- 1Department of Biological Science and Technology, Faculty of Advanced Engineering, Tokyo University of Science, Tokyo, Japan
- 2Laboratory of Chemical Bioscience, Institute of Biomaterials and Bioengineering, Tokyo Medical and Dental University (TMDU), Tokyo, Japan
An efficient method to prepare organomagnesium intermediates having a protected azido group is reported. Protection of azido groups with di-(tert-butyl)(4-(dimethylamino)phenylphosphine (amphos) and following iodine–magnesium exchange realized the preparation of organomagnesium intermediates, which served in the synthesis of diverse azides by transformation with various electrophiles followed by deprotection with elemental sulfur. Furthermore, click reactions of azides with alkynes enabled synthesizing a wide variety of 1,2,3-triazoles.
1 Introduction
Azides are a significant class of compounds in a broad range of research fields, including synthetic organic chemistry, pharmaceutical sciences, and materials chemistry (Figure 1A) (Bräse and Banert, 2010; Banert, 2016; Yoshida, 2020). Triazole formations by copper-catalyzed azide–alkyne cycloaddition (CuAAC) (Rostovtsev et al., 2002; Tornøe et al., 2002; Meldal and Tornøe, 2008) or strain-promoted azide–alkyne cycloaddition (SPAAC) (Agard et al., 2004; Ning et al., 2008; Dommerholt et al., 2010) have served as click reactions. Azides are also frequently used in organonitrogen syntheses through the Staudinger reduction which takes place smoothly by the treatment of phosphines at ambient temperature (Staudinger and Meyer, 1919; Saxon and Bertozzi, 2000). Despite the importance of azides in synthetic organic chemistry, it is not always easy to synthesize azides owing to the electrophilic nature of azido groups which are susceptible to various nucleophiles, such as carbanions (Tanimoto and Kakiuchi, 2013). In particular, the preparation of carbanions having azido groups is, thus, a challenging issue (Figure 1B). For example, Nagaki and coworkers reported that treatment of 4-bromophenyl azide with n-butyllithium at −78°C under microflow conditions followed by protonation afforded phenyl azides in low yield (Figure 1C–1) (Ichinari et al., 2020), notably showing that the preparation of 4-azidophenyllithium is a challenging transformation, even under microflow conditions. An alternative preparation method for the 4-azidophenyllithium equivalent was successfully developed from 1,4-dibromobenzene (3) under microflow conditions through triazene formation with sulfonyl azide 4 and the subsequent bromine–lithium exchange, leading to aryllithium 5, as a carbanion, having a masked azide moiety (Figure 1C–2) (Ichinari et al., 2020). Although this elegant method allowed us to synthesize a limited variety of 4-substituted phenyl azides, a new approach to prepare carbanions bearing masked azide moieties leading to a wide array of azides is sought after.
