- 1Department of Geology, Trinity College Dublin, College Green, Dublin, Ireland
- 2Carrera de Geología, Universidad Santo Tomás, Santiago, Chile
- 3Department of Earth Sciences, University of Geneva, Genève, Switzerland
- 4British Antarctic Survey, Cambridge, United Kingdom
- 5Antarctic and Patagonia Paleobiology Laboratory, Chilean Antarctic Institute-INACH, Punta Arenas, Chile
- 6Institut für Geologie und Paläontologie, Westfälische Wilhelms-Universität, Germany
- 7Departamento de Geología, Universidad de Chile, Santiago, Chile
- 8Institute for Environmental Sciences, University of Geneva, Geneva, Switzerland
- 9Institute of Geomechanics, Chinese Academy of Geological Sciences, Beijing, China
- 10School of Ocean Sciences, China University of Geosciences, Beijing, China
- 11Cartogrip ScRL, Sent, Switzerland
- 12Chilean Antarctic Institute-INACH, Punta Arenas, Chile
Over the last few decades, numerous geological studies have been carried out in the South Shetland Islands, which have greatly contributed to a better understanding of its geological evolution. However, few attempts have been conducted to correlate the geological units throughout this archipelago. We present herein a review of the literature available in the South Shetland Islands, which we use to propose a lithostratigraphical correlation that constitutes a coherent stratigraphy for the main Mesozoic and Cenozoic rocks of the South Shetland Islands along with a new geological map. The lithostratigraphical correlation shows that the geological and environmental evolution comprises three main stages: 1) deep marine sedimentation from ∼164 to 140 Ma, 2) subaerial volcanism and sedimentation with a proliferation of plants and fauna from ∼140 to 35 Ma and 3) glacial and interglacial deposits from ∼35 Ma. The lithostratigraphical correlation also shows a broad geographical trend of decreasing age of volcanism from southwest to northeast, which has been previously suggested. However, this spatial age trend is disrupted by the presence of Eocene magmatism in Livingston Island, located in the centre of the archipelago. We suggest that the migration of volcanism occurred from the Late Cretaceous until the early Eocene. Subsequently, enhanced magmatic activity took place from the mid-Eocene until the Miocene, which we associate with processes related with the waning of subduction. Constraining the protolith age of the metamorphic complex of Smith Island remains challenging, yet holds key implications for the tectonic and accretionary evolution of the Antarctic Peninsula. The rocks recording the glaciation of this sector of Antarctica are well exposed in the northern South Shetland Islands and hold critical information for understanding the timings and processes that lead to the greenhouse to icehouse transition at the end of the Eocene. Finally, contemporaneous rocks to the breakup of Antarctic Peninsula from Patagonia that led to the opening of the Drake Passage and the development of the Scotia Sea are exposed in the centre and north of the South Shetland archipelago. Better constraints on the age and tectonic settings on these units may lead to further understanding the paleobiogeographical evolution of the region, which may have played an important role for speciation as a land bridge between South America and Antarctica. The dataset containing the geological map and associated information is shared as a shapefile or KML file.
1 Introduction
Forearc deposits are extensively exposed in southwestern Gondwana. While they play an essential role for better understanding how the margin evolved, the Antarctic sector remains relatively locally characterised. In particular, the South Shetland Islands, located at the northwest of the Antarctic Peninsula, are dominated by forearc deposits that extend for ∼300 km in a north-eastern direction. This archipelago is separated from the Antarctic Peninsula by an active marginal basin, the Bransfield Strait (Figure 1), which opened during the Pliocene (<5 Ma) as a result of back-arc rifting (e.g., Barker, 1982). Thus, the archipelago is a recently detached block and is comprised of a late Palaeozoic metasedimentary basement (e.g., Castillo et al., 2015) overlain by Jurassic and younger magmatic and sedimentary rocks (e.g., Smellie et al., 1984; Haase et al., 2012; Bastias et al., 2019; Leat and Riley, 2021). The geology of the South Shetland Islands records evidence of Mesozoic to Cenozoic tectonic, global sea-level and climate change, including the emergence of submarine marginal basins as part of a continental island volcanic arc (e.g., Hathway and Lomas, 1998; Riley et al., 2012; Bastias et al., 2019) and the consequent proliferation of Cretaceous plant species (e.g., Philippe et al., 1995; Torres et al., 1997; 2015; Falcon-Lang and Cantrill, 2002; Leppe et al., 2007; Warny et al., 2019a). This was followed during the Eocene by the development of a mountainous landscape with stratovolcanoes and lava fields that were covered by Valdivian-type forest (Poole et al., 2001; Hunt and Poole, 2003), which are similar to wetlands and freshwater environments still present today in Chile and Argentina. Later, approaching the Eocene/Oligocene boundary, northward propagation of the Antarctic icesheet and marine transgression occurred (Baker, 2007; Davies et al., 2012), which led to glacial, glaciomarine and marine sedimentation (Troedson and Riding, 2002; Troedson and Smellie, 2002). This was followed by a period of glaciations and interglacials that are recorded at the northwestern end of the archipelago on King George Island (e.g., Birkenmajer et al., 1985; Birkenmajer, 2001; Troedson and Smellie, 2002; Whittle et al., 2014; Smellie et al., 2021a; Smellie et al., 2021b). These paleoenvironmental events are related to globally significant climate events (e.g., Kennett, 1977; Zachos et al., 2001; De Conto & Pollard, 2003), and the South Shetland Islands are one of the few regions in Antarctica where these processes are preserved in the geological record. Therefore, a better understanding of the lithostratigraphic evolution of the South Shetland Islands is key to determine the timings and extent of these events. However, after decades of research, our geological knowledge of the South Shetland archipelago is limited, with most studies concentrated on the more accessible islands (King George and Livingston islands). This poses a significant problem when regional correlations between geological units are required. Additionally, while there is a plethora of local-scale geological maps, these are mostly located near research stations and unfortunately often introduce new geological units as opposed to attempting regional correlation. The most comprehensive geological map of the South Shetland archipelago was presented in the work of Smellie et al. (1984), and now after almost four decades, significant new information is available (e.g., Bastias et al., 2019; Smellie et al., 2021a; Smellie et al., 2021b; Chen et al., 2021), which we gather and present herein.
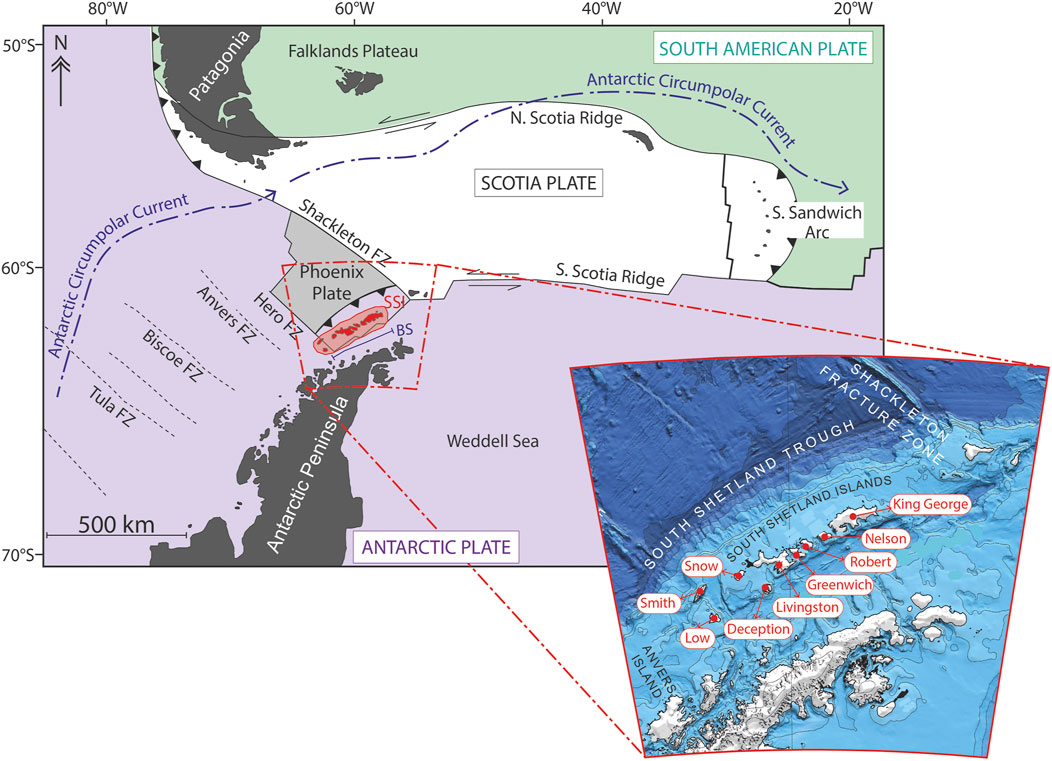
FIGURE 1. Tectonic configuration of the region encompassing the Scotia Plate, Patagonia and Antarctic Peninsula and showing the location of the South Shetland Islands and the Bransfield Strait. BS: Bransfield Strait. SSI: South Shetland Islands. Red box in inset figure shows the location of the archipelago and its main islands.
This study presents a review of the main geological units of the nine major islands of the South Shetland archipelago, which are from south to north Low, Smith, Snow, Deception, Livingston, Greenwich, Robert, Nelson and King George. In doing so, we correlate these units across the archipelago to determine changes in paleoenvironmental conditions (i.e., submarine vs. subaerial settings, the presence of glacial episodes). This exercise has allowed us to present herein a unified stratigraphy for the South Shetland Islands, which is used to show the timings and characteristics of the stages of the geological and environmental evolution of the archipelago. Additionally, this has relevant implications for better understanding the timings for the Cenozoic glaciation of Antarctica and the breakup between the Antarctic Peninsula and Patagonia.
Finally, in addition to the review and correlation of the lithostratigraphical units of the South Shetland Islands, we present a polygon shapefile containing the geology gathered herein that the reader can download and use freely. This should serve as a valuable tool for Earth and environmental sciences researchers working in the region. Although geographically the South Shetland Islands traditionally include the Elephant Islands, which are located ∼100 km further north of King George Island, this work focuses on the nine major islands located to the south. The rocks exposed in the Elephant Islands reveal a different geological evolution and thus we consider that they require an independent review.
2 Geological history
The geological evolution of the Antarctic Peninsula is dominated by an active margin setting during the late Palaeozoic, Mesozoic and Cenozoic resulting from the subduction of the Phoenix Plate beneath the Antarctic Peninsula along southwestern margin of Gondwana (e.g., Burton-Johnson and Riley, 2015; Bastias et al., 2020; Jordan et al., 2020; Bastias et al., 2021; Bastias et al., 2022). During the early Mesozoic the breakup of Gondwana occurred, which caused the separation of the Antarctic Peninsula from Patagonia and Africa by the opening of the Weddell Sea in the South Atlantic (e.g., Ghidella et al., 2002; König and Jokat, 2006). Breakup was associated with magmatism (Pankhurst et al., 2000; Riley et al., 2001; Bastias et al., 2021) and the development of volcano-sedimentary basins on the eastern (Larsen Basin; Hathway, 2000) and western flanks of the peninsula (Byers Basin; Bastias et al., 2019). With the exception of the high pressure-low temperature metamorphic accretionary complex on Smith Island (e.g., Trouw et al., 1998b), the South Shetland archipelago is comprised of Mesozoic and Cenozoic volcanic and volcaniclastic rocks (e.g., Smellie et al., 1984). While waning subduction off the South Shetland Islands and northwestern tip of the Antarctic Peninsula may still be occurring based on seismic activity (Robertson-Maurice et al., 2003), cessation of subduction along most of the margin developed progressively from south to north as a consequence of the collision of spreading ridge segments separated by transform faults (Larter and Barker, 1991). The segment of the margin where the South Shetland Islands lies is flanked by Hero and Shackleton fractures zones to the south and north, respectively (Figure 1; e.g., Galindo-Zaldívar et al., 2004). Subduction is ceasing (Lawver et al., 1995) and has resulted in the absence of arc volcanism for the last ∼20 Myr (Birkenmajer et al., 1985). Subduction-related convergence of the Phoenix Plate with the South Shetland Islands stopped at ∼3 Ma when seafloor spreading in the ridge ceased (Eagles, 2004). This has led to slab rollback and transtensional motions between the Scotia and Antarctic plates, which caused extension and the opening of the Bransfield Strait (Figure 1; Gonzalez-Casado et al., 2000; Maestro et al., 2007; Almendros et al., 2020).
3 Methodology used for the development of the geological map
The geological maps were developed through different stages, which are explained herein.
1. Data from multiple sources were used to construct the geological map presented in this work. Because our primary aim is to present regional lithostratigraphical correlations across the South Shetland archipelago, we have worked at a formation stratigraphic level of detail (Figure 2A). Presenting the information in this form allows us to observe the regional evolution of the geological units throughout the Mesozoic and Cenozoic; including more detail (e.g., members or further subdivisions of units) complicates this regional synthesis. The lithological descriptions presented in this work are mainly derived from the geological maps published by Smellie (1979); Smellie et al. (1984); Smellie et al. (2021a); Smellie et al. (2021b); Grunow et al. (1992); Li et al. (1996); Hathway and Lomas (1998); Lee et al. (2002); Machado et al. (2005); Hervé et al. (2006); Kraus and del Valle (2008); Israel (2015); Warny et al. (2015); Bastias (2014); Gao et al. (2018); Gao et al. (2021); Bastias et al. (2019); Chen et al. (2021); which are for the most part of local scale. The geological symbols employed the library presented by Frigeri (2020). Finally, it should be noted that this compilation of maps does not include all the geological information published in the South Shetlands archipelago and thus this geological compilation is presented as a starting point for further work. Occasionally, maps were not included due to 1) their local scale either in size or time or 2) conflictive stratigraphy. Hence, we consider that this material can be updated and complemented by the geoscientific community working in the northern Antarctic Peninsula, and we welcome such interaction with researchers in the future. The local geological maps of each island presented in Figure 3 throughout Figure 13.
2. Digitization of the geological maps was performed in QGIS v3.24 using the EPSG:3031—WGS 84/Antarctic Polar Stereographic projection, based on the World Geodetic System 1984 ensemble (EPSG: 6326), which has a limited accuracy of 2 m. To digitise the maps, we initially georeferenced each geological map presented in Figure 3 through to Figure 13 (Figure 2B). The coastline derived from the high-definition model of Antarctica (Gerrish et al., 2021) was used as reference guide and forms part of the data presented in the Scientific Committee on Antarctic Research, Antarctic Digital Database, accessed in May 2022.
3. Geological maps were fully digitised within a shapefile polygon type, which includes 326 different polygons (Figure 2C). This shapefile has the following fields: 1) Name, 2) Island, 3) Formation, 4) Age, 5) Environment, 6) Type, 7) Age Max, 8) Age Min and 9) Comment. This information was used to generate maps for each island, which are present in the Supplementary Material.
4. The scale of the maps presented here requires consideration—while the archipelago is more than 300 km long, the largest outcrops have dimensions of a few kilometres across. This results in local maps with (varying) scales, and therefore this work should be considered as a compilation of local geological maps, rather than a consolidated map of the archipelago as a whole.
5. Finally, the shapefile containing the geological information has been named Geology_South Shetland Islands and has been uploaded with all the relevant files for its visualisation to YARETA (https://yareta.unige.ch/home/detail/71ebfa0f-c810-45dd-b79d-036716873550; DOI: 10.26037/yareta:zcqgax5aqzgphky3nhgxlbswc4), the digital repository from the University of Geneva, which allows the reader to download these files. Additionally, a.KML file has been produced that mirrors the geological shapefile and can be visualised on the easily accessible platform Google EarthTM.
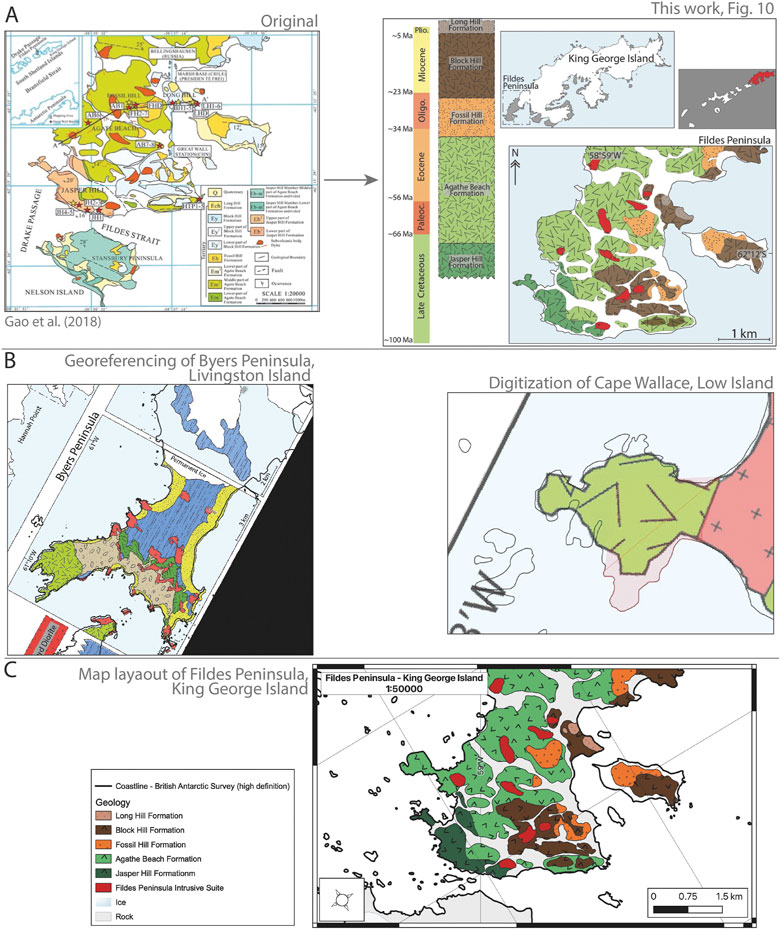
FIGURE 2. Workflow employed in this study. (A) A geological map from the literature (Gao et al., 2018). (B) The geological maps were georeferenced by using the coastline high-definition shapefile from Gerrish et al. (2021) and digitization in QGIS v3.24 of the units to create a polygon shapefile of the geological information. (C) Final map showing the geological units of Fildes Peninsula, King George Island as an example.
