- 1Institute for Advanced Study, Kyushu University, Fukuoka, Japan
- 2Department of Applied Chemistry, Kyushu University, Fukuoka, Japan
Thermally activated delayed fluorescence (TADF) materials with high photoluminescence quantum yields and fast reverse intersystem crossing (RISC) capabilities are highly desirable for applications in high-efficiency organic light-emitting diodes. Herein, we report the synthesis as well as structural and photophysical properties of 5,9-diselena-13b-boranaphtho[3,2,1-de]anthracene (SeBSe) as a narrowband-emissive TADF material. The incorporation of two selenium atoms into the boron-fused pentacyclic π-core results in a small singlet–triplet energy gap (ΔEST) and thereby significant TADF properties. Moreover, theoretical calculations revealed a noticeable spin-orbit coupling enhancement between the singlet and triplet manifolds in SeBSe by virtue of the heavy-atom effect of selenium atoms. Consequently, SeBSe allows ultrafast spin-flip RISC with the rate constant surpassing 108 s−1, which far exceeds the corresponding fluorescence radiative decay rate (∼106 s−1), enabling an ideal singlet–triplet superimposed excited state.
1 Introduction
Thermally activated delayed fluorescence (TADF) is an emission phenomenon induced by the reverse intersystem crossing (RISC) process between the lowest excited triplet (T1) and singlet (S1) states Uoyama et al. (2012). In general, RISC involving spin-flip is the rate-limiting step in the overall TADF process; however, it can be facilitated by minimizing the energy gap (ΔEST) and strengthening the spin-orbit coupling (SOC) between S1 and T1 in TADF systems Wada et al. (2020); Aizawa et al. (2021). In the conventionally designed TADF materials, donor (D) and acceptor (A) units are integrated in a twisting manner to spatially separate the highest occupied molecular orbital (HOMO) and lowest unoccupied molecular orbital (LUMO) onto the D and A units, respectively, hence minimizing ΔEST (typically below 0.2 eV) Wong and Zysman-Colman (2017); Cai and Su (2018); Liu et al. (2018). However, the intrinsic intramolecular charge transfer (ICT) characteristics inevitably cause substantial structural relaxation between the ground and excited states, resulting in broadening of the emission spectrum with a large full width at half maximum (FWHM ≥70 nm). Such broad emissions negatively affect the color purity of the emitters, particularly when considering their application in organic light-emitting diodes (OLEDs).
Recently, Hatakeyama et al. introduced a pioneering concept, multi-resonance (MR)-TADF, by installing electron-accepting boron (B) and electron-donating nitrogen (N) or oxygen (O) atoms at site-specific positions to induce the alternating resonance effects and attain TADF Hatakeyama et al. (2016); Hirai et al. (2015). In contrast to the conventional D–A-type TADF systems, the short-range charge transfer of MR-TADF systems based on the atomically separated HOMO and LUMO allows them to suppress structural relaxation and vibronic coupling, leading to a small ΔEST, narrowband emissions, and high photoluminescence quantum yields Kim and Yasuda (2022). While most MR-TADF materials exhibit very slow RISC rates on the order of 104 s–1, our group (Nagata et al., 2021; Park et al., 2022a; Park et al., 2022b) and others (Chen et al., 2021; Hu et al., 2022) have revealed that electronic perturbations from sulfur (S) or selenium (Se) atoms can significantly enhance SOC and thereby accelerate RISC in MR-TADF systems. In 2021, Chen et al. reported fused pentacyclic molecules, OBS and SBS (Figure 1), in which the O atoms of OBO (Hirai et al., 2015) were replaced stepwise by S atoms. They found that the incorporation of S atoms gradually decreased the ΔEST value and simultaneously enhanced the SOC, increasing the RISC rate up to ∼105 s−1 Chen et al. (2021). In 2022, our group achieved a record-setting RISC rate as high as ∼108 s−1 for a heavier Se-doped MR-TADF emitter Park et al. (2022a). As supported by recent theoretical and computational studies (Pratik et al., 2022; Hagai et al., 2024), systematic chalcogen replacement is a viable and effective approach for controlling the photophysical properties and exciton kinetics of MR-TADF systems.