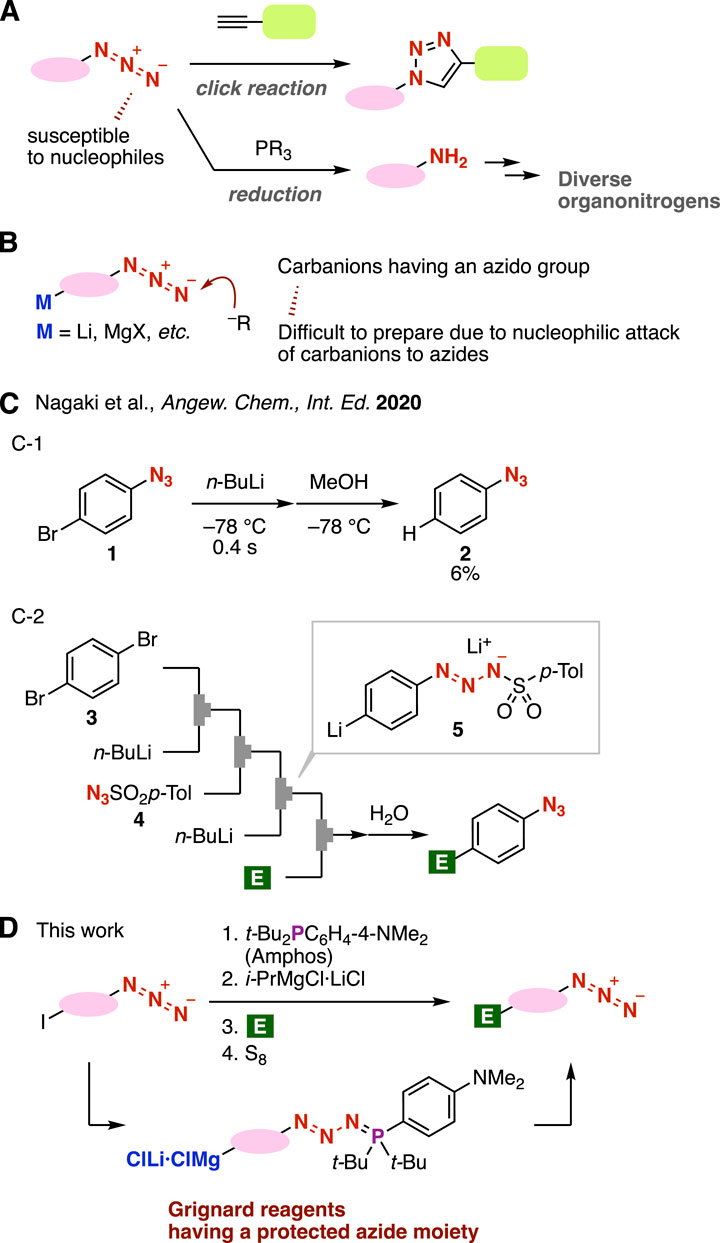
FIGURE 1. (A) Transformations of azides. (B) Carbanions having an azido group. (C) Nagaki’s work. (D) Overview of this work.
In this study, we conceived an idea of preparing carbanions having “protected” azido groups through the treatment of azides with di(tert-butyl)(4-(dimethylamino)phenylphosphine (amphos) (Figure 1D). Previously, we found that amphos smoothly reacts with azides to furnish phosphazides without denitrogenation, and phosphazides can be transformed into azides through deprotection with elemental sulfur (Meguro et al., 2018). Azide protection realized various transformations, such as selective click reactions of diazides and Grignard reactions using carbonyl compounds having azide moieties due to the good stability of phosphazides as protected azides (Aimi et al., 2021). Herein, we describe an efficient method to prepare organomagnesium intermediates by iodine–magnesium exchange with a turbo Grignard reagent after the phosphazide formation of iodine-substituted azides, enabling facile synthesis of diverse 1,2,3-triazoles by Grignard reactions and following CuAAC reactions.
2 Results and discussion
First, we attempted the iodine–magnesium exchange of 4-(4-iodophenyl)phenyl azide (6a) with an isopropylmagnesium chloride lithium chloride complex (Krasovskiy and Knochel, 2004; Bao et al., 2015) in THF at −20°C followed by the addition of N,N-dimethylformamide (DMF) (Figure 2A, route 1). As a result, the desired aldehyde 7a was not obtained due to the decomposition of the azido group. In contrast, we succeeded in the synthesis of aldehyde 7a from iodide 6a in high yield via phosphazide formation (Figure 2A, route 2). Treatment of azide 6a with amphos at room temperature followed by iodine–magnesium exchange with the isopropylmagnesium chloride lithium complex in THF at −20°C and subsequent addition of DMF resulted in efficient formylation. Following deprotection of the phosphazide moiety with elemental sulfur provided azide 7a in good yield without damaging the azido group. The iodine–magnesium exchange with isopropylmagnesium bromide instead of the turbo Grignard reagent also proceeded efficiently (Figure 2B). Aldehyde 7a was prepared in moderate yield when using i-Pr2(n-Bu)MgLi (Inoue et al., 2001) or n-butyllithium for the iodine–metal exchange. Metalation using tert-butyllithium resulted in a complex mixture of products.
