- 1Technology Center, China Tobacco Yunnan Industrial Company Limited, Kunming, Yunnan, China
- 2College of Chemistry and Chemical Engineering, Kunming University of Science and Technology, Kunming, Yunnan, China
Volatile organic chemicals (VOCs) released from e-cigarettes are a special source of air pollutants. In this work, we investigated the VOCs released from six nicotine salts (namely, nicotine benzoate, nicotine tartrate, nicotine citrate, nicotine malate, nicotine lactate, and nicotine levulinate) that are commonly used in e-cigarettes. The pyrolysis-gas chromatography/mass spectrometry (Py-GC/MS) and thermogravimetric methods were used to analyze the thermogravimetric characteristics and product release behavior of different nicotine salts. Moreover, the kinetic models and thermodynamic parameters of nicotine salts during the thermal decomposition process were obtained. Thermogravimetric characteristic parameters of six nicotine salts showed significant differences. By the use of Py-GC/MS, our data showed that the pyrolysis products of nicotine salts were mainly from nicotine, acid anhydrides, carboxylic acids, and N-heterocycles, while more than 90% of the nicotine of citrate, tartrate, and malate was transferred to smoke. The result revealed that activation energies of the nicotine salts range from 21.26 to 74.10 kJ mol-1, indicating that the pyrolysis of the nicotine salts is a non-spontaneous heat absorption process, and the organic acid was the key factor affecting the release of nicotine into the ambient air.
1 Introduction
Environmental tobacco smoke is one of the special air pollutants released by the incomplete combustion of tobacco products (Kavouras et al., 1998; Brauer et al., 2000; Zhu et al., 2022). The volatile organic chemicals (VOCs) from e-cigarettes and other tobacco products is one of the air pollutants that contain many harmful chemicals to human health (Mueller et al., 2011; Schober et al., 2014; Chen et al., 2018; Schober et al., 2019). It is reported that thousands of chemicals can be found in tobacco smoke, many of which are known to be harmful; breathing even small amounts of tobacco smoke can be harmful for humans (Kitamura and Kasai, 2007; Rogers, 2008; Fagerström, 2011). It is well known that tobacco smoking could cause many diseases, including cancer, heart disease, stroke, diabetes, and other chronic diseases (Eyre et al., 2004; Halpin et al., 2010; Stanton et al., 2016). Researchers should face up to the fact that although the law tightens anti-smoking restrictions in many countries, smoking is still allowed in some special smoking areas (Louka et al., 2006). Gaining insights into tobacco smoke would be useful for understanding the properties of smoking behavior. Chemical analysis is one of the powerful analytical strategies for understanding tobacco smoke at the molecular level (Borgerding and Klus, 2005; Orr, 2014; Jiang, 2020). The existence mode and thermal stability of nicotine have a direct impact on its transfer efficiency into smoke. For example, it is reported that nicotine carboxylic acid salts can be transferred to the gas phase via three mechanisms: deprotonation, carboxylic acid anion decomposition, and disproportionation in the lower temperature (Elias and Ling, 2018). Additionally, nicotine salts are more stable, more soluble in water, and less volatile than regular nicotine. As a result, the creation of nicotine salts and their thermal stability have become hot topics in tobacco research (Riggs and Perfetti, 2001; Jasiewicz et al., 2014; Yang et al., 2019). Different types of nicotine salts are usually added into e-cigarettes to change the smoke, resulting in different pyrolysis reactions in the heating process of the e-cigarette. Moreover, pyrolysis kinetic parameters will affect the reactant selection and the reaction process operating conditions (Çepelioğullar et al., 2016). Therefore, kinetic analysis and thermodynamic properties would help understand the pyrolysis products of nicotine salts from e-cigarettes (Cardoso et al., 2011), many of which are mainly volatile organic compounds (VOCs).