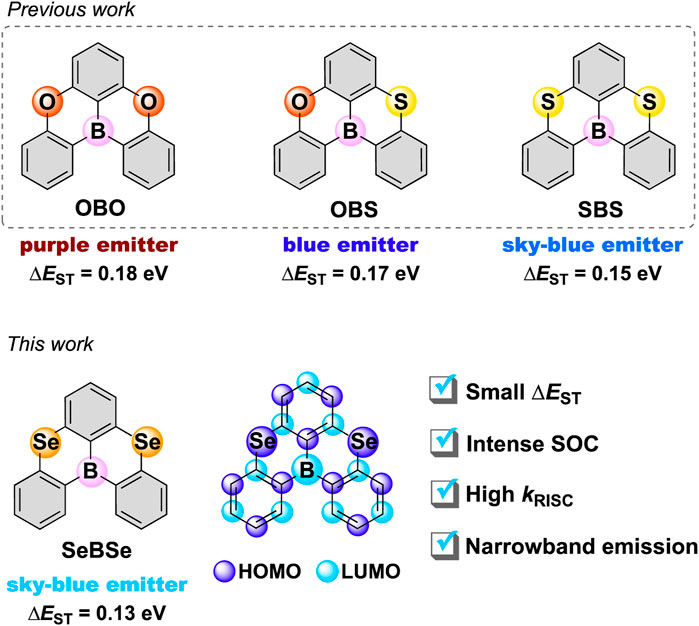
Figure 1. Chemical structures of the XBX-series MR-TADF emitters (X = O, S, and Se). The localized HOMO and LUMO in SeBSe are illustrated by purple and light-blue balls, respectively.
Herein, we report the synthesis as well as structural and photophysical properties of 5,9-diselena-13b-boranaphtho[3,2,1-de]anthracene (SeBSe; Figure 1) as a new MR-TADF framework. The incorporation of two Se atoms into the common fused pentacyclic π-core caused a large helical distortion of the entire skeleton, resulting in structural and electronic property changes compared to the parent OBO. Computational simulations suggested that the SOC matrix elements of SeBSe were significantly enhanced compared to those of the previously reported O- and S-doped congeners (OBO and SBS). In addition, doping with heavier chalcogens led to smaller ΔEST values, thereby enhancing the TADF properties. SeBSe achieved ultrafast spin-flip RISC with a rate constant of ∼108 s−1, which is approximately three orders of magnitude higher than that of SBS.
2 Results and discussion
2.1 Synthesis and structural analysis
SeBSe was synthesized in two steps using commercially available 2-bromo-1,3-diiodobenzene (1) as the starting material (Scheme 1). Precursor 2 was prepared by reacting 1 with diphenyl diselenide (Ph2Se2) in acetonitrile under reflux in the presence of a catalytic amount of copper(I) iodide and a large excess of cesium carbonate Zhang et al. (2021). The latter step is the so-called one-pot borylation (Hatakeyama et al., 2016), which consists of lithiation/substitution followed by tandem bora-Friedel-Crafts reactions. The final target, SeBSe, was fully characterized using 1H and 13C NMR, mass spectrometry, and single-crystal X-ray crystallography. The detailed synthesis procedures and characterization data are provided in the Supplementary Material (Supplementary Figures S1, S2).
Single crystals suitable for X-ray analysis were obtained by the slow diffusion of ethanol into a chloroform solution of SeBSe at room temperature. Interestingly, SeBSe self-organized into a crystal structure with the Sohncke space group P212121, which contained no mirror nor inversion symmetry operations (Supplementary Table S1). The fact that SeBSe without a chiral center crystallizes in such a non-centrosymmetric space group can be related to its molecular helicity. Crystallographic analysis revealed that SeBSe adopts a highly distorted nonplanar structure, forming a pair of helical enantiomers (Figures 2A, B) because of the bond length mismatch caused by the rather long C–Se bonds and intramolecular steric repulsion between the adjacent benzene rings. As indicated by the dihedral angles (φ = ∠C6–C7–C8–C9) around the helicity, the right-handed helicene (P-SeBSe, φ = +53.2°) seems to be slightly more distorted compared to the left-handed one (M-SeBSe, φ = −52.7°). The bond angles ∠C1–Se1–C4 and ∠C3–Se2–C5 are 100.3° and 99.9° for M-SeBSe, while they are 99.6° and 103° for P-SeBSe. For M-SeBSe, all four C–Se bonds fall in the range of 1.88–1.94 Å, suggesting single bond character. In the case of the more distorted P-SeBSe, the outer C4–Se1 and C5–Se2 bonds (2.00–2.03 Å) are elongated, whereas the inner C1–Se1 and C3–Se2 bonds (1.71–1.80 Å) are contracted. Therefore, SeBSe inevitably prefers to form helicene structures rather than planar structures because these C–Se bonds are substantially longer than the C–B bonds (1.54–1.57 Å). As illustrated in Figure 2C, M-SeBSe (green) and P-SeBSe (pink) enantiopairs are alternately arranged and closely packed via noncovalent C–H···π(C) and C–H···n(Se) interactions in the crystals.