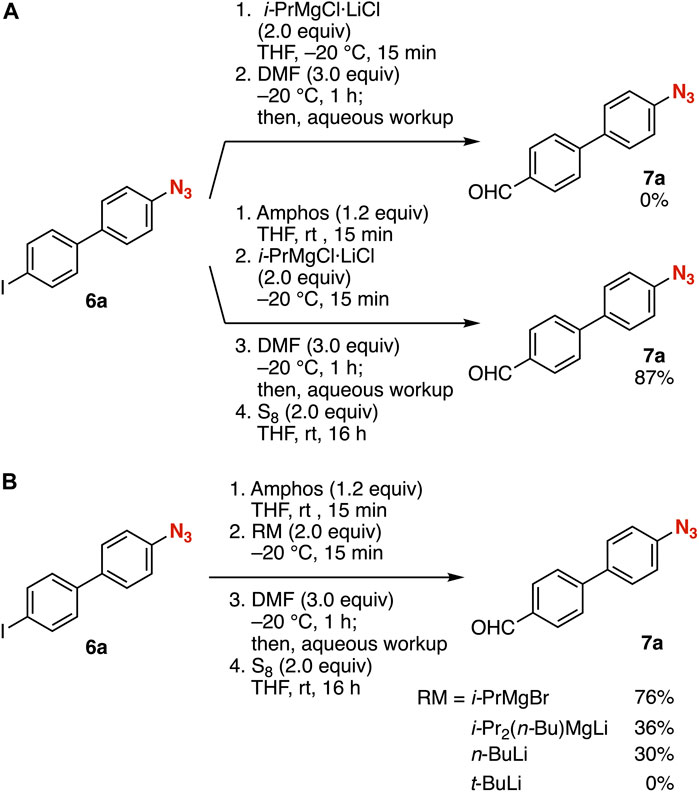
FIGURE 2. (A) Synthesis of 7a from 6a with or without the protection of the azido group. (B) Screening reagents for the iodine–metal exchange.
A wide range of azides 8 were successfully synthesized by the addition of electrophiles to the organomagnesium intermediate prepared in situ from azide 6a (Figure 3). Various aldehydes 9 efficiently reacted with the organomagnesium intermediate, enabling us to synthesize the corresponding alcohols 8a–8e in good yields, leaving azide, benzyl alcohol, chloro, methoxy, and thienyl moieties intact. Tertiary alcohol 8f or 8g was prepared from azide 6a using acetone (10a) or α,α,α-trifluoroacetophenone (10b), respectively, as an electrophile. Allylation of the Grignard reagent prepared from 6a took place to afford the azide 8h after deprotection with elemental sulfur. Bromide 8i was synthesized by bromination of the carbanion with N-bromosuccinimide (NBS), followed by treatment with S8.
We succeeded in the synthesis of aldehydes 7b–7f from a range of azides 6 through phosphazide formation, iodine–magnesium exchange, formylation with DMF, and deprotection with S8 (Figure 4). For example, 4-formyl- or 3-formylphenyl azide 7b or 7c were prepared from 4-iodo- or 3-iodophenyl azide (6b or 6c), respectively. We accomplished the synthesis of trisubstituted benzene 7d from 4-azido-3-methylphenyl iodide (6d) through the phosphazide formation of the ortho methyl-substituted phenyl azide moiety. When using 4-azido-2-chlorophenyl iodide (6e), protection of the azido group, iodine–magnesium exchange, formylation, and deprotection proceeded smoothly for furnishing aldehyde 7e without damaging aldehyde, azide, and chloro moieties. Moreover, we achieved the preparation of the carbanion intermediate having an alkyl azide moiety from azide 6f through phosphazide formation and subsequent iodine–magnesium exchange, which successfully served in formylation with DMF.