Mass spectrometry is a powerful tool in various application fields and has been successfully used for investigating the pollution in chemistry, environmental science, and human health (Daughton, 2001). To date, various mass spectrometry methods have advanced many applications in environmental analysis (Richardson, 2001). Many environmental applications of various MS approaches have focused on air studies. These include investigations and measurements of aerosols, particulates, and VOCs (Richardson, 2001; Cai et al., 2021; Chen et al., 2022; Hu, 2022). Pyrolysis-gas chromatography/mass spectrometry (Py-GC/MS) is one of the powerful MS methods for chemical analysis in which the sample is heated to decomposition to produce smaller molecules. Py-GC/MS has brought significant advances in the field of environmental analysis. By the use of Py-GC/MS, the decomposition products are separated using the GC system and detected using the MS approach. Py-GC/MS has been successfully used for the investigation of semi-volatile and volatile air pollutants (Gregoris et al., 2023).
In this work, we investigated the volatile pyrolysis products released from six typical nicotine salts (namely, nicotine benzoate, nicotine tartrate, nicotine citrate, nicotine malate, nicotine lactate, and nicotine levulinate) that are commonly used in e-cigarettes by using Py-GC/MS and thermogravimetric methods. The thermogravimetric characteristics and product release behavior of different nicotine salts were analyzed. Moreover, the kinetic models and thermodynamic parameters were also obtained. Overall, our results showed that thermal properties and kinetic analysis would help understand the pyrolysis products of nicotine salts from e-cigarettes, highlighting the potential impact of e-cigarettes on the air environment.
2 Materials and methods
2.1 Chemicals and materials
Ethanol was purchased from Sichuan Xilong Chemical Co., Ltd. (Sichuan, China). The nicotine salts were purchased from Nanjing Jingge Chemical Technology Co., Ltd., whose purity was greater than 99%, and the basic physical properties are shown in Supplementary Table S1.
2.2 Thermogravimetric analysis
Thermogravimetric analysis was carried out using a TGA/DSC 1 LF (Mettler Toledo, Germany) instrument. Samples (10.0 ± 0.2 mg) were placed in alumina crucibles. An empty alumina crucible was used as the reference. Nicotine salts were heated from an ambient temperature to 350°C in a 20 mL/min flow of air at heating rates of 10°C/min. Continuous recordings of sample temperature, sample weight, and heat flow were made.
2.3 Pyrolysis-GC/MS
Each of the nicotine salts was formulated into a solution with a concentration of about 10 mg/mL using chromatographic grade absolute ethanol as a solvent; then, 1.0 μL of the solution was added to the quartz tube, and pyrolysis was performed using a Pyroprobe 6200 (CDS Analytical, United States) pyrolyzer. The sample was heated from room temperature to 350°C at a rate of 30°C s-1 under an air atmosphere, while the temperature of the GC oven was raised from 40°C to 240°C at a rate of 10°C min-1 and then to 280°C at a rate of 20°C/min, and the shunt ratio was 100: 1. The Agilent DB-5 MS capillary column with a column length of 30 m, column ID of 0.25 mm, and thickness of 0.25 m was used. The MS was set to the following parameters: 230°C ion source temperature, 280°C transmission line temperature, 29–450 amu mass scanning range, and 2.5 min solvent delay. The chromatograms obtained from the analysis were analyzed using Agilent (NIST 17.0) software after they had been run.
2.4 Kinetic analysis
The kinetics of most reactions under non-isothermal heterogeneous conditions can be summarized by Eq. 1:
where T is the temperature, K(T) is the temperature relationship for reaction rate constants, and f(α) is the reaction mechanism function. The heating rate β was defined as follows:
The Arrhenius equation is
where T is the temperature, A is the pre-exponential factor, E is the activation energy, and R is the universal gas constant (8.314 J (mol·K)−1). Equations 1, 2 were combined into f (α) and the following equation was derived:
An integral function was defined as follows:
Equations 4, 5 were combined togive the following equation:
The most probable degradation mechanism can be determined by the Coats–Redfern approximation (Coats and Redfern, 1964), and applying Eq. 6, the following equation was derived:
Since 2RT/E << 1 in Eq. 7, Eq. 4 can be simplified as
Here, f(α) corresponds to different expressions according to different control mechanisms, as shown in Supplementary Table S1, and different reaction mechanism functions were brought into formula (8); ln [G(α)/T2] and (1/T) showed a linear relationship, and E and A can be calculated by fitting the linear curve. Furthermore, the relevant thermodynamic parameters (ΔH, ΔG, and ΔS) during the thermal decomposition of samples can be calculated (Huang et al., 2013) from Eqs 9–11.
where T is the peak temperature, ΔH is enthalpy, ΔG is Gibbs free energy, and ΔS is the entropy change. KB is the Boltzmann constant (1.3806 × 10–23 J K−1), and h is Planck’s constant (6.6261 × 10–34 J·s).