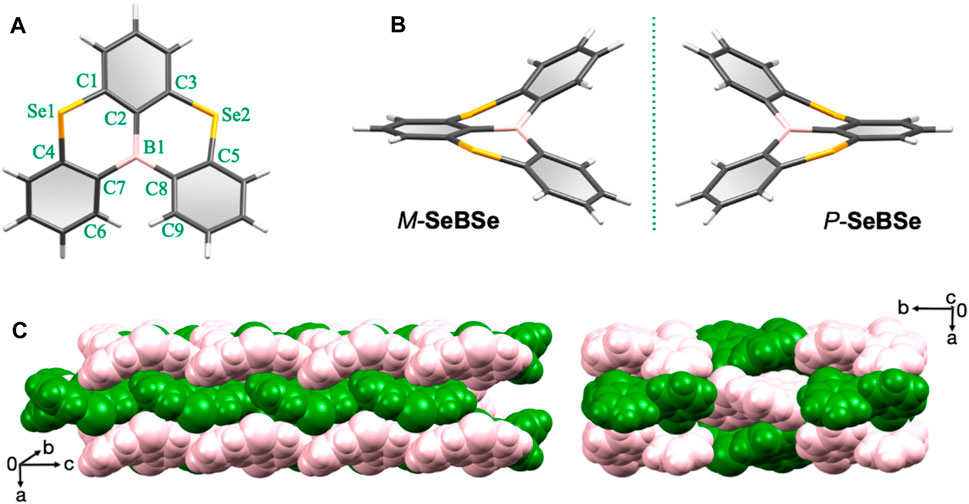
Figure 2. Single-crystal X-ray structures of SeBSe (CCDC 2326001): (A) top view of M-SeBSe, (B) side view of a helical M-SeBSe and P-SeBSe enantiopair with a mirror-image relationship, and (C) space-filling representations of the packing structure viewed from different angles. The M-SeBSe and P-SeBSe are drawn in green and pink colors, respectively, for clarity.
2.2 Computational simulations
The ground-state (S0) geometries of SeBSe and its congeners (SBS and OBO) were optimized using density functional theory (DFT) calculations at the B3LYP/6-31G(d) level (Supplementary Figure S3). In contrast to the fully planar OBO, the computed S0 state of SeBSe adopted a helically distorted structure, as expected, which is in good agreement with the single-crystal structure. The dihedral angle around the helicity (φ) was estimated to be 50.8°, which is comparable to that observed in the single-crystal structure (∼53°). To gain insight into the dynamic helicity inversion (i.e., M-SeBSe ⇄ P-SeBSe), potential energy surface scans were performed for SeBSe by varying the degree of φ using the DFT method (Supplementary Figure S4). A nearly planar conformer of SeBSe has the highest energy, with an energy barrier of ∼69 kJ mol−1 for helicity inversion. Hence, we attempted optical resolution using preparative HPLC equipped with a chiral column but were unable to separate each helical enantiomer under multiple sets of conditions. Unlike in the solid states, helicity interconversion (or racemization) of SeBSe may occur rapidly in solution at room temperature.
To understand the nature of each ring comprising SeBSe, we calculated the nucleus-independent chemical shifts (NICS) (Chen et al., 2005) at the geometrical center of the ring (NICS(0)) (Figure 3A). The large negative NICS(0) values of the three peripheral benzene rings (−5.9 to −6.0) indicate the presence of induced diatropic ring currents owing to aromaticity, whereas the two selenaborin rings exhibit small positive NICS(0) values (+1.6 and +1.1) attributable to non-aromaticity.