Azides bearing the iodo group can be synthesized by formal C–H azidation (Yoshida et al., 2014; Nishiyama et al., 2019) through Ir-catalyzed C–H borylation (Cho et al., 2002; Ishiyama et al., 2002; Mkhalid et al., 2010) and subsequent Cu-catalyzed azidation (Li et al., 2010). Thus, transformations of aryl iodides via the protection of the azido group allowed us to prepare a wide range of highly functionalized aryl azides from simple aryl iodides. For example, C–H borylation of m-iodoanisole catalyzed by iridium proceeded smoothly without damaging the iodo group (Figure 5). Subsequent azidation of the resulting arylboron 14, catalyzed by copper, took place efficiently. Then, we succeeded in the transformation of aryl iodide 6g via phosphazide formation and the iodine–magnesium exchange to provide benzaldehyde 7g, leaving the methoxy, formyl, and azido groups untouched.
A wide variety of 1,2,3-triazoles were easily synthesized from azide 7a without damaging the formyl group (Figure 6). Indeed, we accomplished the synthesis of triazole 16a in high yield by the CuAAC reaction of azide 7a with terminal alkyne 15a in the presence of a catalytic amount of (MeCN)4CuBF4 and tris[(1-benzyl-1H-1,2,3-triazol-4-yl)methyl]amine (TBTA) (Chan et al., 2004). Triazole formation of azide 7a with cycloalkyne 17 proceeded smoothly to afford triazole 16b in good yield without copper catalysis (Dommerholt et al., 2010). We succeeded in the cycloaddition of azide 7a with benzyne generated from o-silylaryl triflate 18 to provide benzotriazole 16c having an aldehyde moiety (Shi et al., 2008). Reductive amination of aldehyde 7a also took place avoiding the reduction of the azido group. Thus, further transformations enabled us to diversify azides after reactions of azide-substituted carbanion equivalents.
Amino alcohols 20a–20d were efficiently synthesized from azides by transformations of carbanions through the protection of azido groups followed by the Staudinger reduction (Figure 7). We achieved the synthesis of amino alcohol 20a from azide 8a with tri-(n-butyl)phosphonium tetrafluoroborate in the presence of triethylamine (Meguro et al., 2017). The Grignard reaction of azide-substituted carbanion equivalents with aldehyde 9a and the following Staudinger reduction realized the preparation of a number of amino alcohols 20b–20d in good yields. Considering the pivotal role of amines and alcohols in the preparation of azaheterocycles, the synthesis of amino alcohols from iodine-substituted azides is poised to make significant contributions to the field of synthetic organic chemistry.
Grignard reactions of azide-substituted carbanion equivalents and the subsequent CuAAC reaction enabled us to synthesize a broad variety of 1,2,3-triazoles from diverse azides, aldehydes, and terminal alkynes (Figure 8). After treatment of 3-iodo-5-methoxyphenyl azide (6g) with amphos followed by the iodine–magnesium exchange, the Grignard reaction with 2-thienyl aldehyde (9e) and deprotection with elemental sulfur resulted in the efficient synthesis of the corresponding alcohol in good yield (Figure 8A). Then, we succeeded in the preparation of triazole 21a by the CuAAC reaction with alkyne 15b bearing an ester moiety. This approach is clearly advantageous over a synthetic route without azide protection, as esters can readily react with carbanions like Grignard reagents. Consequently, the synthesis of triazole 21a from azide 6g, aldehyde 9e, and alkyne 15b was achieved in short steps. Furthermore, triazole 21b was efficiently prepared from azide 6d, aldehyde 9a, and alkyne 15a. We achieved the synthesis of triazole 21c bearing an estradiol scaffold from 4-iodophenyl azide (6b), acetone (10a), and ethinyl estradiol (15c) by a simple protocol through phosphazide formation.
3 Materials and method
For general experimental and instrumental methods, synthetic procedures, and full compound characterization, see the Supplementary Materials.
