg. Nucl. Chem. 5, 264–268. doi:10.1016/0022-1902(58)80003-2
Bai, X. Y., Zou, J. F., Chen, M. Y., Xu, Z., Li, L., Cui, Y. M., et al. (2017). Lewis-base-mediated diastereoselective silylations of alcohols: Synthesis of silicon-stereogenic dialkoxysilanes controlled by chiral aryl BINMOLs. Chem. Asian J. 12, 1730–1735. doi:10.1002/asia.201700640
Breit, N. C., Szilvasi, T., Suzuki, T., Gallego, D., and Inoue, S. (2013). From a zwitterionic phosphasilene to base stabilized silyliumylidene-phosphide and bis(silylene) complexes. J. Am. Chem. Soc. 135, 17958–17968. doi:10.1021/ja409720c
Chang, X., Ma, P. L., Chen, H. C., Li, C. Y., and Wang, P. (2020). Asymmetric synthesis and application of chiral spirosilabiindanes. Angew. Chem. Int. Ed. 59, 9022–9025. doi:10.1002/ange.202002289
Chatgilialoglu, C., Ferreri, C., Landais, Y., and Timokhin, V. I. (2018). Thirty years of (TMS)3SiH: A milestone in radical-based synthetic chemistry. Chem. Rev. 118, 6516–6572. doi:10.1021/acs.chemrev.8b00109
Chen, F., Shao, Y., Li, M., Yang, C., Su, S. - J., Jiang, H. F., et al. (2021). Photocatalyzed cycloaromatization of vinylsilanes with arylsulfonylazides. Nat. Commun. 12, 3304. doi:10.1038/s41467-021-23326-2
Chen, H., Peng, J., Pang, Q., Du, H., Huang, L., Gao, L., et al. (2022). Enantioselective synthesis of spirosilabicyclohexenes by asymmetric dual ring expansion of spirosilabicyclobutane with alkynes. Angew. Chem. Int. Ed. 61, e202212889. doi:10.1002/anie.202212889
Chen, X., Li, M. K., Liu, Z. P., Yang, C., Xie, H. S., Hu, X. W., et al. (2021). Bimetal cooperatively catalyzed arylalkynylation of alkynylsilanes. Org. Lett. 23, 6724–6728. doi:10.1021/acs.orglett.1c02283
Cheng, C., and Hartwig, J. F. (2015). Catalytic silylation of unactivated C-H bonds. Chem. Rev. 115, 8946–8975. doi:10.1021/cr5006414
Denk, M., Lennon, R., Hayashi, R., West, R., Belyakov, A. V., Verne, H. P., et al. (1994). Synthesis and structure of a stable silylene. J. Am. Chem. Soc. 116, 2691–2692. doi:10.1021/ja00085a088
Driver, T. G., Franz, A. K., and Woerpel, K. A. (2002). Diastereoselective silacyclopropanations of functionalized chiral alkenes. J. Am. Chem. Soc. 124, 6524–6525. doi:10.1021/ja020183k
Fang, H., Hou, W., Liu, G., and Huang, Z. (2017). Ruthenium-catalyzed site-selective intramolecular silylation of primary C−H bonds for synthesis of sila-heterocycles. J. Am. Chem. Soc. 139, 11601–11609. doi:10.1021/jacs.7b06798
Fleming, I., and Winter, S. B. D. (1998). Stereocontrol in organic synthesis using silicon-containing compounds. A formal synthesis of prostaglandins controlling the stereochemistry at C-15 using a silyl-to-hydroxy conversion following a stereochemically convergent synthesis of an allylsilane. J. Chem. Soc. Perkin Trans. 17, 2687–2700. doi:10.1039/a804276d
Förster, B., Bertermann, R., Kraft, P., and Tacke, R. (2014). Sila-rhubafuran and derivatives: Synthesis and olfactory characterization of novel silicon-containing odorants. Organometallics 33, 338–346. doi:10.1021/om401070c
Ghavtadze, N., Melkonyan, F. S., Gulevich, A. V., Huang, C., and Gevorgyan, V. (2014). Conversion of 1-alkenes into 1,4-diols through an auxiliary-mediated formal homoallylic C-H oxidation. Nat. Chem. 6, 122–125. doi:10.1038/nchem.1841