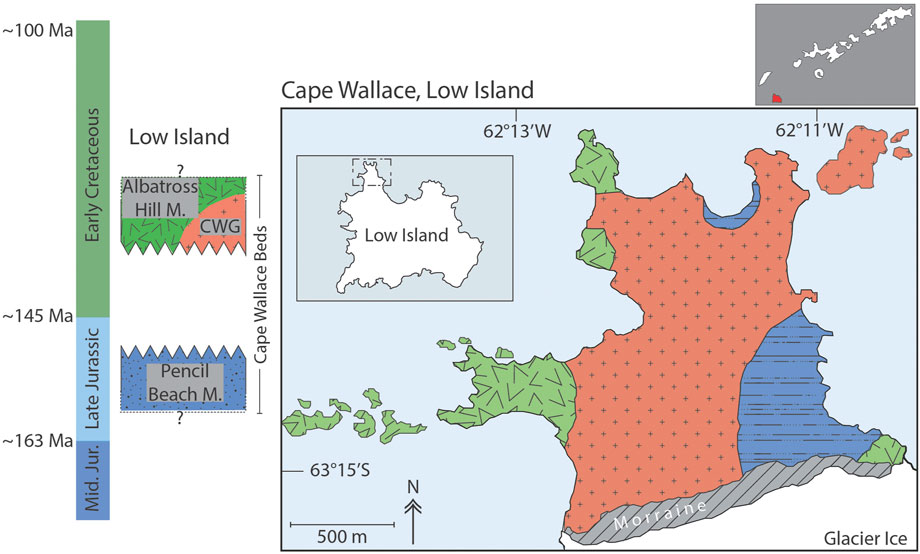
FIGURE 3. Revised geological map of Cape Wallace, Low Island, modified from Bastias (2014).
4 Geological units
The geology of the archipelago is presented here from southwest to northeast.
4.1 Low Island, Cape Wallace: Jurassic deep marine sedimentation, Early Cretaceous volcanism and Valanginian plutonism
Located at the southern end of the South Shetland Islands (Figure 1), Low Island is almost entirely covered by ice. Cape Wallace is the exception, where lies about 4 km2 of exposures (Figure 3). The exposures consist of a fine-grained sedimentary unit overlain by a volcanic succession, both of which are intruded by a granodiorite pluton (Araya and Hervé, 1966; Smellie, 1979; Bastias, 2014). Smellie (1979) first described the bedded sequence at Low Island, later redefined by Bastias and Hervé (2013) as the Cape Wallace Beds (Figure 3). Bastias et al. (2019) defined two members for the Cape Wallace Beds, the Pencil Beach and the Albatross Hill members, which are equivalent to the “sedimentary member” and “volcanic member” defined by Smellie (1979), respectively. Further details of these units are presented below.
4.1.1 Pencil Beach Member: Deep marine Jurassic sedimentation
Outcrops of the Pencil Beach Member are located in the southeast and the northeast parts of Cape Wallace (Figure 3). This sequence is well-bedded and includes fine-grained volcaniclastic claystones, crystal tuffs and lapilli stones. Individual beds are generally 4–30 cm thick, laterally uniform and continuous, and have been interpreted as turbiditic deposits (Smellie et al., 1984). Primary sedimentary structures are uncommon, except for some cross-lamination, disrupted beds, and graded bedding (Smellie, 1979). The strata strike between NW-SE and E-W, with a dip varying from sub-horizontal to 30°N. Thomson (1982) reported in-situ trace and molluscan fossils that suggest a Late Jurassic age, with an ammonite identified as Epimayites aff. Transiens (Waagen) corresponding to the Oxfordian stage. Castillo et al. (2015); Bastias (2014) investigated the provenance (using the U-Pb zircon method) of this unit and determined that the zircon detritus in the Pencil Beach Member was predominantly Permian, akin to the turbidite-like sequences in the northern Antarctic Peninsula (Carboniferous–Triassic Trinity Peninsula Group).
4.1.2 Albatross Hill Member: Subaerial Valanginian volcanism
The Albatross Hill Member unconformably overlies the Pencil Beach Member and consists of a succession of subaerial lava flows. The lava flows and associated hyaloclastites are aphyric or feldspar-phyric augite-andesites, dacites, hornblende-andesites and rare basalts (Smellie, 1979). Both lithostratigraphic units are intruded by the Early Cretaceous Cape Wallace granodiorite (Smellie, 1979; Bastias et al., 2019). Bastias et al. (2019) presented 40Ar/39Ar plateau ages of ∼140–136 Ma on the Albatross Hill Member, which constrains the minimum age of this volcanic unit.
4.1.3 Cape Wallace granodiorite: Valanginian acidic plutonism
This pluton comprises most of the outcrop on Cape Wallace Island and is a granodiorite associated with minor aplite bodies. This pluton intrudes the Pencil Beach and Albatross Hill members (Figure 3). Smellie et al. (1984) obtained a whole-rock K-Ar age of 120 ± 4 Ma, while Bastias et al. (2019) reported a refined age of 137 ± 2 Ma (U-Pb SHRIMP zircon).
4.2 Smith Island: High pressure-low temperature palaeocene–eocene metamorphism
A suite of metamorphic rocks (predominantly blueschist-greenschist facies) crops out on the most inaccessible island of the archipelago, Smith Island. The island is 40 km long and 8 km wide and has a mountainous interior (the Imeon Range) that extends along the entire length of the island and has peaks over 2 km in height. The metamorphic complex on Smith Island consists of polydeformed rocks metamorphosed up to amphibiolite and blueschist facies and has been interpreted as an accretionary complex metamorphosed during the Mesozoic (Trouw et al., 1998a). The exposures are comprised mainly of green and blue phyllites with intercalations of metachert, grey phyllite and marble (Figure 4; Dalziel, 1984; Grunow et al., 1992; Truow et al., 1998a). Valeriano et al. (1997) on the basis of chemical analyses, suggested these rocks had a mid ocean ridge basalt protolith, while hemipelagic sediments from an ocean floor environment were also present (Grunow et al., 1992; Truow et al., 1998a). While Grunow et al. (1992) presented 40Ar/39Ar ages of ∼58–47 Ma in white mica, recently Zhao et al. (2019) presented 40Ar/39Ar plateau ages of 65 ± 1 and 63 ± 1 Ma in phengite and sodic amphibole, respectively. Truow et al. (1998b) determined PT conditions of 8 kbar, 300–350°C, and Chen et al. (2021) presented metamorphic ages for the blueschist of ∼65–62 Ma and showed that it underwent a clockwise P-T evolution. Furthermore, Truow et al. (1998b) and Chen et al. (2021), interpreted these rocks as part of a subduction complex composed of ocean floor material mixed with arc-derived sediments. Bastias et al. (2022) suggested that the uplift of these rocks may have been associated with the resumption of subduction during the Cretaceous. While the Palaeocene–Eocene age of deformation has been established by Grunow et al. (1992) for these rocks, the age of its protolith remains unknown. However, considering the late Palaeozoic and Mesozoic active margin evolution of the Antarctic Peninsula, we can speculate a Palaeozoic to Mesozoic age for the protolith.
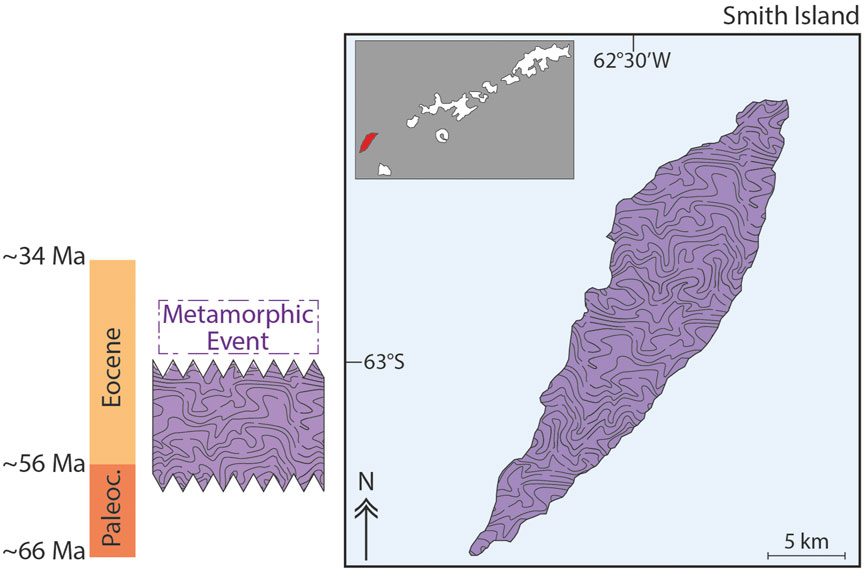
FIGURE 4. Revised geological map of Smith Island, modified from Grunow et al. (1992).
4.3 Snow Island: Early Cretaceous marine sedimentation, subaerial late Cretaceous volcanism and Eocene plutonism
The outcrops of Snow Island are mostly restricted to President Head Peninsula (Figure 5), ∼10 km south of Byers Peninsula on Livingston Island (Figure 7), although other minor shore outcrops are also present to the west and east on Snow Island. On President Head Peninsula, two distinctive units crop out—a sedimentary unit overlain by a volcaniclastic unit (Figure 5; e.g., Israel, 2015; Bastias et al., 2019). Due to their proximity and based on litho-, bio- and tectono-stratigraphic similarities, Crame et al. (1993) suggested correlations between these units and those present on the adjacent Livingston Island (Section 4.5; Figure 7). It have suggested that the marine ammonite-bearing sedimentary succession at the western end of President Head could be correlated with the Sealer Hill Member of the Chester Cone Formation of Livingston Island (Crame et al., 1993; Torres et al., 1997; Hathway and Lomas, 1998; Israel, 2015). Based on the marine invertebrate assemblages, the sedimentary succession on President Head has been assigned to the mid-Valanginian (Ugalde et al., 2013; Israel, 2015). Plant remains found in the terrestrial volcaniclastic rocks at the top of the President Head succession on Snow Island show the emergence of the arc and the abundant flora is represented by bryophyta (Muscites, Thallites), ferns as Lophosoria cupultus with spores Cyatheacidites annulatus and Gleichenites, Aculea, Sphenopteris, Pentoxylales (Taeniopteris), Bennettitales (Zamites, Ptilophyllum, Dictyozamites, Cycadolepis) and Coniferales (Araucarites, Elatocladus, Conites, Podostrobus) (Torres et al., 1997; Cantrill, 1998; Hathway and Lomas, 1998). Furthermore, Bastias et al. (2019) presented a U-Pb zircon age of 109 Ma ± 1 from the upper volcaniclastic succession at President Head, constraining its age to Aptian–Albian. This age was used to refine lithostratigraphic correlations with the units on Livingston (Section 4.5; Figure 7) and Low (Section 4.1; Figure 3) islands and to propose a common evolution within the Byers Basin.
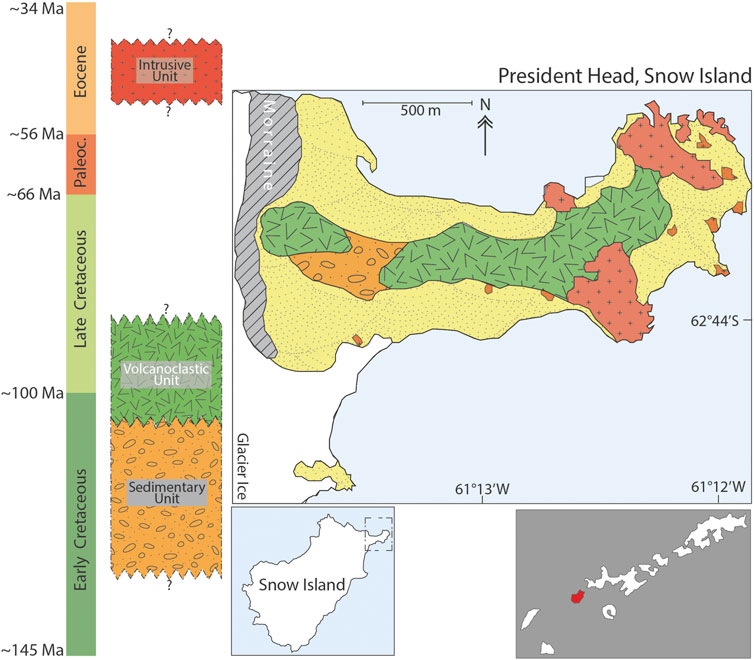
FIGURE 5. Revised geological map of the President Head Peninsula, Snow Island, modified from Israel (2015).
Intrusive bodies constitute a major part of the outcrops on President Head on Snow Island (Figure 5). They are mostly dykes, although there are other hypabyssal intrusions of basaltic to andesitic composition (e.g., Valenzuela and Hervé, 1972; Smellie, 1980; Pankhurst and Smellie, 1983) and minor columnar basalts and dacites (Pankhurst et al., 1979). The geochronological control of this intrusive unit is limited to the age obtained by Pankhurst and Smellie (1983), who reported a K-Ar age of 46 ± 2 Ma from a dacite sampled from the President Head Peninsula. Nevertheless, most of these rocks have experienced low-temperature alteration (Smellie et al., 1980) and likely the daughter isotope is disturbed, as has been shown on other islands of the archipelago (Bastias et al., 2016; Bastias et al., 2019) and thus K-Ar dates may be the result of the combination between the alteration and crystallisation age in these rocks.
4.4 Deception Island: Quaternary volcanism
This island is amongst the most active volcanoes in Antarctica with over 20 explosive eruptions recorded in the last two centuries (e.g., Smellie, 2001). It consists of a composite volcano with a basal diameter of 30 km and rising over 500 m above sea level (e.g., Luzón et al., 2011). The island has a horseshoe-shape, whose submerged central portion represents a collapsed caldera (Figure 6). The normal magnetic polarity of the rocks exposed on Deception Island indicates that the rocks exposed there are younger than 0.75 Ma (Valencio et al., 1979) while K-Ar data suggest that most of the subaerial part of the island was built in the last 0.2 Myr (Keller et al., 1992). This active volcanic island has attracted several volcanologists, which have greatly contributed to a better understanding on its age and evolution (e.g., González-Ferrán et al., 1971; Orheim, 1972; Roobol, 1982; Ibáñez et al., 2003; Almendros et al., 2018; Geyer et al., 2019) along with a detailed geological map (Smellie et al., 2002). Although a considerable amount of detailed information has been obtained on the Quaternary geology of this island, it is not useful for the purposes of small-scale regional lithostratigraphical correlations across the South Shetland archipelago.
4.5 Livingston Island
Most of the rock exposures on Livingston Island are restricted to the Byers Peninsula (Figure 7), where the Byers Group has been defined (Hathway and Lomas, 1998). The base and the top of the group are not exposed, but the overall thickness is estimated to be at least 2.7 km, consisting of 1.3 km of marine sedimentary rocks overlain by 1.4 km of non-marine strata, with a younging direction to the east. Contact relations between the formations are locally obscured by high-angle faults, folding, and intrusion of younger hypabyssal and plutonic rocks (e.g., Hathway and Lomas, 1998); others are covered by the ice cap. Hathway (1997) and Hathway and Lomas (1998) reviewed the Byers Group lithostratigraphy originally proposed by Smellie et al. (1980) and Crame et al. (1993), and is presented here in stratigraphic order.
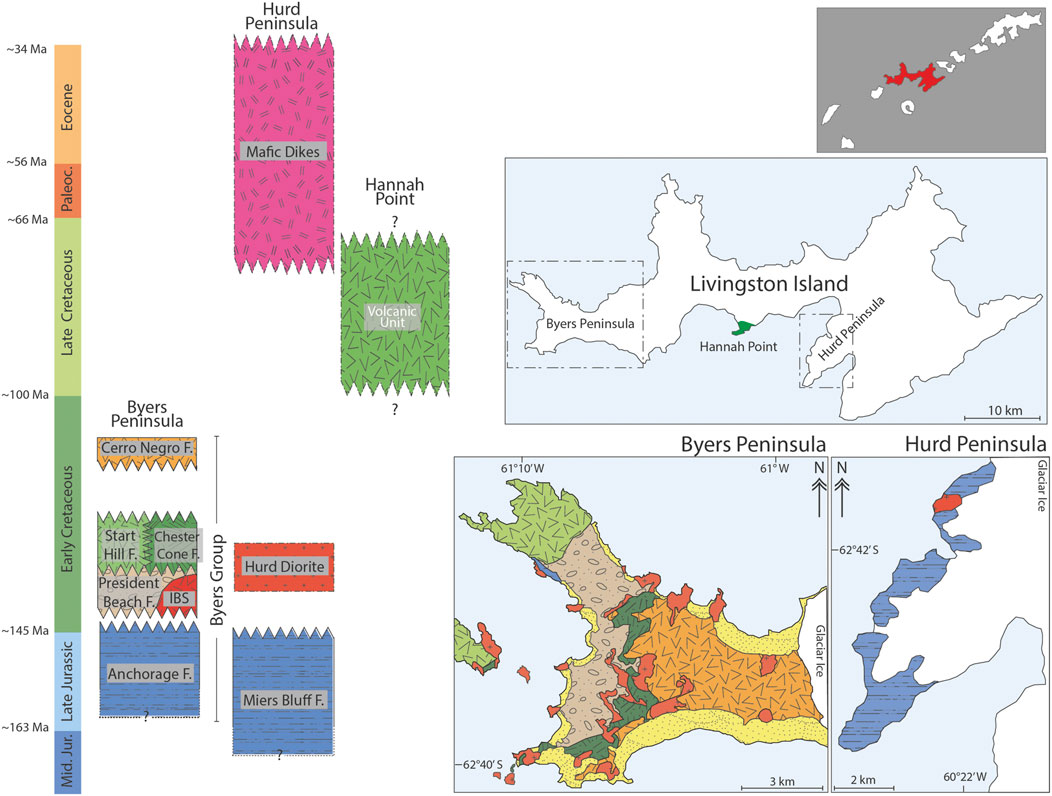
FIGURE 7. Revised geological map of Byers and Hurd peninsulas, Livingston Island, modified from Hathway and Lomas (1998), Hervé et al. (2006) and Gao et al. (2021).
4.5.1 Byers Peninsula
4.5.1.1 Anchorage Formation: Late Jurassic deep marine sedimentation
First defined by Crame et al. (1993), the outcrops of the Anchorage Formation are confined to an area of 0.5 km2 in the northwest sector of Byers Peninsula (Figure 7), where they form a homoclinal succession dipping to the north-west and are intruded by tabular microdioritic bodies. Hathway and Lomas (1998) subdivide the Anchorage Formation into the New Plymouth and Punta Ocoa members, which reach a combined thickness of about 120 m. Although the members are lithologically distinguishable and differ markedly in the degree of bioturbation, these two members are not differentiated on the map presented here. This follows the rationale presented in Deception Island, where detailed information is not useful for the purposes of regional lithostratigraphical correlations across the South Shetland archipelago. The New Plymouth Member consists mainly of mudstones that exhibit notable bioturbation and numerous silicic tuff layers, and normally graded clastic sediments that are locally bioturbated. The Punta Ocoa Member is mainly composed of radiolarian-rich shale with volcanic ash tuff layers and fine layers of massive sandstone with mudstone intraclasts (Pirrie and Crame, 1995; Hathway and Lomas, 1998). The fossil record of the Anchorage Formation suggests a Late Jurassic age (Kiessling et al., 1999). The mudstones can be mostly interpreted as deep hemipelagic deposits but some may have been deposited by minor turbidity currents. The sandstones and some tuffs are interpreted as turbidites (Pirrie and Crame, 1995) and show a limited volcaniclastic input from the Late Jurassic magmatic arc. Bastias et al. (2019) presented a zircon U-Pb age of 153 ± 2 Ma from an ash-layer of the Anchorage Formation, complementing the age control suggested by the fossil record of this unit.