3.1 Synthesis of aldehyde 7a from aryl iodide 6a
To a solution of 4-azido-4'-iodo-1,1'-biphenyl (6a) (96.8 mg, 0.301 mmol) dissolved in THF (4.0 mL) was added di(tert-butyl)(4-(dimethylamino)phenyl)phosphine (amphos) (95.9 mg, 0.361 mmol, and 1.2 equiv) at room temperature. After stirring for 15 min at the same temperature, we slowly added iPrMgCl・LiCl (1.3 M, THF solution, 0.50 mL, 0.650 mmol, and 2.2 equiv) to it at −20°C. After stirring for 30 min at the same temperature, we also slowly added N,N-dimethylformamide (70.0 µL, 0.904 mmol, and 3.0 equiv) to the solution. After stirring for 1 h at −20°C, we slowly added water (5 mL) to it. The mixture was extracted with EtOAc (10 mL × 3). The combined organic extract was washed with brine (10 mL) and dried with Na2SO4. After filtration, the filtrate was concentrated under reduced pressure. We added S8 (19.7 mg, 0.614 mmol, and 2.0 equiv) to the residue dissolved in THF (4.0 mL) at room temperature. After stirring for 16 h at the same temperature, the mixture was concentrated under reduced pressure. The residue was purified by preparative TLC (n-hexane/EtOAc = 1/1) to give 4-(4-azidophenyl)benzaldehyde (7a) (55.6 mg, 0.249 mmol, and 83%) as a pale yellow solid.
3.2 4-Azido-4′-iodo-1,1′-biphenyl (6a)
Pale yellow solid; Mp 122–124°C; TLC Rf 0.65 (n-hexane/EtOAc = 10/1); 1H NMR (CDCl3, 400 MHz): δ 7.07–7.13 (AA’BB’, 2H), 7.25–7.33 (AA’BB’, 2H), 7.51–7.57 (AA’BB’, 2H), and 7.74–7.79 (AA’BB’, 2H); 13C{1H} NMR (CDCl3, 101 MHz): δ 93.1, 119.5, 128.2, 128.6 (two signals overlapped), 136.7, 137.9, and 139.6; IR (Nujol, cm–1): 810, 1,296, 1,306, 1,377, 1,388, 1,463, 1,478, 2,106, 2,139, 2,855, 2,924, and 2,953; and HRMS (FAB) m/z: [M]·+ calcd for C12H8IN3·+ 320.9763; found 320.9779.
4 Conclusion
In conclusion, we succeeded in the preparation of organomagnesium intermediates having protected azido groups. Various azides were successfully synthesized by the Grignard reaction of carbanions having phosphazide moieties with various electrophiles followed by deprotection with elemental sulfur. Since a broad range of organonitrogens, such as amines and triazoles, are easily prepared from azides, reactions involving carbanion equivalents with azide moieties, followed by subsequent transformations, are poised to significantly contribute to organonitrogen synthesis. Our laboratory is currently engaged in further studies, including the preparation and transformations of carbanions with phosphazide moieties.
Data availability statement
The original contributions presented in the study are included in the article/Supplementary Materials; further inquiries can be directed to the corresponding author.
Author contributions
SY directed the study, conceived the experiments, and wrote the paper. RN and MS planned and performed the experiments and wrote the paper. All authors contributed to the article and approved the submitted version.
Funding
This work was supported by JSPS KAKENHI, grant number JP22H02086 (SY), and the Asahi Glass Foundation (SY).
Conflict of interest
The authors declare that the research was conducted in the absence of any commercial or financial relationships that could be construed as a potential conflict of interest.
Publisher’s note
All claims expressed in this article are solely those of the authors and do not necessarily represent those of their affiliated organizations, or those of the publisher, the editors, and the reviewers. Any product that may be evaluated in this article, or claim that may be made by its manufacturer, is not guaranteed or endorsed by the publisher.