Guo, Y., Liu, M. M., Zhu, X., Zhu, L., and He, C. (2021). Catalytic asymmetric synthesis of silicon-stereogenic dihydrodibenzosilines: Silicon central-to-axial chirality relay. Angew. Chem. Int. Ed. 60, 14006–14010. doi:10.1002/ange.202103748
Hirano, K., Yorimitsu, H., and Oshima, K. (2008). Palladium-catalyzed formal cycloaddition of silacyclobutanes with enones: Synthesis of eight-membered cyclic silyl enolates. Org. Lett. 10, 2199–2201. doi:10.1021/ol800603z
Hoshimoto, Y., Yabuki, H., Kumar, R., Suzuki, H., Ohashi, M., and Ogoshi, S. (2014). Highly efficient activation of organosilanes with η2-aldehyde nickel complexes: Key for catalytic syntheses of aryl-vinyl-and alkynyl-benzoxasilolesfficient activation of organosilanes with η2-aldehyde nickel complexes: Key for catalytic syntheses of aryl-vinyl-and alkynyl-benzoxasiloles. J. Am. Chem. Soc. 136, 16752–16755. doi:10.1021/ja510089c
Huang, W. S., Wang, Q., Yang, H., and Xu, L. (2022). State-of-the-art advances in enantioselective transition-metal-mediated reactions of silacyclobutanes. Synthesis 54, 5400–5408. doi:10.1055/a-1929-4890
Huang, Y. H., Wu, Y., Zhu, Z., Zheng, S., Ye, Z., Peng, Q., et al. (2022). Enantioselective synthesis of silicon-stereogenic monohydrosilanes by rhodium-catalyzed intramolecular hydrosilylation. Angew. Chem. Int. Ed. 61, e202113052. doi:10.1002/anie.202113052
Huo, J., Zhong, K., Xue, Y., Lyu, M., Ping, Y., Liu, Z., et al. (2021). Palladium-catalyzed enantioselective carbene insertion into carbon−silicon bonds of silacyclobutanes. J. Am. Chem. Soc. 143, 12968–12973. doi:10.1021/jacs.1c05879
Igawa, K., Kuroo, A., Yoshihiro, D., Yamanaka, Y., and Tomooka, K. (2017). Synthesis of stereoselectively functionalized silacyclopentanes. Synlett 28, 2445–2448. doi:10.1055/s-0036-1590826
Igawa, K., Yoshihiro, D., Abe, Y., and Tomooka, K. (2016). Enantioselective synthesis of silacyclopentanes. Angew. Chem. Int. Ed. 55, 5908–5912. doi:10.1002/ange.201511728
Ilies, L., Tsuji, H., and Nakamura, E. (2009). Synthesis of benzo[b]siloles via KH-promoted cyclization of (2-alkynylphenyl)silanes. Org. Lett. 11, 3966–3968. doi:10.1021/ol9015282
Ilies, L., Tsuji, H., Sato, Y., and Nakamura, E. (2008). Modular synthesis of functionalized benzosiloles by Tin-mediated cyclization of (o-alkynylphenyl)silane. J. Am. Chem. Soc. 130, 4240–4241. doi:10.1021/ja800636g
Ishida, N., Ikemoto, W., and Murakami, M. (2014). Cleavage of C-C and C-Si σ-bonds and their intramolecular exchange. J. Am. Chem. Soc. 136, 5912–5915. doi:10.1021/ja502601g
Ishida, N., Ikemoto, W., and Murakami, M. (2012). Intramolecular σ-bond metathesis between carbon-carbon and silicon-silicon bonds. Org. Lett. 14, 3230–3232. doi:10.1021/ol301280u
Ito, Y., Suginome, M., and Murakami, M. (1991). Palladium (II) acetate-tert-alkyl isocyanide as a highly efficient catalyst for the inter- and intramolecular bis-silylation of carbon-carbon triple bonds. J. Org. Chem. 56, 1948–1951. doi:10.1021/jo00005a055