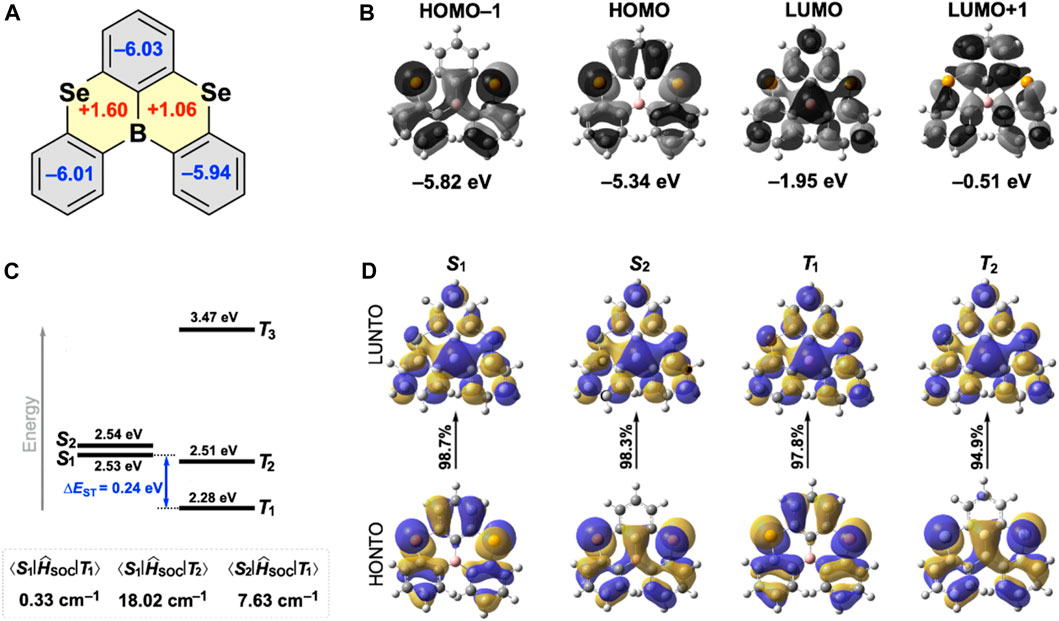
Figure 3. (A) NICS(0) values for SeBSe calculated at the B3LYP/6-311+G(d,p)//B3LYP/6-31G(d) level; the aromatic and non-aromatic rings are drawn in gray and yellow, respectively. (B) FMOs, (C) simulated energy-level diagram with the associated SOC matrix elements, and (D) NTOs for SeBSe calculated at the B3LYP/6-31G(d) level.
As with common MR-TADF molecules, the Frontier molecular orbitals (FMOs) of SeBSe were characterized by significant localization on the constituent atoms (Figure 3B). Despite the helically distorted structure, the HOMO and LUMO of SeBSe are extended throughout the molecule with complementary spatial distributions, where the Se and B atoms intensively contribute to inducing the MR effects. Although similar FMO patterns were observed in OBO and SBS, the electron density distribution on the two chalcogen atoms increased significantly when changing from O to S and Se (Figure 3B and Supplementary Figure S5). The calculated HOMO energy levels gradually increased in the order of OBO, SBS, and SeBSe (−5.62, −5.44, and −5.34 eV, respectively), whereas the LUMO energy levels decreased in the same order (−1.67, −1.90, and −1.95 eV, respectively). Accordingly, the HOMO–LUMO gap of SeBSe (3.39 eV) is considerably smaller than those of SBS (3.54 eV) and OBO (3.95 eV) and therefore, SeBSe is expected to emit at a longer wavelength (or lower energy).
We further computed and analyzed the energy landscape and natural transition orbitals (NTOs) of the excited singlet and triplet states. For SeBSe, the two lowest excited singlet states (S1 and S2) were nearly degenerate and energetically close to the higher-order triplet state (T2) (Figure 3C). This configuration is suitable for effectively harvesting the radiative S1 state through various channels from T1, T2, and S2. The NTO analysis of SeBSe (Figure 3D) indicates that the highest occupied NTOs (HONTOs) for S1 and T1 are very similar to the HOMO, while the HONTOs for S2 and T2 correspond to HOMO−1. The lowest unoccupied NTOs (LUNTOs) for these excited states are predominantly characterized by the LUMO. We also calculated the SOC matrix elements (
2.3 Photophysical properties and kinetics
Figure 4 shows the basic photophysical properties of SeBSe in a dilute toluene solution, and Table 1 summarizes the relevant data. SeBSe exhibited an intense absorption peak (λabs) at 448 nm, which can be attributed to the HOMO→LUMO electronic transition. This main absorption band is considerably red-shifted compared to those reported for OBO (λabs = 378 nm) and SBS (λabs = 431 nm) (Chen et al., 2021), which is in agreement with the results of their computationally simulated absorption spectra (Supplementary Figure S7). The deoxygenated solution of SeBSe exhibited strong sky-blue photoluminescence (PL) with an emission peak (λPL) at 477 nm and absolute quantum yield (ΦPL) of 71%. Similar to the absorption profile, the emission band of SeBSe was red-shifted with respect to those of OBO and SBS (λPL = 396 and 457 nm, respectively) but maintained a narrow spectral FWHM of 34 nm (0.18 eV). The PL emission of SeBSe was completely quenched in an aerated solution (Figure 4C), suggesting that the ISC (S1→T1) and subsequent exciton quenching by triplet oxygen is much faster than the fluorescence radiative process (S1→S0). The S1 and T1 excitation energies (ES and ET) of SeBSe were estimated to be 2.60 and 2.47 eV, respectively, from the fluorescence and phosphorescence peaks (Figure 4B), resulting in a ΔEST of 0.13 eV. This value is smaller than those of OBO (ΔEST = 0.18 eV) and SBS (ΔEST = 0.15 eV) (Chen et al., 2021), suggesting that heavier chalcogen doping reduces the ΔEST.