Supplementary material
The Supplementary Material for this article can be found online at: https://www.frontiersin.org/articles/10.3389/fchem.2023.1237878/full#supplementary-material
References
Agard, N. J., Prescher, J. A., and Bertozzi, C. R. (2004). A strain-promoted [3 + 2] Azide−Alkyne cycloaddition for covalent modification of biomolecules in living systems. J. Am. Chem. Soc. 126, 15046–15047. doi:10.1021/ja044996f
Aimi, T., Meguro, T., Kobayashi, A., Hosoya, T., and Yoshida, S. (2021). Nucleophilic transformations of azido-containing carbonyl compounds via protection of the azido group. Chem. Commun. 57, 6062–6065. doi:10.1039/d1cc01143j
Banert, K. (2016). The chemistry of unusually functionalized azides. Synthesis 48, 2361–2375. doi:10.1055/s-0035-1561454
Bao, R. L.-Y., Zhao, R., and Shi, L. (2015). Progress and developments in the turbo grignard reagent i-PrMgCl·LiCl: A ten-year journey. Chem. Commun. 51, 6884–6900. doi:10.1039/c4cc10194d
Bräse, S., and Banert, K. (2010). Organic azides: Syntheses and applications. Chichester: John Wiley and Sons, Ltd.
Chan, T. R., Hilgraf, R., Sharpless, K. B., and Fokin, V. V. (2004). Polytriazoles as copper(I)-Stabilizing ligands in catalysis. Org. Lett. 6, 2853–2855. doi:10.1021/ol0493094
Cho, J.-Y., Tse, M. K., Holmes, D., Maleczka, R. E., and Smith, M. R. (2002). Remarkably selective iridium catalysts for the elaboration of aromatic C-H bonds. Science 295, 305–308. doi:10.1126/science.1067074
Dommerholt, J., Schmidt, S., Temming, R., Hendriks, L. J. A., Rutjes, F. P. J. T., van Hest, J. C. M., et al. (2010). Readily accessible bicyclononynes for bioorthogonal labeling and three-D imensional imaging of living cells. Angew. Chem. Int. Ed. 49, 9612–9615. doi:10.1002/ange.201003761
Ichinari, D., Ashikari, Y., Mandai, K., Aizawa, Y., Yoshida, J.-i., and Nagaki, A. (2020). A novel approach to functionalization of aryl azides through the generation and reaction of organolithium species bearing masked azides in flow microreactors. Angew. Chem. Int. Ed. 59, 1583–1587. doi:10.1002/ange.201912419
Inoue, A., Kitagawa, K., Shinokubo, H., and Oshima, K. (2001). Selective Halogen−Magnesium exchange reaction via organomagnesium ate complex. J. Org. Chem. 66, 4333–4339. doi:10.1021/jo015597v
Ishiyama, T., Takagi, J., Hartwig, J. F., and Miyaura, N. (2002). A stoichiometric aromatic C–H borylation catalyzed by iridium(I)/2,2’-Bipyridine complexes at room temperature. Angew. Chem. Int. Ed. 41, 3056–3058. doi:10.1002/1521-3773(20020816)41:16<3056::aid-anie3056>3.0.co;2-#
Krasovskiy, A., and Knochel, P. (2004). A LiCl-mediated Br/Mg exchange reaction for the preparation of functionalized aryl- and heteroarylmagnesium compounds from organic bromides. Angew. Chem. Int. Ed. 43, 3333–3336. doi:10.1002/anie.200454084
Li, Y., Gao, L.-X., and Han, F.-S. (2010). Reliable and diverse synthesis of aryl azides through copper-catalyzed coupling of boronic acids or esters with TMSN3. Chem. Eur. J. 16, 7969–7972. doi:10.1002/chem.201000971
Meguro, T., Yoshida, S., and Hosoya, T. (2017). Aromatic azido-selective reduction via the staudinger reaction using tri-n-butylphosphonium tetrafluoroborate with triethylamine. Chem. Lett. 46, 473–476. doi:10.1246/cl.161159
Meguro, T., Yoshida, S., Igawa, K., Tomooka, K., and Hosoya, T. (2018). Transient protection of organic azides from click reactions with alkynes by phosphazide formation. Org. Lett. 20, 4126–4130. doi:10.1021/acs.orglett.8b01692