Kanno, H., Nakamura, K., Noguchi, K., Shibata, Y., and Tanaka, K. (2016). Rhodium-catalyzed cycloisomerization of 2-silylethynyl phenols and anilines via 1,2-silicon migration. Org. Lett. 18, 1654–1657. doi:10.1021/acs.orglett.6b00529
Keipour, H., Carreras, V., and Thierry Ollevier, T. (2017). Recent progress in the catalytic carbene insertion reactions into the silicon-hydrogen bond. Org. Biomol. Chem. 15, 5441–5456. doi:10.1039/c7ob00807d
Kuninobu, Y., Nakahara, T., Takeshima, H., and Takai, K. (2013a). Rhodium-catalyzed intramolecular silylation of unactivated C(sp3)-H bonds. Org. Lett. 15, 426–428. doi:10.1021/ol303353m
Kuninobu, Y., Yamauchi, K., Tamura, N., Seiki, T., and Takai, K. (2013b). Rhodium-catalyzed asymmetric synthesis of spirosilabifluorene derivatives. Angew. Chem. Int. Ed. 52, 1560–1562. doi:10.1002/ange.201207723
Leifert, D., and Studer, A. (2015). 9-Silafluorenes via Base-Promoted Homolytic Aromatic Substitution (BHAS) – the Electron as a Catalystfluorenes via base-promoted homolytic aromatic substitution (BHAS)−the electron as a catalyst. Org. Lett. 17, 386–389. doi:10.1021/ol503574k
Li, L., Zhang, Y., Gao, L., and Song, Z. (2015). Recent advances in C-Si bond activation via a direct transition metal insertion. Tetrahedron Lett. 56, 1466–1473. doi:10.1016/j.tetlet.2015.01.184
Li, Q., Driess, M., and Hartwig, J. F. (2014). Iridium-catalyzed regioselective silylation of aromatic and benzylic C-H bonds directed by a secondary amine. Angew. Chem. Int. Ed. 53, 8471–8474. doi:10.1002/anie.201404620
Liang, Y., Geng, W., Wei, J., and Xi, Z. (2012). Palladium-catalyzed intermolecular coupling of 2-silylaryl bromides with alkynes: Synthesis of benzosiloles and heteroarene-fused siloles by catalytic cleavage of the C(sp3)-Si bond. Angew. Chem. Int. Ed. 51, 1970–1973. doi:10.1002/ange.201108154
Lin, X., Gan, Z., Lu, J., Su, Z., Hu, C., Zhang, Y., et al. (2016). Visible light-promoted radical cyclization of silicon-tethered alkyl iodide and phenyl alkyne. An efficient approach to synthesize benzosilolinesfficient approach to synthesize benzosilolines. Chem. Commun. 52, 6189–6192. doi:10.1039/c6cc00635c
Lin, Y., Jiang, K. -Z., Cao, J., Zheng, Z. -Z., Xu, Z., Cui, Y. -M., et al. (2017). Iridium-catalyzed intramolecular C–H silylation of siloxane-tethered arene and hydrosilane: Facile and catalytic synthesis of cyclic siloxanes. Adv. Synth. Catal. 359, 2247–2252. doi:10.1002/adsc.201700160
Lippert, W. P., Burschka, C., Götz, K., Kaupp, M., Ivanova, D., Gaudon, C., et al. (2009). Silicon analogues of the RXR-selective retinoid agonist SR11237 (BMS649): Chemistry and Biology. ChemMedChem 4, 1143–1152. doi:10.1002/cmdc.200900090
Liu, D., and Kozmin, S. A. (2001). Catalytic enantioselective isomerization of silacyclopentene oxides: New strategy for stereocontrolled assembly of acyclic polyols. Angew. Chem. Int. Ed. 40, 4757–4759. doi:10.1002/1521-3773(20011217)40:24<4757::aid-anie4757>3.0.co;2-s
Long, P. W., Bai, X. F., Ye, F., Li, L., Xu, Z., Yang, K. F., et al. (2018). Construction of six-membered silacyclic skeletons via platinum-catalyzed tandem hydrosilylation/cyclization with dihydrosilanes. Adv. Synth. Catal. 360, 2825–2830. doi:10.1002/adsc.201800456