3 Results and discussion
3.1 Pyrolysis analysis
Figure 1 shows the results of qualitative and semi-quantitative analyses of the volatile components of six nicotine salts under low-temperature heating conditions. In general, the thermal decomposition products of nicotine salts did not undergo more complex reactions at low temperatures, such as polymerization and condensation, so the cracking product components were relatively simple. However, there were differences between the cracking products of different nicotine salts; the released substances were primarily nicotine, anhydrides, carboxylic acids, N-heterocycles, and other compounds. Organic acids were detected in nicotine lactate, nicotine benzoate, and nicotine levulinate, which are thermal decomposition products as listed in Supplementary Table S2, while the organic acids in the remaining nicotine salts were cleaved into small molecules.
3.2 Thermogravimetric analysis
Figure 2 epicts the TG and DTG curves of six nicotine salts at a heating rate of 10°C min-1. The temperature corresponding to the maximum weight loss rate of nicotine levulinate and nicotine lactate was 169.34°C and 181.27°C, respectively. For the remaining nicotine salts, two pyrolysis stages were observed. The temperature corresponding to the maximum weight loss rate was 234.39°C for nicotine benzoate, 206.02°C for nicotine tartrate, 178.55°C for nicotine citrate, and 199.07°C for nicotine malate. The temperature needed to achieve the maximum weight loss rate was the lowest for nicotine levulinate and the highest for nicotine benzoate; the temperature needed to begin pyrolysis was the lowest for nicotine benzoate at 66.72°C and the highest for nicotine lactate at 142.10°C; and the temperature needed to complete pyrolysis was the lowest for nicotine levulinate at 181.93°C and the highest for nicotine benzoate at 256.65°C.
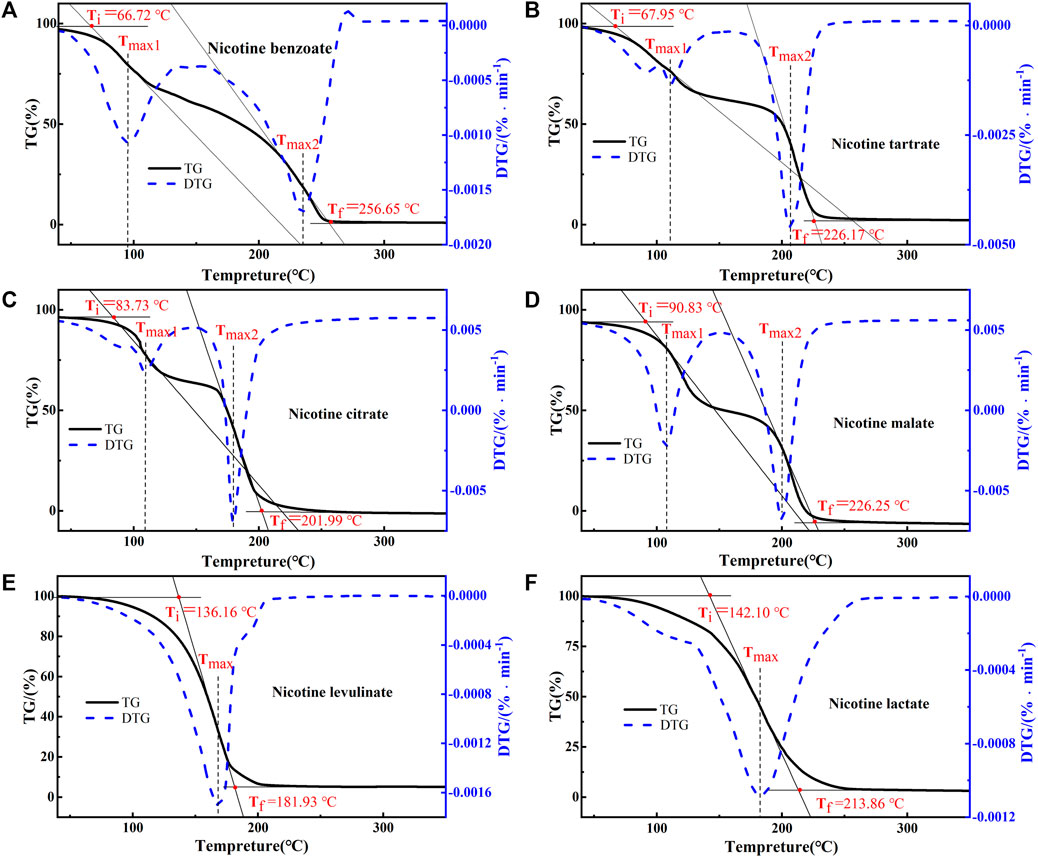
FIGURE 2. TG–DTG curves of six nicotine salts: (A) nicotine benzoate, (B) nicotine tartrate, (C) nicotine citrate, (D) nicotine malate, (E) nicotine levulinate, and (F) nicotine lactate.