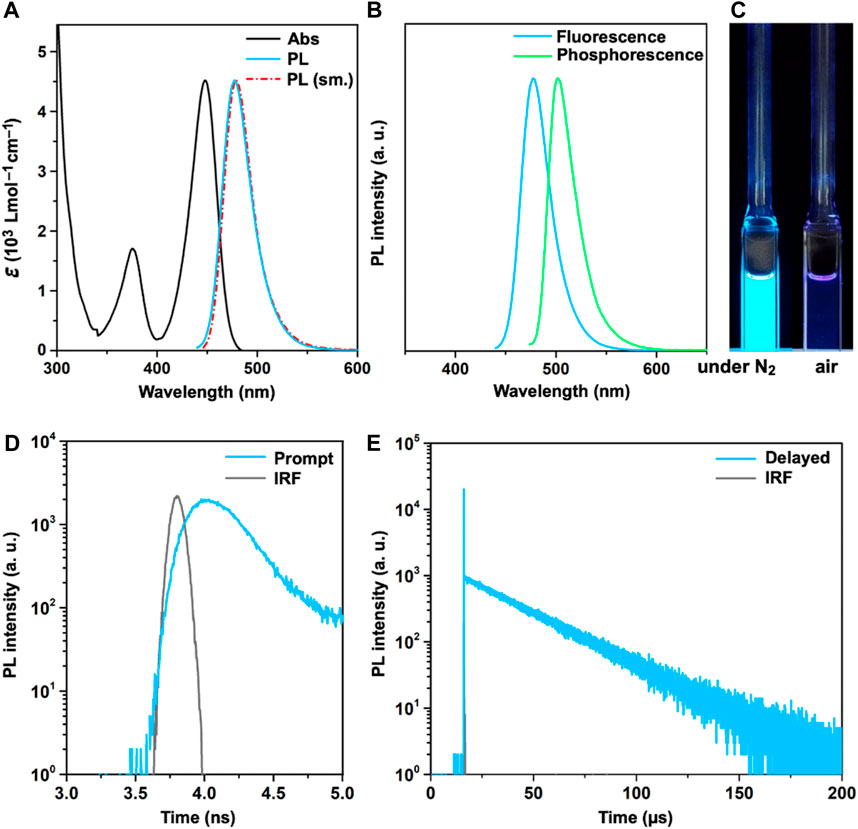
Figure 4. (A) UV/Vis absorption (black) and PL spectra (sky-blue) of SeBSe in a deoxygenated toluene solution (10–5 M) at 300 K, together with its simulated PL profile (red); (B) normalized fluorescence and phosphorescence spectra of the SeBSe solution measured at 300 and 77 K, respectively; (C) photograph showing sky-blue emission under UV illumination at 365 nm; transient PL decay profiles of the SeBSe solution in (D) nanosecond and (E) microsecond regime. The instrument response function (IRF) is shown as gray lines in (D, E).
The transient PL properties highlight the unique exciton kinetics of SeBSe, which are somewhat different from typical TADF (Figures 4D, E). The emission component in the nanosecond regime (typically attributed to prompt fluorescence) was almost negligible, as characterized by an extremely small fractional quantum yield (<0.1%) and short picosecond-order lifetime (τp ∼200 ps). In contrast, the emission component in the microsecond regime (usually regarded as delayed fluorescence) dominated the overall ΦPL. SeBSe thus demonstrated a quasi-single-component transient PL decay with a lifetime (τd) of 27.8 μs, which is markedly different from the two-component behavior commonly observed in TADF. This implies that the spin-flip ISC/RISC cycles were drastically accelerated by Se doping, making them much faster than the competing fluorescence radiative process. Following a recent method for exciton kinetic analysis (Park et al., 2022a), we estimated the photophysical rate constants for the fluorescence radiative decay, ISC, and RISC (kr, kISC, and kRISC, respectively; Table 1). As expected, the kRISC of SeBSe reached 1.2 × 108 s−1, which is nearly three orders of magnitude higher than that of SBS (Chen et al., 2021), as a consequence of enhanced SOC and reduced ΔEST. Furthermore, the kRISC of SeBSe was approximately two orders of magnitude higher than its kr (1.1 × 106 s−1), indicating that the rate-limiting process was no longer RISC but the fluorescence radiative process. This observation is reminiscent of metal-TADF emitters, where the kISC (typically 109–1011 s–1) is much faster than kr owing to the large SOC imparted by the metal ions. To et al. (2020); Li et al. (2022) The fast ICT characteristics in metal-TADF emitters allow for equilibration of the lowest energy singlet and triplet excited states. Consequently, the emission decays of metal-TADF emitters typically show only a delayed component as a single exponential signal in the microsecond range, similar to the emission observed in phosphorescent organometallic complexes.