Ma, W., Liu, L. -C., An, K., He, T., and He, W. (2021). Rhodium-catalyzed synthesis of chiral monohydrosilanes by intramolecular C-H functionalization of dihydrosilanes. Angew. Chem. Int. Ed. 60, 4245–4251. doi:10.1002/anie.202013041
Matsuda, T., and Ichioka, Y. (2012). Rhodium-catalysed intramolecular trans-bis-silylation of alkynes to synthesise 3-silyl-1-benzosiloles. Org. Biomol. Chem. 10, 3175–3177. doi:10.1039/c2ob25242b
Mu, Q.-C., Chen, J., Xia, C.-G., and Xu, L.-W. (2018). Synthesis of silacyclobutanes and their catalytic transformations enabled by transition-metal complexes. Coord. Chem. Rev. 374, 93–113. doi:10.1016/j.ccr.2018.06.015
Murai, M., Takeshima, H., Morita, H., Kuninobu, Y., and Takai, K. (2015). Acceleration effects of phosphine ligands on the rhodium-catalyzed dehydrogenative silylation and germylation of unactivated C(sp3)–H bondsffects of phosphine ligands on the rhodium-catalyzed dehydrogenative silylation and germylation of unactivated C(sp3) −H bonds. J. Org. Chem. 80, 5407–5414. doi:10.1021/acs.joc.5b00920
Namba, T., Kawauchi, S., Shibata, Y., Kanno, H., and Tanaka, K. (2017). Synthesis of alkynylmethylidene-benzoxasiloles through a rhodium-catalyzed cycloisomerization involving 1,2-silicon and 1,3-carbon migration. Angew. Chem. Int. Ed. 56, 3050–3054. doi:10.1002/ange.201612560
Okumura, S., Sun, F., Ishida, N., and Murakami, M. (2017). Palladium-catalyzed intermolecular exchange between C−C and C−Si σ-bonds. J. Am. Chem. Soc. 139, 12414–12417. doi:10.1021/jacs.7b07667
Ouyang, K., Liang, Y., and Xi, Z. (2012). Construction of benzosiloles, Six- and Eight-membered silacyclic skeletons, via a Pd-catalyzed intramolecular Mizoroki-Heck reaction of vinylsilanes. Org. Lett. 14, 4572–4575. doi:10.1021/ol302040j
Parasram, M., Iaroshenko, V. O., and Gevorgyan, V. (2014). Endo-selective Pd-catalyzed silyl methyl Heck reaction. J. Am. Chem. Soc. 136, 17926–17929. doi:10.1021/ja5104525
Pawley, S. B., Conner, A. M., Omer, H. M., and Watson, D. A. (2022). Development of a general method for the Hiyama-Denmark cross-coupling of tetrasubstituted vinyl silanes. ACS Catal. 12, 13108–13115. doi:10.1021/acscatal.2c03981
Pujals, S., Fernández-Carneado, J., Kogan, M. J., Martinez, J., Cavelier, F., and Giralt, E. (2006). Replacement of a proline with silaproline causes a 20-fold increase in the cellular uptake of a pro-rich peptide. J. Am. Chem. Soc. 128, 8479–8483. doi:10.1021/ja060036c
Qin, Y., Han, J. L., Ju, C. W., and Zhao, D. (2020). Ring expansion to 6-7-and 8-membered benzosilacycles through strain-release silicon-based cross-coupling. Angew. Chem. Int. Ed. 59, 8559–8563. doi:10.1002/ange.202001539
Qin, Y., Li, L., Liang, J. Y., Li, K., and Zhao, D. (2021). Silacyclization through palladium-catalyzed intermolecular silicon-based C(sp2)–C(sp3) cross-coupling. Chem. Sci. 12, 14224–14229. doi:10.1039/d1sc04180k
Sakurai, H., Kamiyama, Y., and Nakadaira, Y. (1975). Chemistry of organosilicon compounds 79 Novel [sigma + pi]reactions of hexaorganodisilanes with acetylenes catalyzed by palladium complexes. J. Am. Chem. Soc. 97, 931–932. doi:10.1021/ja00837a061