The combination of the aforementioned analysis shows that the nicotinic salts of levulinate and benzoate require higher temperatures for initiation and complete decomposition, have a wider range of release temperatures and relatively slower release rates, and exhibit better thermal stability. The main thermal decomposition temperature of nicotine ranges from 80°C to 180°C; in this study, the nicotine salts released nicotine at a temperature between 150°C and 260°C, where the greater temperature range indicates that the thermal stability of nicotine salt is stronger than that of nicotine itself. It can be introduced that the aforementioned nicotine salt is transferred to the e-cigarette aerosol in the proton state of nicotine in the environment of e-cigarette smoked temperature below 150 °C. As a result, adding nicotine salts to e-cigarettes produces a softer and smoother smoking experience rather than directly adding nicotine, with a weaker sense of throat hit.
3.3 Kinetic analysis of different nicotine salts
From Figure 3, it can be seen that all kinetic models showed a regression coefficient (R2) greater than 0.9, and these can be considered best fitted models. In region Ⅰ, the reaction model for nicotine lactate was a one-dimensional diffusion-controlled model (D1), and the reaction model for the rest of the nicotine salts was a 1.5-order chemical reaction-controlled model (F1.5); in region Ⅱ, the reaction model for nicotine salts was a two-order chemical reaction-controlled model (F2). The different kinetic models of nicotine salts at each region indicate the complexity of the thermal decomposition process, whereas lower average activation energy values represent that the lower the energy barrier that must be overcome for the reaction to occur, the easier it is for the nicotine salt to begin decomposing when heated. It follows that nicotine benzoate was the first to undergo thermal decomposition, and conversely, nicotine lactate was the latest to begin thermal decomposition. A larger index front factor indicates a faster reaction rate of the sample at the same temperature; therefore, nicotine benzoate and nicotine citrate required the lowest temperature to reach the maximum weight loss rate in the first and second stages, respectively, and the aforementioned kinetic analysis was consistent with the thermogravimetric conclusion.
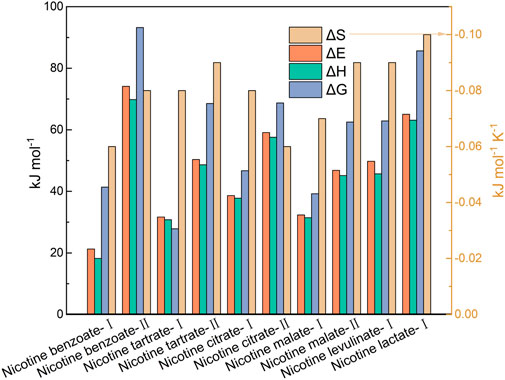
FIGURE 3. Comparison of thermokinetic parameters of different nicotine salts in the main thermal decomposition stages.