4.5.1.2 President Beaches Formation: Berriasian (∼145–140 Ma) marine fan sedimentation
The President Beaches Formation was defined by Crame et al. (1993) and redefined by Hathway and Lomas (1998), and crops out over an area extending 0.5–1.5 km inland from Byers Peninsula and has an estimated thickness of at least 600 m (Figure 7). It overlies the Anchorage Formation in apparent unconformity. The President Beaches Formation consists mainly of dark grey shales with pyrite, radiolarians and isolated trace fossils. There also minor units of yellowish clay-rich strata 0.5–4 cm thick (interpreted as altered tuffs), layers of grey-green sandstones and centimetric carbonate concretions. There are also lenticular bodies of sandstone with a thickness of 8–45 m, with small-scale syn-sedimentary deformation (load structures, flame structures, convolute lamination, clastic dykes) likely caused by the submarine relief and high sedimentation rates (Lomas, 1999). Hathway and Lomas (1998) proposed that the President Beaches Formation was probably deposited on a submarine slope fan below the base level of storm waves, where mud deposition was sporadic and locally interrupted by sandy turbidite flows confined to small channels. The source region may have been a volcanic arc with vegetation located to the southeast (Lomas, 1999). The rocks contain macrofossils of Berriasian affinity, including ammonites (Spiticeras, Blanfordiceras, Himalayites, Bochianites; Smellie et al., 1980), bivalves (Manticula Waterhouse, Praeaucellina, Inoceramus; Crame et al., 1993) and belemnites (Smellie et al., 1980; Crame et al., 1993; Pirrie and Crame, 1995). Duane (1994), Duane (1996) further described the presence of dinoflagellate assemblages that would indicate a mid-to-late Berriasian age (∼145 Ma). This formation does not have any reported geochronological control.
4.5.1.3 Start Hill Formation: Valanginian (∼140–133 Ma) shallow marine fan sedimentation and basaltic subaerial volcanism
The Start Hill Formation was defined by Hathway and Lomas (1998), and has an estimated thickness of at least 265 m. The top is unknown, while its base is well defined and is in apparent discordant contact with the mudstones of the President Beaches Formation (Figure 7; Valenzuela and Hervé, 1972; Smellie et al., 1980). The formation is mainly composed of massive, poorly sorted volcanic breccias and both clast- and matrix-supported conglomerates that form very thick strata (5–30 m). The clasts, mostly smaller than 30 cm but up to 3 m in diameter and generally angular, are of basalt and basaltic andesite composition with porphyritic and amygdaloidal textures. The contacts between stratal packages are mainly gradational with pronounced lateral variation. There are also geographically restricted volcanic agglomerates of grey and brown, finely-laminated lapilli tuffs and lavas. Combined with the presence of a molluscan assemblage dominated by oyster shell fragments (Smellie et al., 1980), this formation has been interpreted as part of a fan/apron of submarine debris that surrounded one or more basaltic subaerial volcanoes. While Hathway and Lomas (1998) assigned a Berriasian age (∼145–140 Ma), Haase et al. (2012) suggested a Valanginian age based on 40Ar/39Ar dating of aphyric lavas that yielded an age of 135 ± 3 Ma.
4.5.1.4 Chester Cone Formation: Valanginian (∼140–133 Ma) marine-continental transitional sedimentation and volcanism
The Chester Cone Formation was defined by Crame et al. (1993) and redefined by Hathway and Lomas (1998), who divided it into two members: the basal Devils Point Member, previously included at the top of the Anchorage Formation by Crame et al. (1993), and an upper Sealer Hill member. We however do not separate this formation into two units on the geological map (Figure 7), with the aim of maintaining consistency on the level of detail presented in the maps. The Devils Point Member is wedge-shaped with an estimated thickness of 300 m and has been affected by numerous faults. Its base is composed of matrix-to clast-supported pebble conglomerates, of mottled appearance and grey-orange colour, with well-rounded clasts of basaltic to intermediate volcanic rocks and beige-grey mudstone intraclasts. The upper part consists of conglomerates of gravel and thick greyish sandstones with fossil remains. The fossil content is dominated by thick-shelled bivalves that appear to be reworked (Crame et al., 1993), probably by turbidity currents that remobilised coastal gravels (Duane, 1996). The Devils Point Member is intruded by the Chester Cone andesite plug, for which Pankhurst et al. (1979) obtained a Valanginian K-Ar amphibole age of 132 ± 4 Ma. The contact of the Sealer Hill Member with the Devils Point Member is gradational (Crame et al., 1993; Hathway, 1997; Hathway and Lomas, 1998). The Sealer Hill Member consists of dark, finely laminated, fairly bioturbated shales, millimetric to centimetric layers of fine-grained graded sandstone that sometimes reach greater thicknesses and exhibit parallel to wavy low-angle stratification, and rare 1-m thick strata of massive and poorly sorted conglomerates, with pebble-size basaltic clasts in a gravel-to-sand matrix. The shales might be low-energy suspension deposits while the sandstones and conglomerates might be the product of storm deposits (Hathway and Lomas, 1998). An ammonite assemblage with species of Bochianites, Uhligites and Neocomites, indicates a Valanginian age (Covacevich, 1976), while belemnites (Belemnopsis (Belemnopsis) alexandri Willey and B. B.) gladiatoris Willey (Crame et al., 1993), plants and bivalves are also present. Duane (1996), by means of palynology, defined the maximum age as Valanginian.
4.5.1.5 Cerro Negro Formation: Late Early Cretaceous volcanism
The Cerro Negro Formation (Hathway, 1997) encompasses all the continental volcaniclastic strata exposed on the eastern side of Byers Peninsula (Figure 7). It has an approximate thickness of 1.4 km, measured in a composite type section, and dips gently to the ENE. The top is obscured under the present ice cap. Hathway (1997) informally divided the Cerro Negro Formation into two members separated by an abrupt and striking colour change, which may represent a diagenetic front rather than a primary stratigraphic colour change. The first member consists of 200–240 m of silicic volcaniclastic rocks of pale green to grey colour (a weathering effect), and silicic welded or non-welded ignimbrites intercalated with reworked and silicic pyroclastic material. The second member is composed of lapilli tuffs and tuff breccias, mostly syn-eruptive and of a dark red–purple colour (due to the predominance of basaltic rocks). The lapilli tuffs and tuff breccias are interpreted as debris flows and flood flows, respectively. Changes in thickness and facies support a syn-sedimentary displacement along normal and south-vergent ENE-trending faults (Hathway, 1997). These rocks contain abundant macro- and micro-fossil vegetation of various plant groups of late Aptian age. Microfossils are represented by the spore species (Muricingulisporites annulatus, Sotasporites elegans, S. triangularis) and the macrofossils show the presence of new species of tree ferns (Alienopteris livingstonensis, Millerocaulis tekellili) and silicified gymnosperm trunks (Araucarioxylon, Podocarpoxylon and Sahnioxylon) (Duane, 1996; Torres et al., 1997; Cantrill, 2000; Falcon-lang and Cantrill, 2002; Vera, 2010; Vera, 2012; Santos et al., 2022). 40Ar/39Ar analysis of plagioclase from a basal tuff yielded an age of 120.3 ± 2.2 Ma (Hathway, 1997; Hathway et al., 1999). Ignimbritic clasts in a conglomerate unit 140 m below the top of the succession yielded 40Ar/39Ar ages of 119.4 ± 0.6 Ma and 119.1 ± 0.8 Ma on biotite and plagioclase, respectively (Hathway et al., 1999), suggesting rapid deposition during the latest Aptian. Many younger K-Ar ages have been obtained from the upper part of the Cerro Negro Formation including the intrusive rocks at Negro Hill, ranging from ∼110 Ma to 80 Ma (Pankhurst et al., 1979; Smellie et al., 1984). Hathway (1997) and Hathway and Lomas (1998) consider the possibility of placing the Cerro Negro Formation in a stratigraphic framework outside the Byers Group, and thus denote its current status within the Byers Group as provisional. While we acknowledge that better constraints on the age and geochemical affinity of these rocks may rule out its inclusion, we retain the unit in the Byers Group, considering the current international stratigraphic conventions.
4.5.2 Late Cretaceous volcanism in Hannah Point
Volcanic rocks are widespread on the central-east side of Livingston Island, such as at Hannah Point (south-central coast of Livingston Island, Figure 7). Pallàs et al. (1999) recognised five members within this 500 m thick succession, which from base to top are represented by: 1) 120 m of polymictic volcaniclastic breccias, 2) 70 m of volcaniclastic breccias, 3) 65 m of basaltic lavas, 4) 65 m of volcaniclastic breccias and 5) 150 m of andesitic lavas, suggesting that this succession was emplaced as pyroclastic flows associated with explosive volcanic activity in a subaerial environment. The age of the succession has been determined by a combination of palaeontology and geochronology. On the basis of leaf imprints consisting of ferns (Adiantites, Matonia jeffersonii, Gleichenites sanmartinii, Coniopteris, Sphenopteris, Cladophlebis antarctica and C. oblonga), pteridosperms (Ptilophyllum, Podozamites, Pachypteris), gymnosperms (Elatocladus and Brachyphyllum) and fossil tree trunks, Leppe et al. (2007) suggested a Late Cretaceous age. This was confirmed by Haase et al. (2012) based on 40Ar/39Ar whole rock dating which yielded an age of 98 ± 1 Ma. Later, Bastias et al. (2016) studied the ubiquitous presence of low-temperature mineral associations in Hannah Point and attributed these assemblages to a combination of burial and hydrothermal alteration.
4.5.3 Hurd Peninsula: Jurassic marine sedimentation, Cretaceous acidic plutonism and palaeogene hypabyssal mafic plutonism
Located to the southeast of Livingston Island, the Hurd Peninsula hosts the Miers Bluff Formation, which is interpreted as a polyphase deformed and metamorphosed turbidite sequence (Hobbs, 1968; Smellie et al., 1984; Willan et al., 1994) unconformably overlain by Cretaceous volcanic strata (Figure 7; Caminos et al., 1973; Smellie et al., 1984; Smellie et al., 1995). These units are cut by widespread mafic dyke swarms (Zheng et al., 2003). The age of this succession was assigned to the Middle to Late Jurassic based on provenance studies and an Early Cretaceous age derived from U-Pb SHRIMP zircon age dating of a granodiorite pluton (138 ± 1 Ma; Hervé et al., 2006) and calcareous nannoplankton fossils (Pimpirev et al., 2002; Pimpirev et al., 2006). This formation has been proposed to be deposited in a multiple source, gravel-rich, deep-sea ramp system and was deposited in a strike-slip setting (Muñoz et al., 1992). Bastias et al. (2019) used the granodiorite age (138 ± 1 Ma) to suggest the presence of an Early Cretaceous arc-related belt of plutons on the South Shetland Islands. Finally, Zheng et al. (2003) based on K-Ar and 40Ar/39Ar ages, suggested that the mafic dyke swarm was emplaced from ∼79 to 31 Ma.
4.6 Greenwich Island, Fort Point: Late Cretaceous volcanism and plutonism
The Fort Point exposures crop out on the east coast of Greenwich Island (Figure 8), and are comprised of volcanic rocks (basalts, basaltic andesites, and andesites) and plutonic rocks (granites, tonalites, diorites and gabbros) (e.g., Machado et al., 2005). Grikurov et al. (1970) reported a ∼105 Ma K-Ar age on a tonalite from the central part of the island (uncertainty was not reported), while Smellie et al. (1984) documented a K-Ar age of 80 ± 2 Ma for a basalt sill.
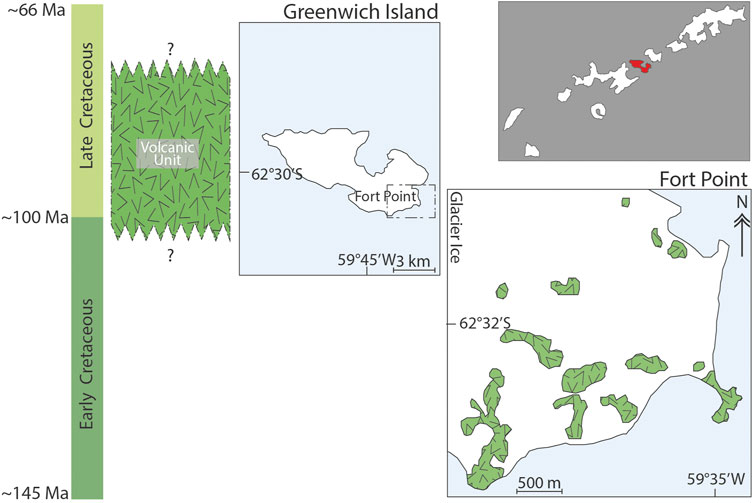
FIGURE 8. Geological map of Fort Point, Greenwich Island, from Machado et al. (2005).
4.7 Robert Island, Coppermine Peninsula: Late Cretaceous volcanism
Located within the central part of the archipelago, Robert Island is 18 km long and 18 km wide, and is mostly covered by an ice-cap (Figure 9). While some regions along the coastline have rock exposures, the largest outcrop is located on the Coppermine Peninsula on the southwest coast (Figure 9). These rocks form part of the Coppermine Formation, which is dominated by basalts and andesitic-basaltic agglomerates (e.g., Caballero and Fourcarde, 1959; Hervé, 1965; González-Ferran and Karsui, 1970). Smellie et al. (1984) reported K-Ar ages of ∼83 to 78 Ma from the Coppermine Formation. Recently, Villanueva (2021) undertook a compilation study of the geology of Coppermine Peninsula and reported 40Ar/39Ar plateau ages between∼81 and 62 Ma.
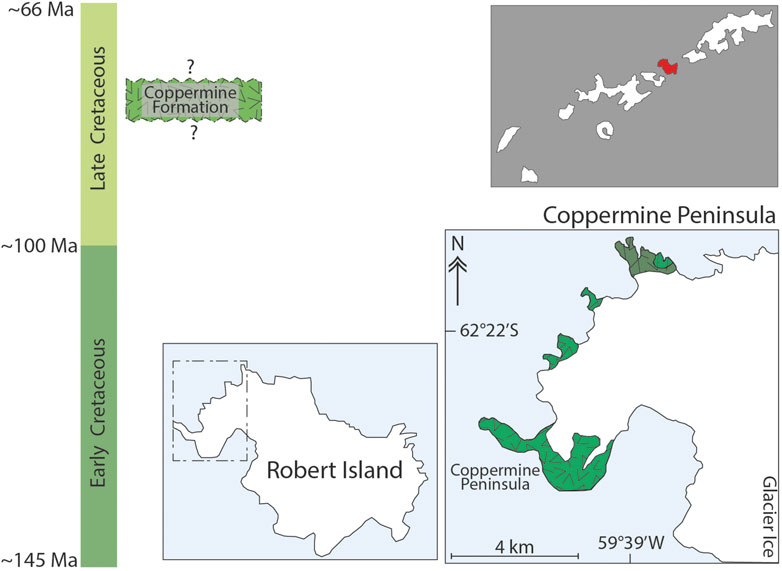
FIGURE 9. Geological map of Coppermine Peninsula, Robert Island, from Machado et al. (2005).
4.8 King George and Nelson islands
Nelson and King George islands are only separated by a narrow channel ∼500 m wide and share a common geological evolution. King George Island is the largest of the South Shetland Islands and given its relative ease of access and proximity to research stations, multiple geological studies have been carried out. This has resulted in a complex stratigraphy that has been supported mainly by poorly constrained K-Ar geochronology (e.g., Leat and Riley, 2021). The exposures in King George Island are dominated by Cenozoic volcanic and sedimentary strata (e.g., Birkenmajer and Zastawniak, 1989; Dutra and Batten, 2000; Poole et al., 2001; Fontes and Dutra, 2010; Warny et al., 2019b; Smellie et al., 2021a; Smellie et al., 2021b; Chen et al., 2021). These exposures (particularly those in northeast King George Island) are relevant because they provide a record of Antarctica’s glacial history, and have been used to suggest episodes of late Cenozoic glaciation (e.g., Warny et al., 2019a; Smellie et al., 2021a; Smellie et al., 2021b). Additionally, the presence of Late Cretaceous rocks has been indicated based on analyses of taphoflora, which are preserved in Fildes Peninsula on King George Island and Rip Point on Nelson Island (Birkenmajer, 1981; Smellie et al., 1984; Li et al., 1989; Cao, 1994; Shen, 1994; Dutra & Batten, 2000). The taphoflora preserved in these deposits consists of an assemblage of carbonized wood, leaves, pollens and spores (Manfroi et al., 2015; Manfroi et al., 2022), they are dominantly pteridophytes (as Dicksoniacea and Cyatheaceae), gymnosperms (as Araucariacites and Cycadopite) and angiosperms (as Nothofagites and Melastomataceae) (Dutra, 1997; Francis, 1999; Bastos et al., 2013; Trevisan et al., 2022).
4.8.1 Nelson Island and Fildes Peninsula: Late Cretaceous to palaeocene volcanism and sedimentation
Situated in southwestern King George Island, the bedrock geology of the Fildes Peninsula (Figure 10) consists of a suite of subalkaline volcanic, plutonic and volcaniclastic rocks (Zheng et al., 1991). Early work indicated the presence of an abundance of volcanic rocks with Cenozoic and Mesozoic fauna and plants (Hawkes, 1961; Barton, 1965). Smellie et al. (1984) placed the rocks of the peninsula into the Fildes Peninsula Formation, comprised of three members: 1) a lower member of coarse porphyritic basalt and basaltic andesite interbedded with laterally discontinuous volcanic rocks, 2) a middle member of volcaniclastic rocks with minor basalt and basaltic andesite lavas and 3) and an upper member of fine-grained aphyric and microporphyritic andesite and dacite lavas. Furthermore, Smellie et al. (1984) reported K-Ar whole-rock ages between ∼58 and 42 Ma. Later; Machado (1997) separated these rocks into four formations from bottom to top: 1) the Clement Hill Formation, composed of basalt and andesites interbedded with polymictic volcanic breccias, 2) the Fildes Strait Formation comprised of trachybasalts and porphyritic basalts associated with volcanic breccias, 3) the Schneider Bay Formation composed of porphyric basalts, andesites and dacites interbedded with breccias and 4) the Winkel Point Formation, which is comprised of basalts and basaltic andesites interbedded with volcanic breccias, agglomerates, conglomerates and tuffs.