Sanderson, R. T. (1983). Electronegativity and bond energy. J. Am. Chem. Soc. 105, 2259–2261. doi:10.1021/ja00346a026
Shen, L., Zhao, K., Doitomi, K., Ganguly, R., Li, Y. X., Shen, Z. L., et al. (2017). Lewis acid-catalyzed selective [2 + 2]-cycloaddition and dearomatizing cascade reaction of aryl alkynes with acrylates. J. Am. Chem. Soc. 139, 13570–13578. doi:10.1021/jacs.7b07997
Shi, Y., Shi, X., Zhang, J., Qin, Y., Li, B., and Zhao, D. (2022). Sila-spirocyclization involving unstrained C(sp3)-Si bond cleavage. Nat. Commun. 13, 6697. doi:10.1038/s41467-022-34466-4
Shintani, R., Kurata, H., and Nozaki, K. (2015). Rhodium-catalyzed intramolecular alkynylsilylation of alkynes. Chem. Commun. 51, 11378–11381. doi:10.1039/c5cc04172d
Shintani, R., Moriya, K., and Hayashi, T. (2012). Palladium-catalyzed desymmetrization of silacyclobutanes with alkynes: Enantioselective synthesis of silicon-stereogenic 1-sila-2-cyclohexenes and mechanistic considerations. Org. Lett. 14, 2902–2905. doi:10.1021/ol301191u
Shintani, R., Moriya, K., and Hayashi, T. (2011). Palladium-catalyzed enantioselective desymmetrization of silacyclobutanes: Construction of silacycles possessing a tetraorganosilicon stereocenter. J. Am. Chem. Soc. 133, 16440–16443. doi:10.1021/ja208621x
Shintani, R., Takano, R., and Nozaki, K. (2016). Rhodium-catalyzed asymmetric synthesis of silicon-stereogenic silicon-bridged arylpyridinones. Chem. Sci. 7, 1205–1211. doi:10.1039/c5sc03767k
Su, B., Zhou, T.-G., Li, X. -W., Shao, X. -R., Xu, P. -L., Wu, W. -L., et al. (2017). A chiral nitrogen ligand for enantioselective, iridium-catalyzed silylation of aromatic C-H bonds. Angew. Chem. Int. Ed. 56, 1092–1096. doi:10.1002/anie.201609939
Tang, R. H., Xu, Z., Nie, Y. X., Xiao, X. Q., Yang, K. F., Xie, J. L., et al. (2020). Catalytic asymmetric trans-selective hydrosilylation of bisalkynes to access AIE and CPL-active silicon-stereogenic benzosiloles. iScience 23, 101268. doi:10.1016/j.isci.2020.101268
Tang, X., Zhang, Y., Tang, Y., Li, Y., Zhou, J., Wang, D., et al. (2022). Ring expansion of silacyclobutanes with allenoates to selectively construct 2- or 3-(E)-enoate-substituted silacyclohexenes. ACS Catal. 12, 5185–5196. doi:10.1021/acscatal.1c05831
Teng, Z., and Keese, R. (1999). Palladium-induced intramolecular coupling reactions of some alkenyl(o-iodobenzyl)silanes. Helv. Chim. Acta. 82, 515–521. doi:10.1002/(sici)1522-2675(19990407)82:4<515::aid-hlca515>3.0.co;2-y
Tobisu, M., Onoe, M., Kita, Y., and Chatani, N. (2009). Rhodium-catalyzed coupling of 2-silylphenylboronic acids with alkynes leading to benzosiloles: Catalytic cleavage of the carbon-silicon bond in trialkylsilyl groups. J. Am. Chem. Soc. 131, 7506–7507. doi:10.1021/ja9022978
Tsukada, N., and Hartwig, J. F. (2005). Intermolecular and intramolecular, platinum-catalyzed, acceptorless dehydrogenative coupling of hydrosilanes with aryl and aliphatic methyl C-H bonds. J. Am. Chem. Soc. 127, 5022–5023. doi:10.1021/ja050612p
Ureshino, T., Yoshida, T., Kuninobu, T., and Takai, K. (2010). Rhodium-catalyzed synthesis of silafluorene derivatives via cleavage of Silicon−Hydrogen and Carbon−Hydrogen bondsfluorene derivatives via cleavage of silicon-hydrogen and carbon-hydrogen bonds. J. Am. Chem. Soc. 132, 14324–14326. doi:10.1021/ja107698p