Further calculation and comparison of the thermodynamic parameters of each nicotine salt sample at the main decomposition stages show that the enthalpy changes (ΔH) of the nicotine salts were all positive and increased with the increase in the system temperature, indicating that the nicotine salt pyrolysis is a heat-absorbing reaction. Nicotine salts’ enthalpy changes varied between 18.21 and 63.14 kJ mol-1 in region Ⅰand 45.16 and 69.82 kJ mol-1 in region Ⅱ. The difference between the enthalpy change and activation energy of nicotine citrate was the smallest, indicating that it was more conducive to the formation of activation complexes. The enthalpy change was proportional to the activation energy E), and the difference was less than 5 kJ mol-1. The Gibbs free energy (ΔG) is a measure of the increase in total energy of the reaction system that occurs during the formation of the activation complex (Sriram and Swaminathan, 2018). The higher the entropy change (ΔS), the faster the formation of an activation complex and the further away the reaction system is from thermodynamic equilibrium. According to Supplementary Table S4, nicotine lactate has the best thermal stability and the highest ΔG of 85.65 kJ mol-1, suggesting that it requires the most energy to pyrolyze. In conclusion, it can be demonstrated that the thermal degradation of nicotine salts is not a spontaneous reaction of heat absorption.
4 Conclusion
In this work, our results showed that main pyrolysis products of nicotine salts are nicotine, acid anhydrides, carboxylic acids, N-heterocycles, and other compounds, and more than 90% of the nicotine of citrate, tartrate, and malate was transferred to smoke. These pyrolysis products of nicotine salts could be the source of air pollutants that were released into the ambient air environment. These transferred and converted products of nicotine under the heating condition could provide new indicators for monitoring the air pollutants in e-cigarettes. Moreover, the kinetic models and thermodynamic parameters of nicotine salts showed that there were significant differences in the thermogravimetric characteristic parameters. Our data also indicated that the pyrolysis of the nicotine salts is a non-spontaneous heat absorption process.
Data availability statement
The raw data supporting the conclusion of this article will be made available by the authors, without undue reservation.
Author contributions
All authors listed have made a substantial, direct, and intellectual contribution to the work and approved it for publication.
Funding
This study received funding from the Science and Technology Project of China Tobacco Yunnan Industrial Co., Ltd. (Nos 2020GY03 and 2021JC05). The funder was not involved in the study design, collection, analysis, interpretation of data, the writing of this article, or the decision to submit it for publication.
Conflict of interest
Authors JY, YL, CL, ST, and ZL were employed by the company China Tobacco Yunnan Industrial Company Limited.
The remaining author declares that the research was conducted in the absence of any commercial or financial relationships that could be construed as a potential conflict of interest.
Publisher’s note
All claims expressed in this article are solely those of the authors and do not necessarily represent those of their affiliated organizations, or those of the publisher, the editors, and the reviewers. Any product that may be evaluated in this article, or claim that may be made by its manufacturer, is not guaranteed or endorsed by the publisher.
Supplementary material
The Supplementary Material for this article can be found online at: https://www.frontiersin.org/articles/10.3389/fenvs.2023.1145056/full#supplementary-material
References
Borgerding, M., and Klus, H. (2005). Analysis of complex mixtures–cigarette smoke. Exp. Toxicol. Pathology 57, 43–73. doi:10.1016/j.etp.2005.05.010