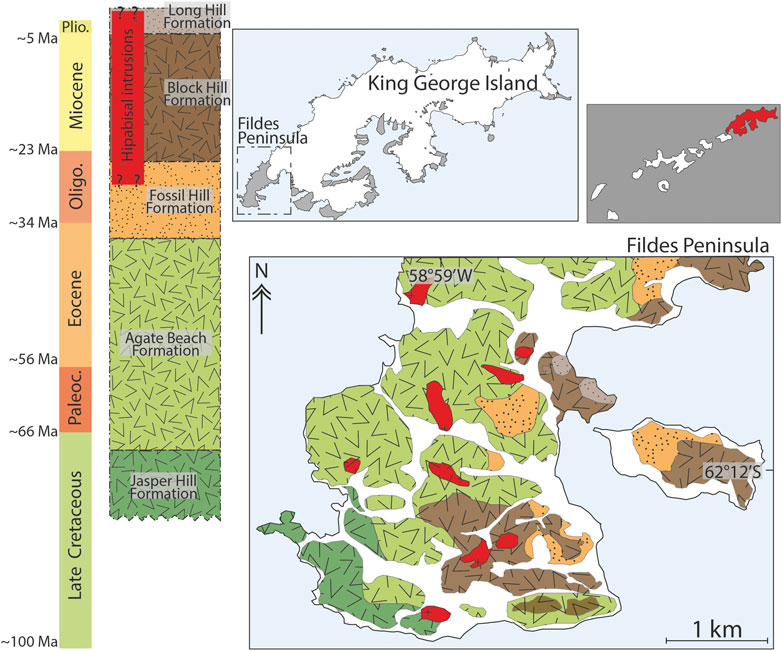
FIGURE 10. Geological map of the Fildes Peninsula, King George Island, modified from Li et al. (1996).
Other workers have developed a different stratigraphy for Fildes Peninsula (Li et al., 1992; Li et al., 1996; Li and Liu, 1987; Liu and Zheng, 1988; Zheng et al., 1991; Gao et al., 2018). These studies have divided the geology of the peninsula into five formations which are the Jasper Hill, Agate Beach, Fossil Hill, Block Hill and Long Hill formations, and this is the stratigraphic nomenclature we adopt here, which we consider has the better age constraint. The basal Jasper Formation is mostly exposed on the southwestern tip of the Fildes Peninsula and is composed of basaltic and basalt-andesitic lavas and breccias. The overlying Agate Beach Formation consists of amygdaloidal basalt, basalt-andesitic lavas and volcanic breccias. The Fossil Hill Formation overlies the Agate Beach Formation and consists of volcanic breccia, tuff and pyroclastic-sedimentary rocks intercalated with several fossiliferous layers. Fossils of middle Eocene age found in the Fossil Hill Formation include pollen spores, plant leaves and stems and bird footprints (Li and Shen, 1990; Li, 1994; Song, 1998; Mansilla et al., 2012; Mansilla et al., 2013). The overlying Block Hill Formation is exposed on the east of the Fildes Peninsula and consists of basaltic andesite, breccia and agglomerate. Finally, the Long Hill Formation is restricted to the eastern part of the peninsula, and consists predominantly of basaltic and basalt-andesitic subvolcanic rocks. The reliability of the geochronological results presented across the South Shetland Islands is affected by the ubiquitous mineral alteration of the volcanic rocks on the archipelago (e.g., Bastias et al., 2016). However, Gao et al. (2018) compiled and updated the geochronological information of Fildes Peninsula to slightly modify the stratigraphy presented by Li et al. (1996), which is presented herein and can be used to construct a succession of subaerial volcanic and volcaniclastic strata of Late Cretaceous to Pliocene age.
The geological history of Nelson Island shares close similarities with King George Island, particularly with the Fildes Peninsula, which is separated from Nelson Island by a narrow strait only ∼500 m wide. Li et al. (1996) presented a common lithostratigraphy, indicating that the units exposed in Nelson Island correspond to the Jasper Hill and Fossil Hill formations. However, it is not clear why Agathe Beach formation is not present, which should be stratigraphically between these two units. Regardless, the geology is presented in Figure 11.
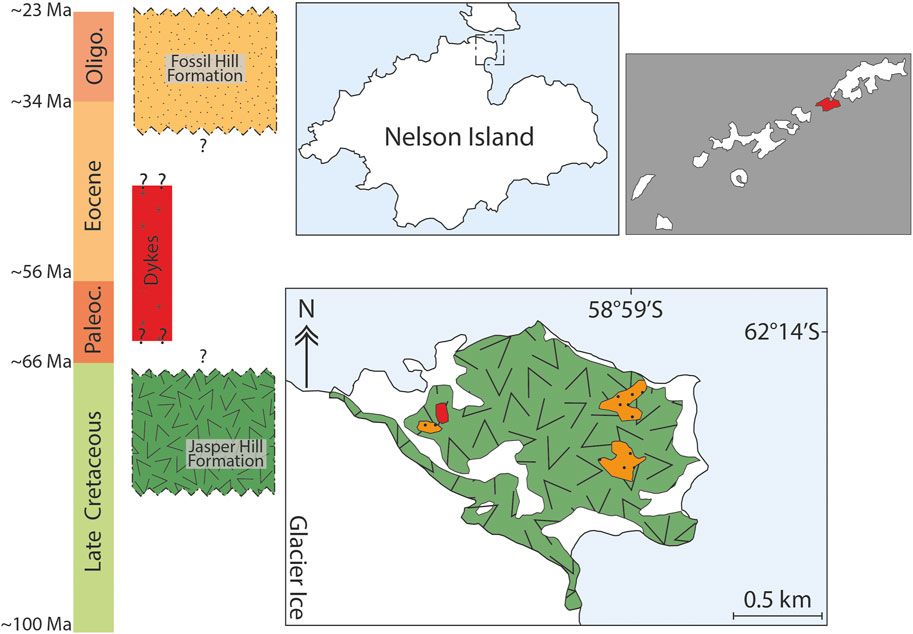
FIGURE 11. Geological map of northern Nelson Island, modified from Li et al. (1996).
4.8.2 Barton Peninsula: Palaeocene to Eocene volcanism and Eocene plutonism
The Barton Peninsula is dominated by volcanic and plutonic rocks (Figure 12). The exposures of the volcanic rocks cover most of the peninsula and range in composition from basalt to andesite (e.g., Hwang et al., 2011). The Sejong Formation crops out along the southern coast of the peninsula (Figure 12; e.g., Yoo et al., 2001). This formation is largely composed of lapilli tuffs and volcanic breccias with a maximum thickness of 100 m and gently dip to the south/southwest. Overlying the Sejong Formation are basic to intermediate volcanic lavas, which are widespread on the peninsula and range from basalt to andesite in composition (e.g., Hwang et al., 2011). Although the presence of alteration minerals prevents constraining the precise age of the lavas (Davies, 1982; Armstrong, 1995; So et al., 1995; Hur et al., 2001; Willan and Armstrong, 2002; Hwang et al., 2011), most of the lavas appear to have erupted during the Palaeocene to Eocene (Park, 1989; Kim et al., 2000; Willan and Armstrong, 2002; Yeo et al., 2004). Fossil plant fragments are contained within a fine-grained tuffaceous bed at Barton Peninsula (Tokarski et al., 1987). Willan and Armstrong (2002) described debris-flow deposits, lacustrine sediments and welded tuffs including eutaxitic rhyolitic welded tuff, probably an ignimbrite, interbedded with lavas at Barton Peninsula. A ca. 1.25 km-diameter caldera may be defined by the arcuate Noel Hill intrusion. Wang et al. (2009) dated two lavas from Barton Peninsula by Ar/Ar geochronology yielding preferred isochron ages of 46 ± 2 and 44 ± 2 Ma, consistent with the age presented by Kim et al. (2000) from a fine-grained diorite (40Ar/39Ar age of 49 ± 2 Ma). Calc-alkaline granodiorite and diorite plutons intrude the volcanic rocks in the northern Barton Peninsula, which have been interpreted to be mid-Eocene in age (Park, 1989; Lee et al., 1996; Kim et al., 2000). Furthermore, Kim et al. (2000) reported a 40Ar/39Ar age of ∼49 ± 2 Ma from a fine-grained diorite.
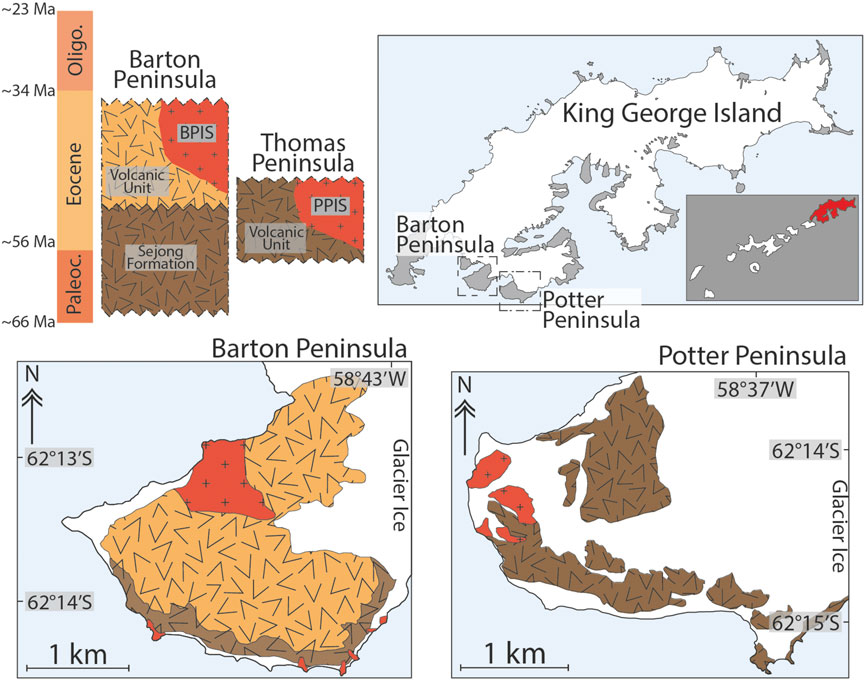
FIGURE 12. Geological map of Barton and Potter peninsulas, King George Island, modified from Kraus and del Valle (2008) and Lee et al. (2002).
4.8.3 Potter Peninsula: Palaeocene to Eocene volcanism and Early Eocene plutonism
The peninsula is dominated by a volcanic sequence with an estimated thickness of ∼500 m (Figure 12; Leat and Riley, 2021). Kraus (2005) reported that the volcanic sequence on the Potter Peninsula is comprised of volcanic centres composed of lava flows, pyroclastic rocks (ash-fallout, pyroclastic flow deposits, volcanic breccia and agglomerates) and hypabyssal intrusions (dykes and sills). These rocks range from basaltic to andesitic in composition and often exhibit columnar jointing. Early K-Ar ages have been reported from Potter Peninsula and range between ∼58 and 42 Ma (Watts, 1982; Smellie et al., 1984), which agree with the stratigraphic interpretation of Kraus (2005) and the Ar/Ar plateau age of 48 ± 1 Ma of a mafic lava from Potter Peninsula (Haase et al., 2012). However, evidence from Kim et al. (2000) and Zheng et al. (2000) indicate that the Eocene ages may be reset as a result of local intrusive events and the volcanic sequences may be mid-Cretaceous in age.
4.8.4 Admiralty Bay and Polonez Cove areas
While the Eocene and Oligocene rocks appear in several small outcrops in west-centre King George Island (Smellie et al., 2021a), here we show the two main exposures, which are located in Admiralty Bay and Polonez Cove (Figure 13). We have used the lithostratigraphy presented by Smellie et al. (2021a), who revised the conflictive models presented by former contributions (e.g., Barton, 1965; Birkenmajer, 1981; Birkenmajer, 1982; Birkenmajer et al., 1985; Birkenmajer and Zastawniak, 1989; Smellie et al., 1984; Kraus, 2005; Panczyket al., 2009; Panczyk & Nawrocki, 2011; Nawrocki et al., 2011) on the base of geochemistry and 40Ar/39Ar geochronology. Nevertheless, further local maps are presented in Smellie et al. (2021a), with a thorough review of the late Cenozoic rocks shown in eastern King George Island.
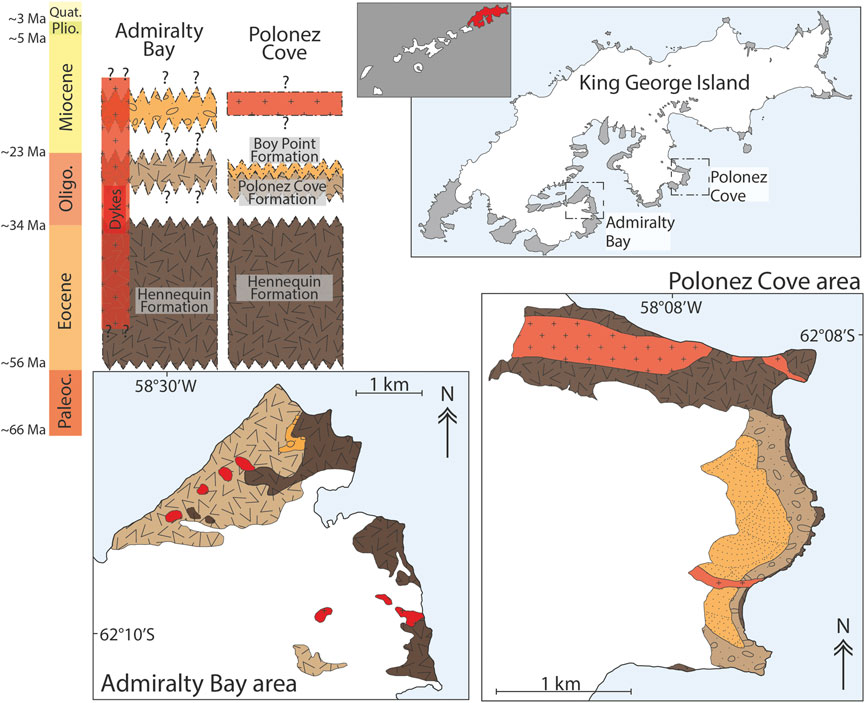
FIGURE 13. Geological map of Admiralty Bay and Polonez Cove areas modified from Smellie et al. (1984); Smellie et al. (2021a).
Admiralty Bay is located in the east of King George Island (Figure 13) and its exposures are dominated by the Hennequin Formation and more recent clastic and lavas rocks. While the Hennequin Formation is constrained by basaltic andesites, andesite lavas and amygdaloidal breccias, with minor presence of rhyolites (Smellie et al., 1984), Smellie et al. (2021a) suggested that these rocks, along with other Eocene strata, extensively crop out in eastern King George Island.
Polonez Cove is located in central-west King George Island (Figure 13); its exposures are dominated by the Chopin Ridge Group (Birkenmajer, 1980). This group of rocks include porphyritic lava flows and pyroclastics, marine tillites, associated glaciogenic sediments and sandstones. The rocks cropping out near Polonez Cove along with other minor exposures have been extensively reviewed by Smellie et al. (2021b), and only a summary is presented here to facilitate comparison with the other units in the archipelago.
In the Polonez Cove region, three formations are recognised, Hennequin (also called Mazurek Point; Panczyk & Nawrocki 2011), Polonez Cove and Boy Point formations (Figure 13; Smellie et al., 2021a; Smellie et al., 2021b). The Hennequin Formation is a subaerial volcanic sequence of Eocene age. While Birkenmajer (1982) considered this unit as part of a larger marine-related group (Chopin Ridge Group, Smellie et al. (2021a); Smellie et al. (2021b) suggested to not include this unit within that group considering its different age, features and origin. The Polonez Cove Formation is perhaps the most intensively investigated unit in the archipelago due to its association with an important glacial episode (Polonez Glaciation; e.g., Barton, 1965; Birkenmajer, 1980; Birkenmajer, 1982; Birkenmajer, 1987; Birkenmajer, 1995; Smellie et al., 1998; Smellie et al., 2021b; Troedson and Smellie, 2002; Quaglio et al., 2008; 2014; Warny et al., 2019b; Nawrocki et al., 2021a). The formation is exposed in a 2 km-long cliff along with other exposures in the area. It is dominated by sandstones, conglomerates with abundant fossils and clear glacial features such as tillites, striations and roche moutonée landforms (Birkenmajer, 1995). These rocks are intercalated with volcanic units consisting of lava-fed deltas and breccias formed during submarine extrusion (Smellie et al., 1998). Provenance studies have suggested that this formation sourced material from the Antarctic Peninsula and the interior of Antarctica in the Ellsworth Mountains (Nawrocki et al., 2021b), The depositional environment ranges from deep to shallow water conditions with a dominant mafic volcanic detrital component (Smellie et al., 2021b). Although fossil material is abundant, represented by marine invertebrates including representatives of Brachiopoda (Neothyris and Liothyrella), chinode (Adamussium and Limatula), bryozoa, polychaeta and chinodermata, much of it has been reworked from older strata (Smellie et al., 2021b), and suggests an imprecise Oligocene palaeontological age (Gazdzicki and Pugaczewska 1984; Gaździcka and Gaździcki 1985; Birkenmajer and Gaździcki 1986; Quaglio et al., 2008). Ages on shell material derived from the global 87Sr/86Sr marine curve suggest an age of ∼29–28 Ma (Dingle et al., 1997; Dingle and Lavelle, 1998); however alteration is widespread and these correlations with the global 87Sr/86Sr marine curve should be treated with caution. Recently, Smellie et al. (2021b), presented new 40Ar/39Ar data on the Polonez Cove Formation, which yield plateau ages between ∼28 and 26 Ma also suggesting an Oligocene age. Overlying the Polonez Cove Formation is the Boy Point Formation, composed of ∼150 to 100 m of felsic-intermediate lavas and agglomerates, conglomerates and sandstones (Birkenmajer, 1982; Birkenmajer, 2001; Smellie et al., 2021b). This unit was formally divided into two different formations (Birkenmajer, 1982; Birkenmajer, 2001) and has been redefined into one formation by Smellie et al. (2021b). While the formation is dominantly composed of lavas, it is interbedded with volcanic-sourced sediments which are mostly comprised of sandstones and conglomerates (Smellie J. L. et al., 2021). Recently, Smellie et al. (2021b) presented new 40Ar/39Ar data on the Boy Point Formation, which yield an age between ∼27 and 25 Ma. This suggests that there is no significant time gap between the Polonez Cove and the Boy Point formations and deposition may have been essentially continuous (Smellie et al., 2021b).
4.8.5 Melville Peninsula
Melville Peninsula is located in northeast King George Island. The rock exposures in the peninsula are comprised of the Sherratt Bay, Destruction Bay and Cape Melville formations along with the recent volcanism associated with the Melville Peak volcano (Figure 14; e.g., Troedson and Smellie, 2002; Warny et al., 2015). The Sherratt Bay Formation represents an andesitic-basaltic succession, which crops out on the eastern edge of the Melville Peninsula. Smellie et al. (2021b) suggested to reconsider this unit as an intrusive complex considering its features. Nevertheless, a stratigraphic hiatus separates the Sherratt Bay Formation and the overlying fossiliferous Oligocene Destruction Bay Formation (Birkenmajer et al., 1985). The Destruction Bay Formation consists of a ∼100–40 m thick succession of volcaniclastic material with horizons rich in marine invertebrates (Birkenmajer et al., 1985). Overlying this formation is the Cape Melville Formation, which is composed of glacio-marine sediments including sandstones, conglomerates, clay-shales and silty shales with occasional ice-rafted dropstones (Birkenmajer et al., 1985; Troedson and Smellie, 2002). The dropstones often show glacial striae and glacially polished facets, thus giving primary evidence for a continental ice-sheet presence in Antarctica (e.g., Birkenmajer et al., 1985; Troedson and Smellie, 2002; Warny et al., 2015). Similar to the Polonez Cave Formation, the Cape Melvile Formation records a glacial event and thus it is one of the most studied geological units on King George Island. The palaeontological record of the Cape Melville formation shows the presence of ophiuroids, crinoids and brachiopods, dominated by infaunal bivalves, with a significant component of decapods (Whittle et al., 2014). Furthermore, this glacial formation has been correlated with the Mi-1 glaciation (e.g., Warny et al., 2015), an event that followed the retreat of the icecap established during the Eocene-Oligocene by brief periods of interglaciation (e.g., Liebrand et al., 2011). Recently, Smellie et al. (2021b), presented an 40Ar/39Ar age of ∼22–21 Ma for a tuff from the Cape Melville Formation. The formations on the Melville Peninsula are cut by a swarm of Miocene basaltic dykes (∼20 Ma; Birkenmajer et al., 1985) and overlain by deposits associated with the Quaternary Melville Peak volcano (Birkenmajer and Keller, 1990).