Van Klink, G. P. M., de Boer, H. J. R., Schat, G., Akkerman, O. S., Bickelhaupt, F., and Spek, A. L. (2002). Carbanions as intermediates in the formation of grignard reagents. Organometallics 21, 2119–2135. doi:10.1021/om011083a
Wagner, P., Gulea, M., Suffert, J., and Donnard, M. (2017). Synthesis of benzo[c]silole derivatives bearing a tetrasubstituted exocyclic C=C double bond by palladium-catalyzed domino reactions. Chem. Eur. J. 23, 7458–7462. doi:10.1002/chem.201701736
Wang, W., Zhou, S., Li, L., He, Y., Dong, X., Gao, L., et al. (2021). 3-Silaazetidine: An unexplored yet versatile organosilane species for ring expansion toward silaazacycles. J. Am. Chem. Soc. 143, 11141–11151. doi:10.1021/jacs.1c04667
Wang, X. B., Zheng, Z. Z., Xie, J. L., Gu, X. W., Mu, Q. C., Yin, G. W., et al. (2020). Controllable Si-C bond activation enables stereocontrol in the palladium-catalyzed [4 + 2] annulation of cyclopropenes with benzosilacyclobutanes. Angew. Chem. Int. Ed. 59, 790–797. doi:10.1002/anie.201913060
Wang, X. C., Wang, H. R., Xu, X., and Zhao, D. (2021). Ring expansion to 8-membered silacycles through formal cross-dimerization of 5-membered palladacycles with silacyclobutanes. Eur. J. Org. Chem. 2021, 3039–3042. doi:10.1002/ejoc.202100535
Wang X, X., Huang, S. S., Zhang, F. J., Xie, J. L., Li, Z., Xu, Z., et al. (2021). Multifunctional P-ligand-controlled “silicon-centered” selectivity in Rh/Cu-catalyzed Si–C bond cleavage of silacyclobutanes. Org. Chem. Front. 8, 6577–6584. doi:10.1039/d1qo01386f
Welsh, K. M., Michl, J., and West, R. (1988). The photochemistry of matrix-isolated di-tert-butyldiazidosilane. observation of di-tert-butylsilylene and N, N′-di-tert-butylsilanediimine. J. Am. Chem. Soc. 110, 6689–6696. doi:10.1021/ja00228a014
Wilkinson, J. R., Nuyen, C. E., Carpenter, T. S., Harruff, S. R., and Van Hoveln, R. (2019). Copper-catalyzed carbon-silicon bond formation. ACS Catal. 9, 8961–8979. doi:10.1021/acscatal.9b02762
Xu, H., Fang, X. J., Huang, W. S., Xu, Z., Li, L., Ye, F., et al. (2022). Catalytic regio- and stereoselective silicon-carbon bond formations on unsymmetric gem-difluorocyclopropenes by capture of silyl metal species. Org. Chem. Front. 9, 5272–5280. doi:10.1039/d2qo00943a
Xu, L., Zhang, S., and Li, P. (2015). Synthesis of silafluorenes and silaindenes via silyl radicals from arylhydrosilanes: Intramolecular cyclization and intermolecular annulation with alkynesfluorenes and silaindenes via silylradicals from arylhydrosilanes: Intramolecular cyclization and intermolecular annulations with alkynes. Org. Chem. Front. 2, 459–463. doi:10.1039/c5qo00012b
Yang, B., Yang, W., Guo, Y., You, L., and He, C. (2020). Enantioselective silylation of aliphatic C-H bonds for the synthesis of silicon-stereogenic dihydrobenzosiloles. Angew. Chem. Int. Ed. 59, 22401–22406. doi:10.1002/ange.202009912
Ye, F., and Xu, L. W. (2021). A glimpse and perspective of current organosilicon chemistry from the view of hydrosilylation and synthesis of silicon-stereo-genic silanes. Synlett 32, 1281–1288. doi:10.1055/a-1408-6795