Brauer, M., Hirtle, R., Lang, B., and Ott, W. (2000). Assessment of indoor fine aerosol contributions from environmental tobacco smoke and cooking with a portable nephelometer. J. Expo. Sci. Environ. Epidemiol. 10 (2), 136–144. doi:10.1038/sj.jea.7500076
Cai, S.-H., Di, D., Yuan, Z.-C., Chen, W., and Hu, B. (2021). Paper-in-Facemask device for direct mass spectrometry analysis of human respiratory aerosols and environmental exposures via wearable continuous-flow adsorptive sampling: A proof-of-concept study. Anal. Chem. 93 (41), 13743–13748. doi:10.1021/acs.analchem.1c03406
Cardoso, C. R., Miranda, M. R., Santos, K. G., and Ataíde, C. H. (2011). Determination of kinetic parameters and analytical pyrolysis of tobacco waste and sorghum bagasse. J. Anal. Appl. Pyrolysis 92 (2), 392–400. doi:10.1016/j.jaap.2011.07.013
Çepelioğullar, Ö., Haykırı-Açma, H., and Yaman, S. (2016). Kinetic modelling of RDF pyrolysis: Model-fitting and model-free approaches. Waste Manag. 48, 275–284. doi:10.1016/j.wasman.2015.11.027
Chen, R., Aherrera, A., Isichei, C., Olmedo, P., Jarmul, S., Cohen, J. E., et al. (2018). Assessment of indoor air quality at an electronic cigarette (Vaping) convention. J. Expo. Sci. Environ. Epidemiol. 28 (6), 522–529. doi:10.1038/s41370-017-0005-x
Chen, W., Zou, Y., Mo, W., Di, D., Wang, B., Wu, M., et al. (2022). Onsite identification and spatial distribution of air pollutants using a drone-based solid-phase microextraction array coupled with portable gas chromatography-mass spectrometry via continuous-airflow sampling. Environ. Sci. Technol. 56 (23), 17100–17107. doi:10.1021/acs.est.2c05259
Coats, A. W., and Redfern, J. P. (1964). Kinetic parameters from thermogravimetric data. Nature 201 (4914), 68–69. doi:10.1038/201068a0
Daughton, C. G. (2001). Emerging pollutants, and communicating the science of environmental chemistry and mass spectrometry: Pharmaceuticals in the environment. J. Am. Soc. Mass Spectrom. 12 (10), 1067–1076. doi:10.1016/s1044-0305(01)00287-2
Elias, J., and Ling, P. M. (2018). Invisible smoke: Third-party endorsement and the resurrection of heat-not-burn tobacco products. Tob. Control 27 (1), s96–s101. doi:10.1136/tobaccocontrol-2018-054433
Eyre, H., Kahn, R., and Robertson, R. M.American Cancer Society, the American Diabetes Association, and the American Heart Association.Collaborative Writing Committee (2004). Preventing cancer, cardiovascular disease, and diabetes: A common agenda for the American cancer society, the American diabetes association, and the American heart association. Circulation 109 (25), 3244–3255. doi:10.1161/01.cir.0000133321.00456.00
Fagerström, K. (2011). Determinants of tobacco use and renaming the FTND to the Fagerström test for cigarette dependence. Nicotine Tob. Res. 14 (1), 75–78. doi:10.1093/ntr/ntr137
Gregoris, E., Gallo, G., Rosso, B., Piazza, R., Corami, F., and Gambaro, A. (2023). Microplastics analysis: Can we carry out a polymeric characterisation of atmospheric aerosol using direct inlet py-GC/MS? J. Anal. Appl. Pyrolysis 170, 105903. doi:10.1016/j.jaap.2023.105903
Halpin, H. A., Morales-Suárez-Varela, M. M., and Martin-Moreno, J. M. (2010). Chronic disease prevention and the new public health. Public Health Rev. 32 (1), 120–154. doi:10.1007/bf03391595
Hu, B. (2022). Recent advances in facemask devices for in vivo sampling of human exhaled breath aerosols and inhalable environmental exposures. TrAC Trends Anal. Chem. 151, 116600. doi:10.1016/j.trac.2022.116600
Huang, M., Lv, S., and Zhou, C. (2013). Thermal decomposition kinetics of glycine in nitrogen atmosphere. Thermochim. Acta 552, 60–64. doi:10.1016/j.tca.2012.11.006
Jasiewicz, B., Mrówczyńska, L., and Malczewska-Jaskóła, K. (2014). Synthesis and haemolytic activity of novel salts made of nicotine alkaloids and bile acids. Bioorg. Med. Chem. Lett. 24 (4), 1104–1107. doi:10.1016/j.bmcl.2014.01.005
Jiang, H. (2020). Investigation of carcinogenic compounds in tobacco smoke. Int. J. Pharma Med. Biol. Sci. 9 (3), 140–143. doi:10.18178/ijpmbs.9.3.140-143