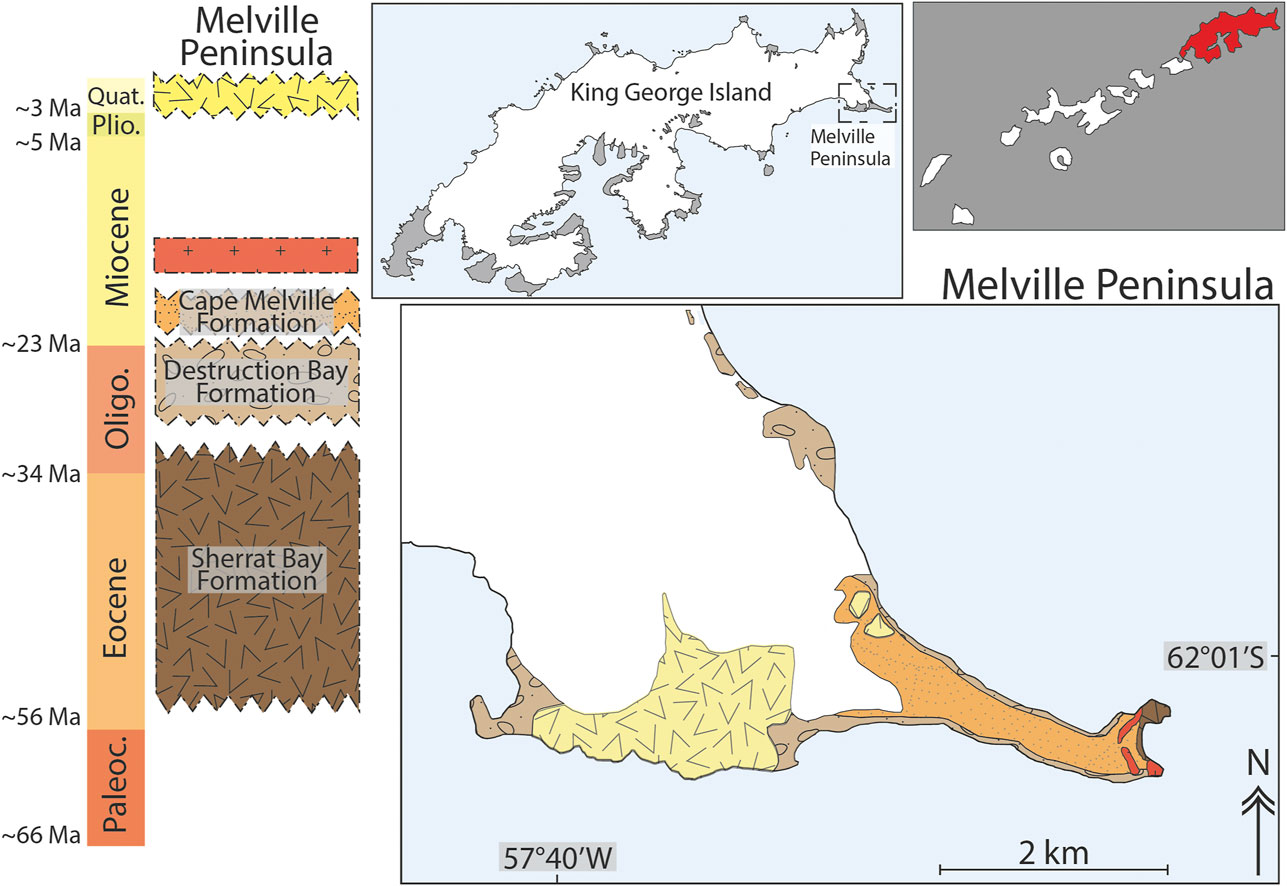
FIGURE 14. Geological map of Melville Peninsula, King George Island, modified from Smellie et al. (2021b) and Warny et al. (2015).
5 Lithostratigraphic correlations
5.1 Deep marine sedimentation: ∼164 (?)—140 Ma
The oldest geological units exposed in the archipelago are Middle to Late Jurassic sedimentary rocks, which crop out on Low and Livingston islands and are represented by the Pencil Beach Member (Low Island) and the Anchorage and Miers Bluff formations (Livingston Island) (Figure 15). These rocks are dominated by mudstones and fine-grained sandstones interbedded with minor ash layers (e.g., Smellie, 1979; Hathway and Lomas, 1998; Bastias et al., 2019), and with fossil assemblages implying a deep-marine setting (Thomson, 1982; Kiessling et al., 1999). While the specific depositional environment is not clear, a turbiditic setting has been proposed (e.g., Hervé et al., 2006). Provenance studies have indicated derivation from a Permian magmatic belt, similar to the Triassic turbidite deposits of the Trinity Peninsula Group on the Antarctic Peninsula (Castillo et al., 2016). While these rocks crop out in the southern and central South Shetland Islands (Figure 15), they may form part of the basement present throughout the archipelago (e.g., Haase et al., 2012). Therefore, their presence suggests that probably most of the South Shetland Islands were under a marine sedimentation environment during the Jurassic.
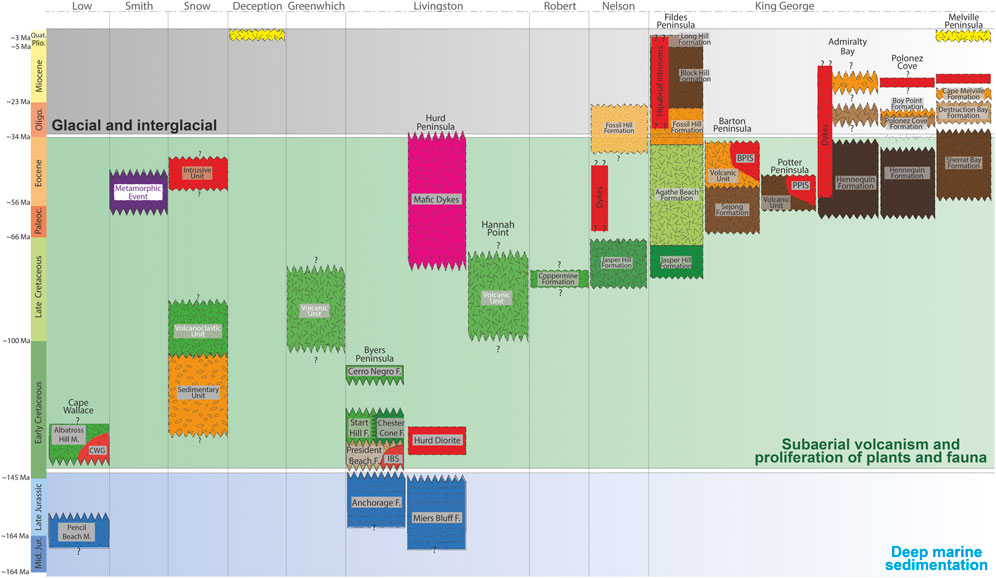
FIGURE 15. Major lithostratigraphic units of the South Shetland Islands. Three main tectonostratigraphic episodes are recognised: 1) deep marine sedimentation from ∼164 to 140 Ma, 2) subaerial volcanism and proliferation of plants and fauna from ∼140 to 35 Ma and 3) glacial and interglacial with sedimentation and volcanism from ∼35 Ma.
5.2 Subaerial volcanism and sedimentation: ∼140–35 Ma
The marine Jurassic successions of Low and Livingston islands are overlain by volcanic and volcaniclastic rocks of continental affinity with an abundant palaeobotanical material and fauna (e.g., Bastias et al., 2019). These two units are separated by an erosional hiatus and provide evidence of a clear change in depositional environment in the South Shetland archipelago. The earliest volcanism is dated at ∼140–137 Ma (Low Island; 40Ar/39Ar in whole-rock; Bastias et al., 2019) and is accompanied by ∼137 Ma acidic plutonism (Livingston and Low islands; Hervé et al., 2006; Bastias et al., 2019). Furthermore, ∼140 Ma volcanism on the South Shetland archipelago links in with the resumption of arc magmatism on the Antarctic Peninsula, which was continuously active throughout the Cretaceous and most of the Cenozoic (e.g., Leat et al., 1995; Riley et al., 2018). Bastias et al. (2022), based on thermochronological studies, suggested that the Antarctic Peninsula experienced rock uplift and erosion during the Early Cretaceous due to the westward migration of South America induced by the opening of the South Atlantic (e.g., Mpodozis and Ramos, 1989). This is in agreement with similar exhumational and erosional processes occurring farther north in the Andes during the Early Cretaceous in Patagonia (Gianni et al., 2018; Gianni et al., 2020), Colombia and Ecuador (Sarmiento and Rangel, 2004; Martin-Gombajov and Winkler, 2008; Villagomez and Spikings, 2013; Spikings et al., 2015). Therefore, the first deposits of subaerial volcanism in the South Shetland Islands may record the early development of the so-called “Andean cycle” in the Chilean-Argentinian Andes, which is responsible for most of the onset of the development of the current Andean range.
The Cretaceous period on Livingston Island is marked by shallow water sedimentation, volcanism, Slope-apron sandstones and conglomerates of the Byers Group (Hathway et al., 1999), and on Low Island it is dominated by volcanic and volcaniclastic rocks (Smellie, 1979; Bastias et al., 2019). Cretaceous volcanic and volcaniclastic rocks are also present on Snow, Greenwich, Robert and King George islands (Figure 15). However, it is noteworthy that several of these successions have not been dated by modern geochronological techniques. Volcanism, minor plutonism and associated deposition of volcaniclastic rocks continued throughout the Palaeocene and Eocene, which is represented by exposures on Livingston and King George islands (Figure 15; e.g., Smellie et al., 1984; Zheng et al., 1991). While the presence of early Cenozoic deposits has not been reported on Low, Snow, Greenwich, Livingston and Robert islands, they may be buried beneath the icecap. The period between ∼140–35 Ma is key for the evolution of life in Antarctica and particularly on the Antarctic Peninsula. The mountainous landscape allowed the development of a complex and diverse palaeobotanical material and fauna that is still providing new paleontological discoveries (e.g., Birkenmajer, 1980; Cao, 1994; Poole et al., 2001; Hunt and Poole, 2003; Warny et al., 2015; Trevisan et al., 2022). Our unified stratigraphy for the South Shetland Islands presented in Figure 15 provides a coherent framework that can serve for further paleontological investigations in the archipelago and the nearby regions.
5.3 Glacial and interglacial with volcanism and sedimentation: ∼35 Ma–recent
The sudden and widespread Cenozoic glaciation of Antarctica is one of the most fundamental reorganisations of global climate known in the Phanerozoic geological record (e.g., Kennett, 1977; Zachos et al., 2001; De Conto and Pollard, 2003; Klages et al., 2020). While there is reasonable consensus on the timing of this rapid and widespread glaciation of Antarctica, there is ongoing debate regarding the causative mechanism responsible for such a change. Two main hypotheses have been posed, the first is related to the Cenozoic opening of sea gateways between Antarctica-Patagonia and Antarctica-Australia, which correspond to the Drake Passage (Barker and Burrell, 1977) and the Tasmanian Passage (Exon et al., 2000). This tectonic event locally changed the organic carbon burial and reduced southward heat transport, cooling the Southern Ocean and the land masses around it (Eagles et al., 2006). The second hypothesis consists of a global decline in atmospheric CO2 (e.g., De Conto and Pollard, 2003) that may be related to the rapid increase in Cenozoic orography (Barbeau et al., 2009; Poblete et al., 2021), the contribution of arc volcanism (Sternai et al., 2020), or an increase of terrestrial and marine organic carbon burial (Galy et al., 2015). Regardless of the responsible mechanism, the rocks that document the onset of this glacial event are exceptionally well exposed in northeast King George Island (Figure 14; e.g., Warny et al., 2015); specifically in Polonez Cove (Figure 14; e.g., Birkenmajer, 1980; Warny et al., 2019a) and on the Melville Peninsula (Figure 13, e.g., Bikenmajer, 2001; Warny et al., 2015). These rocks are mostly comprised of two main sequences, 1) Eocene to Oligocene lava flows and pyroclastics, marine tillites, associated glaciogenic sediments and sandstones and 2) Eocene to Miocene volcano-sedimentary sequences. Research is being currently conducted by a variety of research groups to further understanding of the timing and duration of these glacial events.
5.4 Patagonia and Antarctic Peninsula breakup
While the separation of Patagonia from Antarctic Peninsula leading to the formation of the Drake Passage may have had a profound effect on global circulation and climate during the Cenozoic (e.g., Eagles et al., 2006), it also has relevant repercussions for its relationship with understanding the paleontological evolution of this sector. Furthermore, the timings for the breakup of Patagonia from Antarctic Peninsula have been intensively debated and interpreted to occur during the interval of ∼50 to 30 Ma (e.g., Barker and Burrell, 1977; Livermore et al., 2005; Barbeau et al., 2009; Lagabrielle et al., 2009; Eagles and Jokat, 2014). This tectonic event may be also recorded in the rocks of the South Shetland archipelago, which are one of the closest exposures of the Antarctic Peninsula to Patagonia. Furthermore, Figure 15 also shows that the contemporaneous rocks to the opening of the Drake Passage are located in the northern part of the archipelago; specifically in Livingston, Nelson and King George islands. Thus, better constraints on the geochemistry and the time of formation of these units may be relevant for its potential correlation with the breakup of Patagonia from the Antarctic Peninsula. This information will also help to better understand the faunal and botanical link between the South Shetland Islands and Patagonia, which is usually challenged by the poor age control of the rocks hosting the fossils.
5.5 Smith Island: Age and tectonic implications
The mid-ocean ridge basalts of Smith Island that were metamorphosed under blueschist/greenschist-facies conditions rocks yield an age of ∼58–47 Ma for metamorphism (40Ar/39Ar in white mica; Grunow et al., 1992). While these rocks have been interpreted as part of a subduction complex composed of ocean floor material mixed with arc-derived sediment (Grunow et al., 1992; Truow et al., 1998a), its tectonic implication and protolith age is not clear. Furthermore, Bastias et al. (2022), pointed out that Smith Island resides in the same structural position to the west of the Mesozoic intrusions as elsewhere in the Andes (e.g., the northern Andes; e.g., Spikings et al., 2015). Therefore, it is feasible that these high pressure-low temperature rocks were exhumed during the Early Cretaceous on the west margin of the Antarctic Peninsula by compression associated with the westward migration of South America induced by the opening of the South Atlantic (e.g., Mpodozis and Ramos, 1989), although more work is required (and is ongoing) to investigate the consistency of this hypothesis. Constraining the age of the protolith, formation and exhumation of the rocks in Smith Island is key to understand the tectonic evolution of this sector of the Antarctic Peninsula as it may be connected to major tectonic events.
5.6 Migration of volcanism
A broad geographical trend of decreasing age of volcanism from southwest to northeast has been proposed (Pankhurst and Smellie, 1983; Smellie et al., 1984). The cause of the age migration is unknown. Although a potential explanation has been linked to the rotation of the Antarctic plate (Birkenmajer, 1995), this has been challenged by the presence of Cenozoic dykes in Livingston Island (Willan and Kelley, 1999). Haase et al. (2012) suggested that the volcanic migration proceeded in a series of major steps (i.e., belts), rather than a progressive north-eastern migration. Bastias et al. (2019) reported a southern prolongation of the Early Cretaceous volcanic rocks from Livingston Island (Hervé et al., 2006) to Low Island and suggested the presence of arc belts in the archipelago. Recently, Smellie (2021), suggested that the volcanism occurred in north-easterly-aligned elongate belts that migrated progressively to the south-east and tentatively linked the migration to fore-arc erosion. Nevertheless, the presence of Eocene magmatism in Livingston Island, located in the centre of the archipelago (Willan and Kelley, 1999), challenges any model of progressive migration to the northeast. Figure 15 depicts this issue, where it is possible to observe a younging age trend of volcanism towards the islands located in the north, with the exception of a dyke unit in Livingston Island of early Cenozoic age. Alternatively, while volcanism may have migrated from the southwest to the northeast in the South Shetland archipelago, as it has been suggested (Pankhurst and Smellie, 1983; Smellie et al., 1984), enhanced early Cenozoic volcanism and plutonism may have been developed in most of the archipelago, which interrupted the arc migration. We suggest that this event may be related to the end of the arc magmatism of this region. Furthermore, recently, Burton-Johnson et al. (2022) modelled the waning of the subduction and suggested that most of the Cenozoic magmatism in the Antarctic Peninsula is associated with the progressively younging age of the Phoenix Plate, which is subducted underneath the Antarctic Peninsula. Additionally, this process may have evolved into the development of the Bransfield Strait, a Pliocene marginal back-arc (e.g., Barker, 1982).
6 Conclusion
The South Shetland Islands host Mesozoic to Cenozoic sedimentary, volcanic and volcaniclastic sequences that record three main tectonostratigraphic successions:
1. Deep marine sedimentation from the Middle to Late Jurassic (∼164–140 Ma), present in the centre and south of the archipelago.
2. Subaerial subduction-related volcanism and deposition with proliferation of plants and fauna from the Early Cretaceous to the Eocene/Oligocene boundary (∼140–35 Ma) is present throughout the archipelago.
3. Glacial and interglacial deposits with volcanism and sedimentation from the Oligocene until modern times (from ∼35 Ma), exclusively present on King George Island.
We suggest that a migration in volcanism towards the northeast took place in the South Shetland archipelago from the Late Cretaceous until the early Eocene. This was followed by enhanced volcanism and plutonism from the mid-Eocene until the Miocene. We associate this enhanced magmatic activity to the waning of subduction along the western margin of the South Shetland Islands during the late Cenozoic.
The determination of the protolith, formation and exhumation age of the metamorphic complex that crops out in Smith Island remains an outstanding issue in unravelling the tectonic evolution of the region.
Author contributions
JB, GG, DC, CV, and TR, contributed to conception and design of the study. JB wrote the first draft of the manuscript. GG, TR, and DC reviewed an early version of the manuscript. JM, CT, and ML contributed with paleontological inputs. CV, TR, PC, FP, DT, BL, HC, GZ, YZ, LG, and RC reviewed the local geology. JB, GG, and AR contributed to map developing and methodology review. All authors contributed to manuscript revision, read, and approved the submitted version.