We also measured the steady-state and transient PL characteristics of SeBSe in doped thin films using 3,3′-di(carbazole-9-yl)-1,1′-biphenyl (mCBP) as the host matrix (Table 1 and Supplementary Figure S8). The photophysical properties of the SeBSe doped films agreed with those measured as solutions, still retaining high ΦPL and kRISC values as well as the narrowband emission.
2.4 Electroluminescence performance
To evaluate the electroluminescence (EL) performance of SeBSe, we fabricated OLEDs with the following device structure: indium tin oxide (ITO, 50 nm)/2,3,6,7,10,11-hexacyano-1,4,5,8,9,12-hexaazatriphenylene (HAT-CN, 10 nm)/1,1-bis[(di-4-tolylamino)phenyl]cyclohexane (TAPC, 40 nm)/1,3-bis(1,8-dimethylcarbazol-9-yl)benzene (mMCP, 5 nm)/1 wt%-SeBSe doped in 3,3′-di(carbazole-9-yl)-1,1′-biphenyl (mCBP) (30 nm)/2,8-bis(diphenylphosphinyl)dibenzo[b,d]furan (PPF, 5 nm)/1,3-bis[3,5-di(pyridin-3-yl)phenyl]benzene (B3PyPB, 40 nm)/8-quinolinolato lithium (Liq, 1 nm)/Al (100 nm). As depicted in Figure 5A, the SeBSe-based OLED exhibited narrowband sky-blue EL, with an emission peak (λEL) at 481 nm and the CIE chromaticity coordinates of (0.106, 0.241). The EL spectrum coincided well with the corresponding PL spectrum and retained a narrowband feature. The SeBSe-based device exhibited a maximum external quantum efficiency (EQEmax) of 9.3% (Figure 5C), maximum current efficiency (CEmax) of 13.3 cd A–1, and maximum power efficiency (PEmax) of 9.5 lm W−1 (Supplementary Figure S9).
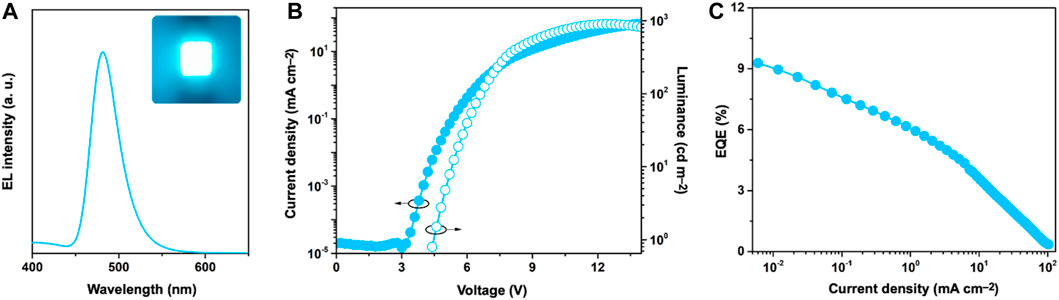
Figure 5. (A) EL spectrum (inset: EL emission color image) measured at 100 cd m–2, (B) current density–voltage–luminance (J–V–L) characteristics, and (C) external EL quantum efficiency (EQE)–current density (J) plot of SeBSe-based device.
3 Conclusion
In this study, we developed a novel Se-doped pentacyclic organoboron emitter (SeBSe) and investigated its structural and photophysical properties. The introduction of heavier Se atoms caused SeBSe to adopt a helically distorted structure. Using spectroscopic analysis, we also demonstrated the ultrafast spin conversion properties of SeBSe with a RISC rate exceeding 108 s−1. Consequently, SeBSe exhibited efficient sky-blue narrowband emission consisting of a quasi-single component from the singlet–triplet superimposed excited state. Currently, pure organic emitters capable of ultrafast spin conversion and excited-state superposition are extremely rare Aizawa et al. (2021); Park et al. (2022a). The SeBSe-based OLED exhibited narrowband sky-blue EL with a maximum external quantum efficiency of 9.3%.