Kavouras, I. G., Stratigakis, N., and Stephanou, E. G. (1998). Iso- and anteiso-alkanes: Specific tracers of environmental tobacco smoke in indoor and outdoor particle-size distributed urban aerosols. Environ. Sci. Technol. 32 (10), 1369–1377. doi:10.1021/es970634e
Kitamura, M., and Kasai, A. (2007). Cigarette smoke as a trigger for the dioxin receptor-mediated signaling pathway. Cancer Lett. 252 (2), 184–194. doi:10.1016/j.canlet.2006.11.015
Louka, P., Maguire, M., Evans, P., and Worrell, M. (2006). ‘I think that it’sa pain in the ass that I have to stand outside in the cold and have a cigarette’ representations of smoking and experiences of disapproval in UK and Greek smokers. J. Health Psychol. 11 (3), 441–451. doi:10.1177/1359105306063317
Mueller, D., Uibel, S., Braun, M., Klingelhoefer, D., Takemura, M., and Groneberg, D. A. (2011). Tobacco smoke particles and indoor air quality (ToPIQ) - the protocol of a new study. J. Occup. Med. Toxicol. 6, 35. doi:10.1186/1745-6673-6-35
Orr, M. S. (2014). Electronic cigarettes in the USA: A summary of available toxicology data and suggestions for the future: Table 1. Tob. control 23 (2), ii18–ii22. doi:10.1136/tobaccocontrol-2013-051474
Richardson, S. D. (2001). Mass spectrometry in environmental sciences. Chem. Rev. 101 (2), 211–254. doi:10.1021/cr990090u
Riggs, D., and Perfetti, T. (2001). Thermochemical properties of nicotine salts. Contributions Tob. Nicotine Res. 19 (6), 289–295. doi:10.2478/cttr-2013-0715
Rogers, J. M. (2008). Tobacco and pregnancy: Overview of exposures and effects. Birth Defects Res. Part C Embryo Today Rev. 84 (1), 1–15. doi:10.1002/bdrc.20119
Schober, W., Fembacher, L., Frenzen, A., and Fromme, H. (2019). Passive exposure to pollutants from conventional cigarettes and new electronic smoking devices (IQOS, e-cigarette) in passenger cars. Int. J. Hyg. Environ. Health 222 (3), 486–493. doi:10.1016/j.ijheh.2019.01.003
Schober, W., Szendrei, K., Matzen, W., Osiander-Fuchs, H., Heitmann, D., Schettgen, T., et al. (2014). Use of electronic cigarettes (e-cigarettes) impairs indoor air quality and increases FeNO levels of e-cigarette consumers. Int. J. Hyg. Environ. Health 217 (6), 628–637. doi:10.1016/j.ijheh.2013.11.003
Sriram, A., and Swaminathan, G. (2018). Pyrolysis of Musa balbisiana flower petal using thermogravimetric studies. Bioresour. Technol. 265, 236–246. doi:10.1016/j.biortech.2018.05.043
Stanton, C. A., Keith, D. R., Gaalema, D. E., Bunn, J. Y., Doogan, N. J., Redner, R., et al. (2016). Trends in tobacco use among US adults with chronic health conditions: National Survey on Drug Use and Health 2005–2013. Prev. Med. 92, 160–168. doi:10.1016/j.ypmed.2016.04.008
Yang, J., Chen, Y., Liu, Z., Yang, L., Tang, J., Miao, M., et al. (2019). Differences between the binding modes of enantiomers S/R-nicotine to acetylcholinesterase. RSC Adv. 9 (3), 1428–1440. doi:10.1039/c8ra09963d
Keywords: nicotine salts, volatile organic compounds, Py-GC/MS, pyrolysis products, kinetic analysis
Citation: Yang J, Li Y, Liu C, Tang S, Li Z and Jiang L (2023) Thermal properties and kinetic analysis of pyrolysis products of nicotine salts from e-cigarettes using pyrolysis-gas chromatography/mass spectrometry. Front. Environ. Sci. 11:1145056. doi: 10.3389/fenvs.2023.1145056
Received: 15 January 2023; Accepted: 20 March 2023;
Published: 12 April 2023.
Edited by:
Hemi Luan, Guangdong University of Technology, ChinaReviewed by:
Wenfen Zhang, Zhengzhou University, ChinaChi Yang, Distinguished Professor of Nanjing University of Information Science and Technology, China
Copyright © 2023 Yang, Li, Liu, Tang, Li and Jiang. This is an open-access article distributed under the terms of the Creative Commons Attribution License (CC BY). The use, distribution or reproduction in other forums is permitted, provided the original author(s) and the copyright owner(s) are credited and that the original publication in this journal is cited, in accordance with accepted academic practice. No use, distribution or reproduction is permitted which does not comply with these terms.
*Correspondence: Ji Yang, eWFuZ2ppMDUyQDE2My5jb20=; Lihong Jiang, amxoNjVAMTYzLmNvbQ==