Ye, F., Xu, Z., and Xu, L. W. (2021). The Discovery of multifunctional chiral P ligands for the catalytic construction of quaternary carbon/silicon and multiple stereogenic centers. Acc. Chem. Res. 54, 452–470. doi:10.1021/acs.accounts.0c00740
Yu, N., Wang, C., Zhao, F., Liu, L., Zhang, W.-X., and Xi, Z. (2008). Diverse reactions of 1,4-Dilithio-1,3-dienes with nitriles: Facile access to tricyclic d1-bipyrrolines, multiply substituted pyridines, siloles, and (Z,Z)-Dienylsilanes by tuning of substituents on the butadienyl skeleton. Chem.-Eur. J. 14, 5670–5679. doi:10.1002/chem.200701742
Yuan, W., You, L., Lin, W., Ke, J., Li, Y., and He, C. (2021). Asymmetric synthesis of silicon-stereogenic monohydrosilanes by dehydrogenative C−H silylation. Org. Lett. 23, 1367–1372. doi:10.1021/acs.orglett.1c00029
Zeng, Y., Fang, X. J., Tang, R. H., Xie, J. Y., Zhang, F. J., Xu, Z., et al. (2022). Rhodium-catalyzed dynamic kinetic asymmetric hydrosilylation to access silicon-stereogenic center. Angew. Chem. Int. Ed. 61, e202214147. doi:10.1002/anie.202214147
Zhang, J., Xu, J. Z., Zheng, Z. J., Xu, Z., Cui, Y. M., Cao, J., et al. (2016). Palladium-catalyzed desymmetrization of silacyclobutanes with alkynes to silicon-stereogenic silanes: A density functional theory study. Chem. Asian J. 11, 2867–2875. doi:10.1002/asia.201600709
Zhang, J., Yan, N., Ju, C. W., and Zhao, D. (2021). Nickel(0)-catalyzed asymmetric ring expansion toward enantioenriched silicon-stereogenic benzosiloles. Angew. Chem. Int. Ed. 60, 25927–25932. doi:10.1002/ange.202111025
Zhang, Q. W., An, K., and He, W. (2014). Rhodium-catalyzed tandem cyclization/Si-C activation reaction for the synthesis of siloles. Angew. Chem. Int. Ed. 53, 5667–5671. doi:10.1002/anie.201400828
Zhang, Q. W., An, K., Liu, L. C., Guo, S., Jiang, C., Guo, H., et al. (2016). Rhodium-catalyzed intramolecular C−H silylation by silacyclobutanes. Angew. Chem. Int. Ed. 55, 6427–6431. doi:10.1002/ange.201602376
Zhao, W. T., Gao, F., and Zhao, D. (2018a). Intermolecular σ-bond cross-exchange reaction between cyclopropenones and (Benzo)silacyclobutanes: Straightforward access towards sila(benzo)cycloheptenones. Angew. Chem. Int. Ed. 57, 6329–6332. doi:10.1002/anie.201803156
Keywords: cyclization, silacycles, transition-metal catalysis, photocatalysis, organocatalysis, silaarenes
Citation: Chen F, Liu L and Zeng W (2023) Synthetic strategies to access silacycles. Front. Chem. 11:1200494. doi: 10.3389/fchem.2023.1200494
Received: 05 April 2023; Accepted: 24 May 2023;
Published: 15 June 2023.
Edited by:
Jianrong Steve Zhou, Peking University, ChinaCopyright © 2023 Chen, Liu and Zeng. This is an open-access article distributed under the terms of the Creative Commons Attribution License (CC BY). The use, distribution or reproduction in other forums is permitted, provided the original author(s) and the copyright owner(s) are credited and that the original publication in this journal is cited, in accordance with accepted academic practice. No use, distribution or reproduction is permitted which does not comply with these terms.
*Correspondence: Wei Zeng, emVuZ3dlaUBzY3V0LmVkdS5jbg==