Data availability statement
The datasets presented in this study can be found in online repositories. The names of the repository/repositories and accession number(s) can be found in the article/Supplementary Material.
Author contributions
SK: Conceptualization, Data curation, Formal Analysis, Funding acquisition, Investigation, Methodology, Validation, Writing–original draft, Writing–review and editing. GL: Formal Analysis, Methodology, Writing–review and editing. TY: Conceptualization, Funding acquisition, Methodology, Project administration, Supervision, Validation, Writing–original draft, Writing–review and editing.
Funding
The author(s) declare financial support was received for the research, authorship, and/or publication of this article. This work was supported in part by Grant-in-Aid for JSPS KAKENHI (Grant No. JP21H04694 and JP23KF0062) and JST CREST (Grant No. JPMJCR21O5). SK acknowledges the JSPS Postdoctoral Fellowships for Research in Japan.
Acknowledgments
SK acknowledges the Japan Society for the Promotion of Science (JSPS) for providing a JSPS Postdoctoral Fellowship for Research in Japan (P23030). SK is grateful to Nanami Kubo, Yusei Tanaka, and Jun Hyeon Lee for their technical assistance. The authors are thankful for the support provided by the Cooperative Research Program of “Network Joint Center for Materials and Devices” and the computer facilities at the Research Institute for Information Technology, Kyushu University.
Conflict of interest
The authors declare that the research was conducted in the absence of any commercial or financial relationships that could be construed as a potential conflict of interest.
Publisher’s note
All claims expressed in this article are solely those of the authors and do not necessarily represent those of their affiliated organizations, or those of the publisher, the editors and the reviewers. Any product that may be evaluated in this article, or claim that may be made by its manufacturer, is not guaranteed or endorsed by the publisher.
Supplementary material
The Supplementary Material for this article can be found online at: https://www.frontiersin.org/articles/10.3389/fchem.2024.1375552/full#supplementary-material
References
Aizawa, N., Matsumoto, A., and Yasuda, A. (2021). Thermal equilibration between singlet and triplet excited states in organic fluorophore for submicrosecond delayed fluorescence. Sci. Adv. 7, eabe5769. doi:10.1126/sciadv.abe5769
Cai, X., and Su, S.-J. (2018). Marching toward highly efficient, pure-blue, and stable thermally activated delayed fluorescent organic light-emitting diodes. Adv. Funct. Mater. 28, 1802558. doi:10.1002/adfm.201802558
Chen, F., Zhao, L., Wang, X., Yang, Q., Li, W., Tian, H., et al. (2021). Novel boron- and sulfur-doped polycyclic aromatic hydrocarbon as multiple resonance emitter for ultrapure blue thermally activated delayed fluorescence polymers. Sci. China. Chem. 64, 547–551. doi:10.1007/s11426-020-9944-1
Chen, Z., Wannere, C. S., Corminboeuf, C., Puchta, R., and von Ragué Schleyer, P. (2005). Nucleus-independent chemical shifts (NICS) as an aromaticity criterion. Chem. Rev. 105, 3842–3888. doi:10.1021/cr030088+
Hagai, M., Inai, N., Yasuda, T., Fujimoto, K. J., and Yanai, T. (2024). Extended theoretical modeling of reverse intersystem crossing for thermally activated delayed fluorescence materials. Sci. Adv. 10, eadk3219. doi:10.1126/sciadv.adk3219
Hatakeyama, T., Shiren, K., Nakajima, K., Nomura, S., Nakatsuka, S., Kinoshita, K., et al. (2016). Ultrapure blue thermally activated delayed fluorescence molecules: efficient HOMO–LUMO separation by the multiple resonance effect. Adv. Mater. 28, 2777–2781. doi:10.1002/adma.201505491
Hirai, H., Nakajima, K., Nakatsuka, S., Shiren, K., Ni, J., Nomura, S., et al. (2015). One-step borylation of 1,3-diaryloxybenzenes towards efficient materials for organic light-emitting diodes. Angew. Chem. Int. Ed. 54, 13581–13585. doi:10.1002/anie.201506335
Hu, Y. X., Miao, J., Hua, T., Huang, Z., Qi, Y., Zou, Y., et al. (2022). Efficient selenium-integrated TADF OLEDs with reduced roll-off. Nat. Phot. 16, 803–810. doi:10.1038/s41566-022-01083-y