Funding
This research was funded by the Instituto Antártico Chileno (INACH, project RT-06-14) and the University of Geneva. JB. acknowledges support from a PhD-scholarship of ANID-Chile and the Swiss National Science Foundation (project P500PN_202847). DC acknowledges support from Science Foundation Ireland under Grant Number 13/RC/2092 and 13/RC/2092_P2. HC, GZ, YZ, and LG acknowledges support from the National Natural Science Foundation of China (Grant No.41930218 and No. 42076223).
Conflict of interest
The author AR was employed by Cartogrip ScRL.
The remaining authors declare that the research was conducted in the absence of any commercial or financial relationships that could be construed as a potential conflict of interest.
Publisher’s note
All claims expressed in this article are solely those of the authors and do not necessarily represent those of their affiliated organizations, or those of the publisher, the editors and the reviewers. Any product that may be evaluated in this article, or claim that may be made by its manufacturer, is not guaranteed or endorsed by the publisher.
Supplementary material
The Supplementary Material for this article can be found online at: https://www.frontiersin.org/articles/10.3389/feart.2022.1002760/full#supplementary-material
References
Almendros, J., Carmona, E., Jiménez, V., Díaz-Moreno, A., and Lorenzo, F. (2018). Volcano-tectonic activity at deception island volcano following a seismic swarm in the bransfield rift (2014–2015). Geophys. Res. Lett. 45, 4788–4798. doi:10.1029/2018GL077490
Almendros, J., Wilcock, W., Soule, D., Teixidó, T., Vizcaíno, L., Ardanaz, O., et al. (2020). Bravoseis: Geophysical investigation of rifting and volcanism in the Bransfield Strait, Antarctica. J. S. Am. Earth Sci. 104, 102834. doi:10.1016/j.jsames.2020.102834
Araya, R., and Hervé, F. (1966). Estudio geomorfológico y geológico en las Islas Shetland del Sur, Antártica. Publicaciones Inst. Antártico Chil. 8, 1–76.
Armstrong, D. C. (1995). Acid sulphate alteration in a magmatic hydrothermal environment, Barton peninsula, king george island, Antarctica. Mineral Mag. 59, 429–441. doi:10.1180/minmag.1995.059.396.05
Baker, P. F. (2007). “The history of Antarctic Peninsula glacia-tion,”. Open-File Report 20071047, Short Research Paper 042, 5p in Ant-arctica: A keystone in a changing WorldOnline proceedings of the 10th isaes. Editor A. K. Cooper (Santa Barbara, California: USGS). doi:10.3133/0f2007-1047.srp042
Barbeau, D. L., Olivero, E. B., Swanson-Hysell, N. L., Zahid, K. M., Murray, K. E., and Gehrels, G. E. (2009). Detrital-zircon geochronology of the eastern magallanes foreland basin: Implications for Eocene kinematics of the northern scotia arc and Drake passage. Earth Planet. Sci. Lett. 284 (3-4), 489–503. doi:10.1016/j.epsl.2009.05.014
Barker, P. F. (1982). The cenozoic subduction history of the pacific margin of the antarctic peninsula: Ridge crest–trench interactions. J. Geol. Soc. Lond. 139, 787–801. doi:10.1144/gsjgs.139.6.0787
Barker, P. F., and Burrell, J. (1977). The opening of Drake passage. Mar. Geol. 25, 15–34. doi:10.1016/0025-3227(77)90045-7
Barton, C. M. (1965). The geology of the South shetland islands: III. The stratigraphy of king george island. Br. Antarct. Surv. 44, 1–33.
Bastias, J., Calderon, M., Israel, L., Herve, F., Spikings, R., Pankhurst, R., et al. (2019). The Byers Basin: Jurassic-cretaceous tectonic and depositional evolution of the forearc deposits of the South shetland islands and its implications for the northern antarctic peninsula. Int. Geol. Rev. 62, 1467–1484. doi:10.1080/00206814.2019.1655669
Bastias, J., Fuentes, F., Aguirre, L., Hervé, F., Demant, A., Deckart, K., et al. (2016). Very low-grade secondary minerals as indicators of palaeo-hydrothermal systems in the Upper Cretaceous volcanic succession of Hannah Point, Livingston Island, Antarctica. Appl. Clay Sci. 134, 246–256. doi:10.1016/j.clay.2016.07.025
Bastias, J., and Hervé, F. (2013). The Cape Wallace beds: A permian-detritus turbidite unit at low island, South Shetland islands. Boll. Geofis. Teor. Appl. 54, 312–314.
Bastias, J. (2014). Mineralogía y geocronología U-Pb en las Islas Shetland del Sur, Antártica, un multienfoque para Punta Hannah, Isla Livingston y Cabo Wallace, Isla Low (Ms Thesis). Santiago: Geology Department, Universidad de Chile.
Bastias, J., Spikings, R., Riley, T., Chew, D., Grunow, A., Ulianov, A., et al. (2022). Cretaceous magmatism in the Antarctic Peninsula and its tectonic implications. J. Geol. Soc. 180. doi:10.1144/jgs2022-067
Bastías, J., Spikings, R., Riley, T., Ulianov, A., Grunow, A., Chiaradia, M., et al. (2021). A revised interpretation of the chon aike magmatic province: Active margin origin and implications for the opening of the Weddell Sea. Lithos 386-387, 386106013–386106387. doi:10.1016/j.lithos.2021.106013
Bastias, J., Spikings, R., Ulianov, A., Burton-Johnson, A., Chiaradia, M., Baumgartner, L., et al. (2020). The gondwanan margin in west Antarctica: Insights from late triassic magmatism of the antarctic peninsula. Gondwana Res. 81, 1–20. doi:10.1016/j.gr.2019.10.018
Bastos, B. Bastos, B. L., Dutra, T. L., Wilberger, T. P., and Trevisan, C. (2013). Uma flora do final do cretáceo na ilha Nelson, ilhas shetland do sul, península antártica. Rev. Bras. Paleontol. 16 (3), 441–464. doi:10.4072/rbp.2013.3.06
Birkenmajer, K., Gazdzicki, A., Kreuzer, H., and Muller, P. (1985). K- Ar dating of the Melville glaciation (early Miocene) in west Antarctica. Bull. Pol. Acad. Sci. Earth Sci. 33, 1523.
Birkenmajer, K., and Keller, R. A. (1990). Pleistocene age of the peak Melville volcano, king george island, west Antarctica. Bull. Pol. Acad. Sci. Earth Sci. 38, 1724.
Birkenmajer, K. (1995). Basal and intraformational unconformities in lower Oligocene glacigenic deposits (Polonez Cove Formation), king george island, South Shetland islands (west Antarctica). Stud. Geol. Pol. 107, 93–123.
Birkenmajer, K. (1980). Discovery of Pliocene glaciation on king george island, South Shetland islands (west Antarctica). Bull. Pol. Acad. Sci. Earth Sci. 27, 59–67.
Birkenmajer, K., and Gazdzicki, A. (1986). Oligocene age of the pecten conglomerate on king george island, west Antarctica. Bull. Pol. Acad. Sci. Earth Sci. 34, 219–226.
Birkenmajer, K. (1981). Lithostratigraphy of the point Hennequin group (Miocene vulcanics and sediments) at king george islands, Antarctica. Stud. Geol. Pol. 72, 59–73.
Birkenmajer, K. (2001). Mesozoic and cenozoic stratigraphic units in parts of the South shetland islands and northern antarctic peninsula (as used by the polish antarctic programmes). Stud. Geol. Pol. 118, 1–188.
Birkenmajer, K. (1987). Oligocene-miocene glacio-marine sequences of king george island (South Shetland islands), Antarctica. Palaeontol. Pol. 49, 9–36.
Birkenmajer, K. (1982). Pliocene tillite-bearing succession of king george island (South Shetland islands, Antarctica). Stud. Geol. Pol. 74, 7–72.
Birkenmajer, K., and Zastawniak, E. (1989). “Late cretaceous-early tertiaryfloras of king george island, west antarctic: Their stratigraphicdistribution and palaeoclimatic significance,” in Origins and evolution of the antarctic biota. Editor J. A. Crame (London, United Kingdom: The Geological Society,Special Publication), 227–240.
Burton-Johnson, A., Bastias, J., and Kraus, S. (2022). Breaking the Ring of Fire: How ridge collision, slab age, and convergence rate narrowed and terminated the Antarctic continental arc. essopenarchive.
Burton-Johnson, A., and Riley, T. R. (2015). Autochthonous v. accreted terrane development of continental margins: A revised in situ tectonic history of the antarctic peninsula. J. Geol. Soc. Lond. 172, 822–835. doi:10.1144/jgs2014-110
Caballero, M. A., and Fourcade, N. H. (1959). Observaciones Geológicas en Caleta Mina de Cobre, Islas Shetland del Sur. Contribución del Instituto Antártico. Argentino n°23.
Caminos, R., Marchese, H. G., Massabie, A. C., Morelli, J. R., Rinaldi, C. A., and Spikermann, J. P. (1973). Geologia del sector noroccidental de la Peninsula Hurd, Isla Livingston, Shetland del Sur, Antartida Argentina. Contibucion del Inst. Antartico Argent. 162, 32.
Cantrill, D. J. (2000). A cretaceous (aptian) flora from president Head, Snow island, Antarctica. Palaeontographica 253, 153–191. doi:10.1127/palb/253/2000/153
Cantrill, D. J. (1998). Early cretaceous fern foliage from president Head, Snow island, Antarctica. Alcheringa 22, 241–258. doi:10.1080/03115519808619203
Cao, L. (1994). “Late cretaceous palynoflora in king george island of antarctic, with references to its paleoclimatic significance,”in Stratigraphy and palaeontology of Fildes peninsula, king george island, antarctic. Monograph. Editor Y. A. N. B. I. N. SHEN (Beijing: Science Press), 51–83.
Castillo, P., Fanning, C. M., Hervé, F., and Lacassie, J. P. (2016). Characterisation and tracing of Permian magmatism in the south-Western segment of the Gondwanan margin; U–Pb age, Lu–Hf and O isotopic compositions of detrital zircons from metasedimentary complexes of northern Antarctic Peninsula and Western Patagonia. Gondwana Res. 36, 1–13. doi:10.1016/j.gr.2015.07.014
Castillo, P., Lacassie, J. P., Augustsson, C., and Hervé, F. (2015). Petrography and geochemistry of the carboniferous–triassic trinity peninsula group, west Antarctica: Implications for pro-venance and tectonic setting. Geol. Mag. 152, 575–588. doi:10.1017/S0016756814000454
Chen, H., Wei, L. J., Zhao, Y., Liu, X. C., Hu, J. M., Zhang, S. H., et al. (2021). Geological map of the South Shetland islands in west Antarctica (1:250000). Geoscientific Data & Discovery Publishing System. doi:10.35080/data.C.2021.P21
Covacevich, C. V. (1976). Fauna valanginiana de Península Byers, isla Livingston, AntaÂrtica. Rev. GeoloÂgica Chile 3, 25–56.
Crame, J. A., Pirrie, D., Crampton, J. S., and Duane, A. M. (1993). Stratigraphy and regional significance of the upper jurassic-lower cretaceous Byers group, Livingston island, Antarctica. J. Geol. Soc. 150, 1075–1087. doi:10.1144/gsjgs.150.6.1075
Dalziel, I. W. D. (1984). Tectonic evolution of a forearc terrane, southern Scotia Ridge, Antarctica. London, United Kingdom: Geological Society of America Special Paper, 32.
Davies, B. J., Hambrey, M. J., Smellie, J. L., Carrivick, J. L., and Glasser, N. F. (2012). Antarctic peninsula ice sheet evolution during the cenozoic era. Quat. Sci. Rev. 31, 30–66. doi:10.1016/j.quascirev.2011.10.012
Davies, R. E. S. (1982). The geology of the marian Cove area, king george island and tertiary age for its supposed jurassic volcanic rocks. Br. Antarct. Surv. Bull. 51, 151–165.
De Conto, R. M., and Pollard, D. (2003). Rapid Cenozoic glaciation of Antarctica induced by declining atmospheric CO2. Nature 421, 245–249. doi:10.1038/nature01290
Dingle, R. V., and Lavelle, M. (1998). Late cretaceous–cenozoic climatic variations of the northern antarctic peninsula: New geochemical evidence and review. Palaeogeogr. Palaeoclimatol. Palaeoecol. 141, 215–232. doi:10.1016/s0031-0182(98)00056-x
Dingle, R. V., McArthur, J. M., and Vroon, P. (1997). Oligocene and Pliocene interglacial events in the Antarctic Peninsula dated using strontium isotope stratigraphy. J. Geol. Soc. 154, 257–264. doi:10.1144/gsjgs.154.2.0257
Duane, A. M. (1996). Palynology of the Byers group (late jurassic-early cretaceous) of Livingston and Snow islands, antarctic peninsula: Its biostratigraphical and palaeoenvironmental significance. Rev. Palaeobot. Palynology 91, 241–281. doi:10.1016/0034-6667(95)00094-1
Duane, A. M. (1994). Preliminary palynological investigation of the Byers group (late jurassic-early cretaceous), Livingston island, antarctic peninsula. Rev. Palaeobot. Palynology 84, 113–120. doi:10.1016/0034-6667(94)90045-0
Dutra, T. L., and Batten, D. J. (2000). Upper cretaceous floras of king george island, west Antarctica, and their palaeoenvironmental and phytogeographic implications. Cretaceos Reserch 21, 181–209. doi:10.1006/cres.2000.0221
Dutra, T. L. (1997). Primitive leaves of Nothofagus (nothofagaceae) in antarctic peninsula: An upper campanian record and a betulaceous more than fagaceous morphological character. Actas del IX Congr. Geol. Chil. Antofagasta 1, 24–29. (Universidad Catolica del Norte, Antofagasta).
Eagles, G., and Jokat, W. (2014). Tectonic reconstructions for paleobathymetry in Drake passage. Tectonophysics 611, 28–50. doi:10.1016/j.tecto.2013.11.021
Eagles, G., Livermore, R., and Morris, P. (2006). Small basins in the Scotia Sea: The Eocene Drake passage gateway. Earth Planet. Sci. Lett. 242 (3-4), 343–353. doi:10.1016/j.epsl.2005.11.060
Eagles, G. (2004). Tectonic evolution of the Antarctic–phoenix plate system since 15 Ma. Earth Planet Sci. Lett. 217 (1–2), 97–109. doi:10.1016/S0012-821X(03)00584-3
Exon, N., Kennett, J., and Malone, M. (2000). The opening of the Tasmanian gateway drove global Cenozoic paleoclimatic and paleoceanographicLeg 189 Shipboard Scientific Party. JOIDES J. 26 (2), 11–17.
Falcon-Lang, H. J., and Cantrill, D. J. (2002). Terrestrial paleoecology of the cretaceous (early aptian) Cerro Negro Formation, South Shetlands islands, Antarctica: A record of polar vegetation in a volcanic arc environment. Palaios 17 (5), 491–506. doi:10.1669/08831351(2002)017<0491:TPOTCE>2.0.CO;2
Fontes, D., and Dutra, T. L. (2010). Paleogene imbricate-leaved podocarps from king george island (Antarctica): Assessing the geological context and botanical affinities. Rev. Bras. Paleontol. 13 (3), 189–204. doi:10.4072/rbp.2010.3.04
Francis, J. E. (1999). “Evidence from fossil plants for Antarctic Palaeoclimates over the past 100 million years,” in Geological records of global and planetary changes, terra antart. Rep. Editors P. J. Barrett, and G. Orombelli (Siena: Terra Antart. Publication), 43–52.
Galindo-Zaldívar, J., Gamboa, L., Maldonado, A., Nakao, S., and Bochu, Y. (2004). Tectonic development of the bransfield basin and its prolongation to the south scotia ridge, northern Antarctic peninsula. Mar. Geol. 206 (1–4), 267–282. doi:10.1016/j.margeo.2004.02.007
Galy, V., Peucker-Ehrenbrink, B., and Eglinton, T. (2015). Global carbon export from the terrestrial biosphere controlled by erosion. Nature 521, 204–207. doi:10.1038/nature14400
Gao, L., Pei, J., Zhao, Y., Yang, Z., Riley, T. R., Liu, X., et al. (2021). New paleomagnetic constraints on the Cretaceous tectonic framework of the Antarctic Peninsula. J. Geophys. Res. Solid Earth 126 (11), e2021JB022503. doi:10.1029/2021JB022503
Gao, L., Zhao, Y., Yang, Z., Liu, J., Liu, X., Zhang, S. H., et al. (2018). New paleomagnetic and 40Ar/39Ar geochronological results for the South Shetland Islands, West Antarctica, and their tectonic implications. J. Geophys. Res. Solid Earth 123 (1), 4–30. doi:10.1002/2017JB014677
Gazdzicka, E., and Gazdzicki, A. (1985). Oligocene coccoliths of the pecten conglomerate, west Antarctica. Neues Jahrb. für Geol. Paläontologie 12, 727–735. doi:10.1127/njgpm/1985/1986/727
Gazdzicki, A., and Pugaczewska, H. (1984). Biota of the 'pecten conglomerate' (Polonez Cove Formation, Pliocene) of king george island (South Shetland islands, Antarctica). Stud. Geol. Pol. 79, 59–120.
Gerrish, L., Fretwell, P., and Cooper, P. (2021). High resolution vector polygons of the Antarctic coastline (7.4), in UK polar data centre, natural environment research council, UK research & innovation (Cambridgeshire, England: British Antarctic Survey). doi:10.5285/cdeb448d-10de-4e6e-b56b-6a16f7c59095
Geyer, A., Álvarez-Valero, A. M., Gisbert, G., Aulinas, M., Hernández-Barreña, D., Lobo, A., et al. (2019). Deciphering the evolution of Deception Island's magmatic system. Sci. Rep. 9, 373. doi:10.1038/s41598-018-36188-4
Geyer, A., Marti, A., Giralt, S., and Folch, A. (2017). Potential ash impact from antarctic volcanoes: Insights from deception island's most recent eruption. Sci. Rep. 7, 16534. doi:10.1038/s41598-017-16630-9
Ghidella, M. E., Yáñez, G., and LaBrecque, J. L. (2002). Revised tectonic implications for the magnetic anomalies of the Western Weddell Sea. Tectonophysics 347 (1-3), 65–86. doi:10.1016/s0040-1951(01)00238-4
Gianni, G. M., Navarrete, C., Echaurren, A., Díaz, X., and Butler, K. (2020). Northward propagation of andean Genesis: Insights from early cretaceous synorogenic deposits in the aysén-río mayo basin. Gondwana Res. 77 (1), 238–2020259. doi:10.1016/j.gr.2019.07.014
Gianni, G. M., Navarrete, C., Liendo, I., Díaz, M., Giménez, M. E., Encinas, A., et al. (2018). Cretaceous intraplate contraction in southern Patagonia: A far-field response to changing subduction dynamics? Tectonics 37 (9), 2915–2937. doi:10.1029/2018TC005173
Gonzalez-Casado, J. M., Giner-Robles, J. L., and Lopez-Martinez, J. (2000). Bransfield Basin, antarctic peninsula: Not a normal backarc basin. Geology 28, 1043–1046. doi:10.1130/0091-7613(2000)28<1043:bbapna>2.0.co;2
González-Ferran, O., and Katsui, (1970). Estudio integral del volcanismo cenozoico superior de las Islas Shetland del Sur, Antártica. Instituto Antártico Chileno. Ser. Científica 1–2, 123–174.