Meldal, M., and Tornøe, C. W. (2008). Cu-catalyzed Azide−Alkyne cycloaddition. Chem. Rev. 108, 2952–3015. doi:10.1021/cr0783479
Mkhalid, I. A. I., Barnard, J. H., Marder, T. B., Murphy, J. M., and Hartwig, J. F. (2010). C−H activation for the construction of C−B bonds. Chem. Rev. 110, 890–931. doi:10.1021/cr900206p
Ning, X., Guo, J., Wolfert, M. A., and Boons, G.-J. (2008). Visualizing metabolically labeled glycoconjugates of living cells by copper-free and fast huisgen cycloadditions. Angew. Chem. Int. Ed. 47, 2253–2255. doi:10.1002/anie.200705456
Nishiyama, Y., Misawa, Y., Hazama, Y., Oya, K., Yoshida, S., and Hosoya, T. (2019). Synthesis of diverse 3-Azido-5-(azidomethyl)benzene Derivatives via formal C–H azidation and functional group-selective transformations. Heterocycles 99, 1053–1072. doi:10.3987/com-18-s(f)72
Rostovtsev, V. V., Green, L. G., Fokin, V. V., and Sharpless, K. B. (2002). A stepwise huisgen cycloaddition process: Copper(I)-Catalyzed regioselective “ligation” of azides and terminal alkynes. Angew. Chem. Int. Ed. 41, 2596–2599. doi:10.1002/1521-3773(20020715)41:14<2596::aid-anie2596>3.0.co;2-4
Saxon, E., and Bertozzi, C. R. (2000). Cell surface engineering by a modified staudinger reaction. Science 287, 2007–2010. doi:10.1126/science.287.5460.2007
Shi, F., Waldo, J. P., Chen, Y., and Larock, R. C. (2008). Benzyne click chemistry: Synthesis of benzotriazoles from benzynes and azides. Org. Lett. 10, 2409–2412. doi:10.1021/ol800675u
Staudinger, H., and Meyer, J. (1919). Über neue organische Phosphorverbindungen III. Phosphinmethylenderivate und Phosphinimine. Helv. Chim. Acta 2, 635–646. doi:10.1002/hlca.19190020164
Tanimoto, H., and Kakiuchi, K. (2013). Recent applications and developments of organic azides in total synthesis of natural products. Nat. Prod. Commun. 8, 1021–1034. doi:10.1177/1934578x1300800730
Tornøe, C. W., Christensen, C., and Meldal, M. (2002). Peptidotriazoles on solid phase: [1,2,3]-Triazoles by regiospecific copper(I)-Catalyzed 1,3-Dipolar cycloadditions of terminal alkynes to azides. J. Org. Chem. 67, 3057–3064. doi:10.1021/jo011148j
Yoshida, S., Misawa, Y., and Hosoya, T. (2014). Formal C–H-Azidation-Based shortcut to Diazido building blocks for the versatile preparation of photoaffinity labeling probes. Eur. J. Org. Chem. 2014, 3991–3995. doi:10.1002/ejoc.201402516
Keywords: azides, triazoles, protection, click chemistry, iodine–magnesium exchange, turbo Grignard reagent, phosphazide, phosphines
Citation: Namioka R, Suzuki M and Yoshida S (2023) Synthesis of 1,2,3-triazoles using Grignard reactions through the protection of azides. Front. Chem. 11:1237878. doi: 10.3389/fchem.2023.1237878
Received: 10 June 2023; Accepted: 17 July 2023;
Published: 31 July 2023.
Edited by:
Takashi Ohshima, Kyushu University, JapanReviewed by:
Yoshitaka Hamashima, University of Shizuoka, JapanRajendra Rohokale, University of Florida, United States
Copyright © 2023 Namioka, Suzuki and Yoshida. This is an open-access article distributed under the terms of the Creative Commons Attribution License (CC BY). The use, distribution or reproduction in other forums is permitted, provided the original author(s) and the copyright owner(s) are credited and that the original publication in this journal is cited, in accordance with accepted academic practice. No use, distribution or reproduction is permitted which does not comply with these terms.
*Correspondence: Suguru Yoshida, cy15b3NoaWRhQHJzLnR1cy5hYy5qcA==