Kim, H. J., and Yasuda, T. (2022). Narrowband emissive thermally activated delayed fluorescence materials. Adv. Opt. Mater. 10, 2201714. doi:10.1002/adom.202201714
Li, T.-y., Schaab, J., Djurovich, P. I., and Thompson, M. E. (2022). Toward rational design of TADF two-coordinate coinage metal complexes: understanding the relationship between natural transition orbital overlap and photophysical properties. J. Mater. Chem. C 10, 4674–4683. doi:10.1039/d2tc00163b
Liu, Y., Li, C., Ren, Z., Yan, S., and Bryce, M. R. (2018). All-organic thermally activated delayed fluorescence materials for organic light-emitting diodes. Nat. Rev. Mater. 3, 18020. doi:10.1038/natrevmats.2018.20
Nagata, M., Min, H., Watanabe, E., Fukumoto, H., Mizuhata, Y., Tokitoh, N., et al. (2021). Fused-nonacyclic multi-resonance delayed fluorescence emitter based on ladder-thiaborin exhibiting narrowband sky-blue emission with accelerated reverse intersystem crossing. Angew. Chem. Int. Ed. 60, 20280–20285. doi:10.1002/anie.202108283
Park, I. S., Min, H., and Yasuda, T. (2022a). Ultrafast triplet–singlet exciton interconversion in narrowband blue organoboron emitters doped with heavy chalcogens. Angew. Chem. Int. Ed. 61, e202205684. doi:10.1002/anie.202205684
Park, I. S., Yang, M., Shibata, H., Amanokura, N., and Yasuda, T. (2022b). Achieving ultimate narrowband and ultrapure blue organic light-emitting diodes based on polycyclo-heteraborin multi-resonance delayed-fluorescence emitters. Adv. Mater. 34, 2107951. doi:10.1002/adma.202107951
Pratik, S. M., Coropceanu, V., and Brédas, J.-L. (2022). Purely organic emitters for multiresonant thermally activated delay fluorescence: design of highly efficient sulfur and selenium derivatives. ACS Mater. Lett. 4, 440–447. doi:10.1021/acsmaterialslett.1c00809
To, W.-P., Cheng, G., Tong, G. S. M., Zhou, D., and Che, C.-M. (2020). Recent advances in metal-TADF emitters and their application in organic light-emitting diodes. Front. Chem. 8, 653. doi:10.3389/fchem.2020.00653
Uoyama, H., Goushi, K., Shizu, K., Nomura, H., and Adachi, C. (2012). Highly efficient organic light-emitting diodes from delayed fluorescence. Nature 492, 234–238. doi:10.1038/nature11687
Wada, Y., Nakagawa, H., Matsumoto, S., Wakisaka, Y., and Kaji, H. (2020). Organic light emitters exhibiting very fast reverse intersystem crossing. Nat. Phot. 14, 643–649. doi:10.1038/s41566-020-0667-0
Wong, M. Y., and Zysman-Colman, E. (2017). Purely organic thermally activated delayed fluorescence materials for organic light-emitting diodes. Adv. Mater. 29, 1605444. doi:10.1002/adma.201605444
Zhang, J., Liu, K., Li, J., Xie, Y., Li, Y., Wang, X., et al. (2021). Harnessing Se=N to develop novel fluorescent probes for visualizing the variation of endogenous hypobromous acid (HOBr) during the administration of an immunotherapeutic agent. Chem. Commun. 57, 12679–12682. doi:10.1039/D1CC04832E
Keywords: thermally activated delayed fluorescence, narrowband emission, selenaborin, spinorbit coupling, heavy atom effect, helicity, OLED
Citation: Keshri SK, Liu G and Yasuda T (2024) Ultrafast spin-flip exciton conversion and narrowband sky-blue luminescence in a fused polycyclic selenaborin emitter. Front. Chem. 12:1375552. doi: 10.3389/fchem.2024.1375552
Received: 24 January 2024; Accepted: 11 March 2024;
Published: 25 March 2024.
Edited by:
Zujin Zhao, South China University of Technology, ChinaCopyright © 2024 Keshri, Liu and Yasuda. This is an open-access article distributed under the terms of the Creative Commons Attribution License (CC BY). The use, distribution or reproduction in other forums is permitted, provided the original author(s) and the copyright owner(s) are credited and that the original publication in this journal is cited, in accordance with accepted academic practice. No use, distribution or reproduction is permitted which does not comply with these terms.
*Correspondence: Sudhir K. Keshri, a2VzaHJpQGlmcmMua3l1c2h1LXUuYWMuanA=; Takuma Yasuda, eWFzdWRhQGlmcmMua3l1c2h1LXUuYWMuanA=