González-Ferrán, O., Munizaga, F., and Moreno, R. H. (1971). 1970 eruption at deception island: Distribution and chemical features of ejected materials. Antarct. J. U. S. 6, 87–89.
Gouiric-Cavalli, S., Burllaile, L. A., Iglesias, A., Moly, J. J., O'Gorman, J. P., Reguero, M., et al. (2017). Late mesozoic marine antarctic fishes: Future perspectives based on the newly collections recovered in the ameghino and lópez de Bertodano formations. Res. Knowl. 3, 16–21. doi:10.14456/randk.2017.5
Grikurov, G. E., Krylov, A., Polyakov, M. M., and Covbun, N. (1970). Vozrastgornych porod v severnoj casti Antarkticeskogo Poluostrova i na Juznych Setlandskich ostrovach (po dannyum kalij-argonovogo metoda). Inf. Byulleten Sov. Antarkt. Ekspeditsii 80, 30–33.
Grunow, A. M., Dalziel, I. W. D., Harrison, T. M., and Heizler, M. T. (1992). Structural geology and geochronology of subduction complexes along the margin of Gondwanaland: New data from the Antarctic Peninsula and southernmost Andes. Geol. Soc. Am. Bull. 104, 1497–1514. doi:10.1130/0016-7606(1992)104<1497:sgagos>2.3.co;2
Haase, K. M., Beier, C., Fretzdorff, S., Smellie, J. L., and Garbe-Schönberg, D. (2012). Magmatic evolution of the South Shetland Islands, Antarctica, and implications for continental crust formation. Contrib. Mineral. Petrol. 163, 1103–1119. doi:10.1007/s00410-012-0719-7
Hathway, B. (2000). Continental rift to back-arc basin: Jurassic– cretaceous stratigraphical and structural evolution of the larsen basin, antarctic peninsula. J. Geol. Soc. 157, 417–432. doi:10.1144/jgs.157.2.417
Hathway, B., Duane, A. M., Cantrill, D. J., and Kelley, S. P. (1999). 40Ar/39Ar geochronology and palynology of the Cerro Negro Formation, South Shetland islands, Antarctica: A new radiometric tie for cretaceous terrestrial biostratigraphy in the southern hemisphere. Aust. J. Earth Sci. 46 (4), 593–606. doi:10.1046/j.1440-0952.1999.00727.x
Hathway, B., and Lomas, S. A. (1998). The jurassic–lower cretaceous Byers group, South Shetland islands, Antarctica: Revised stratigraphy and regional correlations. Cretac. Res. 19, 43–67. doi:10.1006/cres.1997.0095
Hathway, B. (1997). Nonmarine sedimentation in an early cretaceous extensional continental-margin arc, Byers peninsula, Livingston island, South Shetland islands. J. Sediment. Res. 67, 686–697.
Hawkes, D. D. (1961). The geology of the South Shetland islands: I. The petrology of king George island. Munich, Germany: EGU General Assembly 2021.
Hervé, A. (1965). Estudio geomorfológico y geológico en las Islas Greenwich y Robert. Shetland del Sur, Antártica. Tesis de Prueba. Departamento de Geología, U. de Chile, 1–222.
Hervé, F., Calderón, M., Fanning, C. M., Pankhurst, R. J., and Godoy, E. (2013). Provenance variations in the Late Paleozoic accretionary complex of central Chile as indicated by detrital zircons. Gondwana Res. 23 (3), 1122–1135. doi:10.1016/j.gr.2012.06.016
Hervé, F., Faúndez, V., Brix, M., and Fanning, M. (2006). Jurassic sedimentation of the Miers Bluff Formation, Livingston island, Antarctica: Evidence from SHRIMP U-Pb ages of detrital and plutonic zircons. Antarct. Sci. 18, 229–238. doi:10.1017/s0954102006000277
Hobbs, G. J. (1968). The geology of the South shetland islands: IV. The geology of Livingston island. Br. Antarct. Surv. Sci. Rep. 47, 30.
Hunt, R. J., and Poole, I. (2003). “Paleogene West Antarctic climate and vegetation history in light of new data from King George Island,” in Causes and consequences of globally warm climates in the early paleogene. Boulder, colo-rado. Editors S. L. Wing, P. D. Gingerich, B. Schmitz, and E. Thomas (Geolog Soc Am Spec Pap), 395412.
Hur, S. D., Lee, J. I., Hwang, J., et al. (2001). K-Ar age and geochemistry of hydrothermal alteration in the Barton peninsula, king george island, Antarctica. Ocean Polar Res. 23 (1), 11–21.
Hwang, J., Zheng, X., Ripley, E. M., Lee, J.-I., and Shin, D. (2011). Isotope geochemistry of volcanic rocks from the Barton peninsula, king george island, Antarctica. J. Earth Sci. 22, 40–51. doi:10.1007/s12583-011-0156-y
Ibáñez, J. M., Almendros, J., Carmona, E., Martínez-Arévalo, C., and Abril, M. (2003). The recent seismo-volcanic activity at Deception Island volcano. Deep Sea Res. Part II Top. Stud. Oceanogr. 50, 1611–1629. doi:10.1016/S0967-0645(03)00082-1
Israel, L. (2015). Geología de President Head, isla Snow, archipiélago del Sur, Antártica. Santiago: Geology Department, Universidad de Chile.
Jordan, T. A., Riley, T. R., and Siddoway, C. S. (2020). The geological history and evolution of West Antarctica. Nat. Rev. Earth Environ. 1, 117–133. doi:10.1038/s43017-019-0013-6
Kennett, J. P. (1977). Cenozoic evolution of Antarctic glaciation, the circum-Antarctic Ocean, and their impact on global paleoceanography. J. Geophys. Res. 82, 3843–3860. doi:10.1029/jc082i027p03843
Kiessling, W., Scasso, R., Zeiss, A., Riccardi, A., and andMedina, F. (1999). Combined radiolarian-ammonite stratigraphy for the late jurassic of the antarctic peninsula: Implications for radiolarian stratigraphy. Geodiversitas 21, 687–713.
Kim, H., Lee, J. I., and Choe, M. Y. (2000). Geochronologic evidence for early cretaceous volcanic activity on Barton peninsula, king george island, Antarctica. Polar Res. 19 (2), 251–260. doi:10.3402/polar.v19i2.6549
Klages, J. P., Salzmann, U., Bickert, T., Hillenbrand, C. D., Gohl, K., et al. (2020). Temperate rainforests near the South Pole during peak Cretaceous warmth. Nature 580, 81–86. doi:10.1038/s41586-020-2148-5
König, M., and Jokat, W. (2006). The mesozoic breakup of the Weddell Sea. J. Geophys. Res. 111, B12102. doi:10.1029/2005jb004035
Kraus, S., and del Valle, R. A. (2008). “Geology, tectonics and ar−ar ages of the magmatic dykes frompotter peninsula (king george island, south shetland islands),” in The antarctic ecosystem of potter cove, king−george island(isla 25 de mayo): synopsis of research performed 1999–2006 at the dallmann laboratory andjubany station. Reports on polar and marine research, alfred wegener institute for polar andmarine research. Editors c. Wiencke, g. a. ferreyra, d. Abele, and s. Marenssi (bremerhaven, 20–30.
Kraus, S. (2005). Magmatic dyke systems of the South Shetland islands volcanic arc (west Antarctica): Reflections of the geodynamic history. PhD thesis published online (München, Germany: Munich University Library), 160.
Lagabrielle, Y., Goddéris, Y., Donnadieu, Y., Malavieille, J., and Suarez, M. (2009). The tectonic history of Drake Passage and its possible impacts on global climate. Earth Planet. Sci. Lett. 279 (3-4), 197–211. doi:10.1016/j.epsl.2008.12.037
Larter, R. D., and Barker, P. F. (1991). Effects of ridge–crest trench interaction on antarctic phoenix spreading: Forces on a young subducting plate. J.Geophys. Res. 96, 19583–19607. doi:10.1029/91jb02053
Lawver, L. A., Keller, R. A., Fisk, M. R., and Strelin, J. (1995). “Bransfield Strait, Antarctic Peninsula active extension behind a dead arc,” in Backarc basins: Tectonics and magmatism. Editor B. Taylor (New York: Plenum Press), 315–342.
Lawver, L. A., Gahagan, L. M., and Coffin, M. F. (1992). in The antarctic paleoenvironment: A perspective on global change. Editor J. P. D. A. Kennett&Warnke (Washington DC: American Geophysical Union).
Leat, P. T., and Riley, T. R. (2021). “Chapter 3.1a antarctic peninsula and south Shetland islands: Volcanology,” in Volcanism in Antarctica: 200 million years of subduction, rifting and continental break-up. Editors J. L. Smellie, K. S. Panter, and A. Geyer (London, United Kingdom: Geological Society of London Memoir), 55.
Leat, P. T., Scarrow, J. H., and Millar, I. L. (1995). On the antarctic peninsula batholith. Geol. Mag. 132, 399–412. doi:10.1017/s0016756800021464
Lee, J. I., Hur, S. D., Yoo, C. M., Ueo, J. P., Kim, H., Hwang, J., et al. (2002). Geological map of Barton and weaver peninsulas, king George island, Antarctica. Ansan: Korea Ocean Research and Development In-stitute, 30.
Lee, J. I., Hwang, J., Kim, H., et al. (1996). Subvolcanic zoned granitic pluton in the Barton and weaver peninsulas, king george island, Antarctica. Proc. NIPR Symposium Antarct. Geosciences 9, 76–90.
Leppe, M., Michea, W., Muñoz, C., Palma-Heldt, S., and Fernandoy, F. (2007). “Paleobotany of Livingston island: The first report of a cretaceous fossil flora from Hannah point,” in Antarctica: A keystone in a changing World. U.S. Geological survey and the national academies. Editors A. K. Cooper, and C. R. Raymond (USGS). OF-2007-1407 Short Research Paper 081. doi:10.3133/of2007-1047.srp081
Li, H. (1994). “Early tertiary fossil Hill flora from Fildes peninsula of king george island, Antarctica,” in Stratigraphy and palaeontology of Fildes peninsula, king george island, Antarctica. Editor Y. SHEN (Beijing: Science Press), 133–171.
Li, H. M., and Shen, Y. B. (1990). A primary study of fossil Hill flora from Fildes peninsula of king george island, antarctic (in Chinese with English abstract). Acta Palaeontol. Sin. 2, 147–197.
Li, Z., Lui, X., Zheng, X., Jin, Q., and Li, G. (1989). “Tertiary volcanism and formation of volcanic rocks in the Fildes peninsula, king george island, antartic,” in Proceedings of the international symposium on antarctic research. Editor K. Guo (Ocean, 114–118.
Li, Z. N., and Liu, X. H. (1987). Geological characteristics of the volcanic rock series in the great wall station area, Fildes peninsula, king george island, Antarctica (in Chinese with English abstract). Geol. Rev. 33 (5), 45–47.
Li, Z. N., Zheng, X. S., Liu, X. H., Jin, Q. M., Li, G., and Shang, R. X. (1996). Geological map of the the Fildes peninsula, king George island and stansbury peninsula (in Chinese with English abstract). Antarctica: Geological House.
Li, Z. N., Zheng, X. S., Liu, X. H., Shang, X. R., Jin, Q. M., and Wang, B. X. (1992). Volcanic rocks of the Fildes peninsula, king George island. West AntarcticaBeijing: Science Press, 1–227.
Liebrand, D., Lourens, L. J., Hodell, D. A., de Boer, B., van de Wal, R. S. W., and Palike, H. (2011). Antarctic ice sheet and oceanographic response to eccentricity forcing during the early Miocene. Clim. Past. 7, 869–880. doi:10.5194/cp-7-869-2011
Liu, X. H., and Zheng, X. S. (1988). Geology of volcanic rocks on Fildes peninsula, king george island, west Antarctica (in Chinese with English abstract). Antarct. Res. 1 (1), 25–35.
Livermore, R., Nankivell, A., Eagles, G., and Morris, P. (2005). Paleogene opening of Drake passage. Earth Planet. Sci. Lett. 236 (1-2), 459–470. doi:10.1016/j.epsl.2005.03.027
Lomas, S. A. (1999). A lower cretaceous clastic slope succession, Livingston island, Antarctica: Sand-body characteristics, depositional processes and implications for slope apron depositional models. Sedimentology 46, 477–504. doi:10.1046/j.1365-3091.1999.00225.x
Luzón, F., Almendros, J., and García-Jerez, A. (2011). Shallow structure of Deception Island, Antarctica, from correlations of ambient seismic noise on a set of dense seismic arrays. Geophys. J. Int. 185, 737–748. doi:10.1111/j.1365-246X.2011.04962.x
Machado, A., Lima, E. F., Chemale, F., Morata, D., Oteiza, O., Almeida, D. P. M., et al. (2005). Geochemistry constraints of mesozoic–cenozoic calc-alkaline magmatism in the South shetland arc, Antarctica. J. S. Am. Earth Sci. 18 (3), 407–425. doi:10.1016/j.jsames.2004.11.011
Machado, A. (1997). Petrologia, geoquímica e geologia estrutural da Península Fildes. Ilha Rei George, Antártica (M.Sc. thesis. Brazil: Federal University of Rio Grande do Sul, 182.
Maestro, A., Somoza, L., Rey, J., Martínez-Frias, J., and Lopez-Martínez, J. (2007). Active tectonics, fault patterns, and stress field of deception island: A response to oblique convergence between the pacific and antarctic plates. J. South Am. Earth Sci. 23 (2–3), 256–268. doi:10.1016/j.jsames.2006.09.023
Manfroi, J., Dutra, T. L., Gnaedinger, S. C., Uhl, D., and Jasper, A. (2015). The first report of a Campanian palaeo-wildfire in the west Antarctic Peninsula. Palaeogeogr. Palaeoclimatol. Palaeoecol. 418, 12–18. doi:10.1016/j.palaeo.2014.11.012
Manfroi, J., Dutra, T. L., Trevisan, T., Jasper, A., Aquino, F. E., and Leppe, M. (2022). Eventos de incêndios florestais durante o Cretáceo registrados na ilha Rei George, Península Antártica. Paleontol. em Destaque 37, 45.
Mansilla, H., Stinnesbeck, W., Verela, N., and Leppe, M. (2012). New avian tracks from the lower to middle Eocene at fossil Hill, king george island, Antarctica. Antarct. Sci. 24 (5), 500–506. doi:10.1017/s0954102012000260
Mansilla, H., Valais, S., Stinnesbeck, W., Verela, N., and Leppe, M. (2013). Eocene fossil feather from king george island, South Shetland islands, Antarctica. Antarct. Sci. 26, 384–388. –5. doi:10.1017/S0954102013000771
Martin-Gombojav, N., and Winkler, W. (2008). Recycling of proterozoic crust in the andean amazon foreland of Ecuador: Implications for orogenic development of the northern Andes. Terra nova. 20, 22–31. doi:10.1111/j.1365-3121.2007.00782.x
Mpodozis, C., and Ramos, V. A. (1989). “The Andes of Chile and Argentina,” in Geology of theAndes and its relation to hydrocarbon and mineral resources. Editors G. E. Ericksen, M. T. Cañas Punochet, and J. A. Reinemund (Houston: Circum-Pacific for Energy and Mineral Resources Earth Sciencies Series), 59–90.
Muñoz, A., Sàbat, F., and Pallàs, R. (1992). “Estructura pre- Cretácica de la Peninsula Hurd, Isla Livingston, Islas Shetland del Sur,” in Geología de la Antártida Occidental. Simposios T3. III congreso geológico de España y VIII congreso latinoamericano de Geología. Editor J. LÓPEZMARTÍNEZ (Salamanca, España, 127–139.
Nawrocki, J., Panczyk, M., Wójcik, K., and K Ozlowska-Roman, A. (2021a). Age of volcanism on keller peninsula and assessment of age-constrained volcanic activity on king George island, South Shetland islands. Polish Polar Research. (in press).
Nawrocki, J., Pańczyk, M., Wójcik, K., and Tatur, A. (2021b). U–Pb isotopic ages and provenance of some far-travelled exotic pebbles from glaciogenic sediments of the Polonez Cove Formation (Oligocene, King George Island). J. Geol. Soc. 178 (2). doi:10.1144/jgs2020-113
Orheim, O. (1972). A 200-year record of glacier mass balance at Deception Island, southwest Atlantic Ocean, and its bearing on models of global climate change. Ohio: Institute of PolarStudies, Ohio State University, 118.
Pallas, R., Coriano, C. A., Zheng, X., Sábat, F., and Casas, J. M. (1999). Volcanic stratigraphy of Hannah point. South Shetland Islands, Antarctica: Livingston Island.
Pankhurst, R. J., Riley, T. R., Fanning, C. M., and Kelley, S. P. (2000). Episodic silicic volcanism in Patagonia and the antarctic peninsula: Chronology of magmatism associated with the breakup of Gondwana. J. Petrol. 41, 605–625. doi:10.1093/petrology/41.5.605
Pankhurst, R. J., and Smellie, J. L. (1983). K/Ar geochronology of the South shetland islands, lesser Antarctica: Apparent lateral migration of jurassic to quaternary island arc volcanism. Earth Planet. Sci. Lett. 66, 214–222. doi:10.1016/0012-821x(83)90137-1
Pankhurst, R. J., Weaver, S. D., Brook, M., and Saunders, A. D. (1979). K/Ar chronology of Byers peninsula, Livingston island, South Shetland islands. Br. Antarct. Surv. Bull. 49, 277–282.
Park, B. K. (1989). Potassium-Argon radiometric ages of volcanic and plutonic rocks from the Barton peninsula, king george island, Antarctica. J. Geol. Soc. Korea 25 (4), 495–497.
Philippe, M., Torres, T., Barale, G., and Thevenard, F. (1995). President Head, Snow island, South Shetland, a key-point for antárctica mesozoic palaeobotany. C.R. Acad. Sci. París 321.
Pimpirev, C., Ivanov, M., Dimov, D., and Nikolov, T. (2002). First find of the upper tithonian ammonite genus Blanfordiceras from the Miers Bluff Formation, Livingston island, South Shetland islands. Neues Jahrb. für Geol. Monatsh. 2002, 377–384. doi:10.1127/njgpm/2002/2002/377
Pimpirev, C., Stoykova, K., Ivanov, M., and Dimov, V. (2006). “The Miers Bluff Formation, Livingston island, South Shetland islands-part of the late jurassic-cretaceous depositional history of the antarctic peninsula,” in Antarctica: Contributions to global earth sciences. Editors D. K. Futterer, D. Damaske, G. Kleinschmidt, H. Miller, and F. Tessensohn (Berlin: Springer), 249–254.
Pirrie, D., and Crame, J. A. (1995). Late Jurassic palaeogeography and anaerobic-dysaerobic sedimentation in the northern Antarctic Peninsula región. J. Geol. Soc. 152, 469–480. doi:10.1144/gsjgs.152.3.0469
Poblete, F., Dupont-Nivet, G., Licht, A., Van Hinsbergen, D. J., Roperch, P., Mihalynuk, M. G., et al. (2021). Towards interactive global paleogeographic maps, new reconstructions at 60, 40 and 20 Ma. Earth-Science Rev. 214, 103508. doi:10.1016/j.earscirev.2021.103508
Poole, I., Hunt, R. J., and Cantrill, D. J. (2001). A fossil wood flora from king george island: Ecological implications for an ant-arctic Eocene vegetation. Ann. Bot. 88, 33–54. doi:10.1006/anbo.2001.1425
Quaglio, F., Anelli, L. E., dos Santos, P. R., de Perinotto, J. A., and Rocha-Campos, A. C. (2008). Invertebrates from the low Head member (Polonez Cove Formation, Oligocene) at vauréal peak, king george island, west Antarctica. Antarct. Sci. 20, 149–168. doi:10.1017/s0954102007000867
Quaglio, F., Warren, L. V., Anelli, L. E., dos Santos, P. R., Rocha-Campos, A. C., Gazdzicki, A., et al. (2014). Shell beds from the low Head member (Polonez Cove Formation, early Oligocene) at king george island, west Antarctica: New insights on facies analysis, taphonomy and environmental significanceficance. Antarct. Sci. 26, 400–412. doi:10.1017/s0954102013000783
Riley, T. R., Burton-Johnson, A., Flowerdew, M. J., and Whitehouse, M. J. (2018). Episodicity within a mid-cretaceous magmatic flare-up in west Antarctica: U–Pb ages of the lassiter coast intrusive suite, antarctic peninsula, and correlations along the Gondwana margin. Geol. Soc. Am. Bull. 130, 1177–1196. doi:10.1130/B31800.1
Riley, T. R., Flowerdew, M. J., and Whitehouse, M. J. (2012)., 169. London, 381–393. doi:10.1144/0016-76492011-142U–Pb ionmicroprobe zircon geochronology from the basement inliers of eastern Graham Land, Antarctic PeninsulaJ. Geol. Soc.
Riley, T. R., Leat, P. T., Pankhurst, R. J., and Harris, C. (2001). Origins of large volume rhyolitic volcanism in the antarctic peninsula and Patagonia by crustal melting. J. Petrol. 42, 1043–1065. doi:10.1093/petrology/42.6.1043
Robertson Maurice, S. D., Wiens, D. A., Shore, P. J., Vera, E., and Dorman, L. M. (2003). Seismicity and tectonics of the South shetland islands and Bransfield Strait from a regional broadband seismograph deployment. J. Geophys Res. 108. doi:10.1029/2003JB002416
Roobol, M. J. (1982). The volcanic hazard at deception island, South Shetland islands. Br. Antarct. Surv. Bull. 51, 237–245.
Santos, A., Piovezan, K., Guzmán, J., Usma, C. D., Weinschutz, L. C., Santos, R. J. M., et al. (2022). Special volume on paleontology at the AABC. Acad. Bras. Cienc. 94, e202294s3. doi:10.1590/0001-37652022202294s3
Sarmiento, L. F., and Rangel, A. (2004). Petroleumsystems of the upper magdalena valley, Colombia. Mar. Petroleum Geol. 21, 373–391. doi:10.1016/j.marpetgeo.2003.11.019
Shen, Y. (1994). “Cretaceous to paleogene biostratigraphy in antarctic peninsula and its signifi cance in the reconstruction of gondwanaland,” in Stratigraphy and palaeontology of Fildes peninsula, king george island, Antarctica. Editor Y. Yanbin (Beijing, China: Science Press), 329–348. (Monograph 3).
Smellie, J. L. (2021). Antarctic volcanism: Volcanology and palaeoenvironmental overview. London, Memoirs: Geological Society. doi:10.1144/M55-2020-1
Smellie, J. L., Davies, R. E. S., and Thomson, M. R. A. (1980). Geology of a mesozoic intra-arc sequence on Byers peninsula, Livingston island, South Shetland islands. Br. Antarct. Surv. Bull. 50, 55–76.
Smellie, J. L., Hunt, R. J., Mcintosh, W. C., and Esser, R. P. (2021a). Lithostratigraphy, age and distribution of Eocene volcanic sequences on eastern king george island, South Shetland islands, Antarctica. Antarct. Sci. 33, 373–401. (in press). doi:10.1017/s0954102021000213
Smellie, J. L., Liesa, M., Muñoz, J. A., Sabat, F., Pallas, R., and Willan, R. C. R. (1995). Lithostratigraphy of volcanic and sedimentary sequences in central Livingston island, South Shetland islands. Antarct. Sci. 7, 99–113. doi:10.1017/s0954102095000137
Smellie, J. L. (2001). Lithostratigraphy and volcanic evolution of deception island, South Shetland islands. Antarct. Sci. 13 (2), 188–209. doi:10.1017/S0954102001000281
Smellie, J. L., López-Martínez, J., Headland, R. K., Hernández-Cifuentes, F., Maestro, A., Millar, I. L., et al. (2002). “Geology and geomorphology of deception island,” in BAS GEOMAP Series, Sheets 6-A and 6-B, 1:25 000,78pp. with accompanying maps (Cambridge): British Antarctic Survey).
Smellie, J. L., McIntosh, W. C., Whittle, R., Troedson, A., and Hunt, R. J. (2021b). A lithostratigraphical and chronological study of Oligocene-Miocene sequences on eastern King George Island, South Shetland Islands (Antarctica), and correlation of glacial episodes with global isotope events. Antarct. Sci. 33 (5), 502–532. doi:10.1017/s095410202100033x
Smellie, J. L., Millar, I. L., Rex, D. C., and Butterworth, P. J. (1998). Subaqueous, basaltic lava dome and carapace breccia on king george island, South Shetland islands, Antarctica. Bull. Volcanol. 59, 245–261. doi:10.1007/s004450050189
Smellie, J. L., Pankhurst, R. J., Thomson, M. R. A., and Davies, R. E. S. (1984). The geology of the South shetland islands: VI stratigraphy, geochemistry and evolution. Br. Antarct. Surv. Sci. Rep. 87, 85pp.
Smellie, J. L., Panter, K. S., and Reindel, J. (2021c). “Mt early and sheridan bluf.I. Volcanology,” in Volcanism in Antarctica: 200 million years of subduction, rifting and continental break-up. Editors J. L. Smellie, K. S. Panter, and A. Geyer (London, United Kingdom: Geology Society London), 491–498.
Smellie, J. L. (1980). The geology of low island, South Shetland islands and austin rocks. Br. Antarct. Surv. Bull. 49, 239–257.
Smellie, J. L. (1979). The geology of low island, South Shetland islands, and austin rocks. Br. Antarct. Surv. 49, 239–257.
So, C. S., Yun, S. T., and Park, M. E. (1995). Geochemistry of a fossil hydrothermal system at Barton peninsula, king george island. Antarct. Sci. 7 (1), 63–72. doi:10.1017/s0954102095000101
Song, Z. C. (1998). Research on tertiary palynoflora from the fossil Hill Formation of king george island, antarctic (in Chinese with English abstract). Acta Micropalaeontological Sin. 15 (4), 335–350.
Spikings, R., Cochrane, R., Villagomez, D., Van der Lelij, R., Vallejo, C., Winkler, W., et al. (2015). The geological history of northwestern south America: From pangaea to the early collision of the caribbean large igneous province (290–75 Ma). Gondwana Res. 27, 95–139. doi:10.1016/j.gr.2014.06.004
Sternai, P., Caricchi, L., Pasquero, C., Garzanti, E., Hinsbergen, D. J. J., and Castelltort, S. (2020). Magmatic forcing of cenozoic climate? J. Geophys. Res. Solid Earth 125. doi:10.1029/2018JB016460
Thomson, M. R. A. (1982). Late jurassic fossils from low island, South Shetland islands. Br. Antarct. Surv. Bull. 56, 25–35.
Tokarski, A., Danowski, W., and Zastawniak, E. (1987). On the age of fossil flora from Barton peninsula, king george island, west Antarctica. Pol. Polar Res. 8, 293–302.
Torres, T., Barale, G., Méon, H., Philippe, M., and Thévenard, F. (1997). “Cretaceous floras from Snow island (South Shetland islands, Antarctica) and their biostratigraphic significance,” in The antarctic region: Geological evolution and processes. Editor C. A. Ricci (Terra Antarctica Publication Siena), 1023–1028.
Torres, T., Bastias, J., Otero, R., and Calderón, M. (2015). “Nuevos registros de Araucariáceas y Podocarpáceas del Cretácico Superior de Punta Hannah, Isla Livingston, Archipiélago de las Shetland del Sur,” in XIV Congreso Geológico Chileno, La Serena, Antártica, October 2015.
Trevisan, C., Dutra, T., Ianuzzi, R., Sander, A., Wilberger, T., Manríquez, L., et al. (2022). Coniopteris Antarctica sp. nov.(pteridophyta) and associated plant assemblage from the upper cretaceous of Rip point, Nelson island, Antarctica. Cretac. Res. 136, 105185. doi:10.1016/j.cretres.2022.105185
Troedson, A. L., and Riding, J. B. (2002). Upper Oligocene to lower-most Miocene strata of king george island, south shet-land islands, Antarctica: Stratigraphy, facies analysis, and implications for the glacial history of the antarctic peninsula. J. Sediment. Res. 72 (4), 510–523. doi:10.1306/110601720510
Troedson, A. L., and Smellie, J. L. (2002). The Polonez Cove Formation of king george island, Antarctica: Stratigraphy, facies and implications for mid-cenozoic cryosphere development. Sedimentology 49, 277–301. doi:10.1046/j.1365-3091.2002.00441.x
Trouw, R. A. J., Simoes, L. S. A., and Valladares, C. S. (1998a). Metamorphic evolution of a subduction complex, South Shetland islands, Antarctica. J. Metamorph. Geol. 16, 475–490. doi:10.1111/j.1525-1314.1998.00151.x
Trouw, R. A. J., Simoes, L. S. A., and Valladares, C. S. (1998b). Variation in mineral chemical composition across a subduction complex with Sanbagawa-type metamorphism. South Shetland Islands. Antarctica: Pesquisa Antartica Brasileira Academia Brasileira de Ciencias.
Ugalde, R., Torres, T., Israel, L., Galleguillos, M., Hervé, F., and Fanning, C. M. (2013). New geological data and paleontological records of president Head peninsula, Snow island, South Shetland islands, Antarctica: A key for the lower cretaceous. Bollettino di Geofisica Teorica Apl. 54, 359.
Valencio, A., Mendía, E., and Vilas, J. (1979). Palaeomagnetism and K-Ar age of Mesozoic and Cenozoic igneous rocks from Antarctica. Earth Planet. Sci. Lett. 45, 61–68. doi:10.1016/0012-821X(79)90107-9
Valenzuela, E., and Hervé, F. (1972). “Geology of Byers peninsula, Livingston island, South Shetland islands,” in Antarctic geology and geophysics. Editor R. J. Adie (Oslo: Universitetsforlaget), 83–89.
Valeriano, C. M., Heilbron, M., and Trouw, R. A. J. (1997). “Lithogeochemistry of mafic rocks from the scotia metamorphic complex in the South shetland islands: Evidence for ocean floor basalts as protoliths,” in The antarctic region: Geological evolution and processes. 390–400. Editor C. A. Ricci (Siena, Italy: Tipografia Senese).
Vera, E. I. (2010). A new specimen of Millerocaulis (osmundales: Osmundaceae) from the Cerro Negro Formation (lower cretaceous), Antarctica. Rev. del Mus. Argent. Ciencias Nat. 12 (1), 41–46. doi:10.22179/revmacn.12.250
Vera, E. I. (2012). Millerocaulis tekelili sp. nov., a new species of osmundalean fern from the Aptian Cerro Negro Formation (Antarctica). Alcheringa Australas. J. Palaeontol. 36 (1), 35–45. doi:10.1080/03115518.2011.576541
Villagómez, D., and Spikings, R. (2013). Thermochronology and tectonics of the central and western cordilleras of Colombia: Early cretaceous–tertiary evolution of the north-ern Andes. Lithos 160-161, 228–249. doi:10.1016/j.lithos.2012.12.008
Villanueva, C. (2021). Caracterización geocronológica, petrográfica y Geoquímica del volcanismo de arco durante el Cretácico superior en la Península Coppermine, Isla Robert, Antártica: Migración del magmatismo versus Arco continuo. Santiago: Departamento de Geología, Universidad Santo Tomás.
Wang, F., Zheng, X.-S., Lee, J. I. K., Choe, W. H., Evans, N., and Zhu, R.-X. (2009). An 40Ar/39Ar geochronology on a mid-Eocene igneous event on the Barton and Weaver peninsulas: Implications for the dynamic setting of the Antarctic Peninsula. Geochem. Geophys. Geosystems 10 (12). doi:10.1029/2009gc002874
Warny, S., Kymes, C. M., Askin, R., Krajewki, K. P., and Tatura, A. (2019a). Terrestrial and marine floral response to latest Eocene and Oligocene events on the antarctic peninsulafloral response to latest Eocene and Oligocene events on the antarctic peninsula. Palynology 43, 4–21. doi:10.1080/01916122.2017.1418444
Warny, S., Kymes, C. M., Askin, R., Krajewski, K. P., and Tatur, A. (2019b). “Terrestrial and marine floral response to latest Eocene and Oligocene events on the antarctic peninsula. Palynology, 43, watts, D. R. 1982. Potassium-Argon ages and palaeomagnetic results from king george island, South Shetland islands,” in Antarctic geoscience. Editor C. CRADDOCK (Madison, Wisconsin: Univ. Wisc. Press), 255–261.
Warny, Y. S., Kymes, C. M., Askin, R. A., Krajewski, K. P., and Bart, P. J. (2015). Remnants of antarctic vegetation on king george island during the early Miocene Melville glaciation. Palynology 39 (3), 66–82. doi:10.1080/01916122.2014.999954
Whittle, R. J., Quaglio, F., Griffiths, H. J., Linse, K., and Crame, J. A. (2014). The Early Miocene Cape Melville Formation fossil assemblage and the evolution of modern Antarctic marine communities. Naturwissenschaften 101, 47–59. doi:10.1007/s00114-013-1128-0
Willan, R. C. R., and Armstrong, D. C. (2002). Successive geothermal, volcanic-hydrothermal and contact-metasomatic events in cenozoic volcanic-arc basalts, South Shetland islands, Antarctica. Geol. Mag. 139, 209–231. doi:10.1017/s0016756802006301
Willan, R. C. R., and Kelley, S. P. (1999). Mafic dike swarms in the South Shetland Islands volcanic arc: Unravelling multiepisodic magmatism related to subduction and continental rifting. J. Geophys. Res. Solid Earth 104 (B10), 23051–23068. doi:10.1029/1999jb900180
Willan, R. C. R., Pankhurst, R. J., and Hervé, F. (1994). A probable early triassic age for the Miers Bluff Formation, Livingston island, South Shetland islands. Antarct. Sci. 6, 401–408. doi:10.1017/s095410209400060x
Yeo, J. P., Lee, J. L., Hur, S. D., and Choi, B. G. (2004). Geochemistry of volcanic rocks in Barton and weaver peninsulas, king george island, Antarctica: Implications for arc maturity and correlation with fossilized volcanic centers. Geoscience J. 8 (1), 11–25. doi:10.1007/bf02910275
Yoo, C. M., Choe, M. Y., Jo, H. R., Kim, Y., and Kim, K. H. (2001). Volcaniclastic sedimentation of the Sejong Formation (late paleocene-eocene), Barton peninsula, king george island, Antarctica. Ocean Polar Res. 23, 97–107.
Zachos, J., Pagani, M., Sloan, L., Thomas, E., and Billups, K. (2001). Trends, rhythms, and aberrations in global climate 65Ma to present. Science 292, 686–693. doi:10.1126/science.1059412
Zhao, Y., Liu, X., Zhang, S., Zhang, J., Pei, J., Gao, L., et al. (2019). “The final subduction of the Phoenix Plate beneath the antarctic peninsula: A prelude to the opening of the deep Drake passage oceanic gateway,” in International symposium on antarctic earth science (ISAES 2019 22-26 july 2019) (Republic of Korea: Incheon).
Zheng, X., Kamenov, B., Sang, H., and Monchev, P. (2003). New radiometric dating of the dykes from the Hurd peninsula, Livingston island, South Shetland islands. J. South Am. Earth Sci. 15, 925–934. doi:10.1016/s0895-9811(02)00146-3
Zheng, X., Sang, H., Qiu, J., Liu, J., Lee, J, I., and Kim, H. (2000). New discovery of the early cretaceous volcanic rocks on the Barton peninsula, king george island, Antarctica and its geological significance. Acta Geol. Sin. 74, 176–182. doi:10.1111/j.1755-6724.2000.tb00446.x
Zheng, X. S., E, M. L., Liu, X. H., Zhu, M., and Li, J. J. (1991). The volcanic geology, petrological characteristics and the formation and evolution of the tertiary volcanic rocks from the great wall station area, king george island, west antarctic (in Chinese with English abstract). Antarct. Res. 3 (2), 10–108.
Keywords: Antarctica, climate change, antarctic glaciation, geology, stratigraphy, paleobotany
Citation: Bastías J, Chew D, Villanueva C, Riley T, Manfroi J, Trevisan C, Leppe M, Castillo P, Poblete F, Tetzner D, Giuliani G, López B, Chen H, Zheng G-G, Zhao Y, Gao L, Rauch A and Jaña R (2023) The South Shetland Islands, Antarctica: Lithostratigraphy and geological map. Front. Earth Sci. 10:1002760. doi: 10.3389/feart.2022.1002760
Received: 25 July 2022; Accepted: 23 December 2022;
Published: 12 January 2023.
Edited by:
Lawrence H. Tanner, Le Moyne College, United StatesReviewed by:
Graeme Eagles, Alfred Wegener Institute Helmholtz Centre for Polar and Marine Research (AWI), GermanyAdriane Machado, Federal University of Sergipe, Brazil
Eduardo B. Olivero, CONICET Centro Austral de Investigaciones Científicas (CADIC), Argentina
Copyright © 2023 Bastías, Chew, Villanueva, Riley, Manfroi, Trevisan, Leppe, Castillo, Poblete, Tetzner, Giuliani, López, Chen, Zheng, Zhao, Gao, Rauch and Jaña. This is an open-access article distributed under the terms of the Creative Commons Attribution License (CC BY). The use, distribution or reproduction in other forums is permitted, provided the original author(s) and the copyright owner(s) are credited and that the original publication in this journal is cited, in accordance with accepted academic practice. No use, distribution or reproduction is permitted which does not comply with these terms.
*Correspondence: Joaquín Bastías, ai5iYXN0aWFzLnNpbHZhQGdtYWlsLmNvbQ==